ABSTRACT
This study evaluated the effects of endoplasmic reticulum autophagy (ER-phagy) and globular adiponectin (gAPN) on chronic intermittent hypoxia (CIH)-induced H9C2 cardiomyocytes injury while investigating potential mechanisms of action. The CIH model of H9C2 cardiomyocytes was established in this study. CCK-8 assay was used to determine cell viability post-exposure to various CIH times and gAPN concentrations. Flow cytometry was used to observe H9C2 cardiomyocytes apoptosis and immunofluorescence was used to measure ER-phagy and SEC62 activation. Western blot was used to observe ER stress and AMPK pathway. Results indicated that ER stress was activated in H9C2 cardiomyocytes exposed to CIH. Inhibition of ER stress reduced CIH-induced cell apoptosis. gAPN attenuated CIH-induced ER stress and H9C2 cardiomyocytes apoptosis. ER-phagy and SEC62 protein level were induced by CIH, while gAPN highly enhanced these changes. Inhibition of SEC62 expression reduced ER-phagy and increased ER stress and H9C2 cardiomyocytes apoptosis. Moreover, gAPN induced AMPK expression. Inhibition of AMPK expression reduced SEC62-mediated ER-phagy and increased the H9C2 cardiomyocytes apoptosis. Altogether, our study suggested that gAPN upregulated SEC62-mediated ER-phagy to extenuate ER stress, and mitigated H9C2 cardiomyocytes apoptosis induced by CIH through AMPK activation.
Introduction
Obstructive sleep apnea (OSA) is a common clinical disease characterized by repeated upper airway collapses leading to chronic intermittent hypoxia (CIH), excessive sympathetic nervous activation, sleep fragment, exaggerated negative intrathoracic pressure, and micro-arousal. In the late 2000s, the morbidity of OSA was 17% in women and 34% in men. Notably, the morbidity was only 4% in women and 5% in men in 1993 [Citation1]. OSA is associated with various cardiovascular diseases (CVD), including hypertension, coronary artery disease, stroke, atherosclerosis, and heart failure. OSA can not only raise the cardiovascular risk but also worsen CVD outcomes [Citation2]. Mazzotti reported that 26.3% of OSA patients had CVD, while the incident events of major CVD increased to 17.6% in patients with OSA [Citation3]. However, the clear association between OSA and CVD remains to be further investigated.
Adiponectin (APN) is a protein hormone secreted by adipocytes and exerts protective effects on cardiovascular systems, such as anti-inflammation, anti-insulin resistance, antioxidant, anti-atherosclerosis, and anti-apoptotic functions [Citation4]. Adiponectin can exist as full-length adiponectin and globular domain adiponectin (gAPN) styles in plasma, both forms have some bioactivity [Citation4]. It has been reported that the plasma adiponectin level decreased in OSA patients and was inversely proportional to the severity of OSA [Citation5]. APN supplementation ameliorated cardiac cell apoptosis in rats suffering CIH exposure [Citation6]. Therefore, a loss of adiponectin may contribute to cardiovascular injury in OSA patients, while adiponectin supplementation may protect against CIH-induced myocardial injury.
Endoplasmic reticulum phagy (ER-phagy) is a branch of autophagy that selectively degrades ER and plays a central role in ER homeostasis [Citation7,Citation8]. ER-phagy is associated with many diseases, such as CVD, neurodegenerative disease, and diabetes [Citation9]. However, the effect of ER-phagy on CIH injury and the protective role of adiponectin on cardiomyocytes remain unknown. In the current study, a cellular CIH model was established to explore the potential role of ER-phagy on H9C2 cardiomyocytes under CIH exposure, while observing the influence of adiponectin supplementation.
Materials and methods
H9C2 cardiomyocytes culture
The rat H9C2 cardiomyocytes purchased from ATCC were cultured in high glucose Dulbecco’s Modified Eagle’s Medium (DMEM; Gibco, Maryland, USA) with 10% fetal bovine serum (FBS; Gibco) and 1% penicillin/streptomycin (Sigma, Missouri, USA). H9C2 cardiomyocytes were incubated at 37°C with 95% air and 5% CO2. The intermittent hypoxia incubator (Biospherix, New York, USA) controlled the O2 concentration by regulating the contention of N2, CO2, and O2. H9C2 cardiomyocyte was pretreated with 4-phenylbutyrate (4-PBA, 5 mM, Sigma) for 24 h, gAPN (Biovision, New York, USA) for 24 h, and Compound C (10 µM, Sigma) for 2 h, followed by CIH exposure [Citation10] (21% O2/5% CO2/75% N2 for 10 min and 5% CO2/95% N2 for 10 min, three anoxic cycles per hour) or normoxia conditions (21% O2/5% CO2/75% N2) for 48 h.
CCK-8 analysis
The CCK-8 kit (Beyotime, Shanghai, China) was used to determine the beneficial time of CIH and concentration of gAPN by detecting cell viability. The H9C2 cardiomyocytes (104/ml single-cell suspension) were seeded into 96-well plates (Zellkontakt, Hardenberg, Germany) and starved with 100 µl serum-free culture medium for 24 h after cell adhesion. Five plates were randomly assigned to normoxia condition (72 h), and CIH conditions (12 h, 24 h, 48 h, and 72 h). Thereafter, each well was added into 10 µl CCK8 solution and incubated for 4 h at 37°C. Absorbance was detected at 450 nm using a microplate reader (BioTek, Vermont, USA).
The H9C2 cardiomyocytes were seeded into 96-well plate and starved for 24 h after cell adhesion. Varying concentrations of gAPN (0, 0.5, 1, and 2 µg/ml) were randomly added into the 96-well plate and incubated for 24 h, followed by 48 h CIH exposure. The NC group received the normoxia treatment (48 h). Next, CCK-8 solution (10 µl) was added to each well and incubated for 4 h at 37°C. Absorbance was detected at 450 nm using a microplate reader.
