ABSTRACT
Coronary atherosclerosis (CAS) is a major cause of cardiovascular disease. Long non-coding RNAs (lncRNAs) have been implicated as novel biomarkers in coronary artery disease (CAD). APOA1 antisense RNA (APOA1-AS) was proven to show high expression during atherosclerotic development, but no report has uncovered the detailed mechanism of APOA1-AS in CAS. Thus, this paper aims to explore the role of APOA1-AS in CAS. Vascular smooth muscle cells (VSMCs) were treated with oxidized low-density lipoprotein (ox-LDL) to mimic atherosclerosis-like injury. Quantitative real-time PCR (RT-qPCR) and western blot analysis analyzed gene expression. Cell counting kit-8 (CCK-8), wound healing assay, and flow cytometry were implemented to assess the function of APOA1-AS in modulating pathological phenotype of VSMCs. Results demonstrated that APOA1-AS was notably up-regulated in ox-LDL treated VSMCs (ox-LDL-VSMCs). The deficiency of APOA1-AS hindered proliferation and migration and stimulated apoptosis in ox-LDL-VSMCs. Mechanistically, APOA1-AS recruited TATA-box binding protein associated factor 15 (TAF15) protein to stabilized SMAD family member 3 (SMAD3) mRNA and activate the TGF-β/SMAD3 signaling pathway. In conclusion, APOA1-AS contributed to proliferation and migration and repressed apoptosis of VSMCs through TAF15-mediated SMAD3 mRNA stabilization, indicating that APOA1-AS could be a promising target for CAS.
Introduction
CAS is considered to be a major global health issue for its occurrence is increasing around the world [Citation1], and it caused 45% of total 18 million deaths related to cardiovascular diseases worldwide every year [Citation2]. However, CAS is difficult to be diagnosed and cured due to its heterogeneity and unpredictability [Citation3]. The formation of atherosclerotic lesion involves the phenotype switch of several types of cells including macrophages, endothelial cells (ECs), and smooth muscle cells (SMCs) [Citation4]. Notably, dysregulated proliferation, migration, and apoptosis in VSMCs are proved to contribute to CAS development [Citation5,Citation6]. As a key factor for CAS progression, ox-LDL has been proven to regulate VSMCs apoptosis, proliferation, and migration [Citation7]. Therefore, a further understanding of mechanisms relating to the phenotype switch of VSMCs is helpful for optimizing CAS treatment.
It is implicated that lncRNAs participate in the processes of heart diseases through transcriptional events and post-transcriptional events [Citation8]. For instance, lncCIRBIL relieves cardiac ischemia-reperfusion injury by modulating Bclaf1 nuclear translocation [Citation9]. CHAIR protects heart against pathological stress [Citation10]. It is also substantially reported that lncRNAs function as active participators in CAS [Citation11]. For example, Quan et al. have determined that lncRNA PVT1 is up-regulated in CAS and influences the progression of CAS [Citation12]. Zhu et al. have validated that lncRNA SNHG14 plays the promoting role in CAS by targeting miR-19a-3p/RORα axis [Citation13]. LncRNA APOA1-AS has been implicated in remitting multiple sclerosis [Citation14]. Additionally, it has been reported that APOA1-AS displays overtly high expression in CAS tissues, indicating it might be a potential biomarker for the diagnosis of CAS [Citation15,Citation16]. However, the detailed functionality of APOA1-AS on CAS and underlying regulatory mechanism remain to be elucidated.
TAF15 in FET (FUS/EWSR1/TAF15) family is responsible for transcription, splicing, and transportation of RNAs [Citation17–19]. As an RNA binding protein (RBP), TAF15 is also widely reported to regulate mRNA stability. For instance, TAF15 is reported to stabilize LINC00665 in glioma cells [Citation20], and it also stabilized high-mobility group box 3 (HMGB3) mRNA in lung cancer [Citation21]. TAF15 was predicted to bind with APOA1-AS in bioinformatics website starBase, but their relationship with CAS has never been confirmed.
SMAD Family Member 3 (SMAD3) is a principal effector in TGF-β signaling pathway [Citation22]. TGF-β/SMAD3 have been revealed to influence CAS pathology according to several works. For instance, TGF-β/SMAD3 facilitates VSMCs proliferation and differentiation [Citation23,Citation24]. TGF-β/SMAD3 alleviated apoptosis and inflammatory response in vascular endothelial cells [Citation25]. However, TGF-β/SMAD3 has never been related to APOA1-AS in VSMCs before.
Thus, this study established atherosclerosis injury model in ox-LDL-treated VSMCs and aimed to reveal the influence of APOA1-AS on the behaviors of ox-LDL-VSMCs and explore the underlying mechanism. This study might provide APOA1-AS as a potential marker for treatment targeting VSMCs in CAS.
