ABSTRACT
RFWD3 is an E3 ubiquitin ligase that plays important roles in DNA damage response and DNA replication. We have previously demonstrated that the stabilization of RFWD3 by PCNA at the replication fork enables ubiquitination of the single-stranded binding protein, RPA and its subsequent degradation for replication progression. Here, we report that RFWD3 associates with the Origin Recognition Complex (ORC) and ORC-Associated (ORCA/LRWD1), components of the pre-replicative complex required for the initiation of DNA replication. Overexpression of ORC/ORCA leads to the stabilization of RFWD3. Interestingly, RFWD3 seems to stabilize ORC/ORCA in cells expressing wild type p53, as the depletion of RFWD3 reduces the levels of ORC/ORCA. Further, the catalytic activity of RFWD3 is required for the stabilization of ORC. Our results indicate that the RFWD3 promotes the stability of ORC, enabling efficient pre-RC assembly.
Introduction
Accurate genome duplication and faithful segregation to daughter cells are critical for the maintenance of genome stability. During G1 phase of the cell cycle, the Origin recognition complex (ORC), along with ORC-associated protein (ORCA/LRWD1), bind to the origins of replication and serve as the landing pad for the establishment of the pre-replicative complex (pre-RC). Origin licensing involves the recruitment of MCM2-7 that encircles the origins of replication. As cells enter S phase, the “origin firing” is achieved through the activation of the CMG helicase (Cdc45, MCM2-7 and GINS complex) by the cell cycle dependent kinases [Citation1,Citation2,Citation3,Citation8] Insufficient origin licensing leads to replication stress as cells enter S phase, which eventually leads to genome instability [Citation9,Citation10]. The depletion of pre-RC components, including Cdc6, Cdt1, and Orc2 in normal human cells has been shown to activate origin licensing checkpoint and consequently G1 arrest [Citation11–13]. The depletion of ORCA in human fibroblast and human embryonic stem cells leads to G1 arrest [Citation5]. Interestingly, p53-deficient cells do not show similar G1 arrest phenotype, suggesting that p53 is crucial in the activation of origin licensing checkpoint [Citation13]. Many factors can influence this checkpoint. The overexpression of cyclin E perturbs MCM loading which leads to accelerated S phase entry [Citation14,Citation15]. The overexpression of c-Myc also leads to increased replication origin activity [Citation16].
The dynamics of ORC/ORCA are tightly regulated throughout the cell cycle. The changes in ORC/ORCA protein levels or their association with chromatin is one of the mechanisms to avoid inappropriate origin licensing. Cellular levels of the pre-RC, including ORC, ORCA, Cdc6, Cdt1, are controlled, largely governed by posttranslational modifications including phosphorylation and ubiquitination [Citation4,Citation17,Citation18]. In yeast, the phosphorylation of ORC does not affect its association with the chromatin, but prevents its ability to form the pre-RC [Citation19,Citation20,Citation21]. In Drosophila, Orc1, the largest subunit of ORC, is ubiquitinated and degraded by anaphase-promoting complex [Citation22]. In hamster cells, the dissociation of Orc1 from the chromatin is achieved through ubiquitination during S phase and Cdk1/cyclin A-dependent phosphorylation during G2/M [Citation23,Citation24]. In human cells, the protein level of Orc1 and ORCA are cell-cycle regulated, while the level of ORC2-5 remains stable throughout the cell cycle. During G1 phase, RIF1-PP1 complex protects Orc1 from inappropriate phosphorylation and degradation [Citation25]. During S phase, Orc1 is phosphorylated by S phase CDKs, and degraded by the Skp1-culin-F box (SCF) ubiquitin ligase complex [Citation26,Citation27]. Similarly, the protein level of ORCA, and its association with chromatin, are cell-cycle regulated [Citation5]. ORCA is known to be ubiquitinated at the G1/S boundary [Citation28]. More recently, the ubiquitination of Orc3 and Orc5 by ORC-ubiquitin-ligase-1 (OBI1) was shown to be crucial for origin firing [Citation29]. However, the entire repertoire of E3 ligases that control DNA replication initiation and S-phase progression remains far from complete.
The RING finger and WD repeat domain containing protein 3 (RFWD3) has been identified as a crucial player in DNA damage response. RFWD3 was initially identified as ATM/ATR substrate [Citation30,Citation31]. Previous studies have shown that RFWD3 stabilizes p53 upon ionizing radiation through ubiquitination [Citation32]. In response to replication stress, RFWD3 is recruited to the replication fork by RPA [Citation33]. At the stalled fork, RFWD3 ubiquitinates RPA and RAD51, which facilitates homologous recombination [Citation34,Citation35]. In addition to homologous recombination, RFWD3 participates in DNA interstrand crosslinks (ICLs) [Citation36]. Biallelic mutations of RFWD3 have been discovered in Fanconi anemia (FA) patient, suggesting that RFWD3 is crucial for the FA/BRCA pathway [Citation37]. Under unperturbed conditions, RFWD3 at the replication fork is stabilized by PCNA, which enables the ubiquitination of RPA by RFWD3, and ensures the timely removal of RPA for successful fork progression [Citation38].
In the present study, we have identified RFWD3 as an ORC-interacting protein. Overexpression of ORC and ORCA stabilizes cellular levels of RFWD3 in a p53-dependent manner. RFWD3 directly interacts with Orc1 and Orc2 and can ubiquitinate ORCA. The depletion of RFWD3 leads to a p53-dependent decrease of ORC/ORCA levels. We propose that RFWD3 associates with ORC and stabilizes it in a p53-dependent manner.