Cell apoptosis
Flow cytometry was used to determine H9C2 cardiomyocytes apoptosis. H9C2 cardiomyocytes were inoculated into 6-well plates (Zellkontakt) and randomly divided into different experimental groups after cell adhesion. Thereafter, H9C2 cardiomyocytes were collected and centrifuged at 1500 rpm/min for 5 min post-exposure to various treatments. The supernatant was discarded, and the precipitate was washed twice with PBS. Binding buffer (500 µl) with 5 µl FITC-labeled Annexin V (20 µg/ml) and 5 µl PI (50 µg/ml) (BD, PharMingen, California, USA) was added to the precipitate to resuspend the cells and incubated for 15 min in the dark. H9C2 cardiomyocytes apoptosis was measured using flow cytometry (BD FACS ARIA II, SORP, USA).
Western blot
Western blot was used to measure protein expression levels. The H9C2 cardiomyocytes were washed twice with PBS after experimentation. The pre-cooled lysis buffer, with 1 mM PMSF (10 µl) (Roche, Shanghai, China), 1 µl 1000 X protease inhibitor (Roche), and 10 µl 100 X phosphatase inhibitor (Roche), was used to split H9C2 cardiomyocyte on ice for 5 min. The pyrolysis products of H9C2 cardiomyocytes were scraped off the plate and centrifuged at 14,000 × g for 15 min at 4°C. The supernatant was collected to perform western blot. The bicinchoninic acid kit (Thermo Scientific, Shanghai, China) was used to assess protein concentration. Equal amounts of protein were subjected to 10% sodium dodecyl sulfate PAGE (Beyotime, Shanghai, China) and transferred to polyvinylidene fluoride membranes (Roche) after electrophoresis. The membrane was blocked for 1 h at room temperature and then incubated at 4°C overnight with primary antibodies, followed by incubation with HRP-labeled secondary antibody (Cell Signaling Technology (CST), Danvers, MA, USA) at 37°C for 1 h. The protein bands were measured using the enhanced ECL kit (Thermo Scientific). The GRP78 (BIP), p-PERK, PERK, pro-ATF6, p-AMPK, AMPK, and GAPDH antibodies were purchased from CST. The CHOP, LC3I/II, SEC62, p-IRE1, and IRE1 antibodies were purchased from Abcam (Cambridge, UK).
Immunofluorescence
The ER-TrackerTM Red dyes (Thermofisher, Shanghai, China) were used to stain the endoplasmic reticulum according to the manufacturer’s instructions [Citation11]. The fluorescence co-localization of GFP-LC3 and ER-TrackerTM Red was used to measure ER-phagy. The H9C2 cardiomyocytes were transfected with GFP-LC3 adenovirus (MOI = 50, HanBio, Shanghai, China) for 2 h after inoculation into the 6-well plate with coverslips. Following treatment for previous experiments, the cells were also treated with ER-TrackerTM Red staining solution for 30 min at 37°C to co-localize the ER. After incubation with DAPI for 5 min, the images were detected using a confocal laser scanning microscope (Olympus, Tokyo, Japan). The bright green dots represented LC3, the bright red dots represented ER membrane, and the yellow dots represent ER-phagy. ER-phagy was evaluated by calculating the number of yellow dots.
The fluorescence co-localization of SEC62 and GFP-LC3 was used to assess whether SEC62 is involved in the process of ER-phagy. After transfection with GFP-LC3 adenovirus, the H9C2 cardiomyocyte performed differently post-treatment. H9C2 cardiomyocytes were treated with 4% paraformaldehyde for 10 min, and 0.1% Triton X-100 for 10 min. Goat serum was used to block the H9C2 cardiomyocytes for 1 h. The H9C2 cardiomyocytes were then incubated with the anti- SEC62 antibody (rabbit, 1:250, Abcam, Cambridge, UK) overnight at 4°C in the dark. Thereafter, Alexa 594-conjugated goat-anti-rabbit antibody (1:1000, Abcam) was used to label SEC62 for 1 h in the dark. After incubation with DAPI for 5 min, the images were detected using a confocal laser scanning microscope. The bright green dots represented LC3, the bright red dots represented SEC62, and the yellow dots represent SEC62 combination with LC3. The number of yellow dots was calculated to evaluate SEC62 activation.
Silencing SEC62 gene expression by siRNA
H9C2 cardiomyocytes were seeded into 12-well plates before gene silencing. Then, H9C2 cardiomyocytes were transfected with SEC62 siRNA (GenePharma, Shanghai, China) or control siRNA (GenePharma) using Lipofectamin2000 (Thermofisher), according to the manufacturer’s instructions, for 24 h and the silencing efficiencies of the SEC62 siRNA were determined using western blot. The siRNA sequences in this study were as follows: SEC62 siRNA: sense primer 5-CCAAGUAUCUUCGAUUCAATTUUGAA TT-3and antisense primer 5‐UUGAAUCGAAGAUACUUGGTT‐3; control siRNA: sense primer 5‐UUCUCCGAACGUGUCACGUTT‐3 and antisense primer 5‐ACGUGACACGUUCGGAGAATT‐3.
Statistical analysis
The values obtained from at least three independent experiments were presented as means ± standard error. Significant differences among study groups were determined by one-way analysis of variance and Tukey’s multiple comparison test. A p-value < 0.05 was considered statistically significant.
Results
Beneficial time of CIH exposure and concentration of gAPN
As shown in ), cellular viability decreased significantly after 24 h (82.15%±1.022, P= 0.0002), 48 h (73.11%±2.957, P< 0.0001), and 72 h (74.8%±1.589, P< 0.0001) CIH exposure, compared to the NC group, using CCK-8 assays. Cell viability in the 24 h CIH group was higher than in the 48 h CIH group (P= 0.0257). No difference in cell viability was observed between the 48 h CIH group and the 72 h CIH group (P= 0.9536), which is the same results observed in the NC group and 12 h (93.41%±1.561) CIH group (P= 0.1213). 48 h CIH exposure was selected for further study.