Materials and methods
Cell culture
Human embryonic kidney (HEK)-293 T cells and VSMCs were purchased from American Type Culture Collection (ATCC; Manassas, VA, USA). HEK-293 T cells were maintained in Dulbecco’s Modified Eagle’s Medium (DMEM; A4192101, Gibco, Rockville, MD, USA) and VSMCs were incubated in F-12 K Medium (21,127,030, Gibco). The mediums were contained with 10% fetal bovine serum (FBS; 10,082,147, Gibco) in 5% CO2 at 37°C. To mimic atherosclerosis-like injury, VSMCs were treated with oxidized low-density lipoprotein (ox-LDL) (60 μg/mL) for 24 h and then termed as ox-LDL-VSMCs.
Plasmid transfection
Genechem (Shanghai, China) synthesized the short hairpin RNA targeting APOA1-AS or TAF15, as well as respective control shRNAs. Besides, pcDNA 3.1 vector was subcloned with SMAD3 for overexpression of SMAD3, and empty pcDNA3.1 vector was regarded as the negative control. Lipofectamine 3000 (L3000075, Invitrogen, Carlsbad, CA, USA) was used for cell transfection.
Quantitative real-time PCR (RT-qPCR) analysis
Firstly, total RNA was obtained with the utilization of TRIzol Reagent (15,596,026, Introgen, Carlsbad, CA, USA). Then, RNAs were reversely transcribed into cDNA with the application of RevertAid First Strand cDNA Synthesis Kit (K1621, Thermo fisher, IL, USA). SYBR Green PCR Master Mix (4,309,155, Applied Biosystems, Foster City, CA, USA) was used to quantify RNA levels. Expressions of all target genes referred to GAPDH or U6 as the internal control were calculated by 2−ΔΔCt method. The experiment was independently conducted in triplicate.
Cell counting kit-8 (CCK-8) assay
At first, VSMCs were incubated into 96-well plates (5 × 103 cells/well). After that, CCK-8 solution (CK04, Dojindo, Kumamoto, Japan) was added. Finally, cell proliferation was observed with a spectrophotometer (912A0884, Thermo Fisher Scientific) after an additional incubation for 2 h. The experiments were independently conducted in triplicate.
Wound healing assay
A total of 3 × 103 cells were seeded into 6-well plates and cultured in a serum-free medium for 24 h at 37°C. When cells reached 80% confluence, a pipette tip was used to make a straight scratch wound. The cells were then cultured for another 24 h, and scratches were monitored and photographed at 0 and 24 h. The experiment was independently conducted in triplicate.
Flow cytometry analysis
After being transfected for 48 h, VSMCs were collected. Afterward, cells were washed and fixed using 70% precooling ethanol at 4°C for 12 h. Then, the cells were subjected to centrifugation. After being washed with phosphate buffered saline, cells were added with 100 μl propidium iodide (PI) dye (556,463, BD Biosciences, Franklin Lakes, NJ, USA) and 100 μl RNA enzymes (9001–99-4, Sigma-Aldrich, St. Louis, MO, USA), and went through incubation at 37°C for 30 min before slow and full precipitation. The flow cytometer (92821250S, Beckman Coulter, Kraemer Boulevard Brea, CA, USA) was used to observe cell apoptosis. The experiment was independently conducted in triplicate.
Fluorescent in situ hybridization (FISH) and immunofluorescence (IF)
The FISH probe targeting APOA1-AS was synthesized by Ribobio. After being fixed with 4% paraformaldehyde (PFA; E672002, Sangon Biotech, Shanghai, China) for 15 min at 37°C and then permeabilized with 0.5% Triton X-100 (E672002, Sangon Biotech, Shanghai, China), VSMCs were hybridized with APOA1-AS probe in buffer, followed the dye with 4ʹ,6-diamidino-2-phenylindole (DAPI; D9542, Sigma-Aldrich) solution.
For IF analysis, TAF15 primary antibody (1/10,000, ab134916, Abcam, Cambridge, MA, USA) was used to blot overnight at 4°C and then blots were incubated with DyLight 488, the FITC-conjugated secondary antibody (E032210, EarthOx, San Francisco, USA). With a confocal laser microscope (Axio-Imager_LSM-800, Zeiss, Oberkochen, Germany), images were obtained. The experiment was independently conducted in triplicate.
RNA immunoprecipitation (RIP)
RIP assay was conducted with the use of Z-Magna RIPTM RNA-binding Protein Immunoprecipitation kit (17–701, Sigma-Aldrich). Cell lysates were cultured with antibodies targeting Argonaute 2 (Ago2; 1/50, ab186733, Abcam), TAF15 (1/1000, #28,409, Cell Signaling Technology), and immunoglobulin G (IgG; 1/50, ab172730, Abcam) conjugated with magnetic beads. Finally, the immunoprecipitated RNAs were extracted for RT-qPCR analysis. The experimental procedure was independently carried out in triplicate.
