ABSTRACT
Lung adenocarcinoma (LUAD) is a deadly cancer with a high incidence worldwide. Long noncoding RNAs (lncRNAs) have been confirmed to have the regulatory effects on the occurrence and development of LUAD. But the specific functions of lncRNA GLIDR in LUAD are still not explicit and need to be investigated. On the basis of the outcomes of RT-qPCR experiments, the relative expression of GLIDR was evidently up-regulated in LUAD cells, while that of miR-1270 was down-regulated. The down-regulation of GLIDR inhibits cell proliferation in accordance with the results of CCK-8, EdU and colony formation assays, and accelerates cell apoptosis according to the results of flow cytometry and JC-1 analyses. Luciferase reporter, RNA pull down and RIP assays indicated that GLIDR could sponge miR-1270 in LUAD. Additionally, TCF12 was proved as the target gene of miR-1270. Furthermore, rescue experiments indicated that overexpression of TCF12 could offset the inhibitory functions of silencing GLIDR on cell behaviors. In brief, this study has demonstrated that GLIDR/miR-1270/TCF12 axis plays the crucial role in LUAD, which offers a new insight into researches on molecular mechanism concerning LUAD and provides with a new perspective for LUAD treatment.
KEYWORDS:
Introduction
Lung adenocarcinoma (LUAD) is a type of non-small cell carcinoma. Both of them belong to the category of lung cancer [Citation1], which remains the leading cause of cancer-related deaths with an estimate of 1.8 million deaths according to GLOBOCAN 2020. Owing to its high incidence and mortality, LUAD has been a focus of medical research. Smoking has been considered to be the crucial factor in the development of LUAD so far [Citation2]. Although screening methods and treatment options have been improving, most patients are diagnosed in late stage because of the early symptoms are not evident [Citation3], which leads to a dismally low overall survival rate as well as poor prognosis [Citation4]. Therefore, it is imperative to explore the molecular mechanism underlying LUAD for developing effective strategies to diagnose and treat LUAD.
Long noncoding RNAs (lncRNAs) are a type of non-coding RNAs containing longer than 200 nucleotides without the capability of coding proteins [Citation5,Citation6]. Increasing evidence supports that aberrant expressions of lncRNAs play crucial roles in the progression of diseases [Citation7,Citation8], and even in various human cancers, including LUAD [Citation9]. Also, dysregulated lncRNAs have been validated to take part in various cellular processes, like cell proliferation and cell apoptosis. For example, LUADT1 is overexpressed in LUAD and stimulates cell proliferation via the epigenetic inhibition of p27 [Citation10]; SOX21-AS1 is up-regulated in LUAD and promotes LUAD cell growth both in vitro and in vivo and impedes cell-cycle arrest and cell apoptosis. [Citation11]. More importantly, competing endogenous RNA (ceRNA) network has been discovered, where lncRNAs function as ceRNA to regulate the progression of multiple cancers [Citation12]. Specifically, lncRNAs can modulate the expression of messenger RNA (mRNA) through competitively combining with microRNA (miRNA). For example, HMMR-AS1 plays an oncogenic role in LUAD via acting as a ceRNA of miR-138 to repress sirt6 expression [Citation13].
As a novel lncRNA, there are few researches on GLIDR in cancers at present. According to the known literature, GLIDR was upregulated in prostate cancer tissue samples and cell lines and GLIDR could expedite the progression of prostate cancer through ceRNA network [Citation14]. Nevertheless, the specific function and underlying mechanism of GLIDR in LUAD remain unclear.
Herein, we aimed to investigate the biological role and regulatory mechanism of GLIDR in LUAD through a chain of functional assays and mechanism investigations. Also, the GLIDR-mediated ceRNA network in LUAD was explored.
Materials and methods
Cell culture
LUAD cell lines (A549, NCI-H1975, NCI-H1568 and NCI-H2110) were all procured from the ATCC (Manassas, VA, USA) and the human bronchial epithelial cell line (16HBE) was obtained from Procell Life Science & Technology Co., Ltd. (Wuhan, China). All the cells were maintained at 37°C with 5% CO2. RPMI-1640 medium (Gibco, Grand Island, NY, USA) was employed to culture NCI-H1975, NCI-H1568, NCI-H2110 and 16HBE cells separately. A549 cells were kept in F-12 K medium (Gibco). Ten percent fetal bovine serum (FBS; Gibco) served as a supplement for the above mediums.