Figure 1. Effects of various CIH time periods and varying gAPN concentrations on H9C2 cardiomyocytes viability using CCK-8 analysis. (a) H9C2 cardiomyocytes viability under different CIH time periods. (b) H9C2 cardiomyocytes viability under different concentrations of gAPN. Data represent mean ± SD. n = 3. *P< 0.05, vs NC group. #P< 0.05, vs. 12 h CIH group; +P< 0.05, vs. 24 h CIH group; &P< 0.05, vs. 48 h CIH group; ^P< 0.05, vs. 48 h CIH + 0.5 µg/ml gAPN group
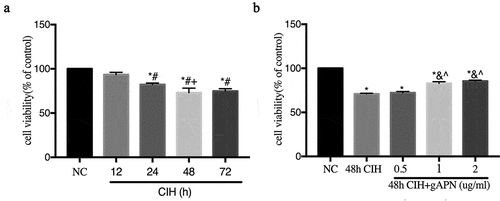
As shown in ), the H9C2 cardiomyocytes were pretreated with different concentrations of gAPN (0, 0.5, 1, and 2 µg/mL) followed by 48 h CIH exposure. Cellular viability decreased in all CIH exposure groups compared to the NC group (all P< 0.05). After treatment with gAPN at the concentrations of 1 (82.91%±1.071, P< 0.0001) and 2 µg/mL (85.44%±0.6311, P< 0.0001), the cell viability was significantly higher than that observed in the CIH exposure group (70.97%±0.4173). No statistically significant difference was observed between the concentrations of 1 and 2 µg/mL groups (P= 0.1518). The 1 µg/mL treatment was chosen as the model concentration.
gAPN mitigated CIH-induced H9C2 cardiomyocytes apoptosis
Flow cytometry was used to explore cellular apoptosis, post-exposure to CIH conditions with or without gAPN pretreatment (). Compared to the NC group, CIH stimulation markedly increased the apoptosis rate (3.047%±0.061 vs 15.35%±0.696, P< 0.0001). Pretreatment with gAPN significantly reduced the CIH-induced cardiomyocytes apoptosis rate (10.06%±0.313 vs 15.35%±0.696, P< 0.0001). 4-PBA is an inhibitor of ER stress. After treatment with 4-PBA, the H9C2 cardiomyocytes apoptosis rate in the CIH+4-PBA group appeared markedly reduced compared to CIH groups (10.05%±0.176 vs 15.35%±0.696, P< 0.0001) while the apoptosis rate in the CIH+gAPN+4-PBA group also decreased compared with the CIH+gAPN group (6.237%±0.559 vs 10.06%±0.313, P= 0.0007).
Figure 2. Effect of ER stress on H9C2 cardiomyocytes exposed to CIH (48 h), gAPN (1 µg/ml) and 4-PBA (5 mM). (a, c) Flow cytometry images representing H9C2 cardiomyocytes apoptosis. (b, d–i) Protein expression levels indicating ER stress (BIP, CHOP, IRE1, p-IRE1, p-PERK, PERK, pro-ATF6, cleaved Caspase-12). Data represent mean ± SD. n = 3. *P< 0.05, vs NC group. #P< 0.05, vs CIH group. &P< 0.05, vs CIH+gAPN group
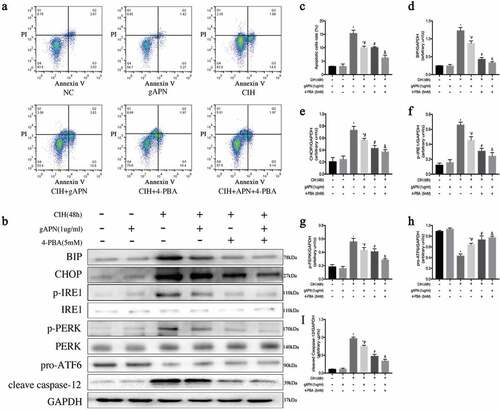
gAPN protected H9C2 cardiomyocytes against CIH-induced cellular apoptosis by inhibiting ER stress
The high protein levels of BIP, p-IRE1, and p-PERK, and low protein level of pro-ATF6 represent ER stress. High protein levels of CHOP and cleaved caspase-12 represent ER stress-associated cell apoptosis. To study the effect of ER stress, the above protein levels were determined. As shown in , CIH markedly increased BIP, CHOP, p-IRE1, p-PERK, and cleaved caspase-12 protein levels, but significantly decreased pro-ATF6 (P< 0.05) compared to the NC group. Compared with the CIH group, gAPN treatment decreased BIP, CHOP, p-IRE1, p-PERK, and cleaved caspase-12 protein levels, and increased pro-ATF6 protein level even under CIH conditions (P< 0.05). When treated with 4-PBA, the protein levels of BIP, CHOP, p-IRE1, p-PERK, and cleaved caspase-12 appeared markedly reduced, while the protein expression level of pro-ATF6 markedly increased in the CIH+4-PBA group compared to the CIH groups (P< 0.05), which is the same results observed in the CIH+gAPN+4-PBA group compared to the CIH+gAPN group (P< 0.05). These results suggested that both ER stress and its associated apoptosis pathway were activated by CIH, and gAPN protected H9C2 cardiomyocytes against CIH-induced apoptosis by inhibiting ER stress and ER stress-associated apoptosis pathway.
gAPN upregulated ER-phagy in H9C2 cardiomyocytes exposed to CIH
ER-phagy plays a protective role, which can mitigate ER stress. To evaluate whether the protective role of gAPN is associated with ER-phagy, we observed the fluorescence co-localization of LC3 and ER-Red (), and the protein level of LC3B (, e)), which consists of LC3-I and LC3-II. LC3-II is regarded as a marker of autophagy activation. After CIH exposure, ER-phagy dots and LC3-II protein level were significantly increased compared to the NC group (P< 0.05). gAPN pretreatment under CIH conditions remarkably increased ER-phagy dots and the LC3-II protein level compared with the CIH group (P< 0.05).