Western blot analysis
Separated protein samples were transferred to polyvinylidene fluoride (PVDF) membranes (IPVH00010, Millipore, Bedford, MA, USA). Subsequently, the membranes went through incubation with the specific primary antibodies including B-cell lymphoma 2 antibody (anti-BCL2; 1/1000, ab32124, Abcam), BCL-2 associated X antibody (anti-Bax; 1/1000, ab32503, Abcam), anti-Cleaved caspase-3 (1/500, ab32042 Abcam), anti-Total caspase-3 (1/5000, ab32351, Abcam), anti-TAF15, upstream frameshift 1 antibody (anti-UPF1; 1/10,000, ab109363, Abcam), anti-p-SMAD3 (1/2000, ab52903, Abcam), anti-SMAD3 (1/1000, ab40854, Abcam), transforming growth factor beta 1 antibody (anti-TGF-β1; 1/1000, ab215715, Abcam), TGF-beta receptor 1 antibody (anti-TGFβ-R1; 1/1000, ab31013, Abcam) and glyceraldehyde-3-phosphate dehydrogenase antibody (anti-GAPDH; 1/2000, ab9485, Abcam) after being blocked with skimmed milk. Afterward, the blots were incubated with secondary antibody. At last, Chemiluminescence system (32,134, Pierce Biotechnology, Rockford, IL, USA) was applied to quantify proteins. The experiment was independently conducted in triplicate.
Luciferase reporter assay
With the aim to screen out the appropriate signaling pathway involved in APOA1-AS modulation mechanism, Cignal Finder Reporter Array (336,841, QIAGEN, Dusseldorf, Germany) was applied for detecting the luciferase activity in the several common signaling pathways in HEK-293 T cells with APOA1-AS depletion, which were seeded on 96-well plates.
Statistical analysis
Experimental data were analyzed by SPSS 22.0 statistical software package (IBM, Armonk, NY, USA). All data were expressed as mean ± standard deviation (SD). The differences between two groups or more groups were analyzed with the employment of Student’s t-test or analysis of variance (ANOVA) with Tukey or Dunnett as the post-hoc analysis. All the experiments were independently performed in triplicate. Differences were considered statistically significant when P < 0.05.
Results
LncRNA APOA1-AS is up-regulated in ox-LDL-VSMCs
First, the atherosclerosis-like injury was mimicked by treating VSMCs with ox-LDL (60 μg/mL). As demonstrated in Supplementary Figure 1a, the proliferation of VSMCs with ox-LDL treatment was observably stimulated 48 h after treatment. Meanwhile, the apoptotic rate of VSMCs declined under the treatment of ox-LDL (Supplementary Figure 1b). Accordingly, western blot was implemented to analyze the protein levels of apoptosis-related factors. The data suggested that the protein level of BCL2 was increased while the protein levels of Bax and Cleaved caspase-3 were decreased in the ox-LDL group compared with the control group (Supplementary Figure 1c). All these findings confirmed the successful establishment of atherosclerosis-like injury in VMSCs. Subsequently, through RT-qPCR analysis, we noticed that the expression of APOA1-AS was relatively high in VSMCs treated with ox-LDL (). Subcellular fractionation results displayed that APOA1-AS was expressed mainly in cytoplasm of VSMCs, and the distribution of APOA1-AS was not changed by ox-LDL treatment (). FISH images also confirmed the distribution of cytoplasmic APOA1-AS fluorescence in VSMCs, and the fluorescence was intensified by ox-LDL treatment in VSMCs (). In a word, APOA1-AS is markedly overexpressed in ox-LDL-induced VSMCs.
APOA1-AS interference mitigates the proliferation and migration but induces the apoptosis of ox-LDL-VSMCs
Next, we detected whether APOA1-AS mediated the influence of ox-LDL on VSMCs. First, we confirmed that APOA1-AS expression was lessened in ox-LDL-VSMCs by sh-APOA1-AS#1/2/3 transfection (). Since sh-APOA1-AS#1/2 led to more significant knockdown of APOA1-AS in VSMCs, the 2 shRNAs were applied for further experiments. Based on the data of CCK-8, the viability of ox-LDL-treated VSMCs was reduced by APOA1-AS knockdown (). Wound healing assay depicted that depletion of APOA1-AS attenuated the migratory capacity of ox-LDL-induced VSMCs (). Additionally, flow cytometry analysis demonstrated that inhibition of APOA1-AS increased apoptosis rate of ox-LDL-stimulated VMSCs (). Accordingly, data from western blot analysis presented that APOA1-AS knockdown led to the decrease in BCL2 level and increase in Bax and cleaved caspase-3 levels in ox-LDL-treated VSMCs (). To be concluded, APOA1-AS knockdown reversed the effect of ox-LDL on promoting proliferation and migration and inhibiting apoptosis in VMSCs.