Material and methods
Cell culture and RNA interference
U2OS cells were grown in Dulbecco’s modified Eagle medium (DMEM) containing high glucose, supplemented with penicillin-streptomycin and 5% fetal bovine serum (FBS) (Hyclone). HEK293T cells were grown in DMEM containing high glucose, supplemented with penicillin-streptomycin and 10% heat-inactivated FBS. HCC38 cells were grown in RPMI medium supplemented with penicillin-streptomycin and 10% FBS. U2OS cells stably expressing HA-RFWD3 were generated as previously described [Citation38]. HCT116 cells were grown in McCoy’s medium containing high glucose and supplemented with 10% FBS. The U2OS-2-6-3 cells were cultured as described [Citation5,Citation39].
Lipofectamine 2000 was used for transient transfection as per the manufacturer’s protocols. Lipofectamine RNAiMAX (Invitrogen) was used for siRNA delivery. The final concentration of siRNA is 100 nM.
The siRNA oligos used in this study were synthesized by Sigma or IDT. The siRNA sequences/source are:
siRFWD3 #1: 5'-GGACCUACUUGCAAACUAU-3'
siRFWD3 #2: 5'- GCAGUCAUGUGCAGGAGUU-3'
siRFWD3 #3: HSC.RNAI.N018124.12.1 3'UTR/13
siOrc1 [Citation40]: 5'-CUGCACUACCAAACCUAUA-3'
siOrc2 [Citation41]: 5'-UGCUCCUCUCAUGUGGGAU-3'
sip53: M-003329-03-0005, siGENOME SMARTpool, Human TP53 (7157)
Plasmids and antibodies
The HA-RFWD3 WT and CA mutant were generated as previously described [Citation38]. RFWD3 WT was cloned in the pFastBac HT B plasmid. RFWD3 WT or CA mutant were cloned in the YFP-LacI plasmid. The ORC and ORCA plasmids were generated as previously described [Citation5,Citation6,Citation7,Citation28]. FLAG-Ubiquitin was a generous gift from Dr. Jie Chen, University of Illinois at Urbana Champaign).
The following antibodies were used for immunoprecipitations and immunoblots as indicated: rabbit anti-ORCA (2853–2; IB), mouse anti-Orc2 (902; IP), rabbit anti-Orc2 (205; IB), rabbit anti-Orc1 (2518–2; IP & IB), mouse anti-T7 (Novagen), mouse anti-HA (12 CA5), mouse anti-FLAG (M2, Sigma), mouse anti-α-Tubulin (Sigma), mouse anti-Actin (Santa Cruz), rabbit anti-RFWD3 (Bethyl, A301-397A, Abcam, ab138030), goat anti-Orc3 (Abcam), mouse anti-p53 (Santa Cruz), rabbit anti-Chk2 (Cell signaling), rabbit anti-Chk2 pT68 (Cell signaling), mouse anti-RPA32 (Santa Cruz)
Immunoprecipitation and immunofluorescence
For co-immunoprecipitation, the cells were collected 24 h after transient transfection. Once collected, cells were first lysed in IP buffer (50 mM Tris-HCl pH 7.4, 500 mM NaCl, 10% glycerol, 0.25% Triton X-100 with protease and phosphatase inhibitors) at 4°C for 20 min, and equal volume of zero salt buffer was added. After centrifugation, the supernatant was pre-cleared, incubated with appropriate antibody overnight, pulled down, and washed with wash buffer (50 mM Tris-HCl pH 7.4, 250 mM NaCl, 10% glycerol, 0.25% Triton X-100 with protease and phosphatase inhibitors).
For immunofluorescence staining, cells were pre-extracted with 0.3% Triton X-100 in Cytoskeletal buffer (CSK: 100 mM NaCl, 300 mM Sucrose, 3 mM MgCl2, 10 mM PIPES pH 6.8) for 3 min on ice followed by fixation with 2% paraformaldehyde in phosphate buffered saline (PBS, pH 7.4) for 15 min in RT. Blocking was then done for 30 min with 1% normal goat serum (NGS) in PBS. Primary antibody (anti-MCM3, TB3) incubation was then carried out for 1 or 2 h in a humidified chamber followed by secondary antibody incubation for 1 h at RT. The cells were then stained with DAPI (4',6-Diamidino-2-Phenylindole) and mounted using VECTASHIELD (Vector Laboratories Inc., Burlingame, CA).
Protein purification
The purification of His-tagged protein was described previously [Citation6]. In short, the recombinant baculoviruses were produced according to the manufacture’s protocol (Bac-to-Bac baculovirus expression system; Invitrogen). Hi5/Sf9 insect cells were infected with baculoviruses expressing His-tagged proteins. The proteins were purified on a Talon column.
In vivo ubiquitination assay
HEK293T cells transfected with flag-ubiquitin were lysed using denature buffer (20 mM Tris pH7.5, 250 mM NaCl, 1 mM EDTA, 0.5% NP40, 0.5% SDS, 0.5% sodium deoxycholate) supplemented with protease and phosphatase inhibitors at 4°C for 20 min, followed by sonication for 15 min and passage through a 27 G needle to shear the DNA. Ubiquitinated proteins were then pulled down by anti-Flag M2 agarose beads (Sigma) at 4°C overnight. The beads were washed in denature buffer three times and captured ubiquitinated proteins were eluted by boiling in Laemmli buffer.
Chromatin fractionation
Detailed procedure was described previously [Citation5]. To isolate chromatin, cells were resuspended in buffer A (10 mM HEPES pH 7.9, 10 mM KCl, 1.5 mM MgCl2, 0.34 M sucrose, 10% glycerol, 1 mM DTT, 0.1% Triton X-100 with protease inhibitors) for 5 min on ice. The cytoplasmic fraction (Cyto) was separated by centrifugation (1,400 g, 4 min). The isolated nuclei were lysed in buffer B (3 mM EDTA, 0.2 mM EGTA, 1 mM DTT with protease inhibitors) for 30 min on ice. The nuclear soluble fraction (Sol) was collected by centrifugation (1,700 g 4 min). Finally, the chromatin insoluble fraction (Insol) was resuspended in buffer A (without Triton X-100) and sonicated. All the fractions were mixed with Laemmli buffer, denatured, and analyzed by Western blotting.