Figure 3. ER-phagy and SEC62 activation in H9C2 cardiomyocytes exposed to CIH (48 h) or with gAPN (1 µg/ml). (a, d) Fluorescence staining of LC3 (green) co-localized with the ER probe (red). Nuclei were stained with DAPI (blue). The scale bars represent 20 μm. (b, e–f) Protein expression levels of LC3II and SEC62. (c, g) Fluorescence staining of LC3 (green) co-localized with SEC62 (red). Nuclei were stained with DAPI (blue). The scale bars represent 10 μm. Data represent mean ± SD. n = 3. *P< 0.05, vs NC group. #P< 0.05, vs CIH group
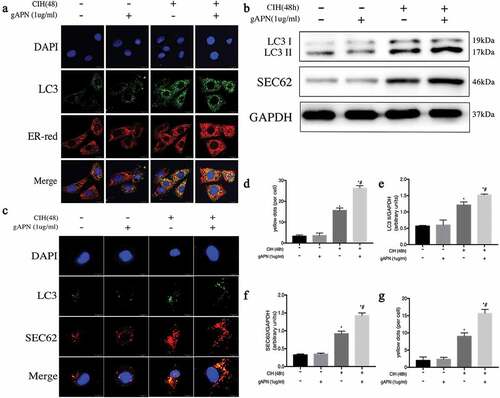
SEC62 is an ER-phagy receptor. To investigate whether SEC62 was activated in the ER-phagy process in H9C2 cardiomyocytes exposed to CIH or gAPN, the SEC62 protein level and fluorescence co-localization of SEC62 and LC3 were determined ()). After CIH exposure, SEC62 protein level and yellow dots were higher in the NC group (P< 0.05). Pretreating with gAPN under CIH conditions significantly increased the protein level and the yellow dots compared to CIH exposure (P< 0.05). These results suggest that SEC62-mediated ER-phagy was activated in gAPN-treated H9C2 cardiomyocytes to protect against CIH injury.
Gene silencing of SEC62 inhibited ER-phagy in H9C2 cardiomyocytes
To further study the effect of ER-phagy on ER stress, SEC62 was inhibited by SEC62 siRNA transfection. First, we verified the effect of SEC62 siRNA on H9C2 cardiomyocytes by examining the protein level of SEC62. As shown in ), the protein expression level of SEC62 was significantly lower after SEC62 siRNA transfection than in the control siRNA transfected cell or untreated cell groups (P< 0.05). There were no differences in SEC62 expression level between control siRNA transfected cells and untreated cell groups (P> 0.05). As shown in , ), protein levels of SEC62 and LC3-II, and ER-phagy dots in the CIH+ control siRNA group appeared higher than that in the control siRNA group (P< 0.05). The SEC62 and LC3-II levels, and ER-phagy dots in the CIH + gAPN + control siRNA group were markedly increased compared to the CIH + control siRNA group (P< 0.05). After inhibiting SEC62 gene expression, these changes in the CIH + SEC62 siRNA group were all decreased compared to the CIH + control siRNA group (P< 0.05), which is the same results observed in the CIH + gAPN + SEC62 siRNA group compared to the CIH + gAPN + control siRNA group. These results further proved that SEC62 was related to ER-phagy in this study.
Figure 4. Role of SEC62 siRNA transfection on ER-phagy in H9C2 cardiomyocytes exposed to CIH (48 h), or with gAPN (1 µg/ml). (a, d) Protein level of SEC62 to verify transfection efficiency. (b, e–f) Protein levels of LC3-II and SEC62. (c, g) Fluorescence staining of LC3 (green) co-localized with the ER probe (red). Nuclei were stained with DAPI (blue). The scale bars represent 20 μm. Data represent mean ± SD. n = 3. *P< 0.05, vs control siRNA group. #P< 0.05, vs CIH+ control siRNA group. &P< 0.05, vs CIH+gAPN+control siRNA group
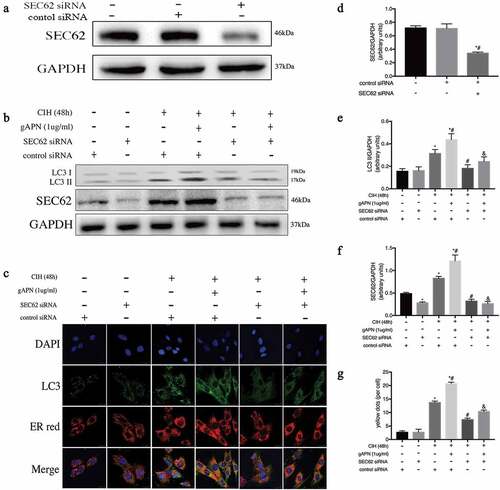
Inhibition of ER-phagy increased ER stress and H9C2 cardiomyocytes apoptosis
ER stress and H9C2 cardiomyocytes apoptosis were detected when ER-phagy was inhibited by SEC62 siRNA transfection. As shown in , CIH exposure significantly increased cell apoptosis rate (17.7%±0.873 vs 4.183%±0.305, P< 0.0001) and BIP, CHOP, p-IRE1, p-PERK, and cleaved caspase-12 protein levels but decreased pro-ATF6 protein level compared with the control siRNA group (P< 0.05). Compared to the CIH+control siRNA group, gAPN pretreatment with control siRNA transfection reduced the CIH-increased BIP, CHOP, p-IRE1, p-PERK, and cleaved caspase-12 expression levels and cell apoptosis rate (17.7%±0.873 vs 11.8%±0.4619, P= 0.005), while increasing the CIH-decreased protein expression of pro-ATF6 in H9C2 cardiomyocytes (P< 0.05). After inhibiting SEC62 gene expression, the expression levels of BIP, CHOP, p-IRE1, p-PERK, and cleaved caspase-12 and the cell apoptosis rate increased, while the protein level of pro-ATF6 decreased compared to control siRNA transfected cells under CIH condition (24.67%±0.731 vs 17.7%±0.873, P= 0.0013), which is the same results observed in the CIH+gAPN+SEC62 siRNA compared to CIH+ gAPN+control siRNA (cell apoptosis: 18.1%±1.682 vs 11.8%±0.4619, P= 0.003). The results showed that gAPN ameliorated CIH-induced ER stress and ER stress-associated apoptosis by increasing SEC62-mediated ER-phagy.