Figure 2. APOA1-AS interference mitigates the proliferation and migration and induces the apoptosis of ox-LDL-VSMCs. (a) APOA1-AS level was measured by RT-qPCR in ox-LDL-VSMCs transfected with sh-APOA1-AS#1/2/3 versus sh-NC. (b) CCK-8 assay measured the viability of ox-LDL-VSMCs transfected with sh-APOA1-AS#1/2 versus sh-NC. (c) Pictures of scratch wound at 0 and 24 h of ox-LDL-VSMCs transfected with sh-APOA1-AS#1/2 or sh-NC were captured. The wound width at 24 h relative to 0 h was measured to assess migration of each group. (d) Flow cytometry analysis was to detect the apoptotic rate of ox-LDL-VSMCs transfected with sh-APOA1-AS#1/2/3 versus sh-NC. (e) Western blot was to analyze the protein levels of apoptosis-related factors (BCL2, Bax, cleaved caspase-3, and total caspase-3) in ox-LDL-VSMCs transfected with sh-APOA1-AS#1/2/3 versus sh-NC. ** P < 0.01
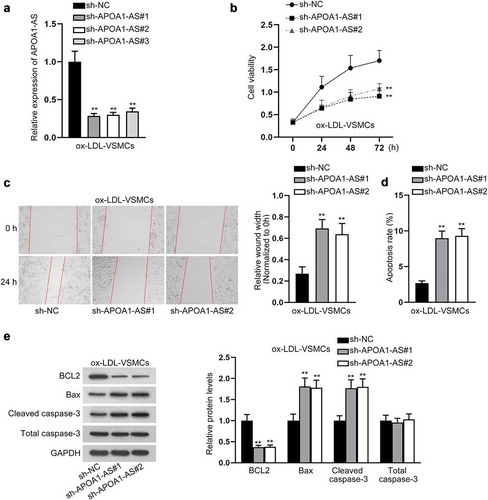
APOA1-AS recruits TAF15 protein in VSMCs
Later, the mechanism of APOA1-AS in VSMCs was investigated. The prominent expression of APOA1-AS in the cytoplasm of VSMCs indicated the regulatory mechanism of APOA1-AS post-transcriptional level. Hence, we speculated that APOA1-AS might be involved in the competing endogenous RNA (ceRNA) network or interact with certain RBP. RIP assay manifested that APOA1-AS was not enriched in binding complex with Ago2 protein in ox-LDL-treated VSMCs (). Thus, we proceeded to search for RBPs potentially bound with APOA1-AS. With the application of starBase (http://starbase.sysu.edu.cn/index.php), TAF15 and UPF1 were predicted under the indicated to bind with APOA1-AS (threshold: CLIP Data ≥ 3) (Supplementary Figure 1d). Further, RNA pull down assay confirmed that APOA1-AS had a strong affinity with TAF15 protein, instead of UPF1 (). The binding between APOA1-AS and TAF15 protein was also verified by RIP assay (). TAF15 belongs to FET family and can influence the transcription of mRNA and RNA splicing and transportation [Citation17–19]. Referring to previous publication, TAF15 has been reported to stabilize LINC00665 to in glioma and stabilize HMGB3 mRNA in lung cancer [Citation20,Citation21]. Thus, we speculated that TAF15 mediated the function of APOA1-AS in VSMCs. In the subsequent FISH assay and IF analysis, we observed APOA1-AS and TAF15 were both accumulated in the cytoplasm of VSMCs, and such accumulation was increased in ox-LDL-VSMCs (), indicating that they interacted in the cytoplasm of VSMCs. RT-qPCR and western blot analysis attested that depletion of APOA1-AS failed to alter the expression of TAF15 in ox-LDL-treated VSMCs (-f), indicating that APOA1-AS worked synergistically with TAF15 instead of affecting its expression in VSMCs. In summary, TAF15 is recruited by APOA1-AS.
