ABSTRACT
Dexmedetomidine (DEX) could serve as an adjuvant analgesic during cancer therapies. Abnormal expression of microRNAs (miRNAs) could lead to cancer development. This study was aimed to explore the roles of DEX in ovarian cancer (OC) development. OC cell lines SKOV3 and HO-8910 were treated with DEX, after which OC development and the miR-185, SOX9, and Wnt/β-catenin pathway were measured. DEX-treated HO-8910 cells were transfected with miR-185 mimic, miR-185 antisense or miR-185 antisense + silenced SOX9 to further measure the OC cell growth. The target relation between miR-185 and SOX9 was identified, and SOX9 and Wnt/β-catenin pathway were protein levels detected after miR-185 transfection. The role of miR-185 in OC in vivo was also measured. Our study found DEX had a dose-dependent inhibition on OC growth, and DEX promoted miR-185 but suppressed SOX9 expression in OC cells. miR-185 targeted SOX9. After interfering with miR-185 expression, HO-8910 cell proliferation, invasion, migration, and apoptosis were affected. SOX9 knockdown repressed OC development and Wnt/β-catenin pathway. The volume, weight, positive rate of Ki67, CyclinD1, p53 and the degree of tumor necrosis were affected by miR-185 expression. This study demonstrated that DEX could inhibit OC development via upregulating miR-185 expression and inactivating the SOX9/Wnt/β-catenin signaling pathway.
Introduction
Ovarian cancer (OC) is a common deadly gynecological malignancy which accounts for about 3% of all cancer occurrence in the female, with 240,000 cases newly found and 150,000 cases dead annually around the world [Citation1]. Owing to the lack of early specific symptoms, OC is always diagnosed at advanced stages when it is hard to be cured by simple surgical resection, and frequent relapses during the clinical course [Citation2], which in turn contributes to unfavorable prognosis and poor survival rate of OC patients [Citation3,Citation4]. Despite the efficient surgical debulking and the application of different anticancer drugs, the overall 5-year survival rate of advanced OC patients is less than 40% with only modest improvement over decades [Citation5,Citation6]. Investigating biomarkers enabling early diagnosis of OC and developing novel therapies continue to be fundamental goals for researchers in the field of OC.
Dexmedetomidine (DEX) is a strong α2-adrenoceptor agonist that is widely used for its anxiolytic, sedative, and analgesic attributes [Citation7]. DEX could serve as an adjuvant analgesic in cancer patients undergoing surgical and nonsurgical procedures [Citation8,Citation9]. Meanwhile, the positive effects of DEX such as reducing respiratory depression and hypotension, and decreasing lung and kidney damage and neuronal protection have been well-established [Citation10–12]. Considering these effects of DEX, one can presume beneficial roles of DEX in cancer patients. MicroRNAs (miRNAs) are small noncoding RNAs which regulate the translation of diverse genes and serve as ideal biomarkers for cancer prognosis [Citation13]. miRNAs play crucial roles in various essential biological processes including cell growth, differentiation, inflammation and tumor development, whose abnormal expression could lead to several diseases including cancer [Citation14,Citation15]. Among them, miR-185 has been revealed as a tumor inhibitor in several cancers such as nasopharyngeal carcinoma [Citation16], non-small cell lung cancer (NSCLC) [Citation17] and OC [Citation18]. Importantly, miR-185 negatively targets the 3ʹ‑untranslated region (3ʹ-UTR) of SRY-box 9 (SOX9) in a former study [Citation19]. Aberrant expression of SOX9 has been suggested participating in the pathogenesis of several cancers [Citation20,Citation21]. Likewise, abnormal SOX9 expression has been demonstrated to promote OC cell survival in hypoxic condition and to affect OC aggressiveness [Citation22]. Meanwhile, a previous study has identified that SOX9 could trigger Wnt/β-catenin pathway activation in prostate cancer [Citation23]. It is well known that the Wnt/β-catenin signaling pathway plays critical roles in cancer development [Citation24]. A recent study suggested that inhibition of Wnt/β-catenin could suppress stemness and drug resistance of OC cells [Citation25]. However, the effects of DEX and the cytokines above on OC remain unknown. Hence, this study was designed to explore the roles of DEX and these potential biomarkers in OC development.
Materials and methods
Ethics statement
The study was approved by the Clinical Ethical Committee of Guangzhou Medical University. All experimental procedures were performed in line with the ethical guidelines for the study of experimental pain in conscious animals.
Cell culture
Human OC cell lines SKOV3 and HO-8910 (purchased from Institute of Cell Biology, Chinese Academy of Sciences, Shanghai, China) were cultured in a 37°C constant incubator with 5% CO2, and the culture solution was refreshed every day. Cells were then passaged when the cell confluence reached 80%.
Cell transfection and grouping
The well-grown SKOV3 and HO-8910 cells at passage 3 were randomly assigned into four groups, which were, respectively, treated with 0 nmol/L, 1 nmol/L, 10 nmol/L and 100 nmol/L DEX to measure the effect of different doses of DEX on cell growth and to select the best dose of DEX for the following transfection.
Cell transfection: the HO-8910 cells were treated with an appropriate dose of DEX for 24 h. After that, the HO-8910 cells were randomized into DEX + negative control (NC) group (transfected with mimic NC), DEX + miR-185 group (transfected with miR-185 mimic), DEX + antisense NC group (transfected with antisense NC), DEX + anti-miR-185 group (transfected with miR-185 antisense) and DEX + anti-miR-185 + si-SOX9 group (transfected with miR-185 antisense and SOX9 siRNA). The transfections were performed as per the instructions of a LipofectamineTM 2000 kit (Invitrogen Inc., Carlsbad, CA, USA). The transfection efficiencies were assessed with reverse transcription-quantitative polymerase chain reaction (RT-qPCR) 24 h following transfection.