Figure 5. Effect of inhibiting ER-phagy on ER stress and cell apoptosis by SEC62 siRNA transfection in H9C2 cardiomyocytes exposed to CIH (48 h), or gAPN (1 µg/ml). (a, c) Flow cytometry images represent H9C2 cardiomyocytes apoptosis. (b, d–i) Protein expression levels of ER stress (BIP, CHOP, IRE1, p-IRE1, p-PERK, PERK, pro-ATF6, cleaved caspase-12). Data represent mean ± SD. n = 3. *P< 0.05, vs control siRNA group. #P< 0.05, vs CIH+ control siRNA group. &P< 0.05, vs CIH+gAPN+control siRNA group
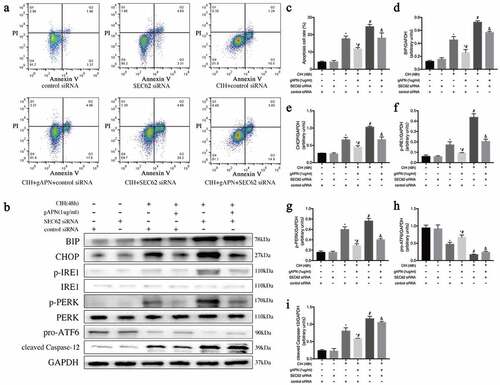
Inhibition of AMPK expression increased H9C2 cardiomyocytes apoptosis
AMPK singling was studied to evaluate how gAPN affected ER-phagy. Compound C, a selective inhibitor of AMPK, was used in this study. As shown in , CIH exposure increased cellular apoptosis compared to the NC group (17.27%±1.618 vs 3.41%±0.865, P< 0.0001), while gAPN supplementation decreased CIH-induced cellular apoptosis compared with the CIH group (9.6%±0.356 vs 17.27%±1.618, P= 0.002). Post-inhibition using compound C, H9C2 cardiomyocytes apoptosis in the CIH + gAPN + compound C group was higher than that in the CIH + gAPN group (13.9%±0.288 vs 19.6%±0.356, P= 0.0491).
Figure 6. Effect of inhibiting AMPK using compound C (10 µM) on cell apoptosis and ER-phagy in H9C2 cardiomyocytes exposed to CIH (48 h), gAPN (1 µg/ml). (a, c) Flow cytometry images represent H9C2 cardiomyocytes apoptosis. (b, d–f) Protein expressions of LC3-II, p-AMPK and SEC62. Data represent mean ± SD. n = 3. *P< 0.05, vs NC group. #P< 0.05, vs CIH group. &P< 0.05, vs CIH+gAPN group
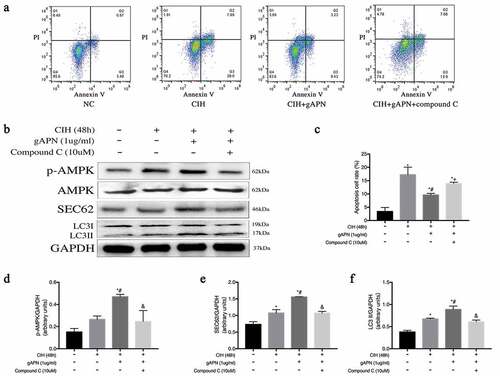
AMPK signaling pathway was involved in gAPN-induced SEC62-mediated ER-phagy in CIH-treated H9C2 cardiomyocytes
As shown in , compared with NC group, CIH exposure increased SEC62 and LC3-II protein levels. gAPN supplementation highly increased SEC62, LC3-II, and p-AMPK protein levels compared to CIH group (P< 0.05). However, treatment with compound C decreased these protein levels when compared to the CIH+gAPN group (P< 0.05).
As shown in , compared with NC group, CIH exposure increased ER-phagy dots and SEC62 co-localization of LC3 dots. Additionally, gAPN pretreatment with CIH exposure significantly increased these changes compared with the CIH group (P< 0.05). However, treatment with compound C decreased these changes (P< 0.05) when compared to the CIH+gAPN group (P< 0.05). These data suggested that gAPN upregulated the SEC62-mediated ER-phagy through activating AMPK pathway in CIH-exposed H9C2 cardiomyocytes.
Figure 7. Effect of inhibiting AMPK using compound C (10 µM) on ER-phagy and SEC62 expression in H9C2 cardiomyocytes exposed to CIH (48 h), gAPN (1 µg/ml). (a, c) Fluorescence staining of LC3 (green) co-localized with the ER probe (red). Nuclei were stained with DAPI (blue). The scale bars represent 20 μm. (b, d) Fluorescence staining of LC3 (green) co-localized with the SEC62 (red). Nuclei were stained with DAPI (blue). The scale bars represent 10 μm. Data represent mean ± SD. n = 3. *P< 0.05, vs NC group. #P< 0.05, vs CIH group. &P< 0.05, vs CIH+gAPN group
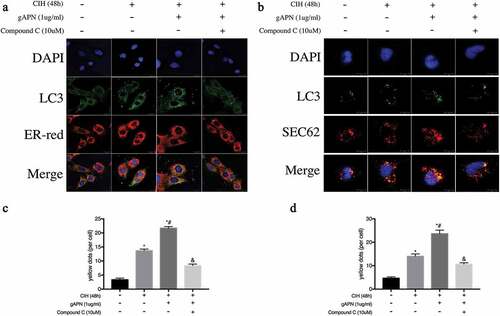
Discussion
In this study, we established a cell model to study the possible mechanism of CIH-induced H9C2 cardiomyocytes injury and the protective effect of gAPN. The results demonstrated that ER stress, ER stress-associated H9C2 cell apoptosis, and SEC62-mediated ER-phagy were activated by CIH exposure. Further, gAPN upregulated SEC62-mediated ER-phagy via activating AMPK pathway to ameliorate ER stress and ER stress-associated apoptosis induced by CIH.