Cycloheximide (CHX) chase assay
To evaluate ORCA protein stability, U2OS ORCA KO cells were transient transfected with T7-ORCA in the presence or absence of HA-RFWD3. 24 h after transient transfection, U2OS ORCA KO cells were treated with 30 µg/ml CHX for 20 h or 4 h, and whole-cell extract were collected and analyzed by Western blot.
Results
RFWD3 interacts with ORC and ORCA
We have previously reported that ORC associates with ORC-associated protein (ORCA/LRWD1), a WD-repeat containing protein that stabilizes the binding of ORC to chromatin [Citation5]. We now report that ORC interacts with another WD-containing protein, RFWD3 (RING finger and WD repeat domain-containing protein 3), an E3 ligase. Co-immunoprecipitation using HA antibody in HEK 293 T cells transiently expressing HA-RFWD3 and T7-Orc1, showed that HA-RFWD3 co-immunoprecipitated with T7-Orc1 ()). We also established HA-RFWD3 stable cell line in U2OS and conducted HA pulldown [Citation38]. Endogenous Orc2 co-immunoprecipitated with HA-RFWD3 ()). In addition, T7-IP in cells co-expressing HA-RFWD3 and T7-ORCA further confirmed the interaction of RFWD3 with ORC and ORCA ()). Finally, IP with Orc1 antibody demonstrated that RFWD3 associated with endogenous ORC ()). To determine if RFWD3 directly interacts with ORC, we purified His-tagged Orc1, Orc2, and RFWD3 from insect cells and conduced immunoprecipitation using RFWD3 antibody. We found that RFWD3 directly interacts with Orc1 and Orc2 (). These experiments demonstrated that RFWD3 interacted with ORC/ORCA complex.
Figure 1. RFWD3 Interacts with ORC. (a). Co-immunoprecipitation (Co-IP) of HA-RFWD3 with T7-Orc1, the protein complex was analyzed by Western blotting. Actin serves as the loading control. “*” denotes cross-reacting band. (b). Immunoprecipitation (IP) of HA-RFWD3 in U2OS HA-RFWD3 WT stable cells, and the protein complex was analyzed by Western blotting. (c). Co-IP of HA-RFWD3 with T7-ORCA, the protein complex was analyzed by Western blotting. Orc2 serves as the positive control. Actin serves as the loading control. “*” denotes cross-reacting band. D. Immunoprecipitation (IP) of Orc1 in U2OS cells, and the protein complex was analyzed by Western blotting. Actin serves as the loading control. (e). Direct interaction of RFWD3 and Orc1 using purified proteins. (f). Direct interaction of RFWD3 and Orc2 using purified proteins
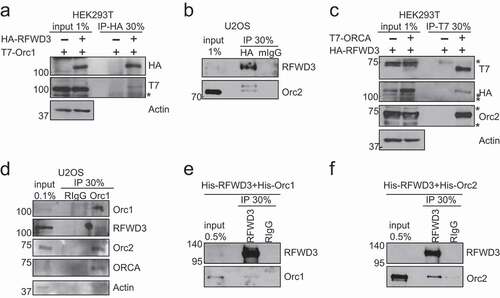
Next we tested whether RFWD3 at a chromatin site could initiate the cell cycle-specific assembly of functional replisome. MCM2-7 is a component of the pre-RC complex and is also associated with the replisome. We utilized an artificially generated in vivo heterochromatic locus in the human U2OS osteosarcoma cells [Citation5,Citation42]. This reporter cell line carries a stably integrated 200-copy transgene array with the Lac operator repeats, and the locus is visualized by the stable expression of Cherry-Lac repressor (Cherry-LacI) ()). YFP-LacI-RFWD3 or YFP-LacI-RFWD3 CA (ligase-dead mutant, C315A) was tethered to the CLTon locus and we examined the association of replisome components. The tethering of RFWD3 showed robust accumulation of the MCM helicase specifically during S-phase of the cell cycle, as is evident from the MCM pattern reminiscent of S phase cells ()), supporting the data that RFWD3 colocalizes with the replisome components during S-phase ()). The RFWD3 CA mutant behaved similar to the WT, suggesting that the ligase activity of RFWD3 is not required for its association with replisome.
Figure 2. RFWD3 co-localized with MCM during S phase. (a). Schematic representation of the 2-6-3 CLTon locus in human U2OS cells. (b). Representative images of YFP-LacI, YFP-LacI-RFWD3 WT, or YFP-LacI-RFWD3-CA with MCM3 during G1 or S phase of the cell cycle. Scale bar denotes 15 µm. (c). Quantification of A. Cell number > 45. Values (means ± S.D.) are from four independent experiments
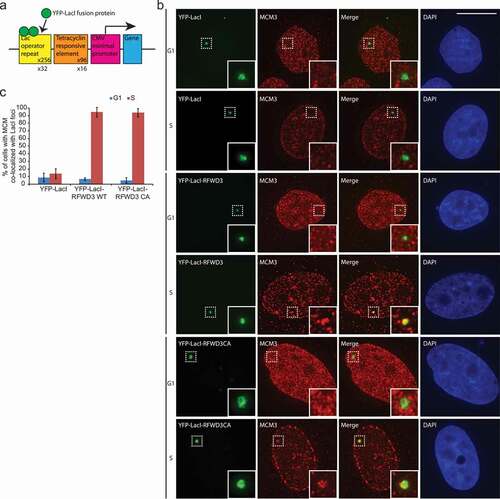
Overexpression of ORC stabilizes RFWD3 in a P53-dependent manner
To determine the functional significance of ORC/ORCA interaction with RFWD3, we co-expressed RFWD3 with ORC subunits or ORCA. Surprisingly, we observed that overexpression of Orc1-5 (shown here Orc1, 2 and 5), and ORCA stabilized RFWD3, whereas the overexpression of Orc6 did not ()). Among the ORC subunits that stabilize RFWD3, Orc2 directly interacts with RFWD3, and showed prominent stabilization of RFWD3. We mapped the minimum domain of Orc2 that is required for RFWD3 stabilization. We found that residues 277–451 aa of Orc2 are required for stabilizing RFWD3 ()). This region of Orc2 is also known to be the Orc3 interaction domain.