3-(4, 5-dimethylthiazol-2-yl)-2, 5-diphenyltetrazolium bromide (MTT) assay
Cells in each group were seeded onto 96-well plates (1 × 104 cells/well) and then incubated at 37°C with 5% CO2 for 24 h, and 6 duplicated wells were set for each group. Thereafter, each well was treated with 5 mg/mL MTT solution (Sigma-Aldrich Chemical Company, St Louis, MO, USA) and the cells were cultured for 4 h. A total of 200 μL dimethyl sulfoxide was added after the supernatant was discarded. Following 10 min of vibration, the optical density (OD) value at 490 nm of each well was evaluated using a microplate reader, and the average value of 6 wells was recorded.
5-ethynyl-2ʹ-deoxyuridine (EdU) labeling assay
In accordance with the instructions of an EdU assay kit purchased from Guangzhou RiboBio Co., Ltd (Guangzhou, Guangdong, China), the cells were seeded onto 48-well plates (8000 cells/well) and incubated with 20 μmol/L EdU for 24. After cells were fixed for 30 min with 4% polyformaldehyde fixing and permeated for 10 min with 0.5% Triton X-100, each well of cells were stained with 150 μL reaction solution for 30 min and 150 μL 1 × Hochest33258 for 5 min. A fluorescence microscope (Olympus Optical Co., Ltd, Tokyo, Japan) was utilized to observe the cells. Five areas were randomly selected, in which the blue fluorescence refers to all cells while the red fluorescence indicates the replicating cells influenced by EdU, and then the EdU-positive cell rates were calculated.
Colony formation assay
The well-grown cells at passage 3 were detached using 0.025% trypsin and incubated in 6-well plates (1000 cell/well) at 37°C with 5% CO2 for 2 weeks, after which they were treated with 75% methanol for 30 min, stained with 0.5% crystal violet for 15 min and finally observed under an optical microscope (IX51, Olympus Optical Co., Ltd, Tokyo, Japan) to analyze and calculate the number of cell colonies. Each experiment was performed for 3 times.
Flow cytometry
An apoptosis assay was conducted using an Annexin V-fluorescein isothiocyanate (FITC)/propidium iodide (PI) cell apoptosis kit (KeyGen, Nanjing, Jiangsu, China). Briefly, cells in each group were sorted onto 6-well plates at the density of 2 × 106 cells per well, after which they were stained with the mixture of 5 μL ALexa Flour 488 AnnexinV-FITC and 1 μL PI (100 mg/mL) and then incubated at room temperature in the dark for 15 min. Next, each well of cells were treated with 400 μL 1 × Annexin binding buffer and then the cell apoptosis was detected using a flow cytometer. Approximately 1 × 104 cells were included and the percentage of Annexin V+ (apoptotic) cells in each group was evaluated.
Terminal deoxynucleotidyl transferase (TdT)-mediated dUTP nick end labeling (TUNEL) staining
Cell suspension was sorted into confocal dishes (Thermo Fisher Scientific Inc., Waltham, MA, USA), which was further cultured in a 37°C incubator with 50 mL/L CO2. When the cell confluence got to 80%, the cells were subjected to TUNEL staining as per the kit instructions (R&D Systems Inc., Minneapolis, MN, USA). Followed by 4ʹ, 6-diamidino-2-phenylindole (DAPI) (10 μg/mL) staining for 10 min, the cell apoptosis was observed under a confocal microscope (Radiance 2100, Bio-Rad Laboratories Inc., Hercules, CA, USA).
Transwell assay
Cells were seeded into the Transwell chambers at the density of 1 × 105 cells/mL, and the apical chambers which coated with Matrigel were treated with serum-free media containing different doses of DEX (0 nmol/L, 1 nmol/L, 10 nmol/L and 100 nmol/L) while the basolateral chambers were treated with normal media. After 24 h of culture, cells invading to the basolateral chamber were stained using crystal violet and observed under a microscope.
Scratch test
Briefly, five parallel guide lines were produced at the back side of 12-well plates. SKOV3 and HO-8910 cells were plated into 12-well plates at the density of 1 × 105cells/mL. A 10 μL pipette tip was applied to produce scratches on the adherent cells perpendicular to the back guide lines, and then the suspension cells were rinsed with binding buffer. Next, the cells were cultured in serum-free media containing different doses of DEX (0 nmol/L, 1 nmol/L, 10 nmol/L and 100 nmol/L), and the cell growths at 0 h and 24 h were recorded for wound-healing rate calculation with the formula as follows: wound-healing rate (%) = (scratch width at 0 h – width at 24 h)/width at 0 h.
RT-qPCR
Total RNA of cells were extracted using Trizol one-step method (Invitrogen, Carlsbad, CA, USA) and the ultraviolet analysis and formaldehyde denaturing gel electrophoresis were applied to identify the high-quality RNA. After that, l μg RNA was collected and reverse-transcribed into cDNA by avian myeloblastosis virus reverse transcriptase. qPCR was conducted using a SYBR Green assay, and the PCR primers were designed and synthesized by Shanghai Sangon Biotech Co., Ltd (Shanghai, China) (), with U6 or β-actin was set as the internal reference. The PCR reaction system consisted of 1.0 μL cDNA, 10 μL 2× SYBR Green Mix, 0.5 μL Forward primer (10 μM), 0.5 μL reverse primer (10 μM) and RNase free water until the solution arrived at 20 μL. The PCR reaction condition was as follows: pre-denaturation at 94°C for 5 min, followed by 40 cycles of denaturation at 94°C for 40 s, annealing at 60°C for 40 s, extension at 72°C for 1 min, and a final extension at 72°C for 10 min. The production was identified using agarose gel electrophoresis. Data were analyzed using 2−ΔΔCt method in which 2−ΔΔCt refers to the ratio of the target gene expression between the experimental and control groups, the formula was as follows: ΔΔCt = [Ct (target gene) – Ct (internal control gene)] experimental group – [Ct (target gene) – Ct (internal control gene)] control group.