An estimated 31% of deaths worldwide are attributed to CVD, while OSA is the most underdiagnosed risk factor for CVD [Citation12]. Continuously OSA leads to myocardial injury, the development and progression of myocardial dysfunction, and even heart failure [Citation13]. It was reported that 50%-70% of patients with heart failure might also have sleep apnea [Citation14]. Previous research indicated that CIH-induced cardiac dysfunction in a rat model [Citation6,Citation15] and apoptosis in H9C2 and human arterial cells [Citation16,Citation17]. In this study, H9C2 cardiomyocytes apoptosis was observed post-CIH exposure. These findings further verified CIH-induced damage to heart cells.
ER, as an important organelle, modulates protein biosynthesis and folding, lipid biosynthesis, and cell homeostasis. When homeostasis is disrupted, excess unfolded or misfolded proteins accumulate in the ER lumen, impair the ER function, and lead to ER stress [Citation18]. To combat this dysfunctional protein accumulation, the cell activates the unfolded protein response (UPR). ER stress initially performs a protective role; however, should this process persist, ER stress may eventually lead to cell death [Citation19]. UPR has three pathways: namely IRE1, PERK, and ATF6 pathways. All pathways can induce CHOP gene expression. CHOP and cleaved caspase-12 expressions are recognized as special proteins related to ER stress-associated apoptosis [Citation20–22]. Evidence shows that ER stress is involved in serious diseases, such as diabetes, nonalcoholic liver disease, and cardiovascular disease [Citation23–25]. In our previous study, ER stress and ER stress-associated apoptosis pathways were activated and contributed to the cardiac dysfunction of CIH-exposed rats [Citation6]. In this study, we found that CIH exposure activated the UPR, increased the expressions of CHOP and caspase-12, and activated the ER stress-associated apoptosis. Inhibiting ER stress ameliorated CHOP and cleaved caspase-12 expression, as well as cellular apoptosis, even under CIH condition. Similar to our study, many studies showed that ischemia/reperfusion induced H9C2 cardiomyocytes apoptosis by activating ER stress [Citation26–28]. These results proved that ER stress and ER stress-associated apoptosis pathway contributed to CIH-induced H9C2 cardiomyocytes apoptosis.
gAPN, as a cleavage fragment of adiponectin, also has its biological activity. It has been reported that gAPN inhibited the oxidative stress in endothelial cells under varying conditions [Citation29,Citation30]. Tao found that gAPN protected against cardiac ischemia/reperfusion injury by inhibiting oxidative stress [Citation31]. Kim reported that gAPN reduced hepatocytes apoptosis induced by acetaminophen through inhibiting ER stress [Citation32]. Our previous study demonstrated that gAPN could eliminate the ER stress-associated myocardial cell apoptosis under CIH condition in rats [Citation6]. Consistent with these studies, our study found that pretreatment with gAPN eliminated CIH-induced ER stress and ER stress-associated H9C2 cardiomyocytes apoptosis. Meanwhile, increased inhibition of ER stress with gAPN and 4-PBA further reduced CIH-induced cardiomyocytes apoptosis. These results indicated that gAPN could protect H9C2 cardiomyocytes against CIH-induced apoptosis via ER stress inhibition.
ER-phagy consists of ER membrane, degrades the dysfunctional ER and misfolded proteins, and attenuates ER stress. ER-phagy is a branch of autophagy and is essential for maintaining the cellular homeostasis [Citation33,Citation34]. ER-phagy is reportedly associated with neurodegenerative disease, cardiovascular disease, fatty liver, and the progression of several cancers [Citation9,Citation35–37]. Our results showed that the ER-phagy was activated after CIH exposure in H9C2 cardiomyocytes. Kim reported that gAPN could induce autophagy in macrophages [Citation38]. Nepal found that gAPN increased autophagy flux and then suppressed ethanol-induced liver cell death via modulating Atg5 [Citation39]. However, there is little available research regarding gAPN and ER-phagy. Our study showed that pretreating with gAPN further promoted ER-phagy under CIH exposure. Since gAPN reduced CIH-induced apoptosis of H9C2 cardiomyocytes, these results suggested that ER-phagy was activated by CIH, while gAPN mitigated H9C2 cardiomyocytes injury through ER-phagy upregulation.
SEC62, one of the four ER-phagy receptors in mammalian cells, is an ER-resident transmembrane protein that can translocate the newly synthesized proteins to the ER lumen for further folding. SEC62 is considered the receptor of ER-phagy because it can regulate the ER turnover after the conclusion of a transient ER stress [Citation40]. SEC62-mediated ER-phagy regulates catabolic processes and reestablishes pre-stress ER volume and ER content, which have been defined as recovER-phagy [Citation40]. SEC62 contains the LC3-interacting region, which is involved in the development of ER-phagy [Citation33]. The fluorescence co-localization of SEC62 and LC3 indicated that SEC62 activation played a role in ER-phagy [Citation33]. Fumagalli F found that silencing of SEC62 gene expression in MEFs could inhibit ER protein markers transfer to the autolysosomes in MEFs [Citation40]. SEC62-mediated ER-phagy plays an important role in specifically eliminating ER subdomains and maintaining ER homeostasis [Citation34,Citation40]. In this study, we found that CIH exposure activated SEC62, demonstrated by the high SEC62 protein expression level and SEC62 co-located LC3 dots; however, gAPN treatment greatly increased SEC62 protein expression and SEC62 co-located LC3 dots. These results suggested that SEC62 is involved in ER-phagy in this study.