Figure 3. Overexpression of ORC stabilizes RFWD3. (a). Immunoblot analysis of whole cell extracts transfected with HA-RFWD3, along with vector or T7-tagged ORC/ORCA. “*” denotes T7-tagged ORC/ORCA. (b). Immunoblot analysis of whole cell extracts transfected with HA-RFWD3, along with vector or T7-tagged Orc2 WT and truncations. (c). Immunoblot analysis of whole cell extracts transfected with HA-RFWD3, in the absence or presence of T7-Orc2 and MG132. (d). Immunoblot analysis of whole cell extracts transfected with HA-RFWD3 WT or CA mutant, in the absence or presence of T7-Orc2
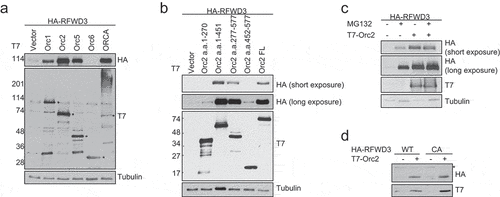
RFWD3 is known to be auto-ubiquitinated and therefore can mediate its own degradation [Citation37]. To determine if Orc2 stabilizes RFWD3 through preventing its auto-ubiquitination and degradation, we co-expressed Orc2 with HA-RFWD3 with or without proteasome inhibitor MG132. The presence of MG132 did not further enhance RFWD3 level, suggesting that Orc2 binding to RFWD3 did not protect RFWD3 from proteasome degradation ()). Consistent with this result, the RFWD3 ligase-dead mutant can be stabilized by Orc2 ()). These results demonstrate that the overexpression of ORC/ORCA can stabilize RFWD3.
It is interesting to note that the overexpression levels of HA-RFWD3 vary depending on the genetic background of the cells. Using HCT116 WT, p53-/- and p21-/- cells, we observed that RFWD3 protein levels were significantly low in p21-/- cells ()). We addressed if overexpression of Orc2 can stabilize RFWD3 in these genetic backgrounds. We observed that Orc2 stabilized RFWD3 in WT and the p21-/- background ()), but not in cells lacking p53 ()). Also, the catalytic dead RFWD3 was found to be less stable than WT RFWD3 in both Orc2-overexpressed WT and p21 -/- cells ()). Because Orc2 is unable to stabilize RFWD3 in cells lacking p53, we treated these cells with MDM2 inhibitor (Nutlin) and proteasomal inhibitor (MG132). Both these treatments resulted in the stabilization of RFWD3 upon Orc2 overexpression in p53-/- cells ()). These results suggest that MDM2 helps in rapid turnover of RFWD3.
Figure 4. P53-dependent stabilization of RFWD3 by ORC. (a). Immunoblot analysis of HA-RFWD3 expression level in HCT116 WT, p21 null, and p53 null cells. “*”denotes cross-reacting band and loading control. (b). Immunoblot analysis of whole cell extracts (HCT116 WT or p21 null) transfected with HA-RFWD3 WT or CA mutant, in the absence or presence of T7-Orc2. (c). Immunoblot analysis of HCT116 p53 null whole cell extracts transfected with HA-RFWD3 WT or CA mutant, in the absence or presence of T7-Orc2, Nutilin-3, or MG132. (d). Immunoblot analysis of whole cell extracts from cells treated with control, Orc1 or Orc2 siRNAs. Varying concentrations (25%, 50%, and 100%) of whole-cell extracts treated with control siRNA (siGl3) are shown to provide information on the percentage of knockdown and the reduction of protein levels. (e). Immunoblot analysis of chromatin fractionation from cells treated with control, Orc1 or Orc2 siRNAs. S1 represents cytosolic fraction, S2 represents nuclear soluble fraction, P represents chromatin insoluble fraction
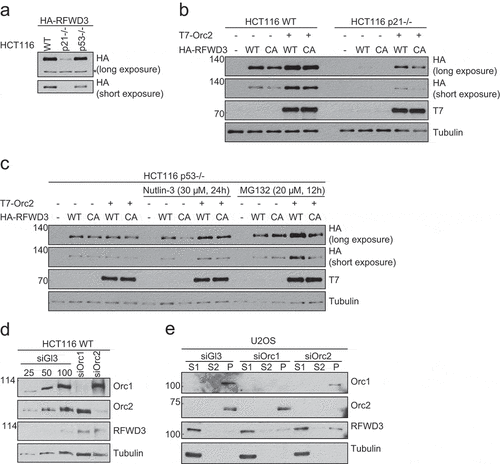
However, depletion of endogenous Orc1 or Orc2 in human cells (HCT116 and U2OS) did not destabilize endogenous RFWD3 level, rather, it resulted in modest increase in total RFWD3 and chromatin-bound RFWD3 levels (), suggesting multiple mechanisms operate to stabilize RFWD3 in vivo. Based on the inability in generating RFWD3 knockout cells [Citation34,Citation37,Citation38], and the fact that RFWD3 plays essential roles in DNA damage response and replication fork progression, we reasoned that the endogenous RFWD3 level is controlled by multiple redundant mechanisms, and thus remains unperturbed upon the loss of Orc1 or Orc2.