Table 1. Primer sequences for RT-qPCR
Western blot analysis
Proteins were extracted from cells and their concentrations were evaluated as per the instructions of a bicinchoninic acid assay kit (Wuhan Boster Biological Technology, Ltd., Hubei, China). Followed by blending with the loading buffer, the extracted proteins were boiled at 95°C for 10 min and then loaded into the wells with 30 µg per well. Next, the proteins were detached using 10% sodium dodecyl sulfate-polyacrylamide gel electrophoresis (Wuhan Boster Biological Technology, Ltd., Hubei, China) with the voltage from 80 V to 120 V and transferred to polyvinylidene difluoride membranes (100 mV for 45 ~ 70 min). Thereafter, the membranes were sealed with 5% bovine serum albumin at room temperature for 1 h and incubated with the following antibodies at 4°C overnight: cleaved caspase-3 (1 µg/mL, ab2302), Bax (1:1000, ab53154), Bcl-2 (1:1000, ab32124), SOX9 (1:1000, ab185230), β-catenin (1:2000, ab32572), C-myc (1:1000, ab32072) and cyclinD1 (1:200, ab16663). All these antibodies were purchased from Abcam Inc., (Cambridge, MA, USA). Followed by Tris-buffered saline with Tween 20 (TBST) washing (3 times/5 min), the protein were incubated with secondary antibodies (ZSGB-Bio, Beijing, China) for 1 h and washed with TBST for 3 times before chemiluminescence developing. A Gel Doc EZ Imager (Bio-Rad, CA, USA) was used to develop the protein bands. The gray value analysis for target bands was performed using Image J software (National Institutes of Health. Bethesda, Maryland, USA).
Dual luciferase reporter gene assay
An online miRNA target detection program was conducted to predict the binding sites of miR-185 and SOX9 (miRNA.org). The 3ʹ untranslated region (3ʹUTR) sequence of SOX9 which containing the miR-185 binding site was synthesized, and then a SOX9 3ʹUTR wild-type (WT) plasmid (SOX9-WT) and another SOX9 3ʹUTR mutant-type plasmid (SOX9-MUT) plasmid were constructed. Well-designed plasmids along with miR-185 mimic or mimic NC were co-transfected into 293 T cells. Cells were collected and lysed 48 h after transfection, and their luciferase activities were detected based on luciferase assay kit (BioVision, San Francisco, CA, USA) and Glomax20/20 illuminometer (Promega, Madison, Wisconsin, USA).
Immunofluorescence assay
Cells were sorted into 6-well plates which laid with 0.1 gelatin-coated cover glass until 80% ~ 90% of cell confluence was reached, the culture solution was discarded after which the cells were fixed with 4% polyformaldehyde for 20 min. Followed by 10% goat serum sealing for 1 h, the cells were incubated with primary rabbit polyclonal antibody β-catenin, fluorescence-labeled secondary antibody and stained with 4ʹ, 6-diamidino-2-phenylindole at room temperature for 30 min, after which the staining was observed under a confocal microscope.
Xenograft tumors in nude mice
BALB/c nude mice (male, 16–18 g, 4–6 weeks) purchased from Beijing Vital River Laboratory Animal Technology Co., Ltd., (Beijing, China) were fed in a specific pathogen-free grade animal center. After hypodermic injection of 100 μL (1 × 106) cells into the nude mice, the length (L) and width (W) of the tumors were recorded every 5 days, and the tumor volume (V) was calculated as follows: V (mm3) = L × W2 × 0.5, after which a tumor growth curve was produced. The mice were euthanized at day 30, and the subcutaneous tumors were extracted and weighted.
Immunohistochemistry
After weighted, the tumors were successively fixed with 4% polyformaldehyde for 24 h, dehydrated in series of alcohol, waxed twice, embedded in paraffin and cut into 4 μm sections. The paraffin-embedded sections were set on cover glasses coated with polylysine, baked for 2 h and then cooled at room temperature. Next, the sections were dewaxed in xylene and ethanol and added into 0.01 mol/L citrate buffer for 15 min of high pressure thermal treatment. After cooled at room temperature, the sections were treated with 3% H2O2 for 15 min to block the activity of endogenous peroxidase, which was followed by phosphate-buffered saline (PBS) washing for 15 min and incubated with primary antibodies at 4°C overnight. The second day, the sections were washed in PBS for 15 min and incubated with corresponding secondary antibody at 37°C for 40 min, which was followed by PBS washing for 15 min again. Then the sections were successively stained with 2, 4-diaminobutyric acid, rinsed with water for 15 min, counterstained with hematoxylin for 1 min, differentiated, turned to blue, washed, dehydrated and sealed. A NC was set with the primary antibodies were replaced as PBS, and the positive sections provided by the company were set as positive control, and the sections were observed under a microscope. Cells with positive ki67, cyclinD1 and p53 were showing with yellow or brown cytoplasm. Followed by comprehensive observations under low magnification, the integrated OD (IOD) values of cells were detected using an Image-propluse6.0 program (Media Cybernetics, Silver Spring, MD, USA) under a × 200 microscope magnification. A total of 5 views were randomly selected and the average IOD value was calculated.
Hematoxylin-eosin (HE) staining
The xenograft tumors were embedded with paraffin and sectioned, which was followed by HE staining and pathomorphologic characteristics observation under a microscope.
Statistical analysis
The Statistical Package for the Social Sciences (SPSS) 21.0 (IBM Corp. Armonk, NY, USA) was applied for data analysis. The normality test was conducted using the Kolmogorov-Smirnov method. Measurement data were showing as normal distribution and expressed as mean ± standard deviation. Differences between pairwise groups were evaluated using the t-test while the differences in multiple groups were compared using one-way analysis of variance (ANOVA). p was obtained using a two-sided test and p < 0.05 meant statistical significance.
Results
DEX inhibits proliferation and metastasis of OC cells
To evaluate the effect of DEX on the growth, invasion and metastasis of OC, the proliferation of OC cells were evaluated using MTT, EdU and colony formation assays, and the results suggested that the activity, EdU-positive rate and colony number of SKOV3 and HO-8910 cells were decreased with the increased doses of DX, showing a time- and dose-dependence ()-)). These results indicated that DEX could suppress the proliferation of OC cells.