To further explore ER-phagy and the role of SEC62, we silenced SEC62 gene expression. When SEC62 gene expression was inhibited by siRNA, ER-phagy was significantly decreased, regardless of whether cells were exposed to CIH or CIH with gAPN treatment. These results further demonstrated that SEC62 was involved in ER-phagy activation. As mentioned above, ER-phagy can degrade dysfunctional ER and misfolded proteins, and attenuate ER stress [Citation33,Citation34]. We found that ER-phagy inhibited by SEC62 siRNA significantly exacerbated the ER stress and cell apoptosis in H9C2 cardiomyocytes under CIH condition. In addition, this study also showed that inhibition of ER-phagy by SEC62 siRNA increased gAPN-reduced ER stress and H9C2 cardiomyocytes apoptosis. These results demonstrated that SEC62-mediated ER-phagy played a protective role, while gAPN upregulated ER-phagy and further extenuated ER stress and cellular apoptosis in CIH-treated H9C2 cardiomyocytes via SEC62 activation.
It has been demonstrated that gAPN exerts biological effects via AMPK activation, which is the downstream target of adiponectin after contacting with its receptor [Citation41]. Activating AMPK pathway by gAPN has several protective efficacies, such as pro-angiogenic, and anti-apoptotic functions, as well as upregulation of nitric oxide production [Citation41]. Kim found that gAPN modulated autophagy through activating AMPK signaling in the lipopolysaccharide-induced inflammasomes of macrophages [Citation38]. Consistent with these studies, we also found that gAPN significantly upregulated protein expression of p-AMPK which represents AMPK activation, while treatment with compound C to inhibit AMPK reduced the SEC62 activation and ER-phagy, and increased the H9C2 cardiomyocytes apoptosis. These data indicated that gAPN induced the SEC62-mediated ER-phagy and reduced the H9C2 cardiomyocytes apoptosis via AMPK activation.
However, there are some limitations of this study. First, since the difference of survival environment for cells in vivo and in vitro, the CIH protocol in vitro in this study could not completely simulate OSA model in vivo. Second, lacking of in vivo validation is another limitation of this study.
Conclusion
This study demonstrated that activation of SEC62-mediated ER-phagy protected the H9C2 cardiomyocytes against CIH injury. gAPN upregulated the SEC62-mediated ER-phagy and extenuated the CIH-induced ER stress and ER stress-associated apoptosis via AMPK activation ().
Figure 8. Possible mechanisms of CIH-induced H9C2 cardiomyocytes injury and gAPN protective role. In our study, we found that CIH exposure activated the ER stress and ER stress-associated H9C2 cardiomyocytes apoptosis. Meanwhile, CIH activated the SEC62-associated ER-phagy to alleviate ER stress and further reduce the cell apoptosis. gAPN supplementation upregulated the SEC62-associated ER-phagy to alleviate ER stress and reduce the cell apoptosis via activating AMPK pathway
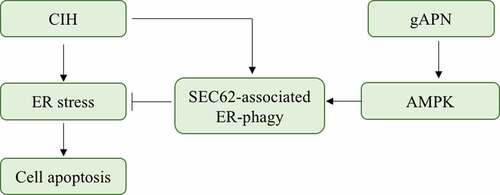
Disclosure statement
The authors declare that they have no potential conflict of interest.
Additional information
Funding
References
- Peppard PE, Young T, Barnet JH, et al. Increased prevalence of sleep-disordered breathing in adults. Am J Epidemiol. 2013;177:1006–1014.
- Perger E, Gonzaga-Carvalho C, Inami T, et al. Obstructive sleep apnea and cardiovascular disease. Am J Respir Crit Care Med. 2019;199:377–379.
- Mazzotti DR, Keenan BT, Lim DC, et al. Symptom subtypes of obstructive sleep apnea predict incidence of cardiovascular outcomes. Am J Respir Crit Care Med. 2019;200(4):493–506.
- Wang Y, Ma XL, Lau WB. Cardiovascular adiponectin resistance: the critical role of adiponectin receptor modification. Trends Endocrinol Metab. 2017;28:519–530.
- Al Mutairi S, Mojiminiyi OA, Al Alawi A, et al. Study of leptin and adiponectin as disease markers in subjects with obstructive sleep apnea. Dis Markers. 2014;2014:706314.
- Ding W, Zhang X, Huang H, et al. Adiponectin protects rat myocardium against chronic intermittent hypoxia-induced injury via inhibition of endoplasmic reticulum stress. PLoS One. 2014;9:e94545.
- Smith MD, Wilkinson S. CCPG1, a cargo receptor required for reticulophagy and endoplasmic reticulum proteostasis. Autophagy. 2018;14:1090–1091.
- Mizushima N. A dual binding receptor for ER-phagy. Dev Cell. 2018;44:133–135.
- Li L, Xu J, Chen L, et al. Receptor-mediated reticulophagy: a novel promising therapy target for diseases. Acta Biochim Biophys Sin (Shanghai). 2016;48:774–776.
- Wu J, Stefaniak J, Hafner C, et al. Intermittent hypoxia causes inflammation and injury to human adult cardiac myocytes. Anesth Analg. 2016;122:373–380.
- Wang Y, Zhao Z, Wei F, et al. Combining autophagy-inducing peptides and brefeldin A delivered by perinuclear-localized mesoporous silica nanoparticles: a manipulation strategy for ER-phagy. Nanoscale. 2018;10:8796–8805.
- Benjamin EJ, Blaha MJ, Chiuve SE, et al. Heart disease and stroke statistics-2017 update: a report from the American heart association. Circulation. 2017;135:e146–e603.
- Tietjens JR, Claman D, Kezirian EJ, et al. Obstructive sleep apnea in cardiovascular disease: a review of the literature and proposed multidisciplinary clinical management strategy. J Am Heart Assoc. 2019;8:e010440.
- Oldenburg O, Teerlink JR. Screening for sleep-disordered breathing in patients hospitalized for heart failure. JACC Heart Fail. 2015;3:732–733.