Depletion of RFWD3 results in p53-dependent reduction of ORC
In order to gain functional insights into the interaction of RFWD3 with ORC/ORCA, we depleted RFWD3 and studied the impact on ORC/ORCA protein levels. The depletion of RFWD3 resulted in reduced levels of ORC/ORCA (,d)). The depletion of RFWD3 in HCC38, a breast cancer cell line, which carries a gain of function mutation of p53 (R273L), also leads to reduced levels of ORC ()). However, depletion of RFWD3 in p53 null cells did not show reduction in ORC levels (). The activation of p21 by p53 is crucial in G1 checkpoint [Citation43,Citation44]. We therefore monitored the levels of ORC upon RFWD3 depletion in p21 -/- cells. ORC levels remained unchanged in control and RFWD3-depleted p21-/- cells ()), suggesting that the regulation of p53 pathway by RFWD3 governs ORC/ORCA stability. These results were further confirmed in U2OS cells where we co-depleted RFWD3 and p53 by siRNA. While loss of RFWD3 resulted in degradation of ORC, co-depletion of RFWD3 and p53 resulted in restoration of ORC levels ()). To determine whether the E3 ligase activity is essential for ORC/ORCA stabilization by RFWD3, Orc2 level was monitored in RFWD3-depleted samples rescued by RFWD3 WT or CA mutant. The changes of Orc2 level were rescued with RFWD3 WT, but not the CA mutant, suggesting that the E3 ligase activity of RFWD3 is required for stabilizing ORC/ORCA ()).
Figure 5. RFWD3 loss results in p53-dependent reduction of ORC/ORCA. (a-c). Immunoblot analysis of whole cell extracts from HCT116 WT (a), p53 null (b), or p21 null (c) cells treated with RFWD3 siRNAs. Varying concentrations (25%, 50%, and 100%) of whole-cell extracts treated with control siRNA (siGl3) are shown to provide information on the percentage of knockdown and the reduction of protein levels. (d-e). Immunoblot analysis of whole cell extracts from HCT116 WT (d) or p53 null (e) cells treated with RFWD3 siRNAs. Varying concentrations (25%, 50%, and 100%) of whole cell extracts treated with control siRNA (siGl3) are shown to provide information on the percentage of knockdown and the reduction of protein levels. (f). Immunoblot analysis of whole cell extracts from HCC38 cells treated with control or RFWD3 siRNAs. Varying concentrations (25%, 50%, and 100%) of whole cell extracts treated with control siRNA (siGl3) are shown to provide information on the percentage of knockdown and the reduction of protein levels. (g). Immunoblot analysis of whole cell extracts from cells treated with RFWD3 siRNAs in the absence or presence of p53 siRNA. Varying concentrations (25%, 50%, and 100%) of whole-cell extracts treated with control siRNA (siGl3) are shown to provide information on the percentage of knockdown and the reduction of protein levels. (h). Immunoblot analysis of whole cell extracts treated with RFWD3 siRNAs in the absence or presence of HA-RFWD3 WT or CA mutant. Varying concentrations (25%, 50%, and 100%) of whole-cell extracts treated with control siRNA (siGl3) are shown to provide information on the percentage of knockdown and the reduction of protein levels. (i). Immunoblot analysis of whole cell extracts from U2OS treated with different DNA damage agents. Cells were treated with 2 mM hydroxyurea (HU) for 4 h or 24 h, 1 µM of cisplatin for 4 h, or 20 ng/ml mitomycin C (MMC) for 4 h
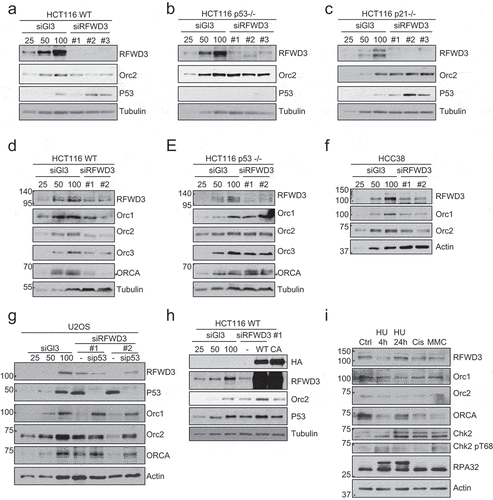
The depletion of RFWD3 is known to cause replication stress and defects in interstrand crosslink repair [Citation33–36,Citation38,Citation45]. To rule out the possibility that the changes in ORC/ORCA is an indirect result of DNA damage, we treated cells with various DNA damaging agents and monitored the changes in ORC/ORCA level ()). Treatment with various DNA damaging agents that induce fork stalling, fork collapse, interstrand crosslink, and alkylation do not lead to a global reduction in ORC/ORCA, suggesting that the destabilization of ORC/ORCA upon loss of RFWD3 is not a result of DNA damage.
RFWD3 ubiquitinates ORCA
Since RFWD3 is an E3 ligase, which directly interacts with Orc1 and Orc2, we aimed to determine whether RFWD3 ubiquitinates any of the ORC subunits and/or ORCA. Human Orc1 is known to be ubiquitinated and degraded during S phase [Citation26,Citation27]. Several ubiquitination sites on Orc2 have been identified [Citation46]. ORCA is also known to be ubiquitinated at the G1/S boundary [Citation28]. We did not observe any changes in the ubiquitination of Orc1 in the presence of RFWD3 WT. Minimal ubiquitination of Orc2 was observed with/without RFWD3, similar to previous findings [Citation29] ()). The overexpression of RFWD3 WT, but not the CA mutant, led to enhanced ubiquitination of ORCA ()). The differences in ORCA ubiquitination level was not due to disrupted interaction between ORCA and RFWD3 CA mutant, since the E3 ligase activity of RFWD3 was not found to be required for its interaction with ORCA ()). We have previously shown that the interaction of ORCA with Orc2 is essential for its stability [Citation28]. To determine the impact of ORCA ubiquitination, we evaluated if this affected its interaction with ORC. We generated a ubiquitination-deficient mutant of ORCA (28KR, )) and found that this mutant showed significantly reduced interaction with Orc2 ()), indicating that the RFWD3-mediated ubiquitination of ORCA facilitates its interaction with Orc2, thereby enhancing its stability. Finally, we analyzed ORCA protein stability with or without HA-RFWD3 overexpression. Upon treatment with cycloheximide (CHX), ORCA protein decreased significantly after 4 h. However, upon the overexpression of RFWD3, 4 h of CHX treatment did not cause significant reduction of ORCA protein level, which further supports our model that RFWD3 stabilizes ORCA ()). All of this data supports the model that RFWD3-mediated ubiquitination of ORCA increases its interaction with ORC complex and also enhances the stability of ORCA.