Figure 3. APOA1-AS recruits TAF15 protein. (a) RIP assay and RT-qPCR examined the enrichment of APOA1-AS in binding complex with Ago2 protein. (b) RNA pull down assay and western blot detected the enrichment of TAF15 protein and UPF1 protein in the pulldown of Sense APOA1-AS and anti-sense APOA1-AS. (c) The interaction between APOA1-AS and TAF15 was verified by RIP assay. (d) Pictures of FISH fluorescence of APOA1-AS (green) and IF fluorescence of TAF15 protein (red) showed the location of APOA1-AS and TAF15 in VSMCs and ox-LDL-VSMCs (Scale bar: 10 μm). (e-f) TAF15 expression was tested by RT-qPCR and western blot analysis in ox-LDL-VSMCs transfected with sh-APOA1-AS#1/2/3 versus sh-NC. ** P < 0.01
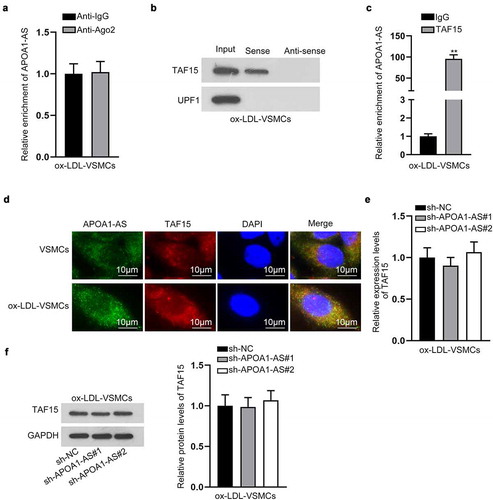
APOA1-AS activates TGF-β/SMAD3 signaling pathway by binding with TAF15
Then, we tried to figure out the functional pathway downstream of APOA1-AS/TAF15. Intriguingly, by detecting the luciferase activities of several common signaling pathways reported to be involved in CAS [Citation26–32], we found that the knockdown of APOA1-AS reduced the luciferase activity of the TGF-β/SMAD3 signaling pathway (). Accordingly, western blot analysis confirmed that the protein levels of p-SMAD3, SMAD3, TGF-β1 and TGFβ-R1 were all cut down when APOA1-AS was down-regulated in ox-LDL-treated VSMCs (), which indicated that APOA1-AS inhibition inactivated the TGF-β/SMAD3 signaling pathway. Especially, we found here that the total SMAD3 level could be affected by APOA1-AS. SMAD Family Member 3 (SMAD3) has been identified as a principal effector of TGF-β signals [Citation22], and, interestingly, it was predicted by starBase that SMAD3 was a downstream gene of TAF15 (Supplementary ). Besides, emerging evidence has validated that SMAD3 is associated with CAS [Citation33]. Hence, we speculated that SMAD3 was a downstream target for APOA1-AS/TAF15. Expectedly, RIP assay verified the interaction between TAF15 and SMAD3 mRNA (). To assess the influence of TAF15 on SMAD3 expression, TAF15 expression was lessened in ox-LDL-VSMCs by transfecting sh-TAF15#1/2 (-e). SMAD3 expression at mRNA level and protein level was also decreased due to silencing of TAF15 (-g). Eventually, after treatment with Actinomycin D (Act D), the stability of SMAD3 mRNA was also discovered to be weakened in response to TAF15 depletion (). Collectively, APOA1-AS activates TGF-β/SMAD3 signaling pathway through TAF-mediated SMAD3 mRNA stabilization.
Figure 4. APOA1-AS activates TGF-β/SMAD3 signaling pathway by binding with TAF15. (a) The luciferase activities of seven common signaling pathways were detected in HEK-293 T cells transfected with sh-APOA1-AS#1/2/3 versus sh-NC. (b) Western blot analyzed the protein levels of key factors in the TGF-β/SMAD3 signaling pathway in ox-LDL-VSMCs transfected with sh-APOA1-AS#1/2 versus sh-NC. (c) RIP assay examined the enrichment of SMAD3 in the precipitates for TAF15 antibody relative to that for IgG antibody. (d-e) TAF15 expression was measured by RT-qPCR and western blot in ox-LDL-VSMCs transfected with sh-TAF15#1/2 versus sh-NC. (f-g) The mRNA level and protein level of SMAD3 were measured by RT-qPCR and western blot in ox-LDL-VSMCs transfected with sh-TAF15#1/2 versus sh-NC. (h) The stability of SMAD3 mRNA was analyzed by RT-qPCR in ox-LDL-VSMCs transfected with sh-TAF15#1/2 versus sh-NC at 0, 2, 4, 6, and 8 h after Act D was added. ** P < 0.01
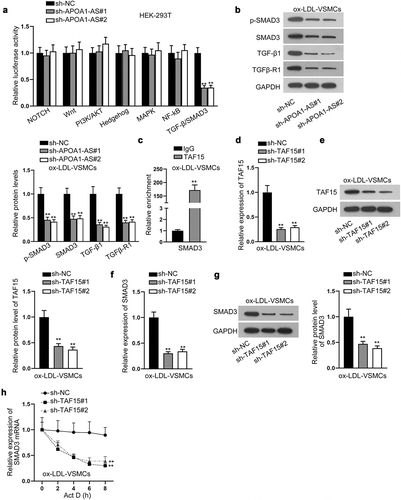
APOA1-AS positively regulates proliferation and migration of ox-LDL-VSMCs via modulating SMAD3 expression
To confirm the function of APOA1-AS/SMAD3 axis in modulating biological behaviors of ox-LDL-VSMCs, rescue experiments were carried out. First, SMAD3 expression was elevated in ox-LDL-VSMCs by the transfection of pcDNA3.1/SMAD3 (). The ox-LDL-VSMCs were transfected with sh-NC, sh-APOA1-AS#1 or sh-APOA1-AS#1+ pcDNA3.1/SMAD3 separately. The results of CCK-8 assay manifested that silencing of APOA1-AS impeded cell proliferation while this effect was completely restored by SMAD3 overexpression (). Likewise, wound healing assay also demonstrated that the migratory capacity of ox-LDL-VSMCs weakened by APOA1-AS deficiency was completely rescued by SMAD3 up-regulation (). Moreover, the elevated expression of SMAD3 completely reversed the cell apoptosis aggravated by APOA1-AS interference (-e). In conclusion, overexpression of SMAD3 rescues the influence of APOA1-AS deficiency on proliferation and migration of ox-LDL-VSMCs.