Figure 1. DEX has a dose-dependent inhibitory effect on OC growth and invasion. A, cell viability detected using MTT assay; B, number of cell colonies detected using colony formation assay; C, cell proliferation measured using EdU assay; D, cell apoptosis detected using flow cytometry; E, cell apoptosis detected using TUNEL assay; F, expression of apoptosis-related proteins detected using Western blot analysis; G, cell invasion evaluated using Transwell assay; H, cell migration detected using scratch test; all experiments were performed for three times, and data in panels B/C/D/E/G/H were analyzed using one-way ANOVA, and data in panels A/F were analyzed using two-way ANOVA, followed by Tukey’s multiple comparisons test; *, p < 0.05; **, p < 0.01
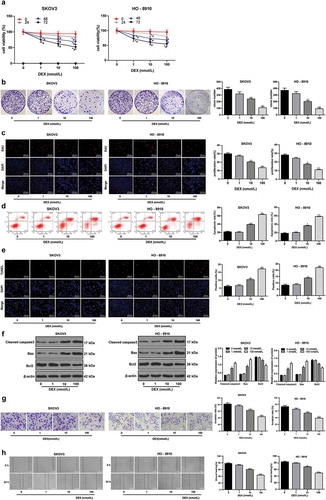
Moreover, the apoptotic rate of SKOV3 and HO-8910 cells was measured using flow cytometry, TUNEM staining and Western blot analysis. It was found that with the increase of DEX concentration, the apoptosis rate of SKOV3 and HO-8910 cells was increased significantly, the expression of apoptosis-related proteins cleaved caspase3 and Bax were enhanced while the expression of Bcl-2 was notably reduced (all p < 0.05) (-)). Besides, according to Transwell assay and scratch test, the invasion and migration abilities of SKOV3 and HO-8910 cells were significantly reduced after an ascending series of DEX treatment (-). The results above indicated that DEX has a dose-dependent inhibitory effect on OC cell proliferation and metastasis.
DEX inhibits OC cell proliferation and metastasis by upregulating miR-185 expression
The antioxidative, anti-inflammatory properties of DEX have been well established [Citation26]. Importantly, DEX can affect tumor development by regulating miRNA expression [Citation27]. Overexpression of miR-185 has been found to suppress proliferation and to promote apoptosis of OC cells [Citation18]. We speculate that DEX may affect the malignant behavior of OC cells by affecting the expression of miR-185. The results showed that miR-185 was increased with the concentration of DEX (all p < 0.05) (). In order to further study the mechanism of miR-185 in OC cells, we transfected anti-miR-185 or miR-185 mimic into 100 nmol/L DEX-treated OC cells HO-8910. RT-qPCR showed that transfection was successful (p < 0.05) (). After overexpression of miR-185, the activity and proliferation of HO-8910 cells treated with DEX were decreased (all p < 0.05) (), and the apoptosis rate was increased (all p < 0.05) (). Transwell and scratch test results showed that the invasion and migration ability of HO-8910 cells were significantly decreased (all p < 0.05) (-). However, after miR-185 knockdown, the activity and proliferation of HO-8910 cells treated with DEX were increased, the apoptosis rate decreased, and the invasion and migration ability of HO-8910 cells were significantly enhanced (all p < 0.05) (). These results suggest that DEX inhibits the proliferation and invasion of OC cells by upregulating miR-185.
Figure 2. DEX inhibits OC proliferation and invasion by upregulating miR-185 expression. miR-185 with low or over expression was transfected into HO-8910 cells treated with 100 nmol/L DEX. A, miR-185 expression detected using RT-qPCR; B, cell viability detected using MTT assay; C, number of cell colonies detected using colony formation assay; D, cell proliferation measured using EdU assay; E, cell apoptosis detected using flow cytometry; F, cell apoptosis detected using TUNEL assay; G, expression of apoptosis-related proteins detected using Western blot analysis; H, cell invasion evaluated using Transwell assay; I, cell migration detected using scratch test; all experiments were performed for three times, and data in panels A/B/C/D/E/F/H/I were analyzed using one-way ANOVA, and data in panel G were analyzed using two-way ANOVA, followed by Tukey’s multiple comparisons test; *, p < 0.05; **, p < 0.01
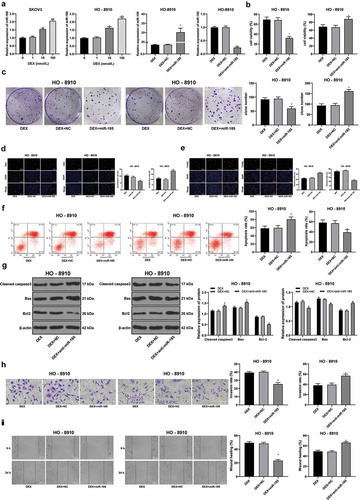
MiR-185 targets SOX9
The miRNA.org bioinformatics website predicted the downstream targets of miR-185. Among them, SOX9 is highly expressed in OC [Citation28]. According to the binding site of miR-185 and SOX9 3 ʹ UTR (), the fluorescence intensity of cells transfected with SOX9 (WT) and miR-185 was significantly decreased compared with NC group (p < 0.05). In addition, the mRNA and protein levels of SOX9 in SKOV3 and HO-8910 cells were decreased with the concentration of DEX (p < 0.05) (). Overexpression of miR-185 decreased the mRNA and protein levels of SOX9, while miR-185 knockdown increased the mRNA and protein levels of SOX9 (p < 0.05) (). In conclusion, miR-185 inhibits the expression of SOX9.