Figure 6. RFWD3 ubiquitinates ORCA. (a). In vivo ubiquitination assay in control, RFWD3 WT, or RFWD3 CA over-expressing samples co-transfected with T7-Orc2 and FLAG-ubiquitin. IP was performed with anti-FLAG beads and immunoblotting with Orc2 antibody. (b). In vivo ubiquitination assay in control, RFWD3 WT, or RFWD3 CA over-expressing samples co-transfected with T7-ORCA and FLAG-ubiquitin. PCNA serves as positive control for RFWD3-mediated ubiquitination. IP was performed with anti-FLAG beads and immunoblotting with ORCA antibody. (c). Co-IP of HA-RFWD3 WT or CA mutant with T7-ORCA. IP was performed with anti-T7 antibody, and the protein complex was analyzed by Western blotting. Orc2 serves as the positive control. Actin serves as the loading control “*” denotes cross-reacting band. (d). Schematic of ORCA 28KR mutant. (e). Co-IP of T7-ORCA WT or 28KR with Orc2, the protein complex was analyzed by Western blotting. Actin serves as the loading control. (f). Cycloheximide (CHX) chase assay showing T7-ORCA stability in the absence or presence of HA-RFWD3. Actin is served as the loading control. (g). The schematic of RFWD3 protects ORC/ORCA in a p53-dependent manner. In the absence of p53, ORC/ORCA are targeted by a p53-induced E3 ligase for degradation
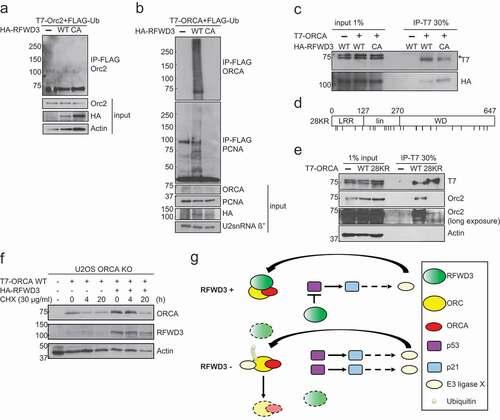
Our results point to an interaction between RFWD3 and ORC/ORCA and our study uncovers a novel mode of ORC stabilization by virtue of binding to an E3 ligase. We propose that the association of RFWD3 with ORC/ORCA prevents its destabilization, which is mediated by a p53 target E3 ligase ()).
Discussion
The proper regulation of DNA replication is essential for preventing genome instability. In G1 phase of the cell cycle, the “origin licensing” is achieved by sequential assembly of pre-RC components on the origins of replication [Citation1–4]. The appropriate regulation of origin licensing and timing is essential for maintaining genome stability [Citation47]. The regulation of origin licensing is achieved through modulating the dynamics of pre-RC components throughout the cell cycle. Multiple mechanisms lead to the removal of preRCs from origins once licensing is achieved and this ensures that DNA replication occurs once and once per cell division cycle. For example, as cells enter S phase, Orc1 is phosphorylated and consequently degraded [Citation26,Citation27]. The ubiquitination of ORCA occurs during the G1/S boundary, which leads to its degradation [Citation28]. The protein level of ORC2-5 remain constant throughout the cell cycle. However, the phosphorylation of Orc2 during S phase is required for the dissociation of ORC2-5 from the chromatin [Citation48]. The depletion of pre-RC components in normal human cells activates origin licensing checkpoint and leads to G1 arrest [Citation11,Citation12,Citation13].
In the present study, we show that ORC/ORCA associates with an E3 ligase RFWD3. Recent studies have shown that RFWD3 is essential for DNA damage response and S phase progression [Citation32–38,Citation45]. We found that RFWD3 directly interacts with components of the ORC. Binding of ORC to RFWD3 protects the ORC complex from degradation, specifically in cells that have an intact p53 pathway. However, when cells lose the p53 protein or have an abrogated p53 pathway, loss of RFWD3 doesn’t cause degradation of ORC. Our results support a model whereby p53 activates a E3 ubiquitin ligase that is responsible for the destruction of ORC. In RFWD3-depleted cells, ORC is not protected and in the presence of p53, a downstream p53 target E3 ligase results in degradation of ORC. Consistent with this model, depletion of RFWD3 in p53-/- cells causes stabilization of ORC. It is interesting to note that the decrease in Orc2 upon RFWD3 depletion (in p53 proficient cells) can be rescued by RFWD3 WT but not the CA mutant. This suggests that the E3 ligase activity of RFWD3 is needed for the ORC stability. It could be that the autoubiquitinated form of RFWD3 associates with ORC and protects it from destruction. Alternatively, active form of RFWD3 ubiquitinates ORC and this form of ORC cannot be targeted for destruction by the p53 pathway. We found that RFWD3 does not ubiquitinate Orc1 and Orc2 but can ubiquitinate ORCA. Depletion of RFWD3 doesn’t result in the accumulation of ORC/ORCA suggesting that RFWD3-mediated ubiquitination of ORC/ORCA does not target this complex for proteasomal degradation. Our results support a model whereby RFWD3-mediated ubiquitination of ORCA is required for interaction with ORC and this enhances the stability of ORC-ORCA. Thus, the depletion of RFWD3 leads to destabilization of ORCA, and consequently decreased ORC2-5. Future work will focus on identifying the p53 target E3 ligase that is responsible for ORC degradation.