Figure 5. APOA1-AS contributes to proliferation and migration of ox-LDL-VSMCs via enhancing SMAD3 expression. (a) SMAD3 expression was measured in ox-LDL-VSMCs transfected with pcDNA3.1/SMAD3 versus pcDNA3.1. (b) Proliferation of ox-LDL-VSMCs was detected by CCK-8 with the transfection of sh-NC, sh-APOA1-AS#1 or sh-APOA1-AS#1+ pcDNA3.1/SMAD3. (c) Wound healing assay detected the migratory capacity of ox-LDL-VSMCs in the aforementioned groups. (d-e) Cell apoptosis was analyzed in ox-LDL-VSMCs of the aforementioned groups through flow cytometry and western blot analysis of BCL2, Bax, cleaved caspase-3, and total caspase-3. ** P < 0.01
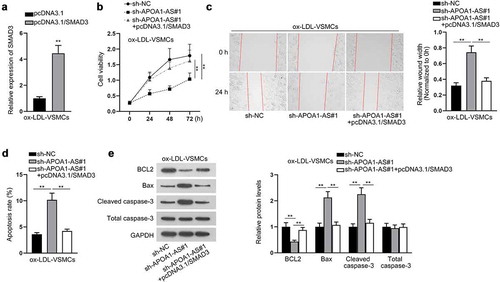
Discussion
Previously, APOA1-AS has been affirmed to play a pivotal role in atherosclerosis in Egyptian systemic lupus erythematosus patients [Citation16]. Furthermore, Zhang et al. have demonstrated that APOA1-AS is overexpressed in CAD and considered as a novel biomarker for CAD diagnosis [Citation15]. These findings suggested that APOA1-AS could be a key participant in CAS. As widely reported, CAS formation involves proliferation, migration, and apoptosis in VSMCs [Citation5,Citation6]. Several lncRNAs are suggested as participant in CAS for they can influence pathological phenotypes of VSMCs, such as SNHG7-003 [Citation34], RNCR3 [Citation35], and H19 [Citation36]. However, the role of APOA1-AS in CAS-related phenotypes of VSMCs was first explored by our work. First, we mimicked CAS injury in VSMCs by ox-LDL treatment, and confirmed that ox-LDL triggered proliferation and migration of VSMCs and attenuated apoptosis, which was concordant with previous report [Citation7]. Then, we showed that APOA1-AS was significantly up-regulated in ox-LDL-treated VSMCs, indicating that APOA1-AS was associated with CAS-related phenotype in VSMCs. Further, functional assays uncovered that down-regulation of APOA1-AS attenuated the proliferative and migratory capacities while strengthening the apoptotic ability of ox-LDL-VSMCs. These findings suggested that APOA1-AS affected ox-LDL-caused CAS-related phenotypes in VSMCs.
RBPs have great significance in gene regulation at post-transcriptional level [Citation37]. Through our investigation, we discovered that APOA1-AS was primarily located in the cytoplasm of VSMCs, indicating the potential function of APOA1-AS in post-transcriptional level regulation. It was confirmed that APOA1-AS could not function as a competing ceRNA based on the findings that APOA1-AS could not bind with Ago2. However, TAF15 was screened out the putative RBP for APOA1-AS. Formerly, TAF15 was reported to stabilize HMGB3 in lung squamous cell carcinoma cells [Citation21]. Herein, we first revealed that APOA1-AS interacted with TAF15 protein in the cytoplasm of ox-LDL-VSMCs. Additionally, APOA1-AS exerted no influence on TAF15 expression at mRNA level and protein level, indicating that APOA1-AS functioned synergistically with TAF15 instead of affecting its expression.
TGF-β/SMAD signaling pathway has been substantiated to regulate the growth and differentiation of various types of cells [Citation38]. As a key molecule for the regulation of TGF-β pathway, SMAD3 has also been widely reported in CAS. For instance, Chen et al. argued that SMAD3 can be used as a potential marker for coronary artery disease [Citation39]. TGF-β/SMAD3 are also proved to facilitate VSMCs proliferation and differentiation [Citation23,Citation24]. Herein, our study first discovered that APOA1-AS activated TGF-β/SMAD3 signaling pathway. Interestingly, we predicted and confirmed that TAF15 bound to SMAD3 mRNA in ox-LDL-VSMCs. Furthermore, TAF15 regulated SMAD3 expression and stabilized SMAD3 mRNA to activate TGF-β/SMAD3 signaling pathway in ox-LDL-VSMCs. Eventually, rescue experiments confirmed that up-regulation of SMAD3 completely counteracted the impacts of APOA1-AS knockdown on proliferation, migration, and apoptosis of ox-LDL-VSMCs, indicating that APOA1-AS regulated CAS-related phenotype in VSMCs via SMAD3 expression.