Figure 3. miR-185 directly binds to SOX9. A, target relation between miR-185 and SOX9 predicted via miRNA.org website; B, target relation between miR-185 and SOX9 identified using dual-luciferase reporter gene assay; C, SOX9 mRNA expression in OC cells detected using RT-qPCR; D, SOX9 protein expression in OC cells detected using Western blot analysis; the experiment was performed for 3 times; in panels C and D, data were analyzed using one-way ANOVA, and in panel B, data were analyzed using two-way ANOVA, followed by Tukey’s multiple comparisons test; *, p < 0.05; **, p < 0.01
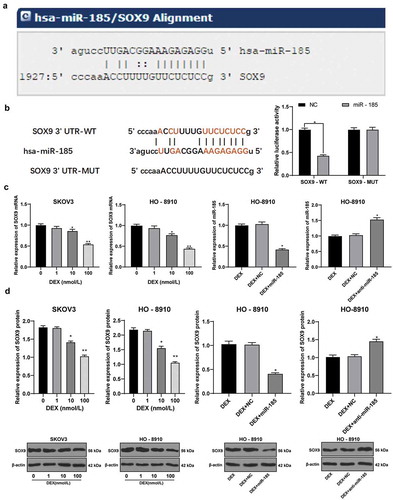
SOX9 knockdown inhibits OC cells proliferation and metastasis
To study the effect of SOX9 in OC cell growth, a rescue experiment was performed. SOX9 was downregulated in HO-8910 cells with anti-miR-185, and then cells were treated with 100 nmol/L DEX. It was shown that SOX9 knockdown led to significant declines in HO-8910 cell viability, number of cell colonies and EdU-positive cell rates (all p < 0.05) (-), and the cell apoptotic rate and cleaved caspase 3 and Bax expression were significantly elevated while the Bcl-2 expression was notably decreased (all p < 0.05) (). Besides, the invasion and migration of HO-8910 cells obviously reduced after SOX9 knockdown (all p < 0.05) (-). These results demonstrated that SOX9 knockdown could reverse the effect of miR-185 knockdown on OC cell proliferation and apoptosis.
Figure 4. SOX9 knockdown inhibits OC cells proliferation and metastasis. SOX9 siRNA was transfected into HO-8910 cells with anti-miR-185, and NC siRNA was used as control, and then cells were treated with 100 nmol/L DEX. A, cell viability detected using MTT assay; B, number of cell colonies detected using colony formation assay; C, DNA replication measured using EdU assay; D, cell apoptosis detected using flow cytometry; E, cell apoptosis rate detected using TUNEL assay; F, expression of apoptosis-related proteins detected using Western blot analysis; G, cell invasion evaluated using Transwell assay; H, cell migration detected using scratch test; all experiments were performed for 3 times and t test was used to analyze statistical significance in panels A/B/C/D/E/G/H, and two-way ANOVA and Tukey’s multiple comparisons test were used to analyze data in panel F. *, p < 0.05
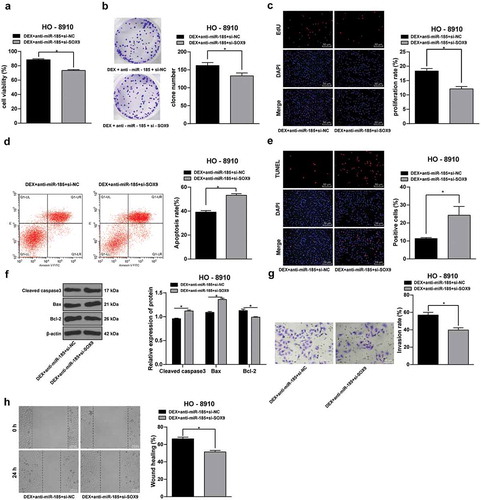
DEX inactivates the Wnt/β-catenin signaling pathway via the miR-185/SOX9 axis
Next, we continued to explore the downstream mechanism of SOX9. Wnt/β-catenin signaling pathway is inhibited in human non-small cell lung cancer cells lacking SOX9 [Citation29]. In addition, Wnt/β-catenin signaling pathway is involved in the development of OC [Citation30]. Therefore, we measured the protein levels of key factors in Wnt/β-catenin signaling pathway β-catenin, C-myc and cyclinD1 in OC cells. The results suggested that after DEX treatment, the protein levels of SOX9, β-catenin, C-myc and cyclinD1 were significantly reduced with the increase of DEX concentration (all p < 0.05) (). Overexpression of miR-185 resulted in decreased levels of β-catenin, C-myc and CyclinD1, while miR-185 knockdown promoted the levels of β-catenin, C-myc and CyclinD1, but this promotion was weakened after the expression of SOX9 was decreased (all p < 0.05) (). Moreover, the fluorescence intensity of β-catenin was consistent (all p < 0.05) (). These results suggest that DEX inhibits the activation of Wnt/β-catenin signaling pathway through the miR-185/SOX9 axis.
Figure 5. DEX inactivates the Wnt/β-catenin signaling pathway via the miR-185/SOX9 axis. A-B, protein expression of key factors in Wnt/β-catenin signaling pathway (β-catenin, C-myc and cyclinD1) detected using Western blot analysis; C, fluorescence intensity of β-catenin evaluated using immunofluorescence assay; the experiment was performed for 3 times and two-way ANOVA was used to determine statistical significance in panels A/B; *, p < 0.05; **, p < 0.01
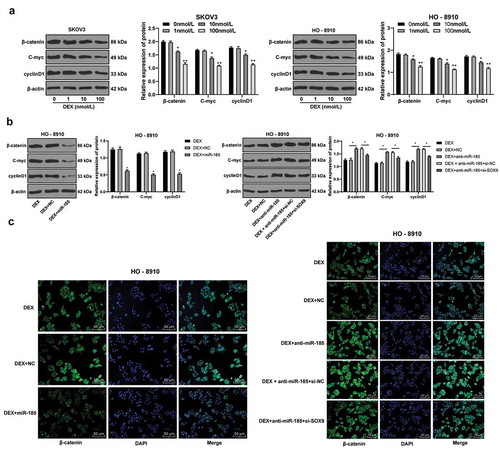
DEX suppresses OC tumor growth in vivo
Furthermore, a xenograft tumor model was established to explore the role of DEX and miR-185 in vivo. HO-8910 cells transfected with anti-miR-185 were subcutaneously injected into nude mice, and then the mice were treated with DEX. The changes of tumor volume and weight were measured. miR-185 knockdown significantly increased tumor volume and weight (all p < 0.05) (-). Moreover, the immunohistochemistry results of ki67, P53, CyclinD1, C-myc and β-catenin suggested that miR-185 knockdown significantly increased positive rates of ki67, P53, CyclinD1, C-myc and β-catenin (all p < 0.05) (). Besides, HE staining results suggested that the tumor tissues showed obvious necrosis after miR-185 knockdown (). In addition, after injection of the transfected HO-8910 cells subcutaneously into nude mice, the expression of miR-185 was decreased and the expression of SOX9 was increased (p < 0.05) (-). These results suggest that DEX inhibits the activation of Wnt/β-catenin signaling pathway through the miR-185/SOX9 axis, and then affects the growth of OC.