We have previously reported that in cancer cells, the depletion of RFWD3 leads to accumulation of S phase population, whereas in WI38 (human diploid fibroblast), RFWD3-deficient cells showed G1 arrest after serum release [Citation38]. It is known that the depletion of preRC components in diploid fibroblasts causes a G1 arrest. We propose that in the absence of RFWD3 origin licensing checkpoint is activated because of a reduction in ORC proteins. Future work will entail understanding if RFWD3 has a direct role in DNA replication initiation, and the molecular mechanism of its association and control of ORC function.
Author contributions
RH, SG, YW, YCL, SW, AC, DL designed, performed, and analyzed the experiments. SGP and KVP supervised the project. RH and SGP wrote the manuscript.
Acknowledgments
We thank members of the Prasanth laboratory for discussions and suggestions. We thank Drs. J. Chen, A. Gambus, Z. Gong, A. Maréchal, K. Sato, D. Spector, B. Stillman, M. Takata, Y. Wang, and L. Zou for providing reagents, protocols, and suggestions. This work was supported by NSF-CMMB-IGERT fellowship to RH; Cancer center at Illinois seed grant and Prairie Dragon Paddlers and NSF EAGER awards and NIH (GM132458 and AG065748) to KVP; and NSF (1243372 and 1818286) and NIH (GM125196) awards to SGP. The authors declare no competing financial interests.
Disclosure statement
The authors declare no competing interests.
Data availability statement
The authors confirm that the data supporting the findings of this study are available within the article and openly available in reference number [Citation5, Citation38].
Additional information
Funding
References
- Bell SP, Dutta A. DNA replication in eukaryotic cells. Annu Rev Biochem. 2002;71:333–374.
- Arias EE, Walter JC. Strength in numbers: preventing rereplication via multiple mechanisms in eukaryotic cells. Genes Dev. 2007;21:497–518.
- Drury LS, Diffley JF. Factors affecting the diversity of DNA replication licensing control in eukaryotes. Curr Biol. 2009;19:530–535.
- Truong LN, Wu X. Prevention of DNA re-replication in eukaryotic cells. J Mol Cell Biol. 2011;3:13–22.
- Shen Z, Sathyan KM, Geng Y, et al. A WD-repeat protein stabilizes ORC binding to chromatin. Mol Cell. 2010;40:99–111. doi:10.1016/j.molcel.2010.09.021
- Shen Z, Chakraborty A, Jain A, et al. Dynamic association of ORCA with prereplicative complex components regulates DNA replication initiation. Mol Cell Biol. 2012;32:3107–3120.
- Gambus A, Jones RC, Sanchez-Diaz A, et al. GINS maintains association of Cdc45 with MCM in replisome progression complexes at eukaryotic DNA replication forks. Nat Cell Biol. 2006;8:358–366.
- Moyer SE, Lewis PW, Botchan MR. Isolation of the Cdc45/Mcm2-7/GINS (CMG) complex, a candidate for the eukaryotic DNA replication fork helicase. Proc Natl Acad Sci U S A. 2006;103:10236–10241.
- Ge XQ, Jackson DA, Blow JJ. Dormant origins licensed by excess Mcm2-7 are required for human cells to survive replicative stress. Genes Dev. 2007;21:3331–3341.
- Ibarra A, Schwob E, Mendez J. Excess MCM proteins protect human cells from replicative stress by licensing backup origins of replication. Proc Natl Acad Sci U S A. 2008;105:8956–8961.
- Lunn CL, Chrivia JC, Baldassare JJ. Activation of Cdk2/Cyclin E complexes is dependent on the origin of replication licensing factor Cdc6 in mammalian cells. Cell Cycle. 2010;9:4533–4541.
- Machida YJ, Teer JK, Dutta A. Acute reduction of an origin recognition complex (ORC) subunit in human cells reveals a requirement of ORC for Cdk2 activation. J Biol Chem. 2005;280:27624–27630.
- Nevis KR, Cordeiro-Stone M, Cook JG. Origin licensing and p53 status regulate Cdk2 activity during G1. Cell Cycle. 2009;8:1952–1963.
- Ekholm-Reed S, Méndez J, Tedesco D, et al. Deregulation of cyclin E in human cells interferes with prereplication complex assembly. J Cell Biol. 2004;165:789–800.
- Macheret M, Halazonetis TD. Intragenic origins due to short G1 phases underlie oncogene-induced DNA replication stress. Nature. 2018;555:112–116.
- Dominguez-Sola D, Ying CY, Grandori C, et al. Non-transcriptional control of DNA replication by c-Myc. Nature. 2007;448:445–451.
- Hernandez-Carralero E, Cabrera E, Alonso-de Vega I, et al. Control of DNA replication initiation by ubiquitin. Cells. 2018;7. DOI:10.3390/cells7100146
- Parker MW, Botchan MR, Berger JM. Mechanisms and regulation of DNA replication initiation in eukaryotes. Crit Rev Biochem Mol Biol. 2017;52:107–144.
- Diffley FX, Cocker JH, Dowell SJ, et al. Two steps in the assembly of complexes at yeast replication origins in vivo. Cell. 1994;70:303–316.
- Liang. C, Stillman B. Genes and development persistent initiation of DNA replication and chromatin-bound MCM proteins during the cell cycle in cdc6 mutants. Genes Dev. 1997;11:3375–3386.