In conclusion, our study first illustrated that lncRNA APOA1-AS expression was elevated in ox-LDL-VSMCs. Depletion of APOA1-AS suppressed the proliferation, migration whereas inducing the apoptosis of VSMCs treated with ox-LDL. From the perspective of mechanism, APOA1-AS recruited TAF15 protein and stabilized SMAD3 mRNA to activate TGF-β/SMAD3 signaling pathway. As VSMC proliferation and migration contribute to the development of atherosclerosis, this study suggested that APOA1-AS had the potential to affect CAS progression, and that APOA1-AS could be a potential marker in CAS.
Supplemental Material
Download Zip (5.1 MB)Acknowledgments
We sincerely thank for the help provided by all lab personnel in this research.
Disclosure statement
No potential conflict of interest was reported by the author(s).
Supplementary material
Supplemental data for this article can be accessed here.
Data availability statement
Not applicable
Additional information
Funding
References
- Parsons C, Agasthi P, Mookadam F, et al. Reversal of coronary atherosclerosis: role of life style and medical management. Trends Cardiovasc Med. 2018;28(8):524–531.
- Aengevaeren VL, Mosterd A, Sharma S, et al. Exercise and coronary atherosclerosis: Observations, explanations, relevance, and clinical management. Circulation. 2020;141(16):1338–1350.
- Elffers TW, Jukema JW. [The (un)predictability of coronary atherosclerosis]. Ned Tijdschr Geneeskd. 2018;162:D2109.
- Lusis AJ. Atherosclerosis. Nature. 2000;407(6801):233–241.
- Wang P, Xu TY, Guan YF, et al. Vascular smooth muscle cell apoptosis is an early trigger for hypothyroid atherosclerosis. Cardiovasc Res. 2014;102(3):448–459. .
- Doran AC, Meller N, McNamara CA. Role of smooth muscle cells in the initiation and early progression of atherosclerosis. Arterioscler Thromb Vasc Biol. 2008;28(5):812–819.
- Pirillo A, Norata GD, Catapano AL. LOX-1, OxLDL, and atherosclerosis. Mediators Inflamm. 2013;2013:152786.
- Uchida S, Dimmeler S. Long noncoding RNAs in cardiovascular diseases. Circ Res. 2015;116(4):737–750.
- Zhang Y, Zhang X, Cai B, et al. The long noncoding RNA lncCIRBIL disrupts the nuclear translocation of Bclaf1 alleviating cardiac ischemia-reperfusion injury. Nat Commun. 2021;12(1):522. .
- Qian Y, Zhang M, Zhou N, et al. A Long Noncoding RNA CHAIR protects the heart from pathological stress. Clin Sci. 2020;134(13):1843–1857.
- Wang Y, Song X, Li Z, et al. Long non-coding RNAs in coronary atherosclerosis. Life Sci. 2018;211:189–197.
- Quan W, Hu PF, Zhao X, et al. Expression level of lncRNA PVT1 in serum of patients with coronary atherosclerosis disease and its clinical significance. Eur Rev Med Pharmacol Sci. 2020;24:6333–6337.
- Zhu B, Liu J, Zhao Y, et al. lncRNA-SNHG14 promotes atherosclerosis by regulating RORα expression through sponge miR-19a-3p. Comput Math Methods Med. 2020;2020:3128053.
- Ghaiad HR, Elmazny AN, Nooh MM, et al. Long noncoding RNAs APOA1-AS, IFNG-AS1, RMRP and their related biomolecules in Egyptian patients with relapsing-remitting multiple sclerosis: relation to disease activity and patient disability. J Adv Res. 2020;21:141–150.
- Zhang Y, Zhang L, Wang Y, et al. KCNQ1OT1,HIF 1A-AS2 and APOA1-ASare promising novel biomarkers for diagnosis of coronary artery disease. Clin Exp Pharmacol Physiol. 2019;46(7):635–642. .
- Abd-Elmawla MA, Fawzy MW, Rizk SM, et al. Role of long non-coding RNAs expression (ANRIL, NOS3-AS, and APOA1-AS) in development of atherosclerosis in Egyptian systemic lupus erythematosus patients. Clin Rheumatol. 2018;37(12):3319–3328.
- Gong C, Tang Y, Maquat LE. mRNA-mRNA duplexes that autoelicit Staufen1-mediated mRNA decay. Nat Struct Mol Biol. 2013;20(10):1214–1220.
- Guo B, Wu S, Zhu X, et al. Micropeptide CIP2A-BP encoded by LINC 00665 inhibits triple-negative breast cancer progression. EMBO J. 2020;39(1):e102190. .