Figure 6. DEX suppresses OC tumor growth in vivo. HO-8910 cells with low or over expression of miR-185 were subcutaneously injected into nude mice, and then treated with 100 nmol/L DEX. A, tumor volume detection after OC cell transfection; B, tumor weight detection after OC cell transfection; C, ki67, cyclinD1 and p53 expression detected using immunohistochemistry; D, morphologies of tumor tissues observed using HE staining; E: the expression of miR-185 and SOC9 was detected by RT-qPCR; F, SOX9 protein level detected by Western blot. N = 6. In panels B and E, one-way ANOVA was used to determine statistical significance; in panels A and C, two-way ANOVA was used to determine statistical significance, followed by Tukey’s multiple comparisons test; *, p < 0.05
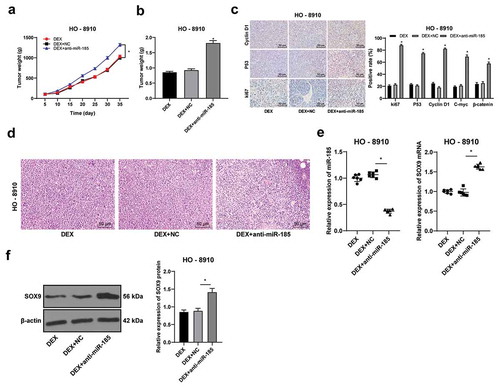
Discussion
OC is a life-threatening malignancy which has not seen a major therapeutic improvement over 3 decades [Citation31]. DEX is a widely used anesthetic and also has organ-protective [Citation32], neuroprotective [Citation33] and in some cases, has anticancer [Citation34] effects. Meanwhile, dysregulation of miRNAs has been well established involving in a number of diseases including cancers [Citation35]. The current study was designed to figure out the possible relationship between DEX and miR-185 and their effects on OC development, with a conclusion drawn that DEX could inhibit OC development via up-regulating miR-185 expression and inactivating the SOX9/Wnt/β-catenin signaling pathway.
Emerging studies have suggested that anesthetics may also have anti-tumor properties such as morphine [Citation36] and propofol [Citation37]. Likewise, DEX has been demonstrated to suppress cell proliferation and migration and to promote cell death in osteosarcoma cells [Citation34]. Initially, our study found that DEX showed a dose-dependent inhibitory effect on OC growth, invasion and migration. Besides, a previous study suggested that DEX treatment could inhibit OC growth and the tumor weight of OS rats in vivo [Citation38]. Recently, Lei Hou et al. found that DEX could improve the immune function of OC rats and inhibit the invasion and migration of OC cells via inactivating the insulin-like growth factor 2 signaling pathway [Citation39]. Meanwhile, in this present study, DEX also presented a dose-dependent promoting role in OC cell apoptosis, with increased expression of cleaved caspase 3 and Bax while declined expression of Bcl-2 shown after DEX treatment. Caspase 3 and Bax/Bcl-2 ratio are well-known proapoptotic factors [Citation40]. Bcl-2 is an antiapoptotic protein which regulates apoptosis via modulating the permeability of mitochondrial membrane, while Bax is capable of damaging the outer mitochondrial membrane [Citation41,Citation42]. Thus, DEX could inhibit proliferation, metastasis and resistance to apoptosis of OC cells.
DEX can affect tumor progression by affecting miRNA expression [Citation27]. Overexpression of miR-185 can inhibit OC cell proliferation and promote apoptosis [Citation18]. To further identify the molecular mechanism of DEX on OC development, we detected the miR-185 expression in OC cells after DEX treatment. Importantly, DEX elevated miR-185 expression in OC cells. In order to further study the mechanism of miR-185 in DEX-treated OC cells, we transfected low/over expression of miR-185 into 100 nmol/L DEX treated OC cells. After overexpression of miR-185, the activity and proliferation of HO-8910 cells treated with DEX were decreased, the apoptosis rate increased, and the invasion and migration ability were decreased significantly. Emerging studies have revealed that miR-185 works as a tumor suppressor. For instance, miR-185 could inhibit the proliferative potential of human colorectal cancer cells [Citation43]. JS Imam et al. suggested that miR-185 is lowly expressed in several human cancers including OC and it could impede tumor growth and progression [Citation44]. These results suggest that DEX inhibits the proliferation and invasion of OC cells by upregulating the expression of miR-185.
According to bioinformation prediction and dual-luciferase reporter gene assay, miR-185 was identified to negatively bind to 3ʹ-UTR of SOX9, which is coincident with the study published by Zhengwen Lei et al. [Citation19]. After DEX treatment, the mRNA and protein levels of SOX9 in SKOV3 and HO-8910 cells were decreased with the concentration of DEX. SOX9 is highly expressed in OC cells [Citation28]. To study the action of SOX9 in OC development, SOX9 was downregulated in HO-8910 cells with anti-miR-185, and then cells were treated with 100 nmol/L DEX. In our study, knockdown of SOX9 in OC cells inhibited cell growth and metastasis. SOX9 is a key transcription factor required for cancer development [Citation45]. Likewise, overexpression of SOX9 has been demonstrated to cause neoplasia in murine prostate and to promote tumor invasion [Citation46]. Furthermore, we explored the downstream pathway of SOX9. SOX9 activated the Wnt/β-catenin pathway in endometrial carcinoma cells [Citation47]. Previous study has shown that in OC, Wnt/β-catenin pathway activation contributes to this immune phenotype [Citation48]. Our study found that DEX treatment or overexpression of miR-185 reduced the protein expression of β-catenin, C-myc and cyclin D1 in OC cells. C-myc and cyclinD1 are two major downstream target of Wnt/β-catenin signaling pathway that are normally over-expressed in several cancer types [Citation49,Citation50]. Similarly, miR-185 has been suggested to negatively target SOX9 thus leading to Wnt signaling inactivation and inhibition on NSCLC development [Citation19]. Besides, in addition to the inhibitory effect of DEX and miR-185 on OC cell growth in vitro, the in vivo studies presented a same trend. These results suggested that DEX treatment and overexpression of miR-185 could inhibit OC development with the involvement of SOX9 and the Wnt/β-catenin signaling pathway.