- Xie S, Deng Y, Pan YY, et al. Chronic intermittent hypoxia induces cardiac hypertrophy by impairing autophagy through the adenosine 5ʹ-monophosphate-activated protein kinase pathway. Arch Biochem Biophys. 2016;606:41–52.
- Lin G, Huang J, Chen Q, et al. miR-146a-5p mediates intermittent hypoxia-induced injury in H9c2 cells by targeting XIAP. Oxid Med Cell Longev. 2019;2019:6581217.
- Zhang X, Rui L, Lv B, et al. Adiponectin relieves human adult cardiac myocytes injury induced by intermittent hypoxia. Med Sci Monit. 2019;25:786–793.
- Fernandez A, Ordonez R, Reiter RJ, et al. Melatonin and endoplasmic reticulum stress: relation to autophagy and apoptosis. J Pineal Res. 2015;59:292–307.
- Sun X, Liao W, Wang J, et al. CSTMP induces apoptosis and mitochondrial dysfunction in human myeloma RPMI8226 cells via CHOP-dependent endoplasmic reticulum stress. Biomed Pharmacother. 2016;83:776–784.
- Ma J, Yang YR, Chen W, et al. Fluoxetine synergizes with temozolomide to induce the CHOP-dependent endoplasmic reticulum stress-related apoptosis pathway in glioma cells. Oncol Rep. 2016;36:676–684.
- Rozpedek W, Pytel D, Mucha B, et al. The role of the PERK/eIF2alpha/ATF4/CHOP signaling pathway in tumor progression during endoplasmic reticulum stress. Curr Mol Med. 2016;16:533–544.
- Zhang Q, Liu J, Chen S, et al. Caspase-12 is involved in stretch-induced apoptosis mediated endoplasmic reticulum stress. Apoptosis. 2016;21:432–442.
- Ivanova EA, Orekhov AN. The role of endoplasmic reticulum stress and unfolded protein response in atherosclerosis. Int J Mol Sci. 2016;17(2):193.
- Clark AL, Urano F. Endoplasmic reticulum stress in beta cells and autoimmune diabetes. Curr Opin Immunol. 2016;43:60–66.
- Pagliassotti MJ. Endoplasmic reticulum stress in nonalcoholic fatty liver disease. Annu Rev Nutr. 2012;32:17–33.
- Chen M, Liu Q, Chen L, et al. Remifentanil postconditioning ameliorates histone H3 acetylation modification in H9c2 cardiomyoblasts after hypoxia/reoxygenation via attenuating endoplasmic reticulum stress. Apoptosis. 2017;22:662–671.
- Guan G, Zhang J, Liu S, et al. Glucagon-like peptide-1 attenuates endoplasmic reticulum stress-induced apoptosis in H9c2 cardiomyocytes during hypoxia/reoxygenation through the GLP-1R/PI3K/Akt pathways. Naunyn-Schmiedeberg’s Arch Pharmacol. 2019;392:715–722.
- Jian L, Lu Y, Lu S, et al. Chemical chaperone 4-phenylbutyric acid protects H9c2 cardiomyocytes from ischemia/reperfusion injury by attenuating endoplasmic reticulum stress-induced apoptosis. Mol Med Rep. 2016;13:4386–4392.
- Ouedraogo R, Wu X, SQ X, et al. Adiponectin suppression of high-glucose-induced reactive oxygen species in vascular endothelial cells: evidence for involvement of a cAMP signaling pathway. Diabetes. 2006;55:1840–1846.
- Motoshima H, Wu X, Mahadev K, et al. Adiponectin suppresses proliferation and superoxide generation and enhances eNOS activity in endothelial cells treated with oxidized LDL. Biochem Biophys Res Commun. 2004;315:264–271.
- Tao L, Gao E, Jiao X, et al. Adiponectin cardioprotection after myocardial ischemia/reperfusion involves the reduction of oxidative/nitrative stress. Circulation. 2007;115:1408–1416.
- Kim EH, Park PH. Globular adiponectin protects rat hepatocytes against acetaminophen-induced cell death via modulation of the inflammasome activation and ER stress: critical role of autophagy induction. Biochem Pharmacol. 2018;154:278–292.
- Loi M, Fregno I, Guerra C, et al. Eat it right: ER-phagy and recovER-phagy. Biochem Soc Trans. 2018;46:699–706.
- Fregno I, Molinari M. Endoplasmic reticulum turnover: ER-phagy and other flavors in selective and non-selective ER clearance. F1000Res. 2018;7:454.
- Lennemann NJ, Coyne CB. Dengue and Zika viruses subvert reticulophagy by NS2B3-mediated cleavage of FAM134B. Autophagy. 2017;13:322–332.
- Wemmert S, Lindner Y, Linxweiler J, et al. Initial evidence for Sec62 as a prognostic marker in advanced head and neck squamous cell carcinoma. Oncol Lett. 2016;11:1661–1670.
- Grumati P, Dikic I, Stolz A. ER-phagy at a glance. J Cell Sci. 2018;131(17):jcs217364.
- Kim MJ, Kim EH, Pun NT, et al. Globular adiponectin inhibits lipopolysaccharide-primed inflammasomes activation in macrophages via autophagy induction: the critical role of AMPK signaling. Int J Mol Sci. 2017;18(6):1275.
- Nepal S, Kim MJ, Lee ES, et al. Modulation of Atg5 expression by globular adiponectin contributes to autophagy flux and suppression of ethanol-induced cell death in liver cells. Food Chem Toxicol. 2014;68:11–22.
- Fumagalli F, Noack J, Bergmann TJ, et al. Translocon component Sec62 acts in endoplasmic reticulum turnover during stress recovery. Nat Cell Biol. 2016;18:1173–1184.
- Orlando A, Nava E, Giussani M, et al. Adiponectin and cardiovascular risk. From pathophysiology to clinic: focus on children and adolescents. Int J Mol Sci. 2019;20(13):3228.