- Guo L, Zhang S, Zhang B, et al. Silencing GTSE-1 expression inhibits proliferation and invasion of hepatocellular carcinoma cells. Cell Biol Toxicol. 2016;32(4):263–274.
- Ruan X, Zheng J, Liu X, et al. lncRNA LINC00665 Stabilized by TAF15 Impeded the Malignant Biological Behaviors of Glioma Cells via STAU1-Mediated mRNA Degradation. Mol Ther Nucleic Acids. 2020;20:823–840.
- Ren P, Xing L, Hong X, et al. LncRNA PITPNA-AS1 boosts the proliferation and migration of lung squamous cell carcinoma cells by recruiting TAF15 to stabilize HMGB3 mRNA. Cancer Med. 2020;9(20):7706–7716.
- Feng XH, Derynck R. Specificity and versatility in tgf-beta signaling through Smads. Annu Rev Cell Dev Biol. 2005;21(1):659–693.
- DiRenzo DM, Chaudhary MA, Shi X, et al. A crosstalk between TGF-β/Smad3 and Wnt/β-Catenin pathways promotes vascular smooth muscle cell proliferation. Cell Signal. 2016;28(5):498–505.
- Shi X, DiRenzo D, Guo LW, et al. TGF-β/Smad3 stimulates stem cell/developmental gene expression and vascular smooth muscle cell de-differentiation. PloS One. 2014;9(4):e93995.
- He Z, Xue H, Liu P, et al. MiR-4286/TGF-β1/Smad3 negative feedback loop ameliorated vascular endothelial cell damage by attenuating apoptosis and inflammatory response. J Cardiovasc Pharmacol. 2020;75(5):446–454.
- Gao ZF, Ji XL, Gu J, et al. microRNA-107 protects against inflammation and endoplasmic reticulum stress of vascular endothelial cells via KRT1-dependent Notch signaling pathway in a mouse model of coronary atherosclerosis. J Cell Physiol. 2019;234:12029–12041.
- Yu DR, Wang T, Huang J, et al. MicroRNA-9 overexpression suppresses vulnerable atherosclerotic plaque and enhances vascular remodeling through negative regulation of the p38MAPK pathway via OLR1 in acute coronary syndrome. J Cell Biochem. 2020;121(1):49–62.
- Jin C, Gao S, Li D, et al. MiR-182-5p inhibits the proliferation of vascular smooth muscle cells induced by ox-LDL through targeting PAPPA. Int Heart J. 2020;61(4):822–830. .
- Aravani D, Morris GE, Jones PD, et al. HHIPL1, a gene at the 14q32 coronary artery disease locus, positively regulates hedgehog signaling and promotes atherosclerosis. Circulation. 2019;140(6):500–513.
- Zhang M, Zhu R, Zhang L. Triclosan stimulates human vascular endothelial cell injury via repression of the PI3K/Akt/mTOR axis. Chemosphere. 2020;241:125077.
- Brown BA, Connolly GM, Mill CEJ, et al. Aging differentially modulates the Wnt pro-survival signalling pathways in vascular smooth muscle cells. Aging Cell. 2019;18:e12844.
- Talior-Volodarsky I, Connelly KA, Arora PD, et al. α11 integrin stimulates myofibroblast differentiation in diabetic cardiomyopathy. Cardiovasc Res. 2012;96(2):265–275.
- Iyer D, Zhao Q, Wirka R, et al. Coronary artery disease genes SMAD3 and TCF21 promote opposing interactive genetic programs that regulate smooth muscle cell differentiation and disease risk. PLoS Genet. 2018;14(10):e1007681. .
- Zheng J, Tan Q, Chen H, et al. lncRNA‑SNHG7‑003 inhibits the proliferation, migration and invasion of vascular smooth muscle cells by targeting the miR‑1306‑5p/SIRT7 signaling pathway. Int J Mol Med. 2020;47(2):741–750.
- Shan K, Jiang Q, Wang XQ, et al. Role of long non-coding RNA-RNCR3 in atherosclerosis-related vascular dysfunction. Cell Death Dis. 2016;7(6):e2248. .
- Zhang L, Cheng H, Yue Y, et al. H19 knockdown suppresses proliferation and induces apoptosis by regulating miR-148b/WNT/β-catenin in ox-LDL -stimulated vascular smooth muscle cells. J Biomed Sci. 2018;25(1):11.
- Brinegar AE, Cooper TA. Roles for RNA-binding proteins in development and disease. Brain Res. 2016;1647:1–8.
- Miyazono K. TGF-beta/SMAD signaling and its involvement in tumor progression. Biol Pharm Bull. 2000;23(10):1125–1130.
- Chen C, Lei W, Chen W, et al. Serum TGF-β1 and SMAD3 levels are closely associated with coronary artery disease. BMC Cardiovasc Disord. 2014;14(1):18.