To sum up, the current study provided new insights that DEX could up-regulate miR-185 expression, which further inhibits SOX9 expression and then inactivates the Wnt/β-catenin signaling pathway, thus suppressing OC growth and development. Meanwhile, this finding provides evidence that DEX might serve as an anti-cancer agent more than just an anesthetic during cancer therapies. Hopefully, more studies would be applied in this field to validate our findings and to develop more novel therapies in treating OC.
Availability of data and materials
All the data generated or analyzed during this study are included in this published article.
Author contributions
HT is the guarantor of integrity of the entire study and contributed to the concepts and design of this study; LH contributed to the definition of intellectual content and literature research of this study; YMX contributed to the experimental studies and clinical studies; QJC contributed to the data analysis and statistical analysis; HT took charge of the manuscript preparation; LH and YMX contributed to the manuscript review. All authors read and approved the final manuscript.
Acknowledgments
Not applicable.
Disclosure statement
All authors declare that there is no conflict of interests in this study.
References
- Hua F, Li C-H, Chen X-G, et al. Daidzein exerts anticancer activity towards SKOV3 human ovarian cancer cells by inducing apoptosis and cell cycle arrest, and inhibiting the Raf/MEK/ERK cascade. Int J Mol Med. 2018;41(6):3485–3492.
- Bareiss PM, Paczulla A, Wang H, et al. SOX2 expression associates with stem cell state in human ovarian carcinoma. Cancer Res. 2013;73:5544–5555.
- Nikpayam E, Tasharrofi B, Sarrafzadeh S, et al. The role of long non-coding RNAs in ovarian cancer. Iran Biomed J. 2017;21(1):3–15.
- Ozga M, Aghajanian C, Myers-Virtue S, et al. A systematic review of ovarian cancer and fear of recurrence. Palliat Support Care. 2015;13:1771–1780.
- Gao Y, Liu X, Li T, et al. Cross-validation of genes potentially associated with overall survival and drug resistance in ovarian cancer. Oncol Rep. 2017;37:3084–3092.
- Varughese J, Cocco E, Bellone S, et al. High-grade, chemotherapy-resistant primary ovarian carcinoma cell lines overexpress human trophoblast cell-surface marker (Trop-2) and are highly sensitive to immunotherapy with hRS7, a humanized monoclonal anti-Trop-2 antibody. Gynecol Oncol. 2011;122:171–177.
- Weerink MAS, Struys M, Hannivoort LN, et al. Clinical pharmacokinetics and pharmacodynamics of dexmedetomidine. Clin Pharmacokinet. 2011;408(8):893–913.
- Mohta M, Kalra B, Sethi AK, et al. Efficacy of dexmedetomidine as an adjuvant in paravertebral block in breast cancer surgery. J Anesth. 2016;30:252–260.
- Roberts SB, Wozencraft CP, Coyne PJ, et al. Dexmedetomidine as an adjuvant analgesic for intractable cancer pain. J Palliat Med. 2011;14:371–373.
- Endesfelder S, Makki H, Von Haefen C, et al. Neuroprotective effects of dexmedetomidine against hyperoxia-induced injury in the developing rat brain. PLoS One. 2017;12(2):e0171498.
- Wang W-X, Wu Q, Liang -S-S, et al. Dexmedetomidine promotes the recovery of neurogenesis in aged mouse with postoperative cognitive dysfunction. Neurosci Lett. 2018;677:110–116.
- Zhang Y, Jia S, Gao T, et al. Dexmedetomidine mitigate acute lung injury by inhibiting IL-17-induced inflammatory reaction. Immunobiology. 2018;223(1):32–37.
- Ji J, Shi J, Budhu A, et al. MicroRNA expression, survival, and response to interferon in liver cancer. N Engl J Med. 2009;361(15):1437–1447.
- Palma E, Gonzalez V, Grunholz D, et al. Tormenta de citoquinas: reacción adversa inhabitual por rituximab. Caso clínico. Rev Med Chil. 2017;145(2):260–263.
- Xie L, Zhang Z, Tan Z, et al. MicroRNA-124 inhibits proliferation and induces apoptosis by directly repressing EZH2 in gastric cancer. Mol Cell Biochem. 2014;392(1–2):153–159.
- Liu C, Li G, Ren S, et al. miR-185-3p regulates the invasion and metastasis of nasopharyngeal carcinoma by targeting WNT2B in vitro. Oncol Lett. 2017;13(4):2631–2636.
- Li S, Ma Y, Hou X, et al. MiR-185 acts as a tumor suppressor by targeting AKT1 in non-small cell lung cancer cells. Int J Clin Exp Pathol. 2015;8:11854–11862.
- Xiang Y, Ma N, Wang D, et al. MiR-152 and miR-185 co-contribute to ovarian cancer cells cisplatin sensitivity by targeting DNMT1 directly: a novel epigenetic therapy independent of decitabine. Oncogene. 2014;33:378–386.
- Lei Z, Shi H, Li W, et al. miR185 inhibits nonsmall cell lung cancer cell proliferation and invasion through targeting of SOX9 and regulation of Wnt signaling. Mol Med Rep. 2018;17:1742–1752.
- Lu B, Fang Y, Xu J, et al. Analysis of SOX9 expression in colorectal cancer. Am J Clin Pathol. 2008;130:897–904.
- Thomsen MK, Ambroisine L, Wynn S, et al. SOX9 elevation in the prostate promotes proliferation and cooperates with PTEN loss to drive tumor formation. Cancer Res. 2010;70(3):979–987.
- Raspaglio G, Petrillo M, Martinelli E, et al. Sox9 and Hif-2alpha regulate TUBB3 gene expression and affect ovarian cancer aggressiveness. Gene. 2014;542:173–181.
- Ma F, Ye H, He HH, et al. SOX9 drives WNT pathway activation in prostate cancer. J Clin Invest. 2016;126:1745–1758.
- Li F, Zhang L, Li W, et al. Circular RNA ITCH has inhibitory effect on ESCC by suppressing the Wnt/beta-catenin pathway. Oncotarget. 2015;6:6001–6013.