- Nguyen VQ, Co. C, Li JJ. Cyclin-dependent kinases prevent DNA re-replication through multiple mechanisms. Nature. 2001;411:1068–1073.
- Araki M, Wharton RP, Tang Z, et al. Degradation of origin recognition complex large subunit by the anaphase-promoting complex in Drosophila. Embo J. 2003;22:6115–6126.
- Li CJ, DePamphilis ML. Mammalian Orc1 protein is selectively released from chromatin and ubiquitinated during the S-to-M transition in the cell division cycle. Mol Cell Biol. 2002;22:105–116.
- Li CJ, Vassilev A, DePamphilis ML. Role for Cdk1 (Cdc2)/cyclin A in preventing the mammalian origin recognition complex’s largest subunit (Orc1) from binding to chromatin during mitosis. Mol Cell Biol. 2004;24:5875–5886.
- Hiraga SI, Ly T, Garzón J, et al. Human RIF 1 and protein phosphatase 1 stimulate DNA replication origin licensing but suppress origin activation. EMBO Rep. 2017;18:403–419.
- Tatsumi Y, Ohta S, Kimura H, et al. The ORC1 cycle in human cells: I. cell cycle-regulated oscillation of human ORC1. J Biol Chem. 2003;278:41528–41534.
- Méndez J, Zou-Yang XH, Kim S-Y, et al. Human origin recognition complex large subunit is degraded by ubiquitin-mediated proteolysis after initiation of DNA replication. Mol Cell. 2002;9:481–491.
- Shen Z, Prasanth SG. Orc2 protects ORCA from ubiquitin-mediated degradation. Cell Cycle. 2012;11:3578–3589.
- Coulombe P, Nassar J, Peiffer I, et al. The ORC ubiquitin ligase OBI1 promotes DNA replication origin firing. Nat Commun. 2019;10:2426.
- Matsuoka S, Ballif BA, Smogorzewska A, et al. ATM and ATR substrate analysis reveals extensive protein networks responsive to DNA damage. Science. 2007;316:1160–1166.
- Mu JJ, Wang Y, Luo H, et al. A proteomic analysis of ataxia telangiectasia-mutated (ATM)/ATM-Rad3-related (ATR) substrates identifies the ubiquitin-proteasome system as a regulator for DNA damage checkpoints. J Biol Chem. 2007;282:17330–17334.
- Fu X, Yucer N, Liu S, et al. RFWD3-Mdm2 ubiquitin ligase complex positively regulates p53 stability in response to DNA damage. Proc Natl Acad Sci U S A. 2010;107:4579–4584.
- Gong Z, Chen J. E3 ligase RFWD3 participates in replication checkpoint control. J Biol Chem. 2011;286:22308–22313.
- Inano S, Sato K, Katsuki Y, et al. RFWD3-mediated ubiquitination promotes timely removal of both RPA and RAD51 from DNA damage sites to facilitate homologous recombination. Mol Cell. 2017;66:622–634 e628.
- Elia AE, Wang D, Willis N, et al. RFWD3-dependent ubiquitination of RPA regulates repair at stalled replication forks. Mol Cell. 2015;60:280–293.
- Feeney L, Muñoz IM, Lachaud C, et al. RPA-mediated recruitment of the E3 ligase RFWD3 is vital for interstrand crosslink repair and human health. Mol Cell. 2017;66:610–62Re614.
- Knies K, Inano S, Ramírez MJ, et al. Biallelic mutations in the ubiquitin ligase RFWD3 cause Fanconi anemia. J Clin Invest. 2017;127:3013–3027.
- Lin Y-C, Wang Y, Hsu R, et al. PCNA-mediated stabilization of E3 ligase RFWD3 at the replication fork is essential for DNA replication. Proc Natl Acad Sci U S A. 2018;115:13282–13287. doi:10.1073/pnas.1814521115
- Giri S, Chakraborty A, Sathyan KM, et al. Orc5 induces large-scale chromatin decondensation in a GCN5-dependent manner. J Cell Sci. 2016;129:417–429.
- Hsu RYC, Lin Y-C, Redon C, et al. ORCA/LRWD1 regulates homologous recombination at ALT-telomeres by modulating heterochromatin organization. iScience. 2020;23:101038.
- Prasanth SG, Prasanth KV, Siddiqui K, et al. Human Orc2 localizes to centrosomes, centromeres and heterochromatin during chromosome inheritance. Embo J. 2004;23:2651–2663.
- Janicki SM, Tsukamoto T, Salghetti SE, et al. From silencing to gene expression: real-time analysis in single cells. Cell. 2004;116:683–698.
- El-Deiry WS, Tokino T, Velculescu VE, et al. WAF1, a potential mediator of p53 tumor suppression. Cell. 1993;75: 817–825.
- Harper JW, Adami GR, Wei N, et al. The p21 Cdk-interacting protein Cip1 is a potent inhibitor of G1 cyclin-dependent kinases. Cell. 1993;75:805–816.
- Liu S, Chu J, Yucer N, et al. RING finger and WD repeat domain 3 (RFWD3) associates with replication protein A (RPA) and facilitates RPA-mediated DNA damage response. J Biol Chem. 2011;286:22314–22322.
- Akimov V, Barrio-Hernandez I, Hansen SVF, et al. UbiSite approach for comprehensive mapping of lysine and N-terminal ubiquitination sites. Nat Struct Mol Biol. 2018;25:631–640.
- Petropoulos M, Champeris Tsaniras S, Taraviras S, et al. Replication licensing aberrations, replication stress, and genomic instability. Trends Biochem Sci. 2019;44:752–764.
- Lee KY, Bang SW, Yoon SW, et al. Phosphorylation of ORC2 protein dissociates origin recognition complex from chromatin and replication origins. J Biol Chem. 2012;287:11891–11898.