- Ruan X, Liu A, Zhong M, et al. Silencing LGR6 attenuates stemness and chemoresistance via inhibiting Wnt/beta-Catenin signaling in ovarian cancer. Mol Ther Oncolytics. 2019;14:94–106.
- Wang X, Zhao B, Li X. Dexmedetomidine attenuates isoflurane-induced cognitive impairment through antioxidant, anti-inflammatory and anti-apoptosis in aging rat. Int J Clin Exp Med. 2015;8:17281–17288.
- Abukiwan A, Nwaeburu CC, Bauer N, et al. Dexamethasone-induced inhibition of miR-132 via methylation promotes TGF-beta-driven progression of pancreatic cancer. Int J Oncol. 2019;54(1):53–64.
- Xiao S, Li Y, Pan Q, et al. MiR-34c/SOX9 axis regulates the chemoresistance of ovarian cancer cell to cisplatin-based chemotherapy. J Cell Biochem. 2019;120(3):2940–2953.
- Guo YZ, Xie XL, Fu J, et al. SOX9 regulated proliferation and apoptosis of human lung carcinoma cells by the Wnt/beta-catenin signaling pathway. Eur Rev Med Pharmacol Sci. 2018;22(15):4898–4907.
- Liu S, Liu Y, Lu Q, et al. The lncRNA TUG1 promotes epithelial ovarian cancer cell proliferation and invasion via the WNT/beta-catenin pathway. Onco Targets Ther. 2018;11:6845–6851.
- Basuli D, Tesfay L, Deng Z, et al. Iron addiction: a novel therapeutic target in ovarian cancer. Oncogene. 2017;36:4089–4099.
- Heimisdottir M. [Unlocking the full potential of Landspitali University Hospital - Icelandic healthcare at a crossroads. An outsiders view from McKinsey and Company[Editorial]]. Laeknabladid. 2016;102:425.
- Shanbehzadeh S, Salavati M, Talebian S, et al. Attention demands of postural control in non-specific chronic low back pain subjects with low and high pain-related anxiety. Exp Brain Res. 2018;236:1927–1938.
- Wang X, Xu Y, Chen X, et al. Dexmedetomidine inhibits osteosarcoma cell proliferation and migration, and promotes apoptosis by regulating miR-520a-3p. Oncol Res. 2018;26:495–502.
- Palanichamy JK, Rao DS. miRNA dysregulation in cancer: towards a mechanistic understanding. Front Genet. 2014;5:54.
- Yam C, He Y, Zhang D, et al. Divergent strategies for controlling the nuclear membrane satisfy geometric constraints during nuclear division. Curr Biol. 2011;21:1314–1319.
- Xu YB, Du QH, Zhang MY, et al. Propofol suppresses proliferation, invasion and angiogenesis by down-regulating ERK-VEGF/MMP-9 signaling in Eca-109 esophageal squamous cell carcinoma cells. Eur Rev Med Pharmacol Sci. 2013;17:2486–2494.
- Cai QH, Tang Y, Fan SH, et al. In vivo effects of dexmedetomidine on immune function and tumor growth in rats with ovarian cancer through inhibiting the p38MAPK/NF-kappaB signaling pathway. Biomed Pharmacother. 2017;95:1830–1837.
- Tian H, Hou L, Xiong Y, et al. Effect of dexmedetomidine-mediated Insulin-Like Growth Factor 2 (IGF2) signal pathway on immune function and invasion and migration of cancer cells in rats with ovarian cancer. Med Sci Monit. 2019;14:94–106.
- Freitas M, Alves V, Sarmento-Ribeiro AB, et al. Combined effect of sodium selenite and docetaxel on PC3 metastatic prostate cancer cell line. Biochem Biophys Res Commun. 2011;408:713–719.
- Lv J, Liang Y, Tu Y, et al. Hypoxic preconditioning reduces propofol-induced neuroapoptosis via regulation of Bcl-2 and Bax and downregulation of activated caspase-3 in the hippocampus of neonatal rats. Neurol Res. 2018;40:767–773.
- Peng X, Chen K, Chen J, et al. Aflatoxin B1 affects apoptosis and expression of Bax, Bcl-2, and Caspase-3 in thymus and bursa of fabricius in broiler chickens. Environ Toxicol. 2018;17(9):1742–1752.
- Liu M, Lang N, Chen X, et al. miR-185 targets RhoA and Cdc42 expression and inhibits the proliferation potential of human colorectal cells. Cancer Lett. 2011;301(2):151–160.
- Imam JS, Buddavarapu K, Lee-Chang JS, et al. MicroRNA-185 suppresses tumor growth and progression by targeting the Six1 oncogene in human cancers. Oncogene. 2010;29:4971–4979.
- Jiang SS, Fang WT, Hou YH, et al. Upregulation of SOX9 in lung adenocarcinoma and its involvement in the regulation of cell growth and tumorigenicity. Clin Cancer Res. 2010;16:4363–4373.
- Cai C, Wang H, He HH, et al. ERG induces androgen receptor-mediated regulation of SOX9 in prostate cancer. J Clin Invest. 2013;123:1109–1122.
- Li Y, Liu J, Piao J, et al. Circ_0109046 promotes the malignancy of endometrial carcinoma cells through the microRNA-105/SOX9/Wnt/beta-catenin axis. IUBMB Life. 2020;73(1):159–176.
- Wall JA, Meza-Perez S, Scalise CB, et al. Manipulating the Wnt/beta-catenin signaling pathway to promote anti-tumor immune infiltration into the TME tosensitize ovarian cancer to ICB therapy. Gynecol Oncol. 2021;160(1):285–294.
- Jin X-T, Song L, Zhao J-Y, et al. Dichlorodiphenyltrichloroethane exposure induces the growth of hepatocellular carcinoma via Wnt/β-catenin pathway. Toxicol Lett. 2014;225(1):158–166.
- Koehler A, Schlupf J, Schneider M, et al. Loss of Xenopus cadherin-11 leads to increased Wnt/β-catenin signaling and up-regulation of target genes c-myc and cyclin D1 in neural crest. Dev Biol. 2018;26(1):495–502.