Cell transfection
LUAD cells were seeded into 6-well plates at a density of 1 × 106 cells per well for the transfection with the specific shRNAs (GenePharma, Shanghai, China) targeting GLIDR or TCF12 and their nonspecific shRNAs (sh/NC). In addition, miR-1270 mimics/inhibitor and NC mimics/inhibitor as well as pcDNA3.1/TCF12 and pcDNA3.1 were obtained from RiboBio (Guangzhou, China). Lipofectamine 3000 reagent (Invitrogen, Carlsbad, CA, USA) was used for cell transfection for 48 hours.
Real-time quantitative polymerase chain reaction (RT-qPCR)
Total RNA was extracted from LUAD cells by TRIzol Reagent (Invitrogen). Afterward, PrimeScript™ II Reverse Transcriptase (Takara, Kusatsu, Japan) was utilized to conduct complementary DNA (cDNA) synthesis. Next, qPCR was performed with SYBR Green Master Mix (Roche, Basel, Switzerland) on Bio-Rad CFX96 System (Bio-Rad, Hercules, CA, USA). Finally, the relative gene expression was calculated by 2−ΔΔCt method; mean values of cycle threshold (Ct) for GLIDR, miR-1270, TCF12 and housekeeping gene (GAPDH or U6) were reported in Supplementary Table 1. Bio-repeats were conducted in triplicate.
Western blot analysis
Total protein extracted from transfected LUAD cells using RIPA lysis buffer was subjected to SDS-PAGE and transferred into PVDF membranes. Then, membranes were sealed with 5% nonfat dry milk for 60 min. Subsequently, blots were incubated with specific antibody against TCF12 (anti-TCF12, Abcam, Cambridge, MA, USA) overnight at 4°C. GAPDH (Abcam) was used as internal reference. Protein concentration was quantified using Bradford’s method. Bio-repeats were conducted in triplicate.
Colony formation assay
The transfected LUAD cells were seeded into 6-well plates at a density of 500 cells per well and cultivated for 14 days. After that, colonies were subjected to fixation by 4% paraformaldehyde. Then, 0.5% crystal violet was utilized to stain cells for 20 min. In the end, the number of colonies was counted manually. Bio-repeats were conducted in triplicate.
EdU assay
The transfected LUAD cells were seeded in the 96-well plates at a density of 1 × 104 cells per well and then treated with BeyoClick™ EdU Cell Proliferation Kit (Beyotime, Shanghai, China). Cell nuclei were stained by DAPI solution. Finally, we applied a fluorescence microscope (Olympus, Tokyo, Japan) to observe the EdU positive cells. Bio-repeats were conducted in triplicate.
CCK-8 assay
After transfection, LUAD cells (5 × 103) were seeded in 96-well plates and then 10 μL of CCK-8 solution (Dojindo, Kumamoto, Japan) was supplemented into the plates for 2 h. After that, we utilized the spectrophotometer (Thermo Fisher Scientific, Waltham, MA, USA) to detect the optical density values at 450 nm. Bio-repeats were conducted in triplicate.
Flow cytometry analysis
After being seeded in 6-well plates, the transfected LUAD cells were washed with PBS (Beyotime) and fixed with ice-cold ethanol (Sigma-Aldrich, St. Louis, MO, USA). Annexin V in conjunction with propidium iodide (PI) labeling of cells was widely used to identify viable, apoptotic, or necrotic cells [Citation15]. Then PI and Annexin V-fluorescein isothiocyanate was used to double stain transfected LUAD cells. Finally, the flow cytometry was applied for detecting cell apoptosis. Bio-repeats were conducted in triplicate.
JC-1 analysis
Cells were cultivated with 10 mM JC-1 (Beyotime) for 30 min. Then, cells were washed with PBS and observed by EnSpire Reader. The loss of mitochondrial membrane potential indicates apoptosis. The fluorescence rate at 590 nm versus 530 nm emission was applied to estimate the mitochondrial membrane potential. Bio-repeats were conducted in triplicate.
Subcellular fractionation assay
As per the protocols of supplier, we utilized Cytoplasmic & Nuclear RNA Purification Kit (Norgen Biotek Corp, Thorold, ON, Canada) to conduct subcellular fractionation assay in LUAD cells. The cell fractionation buffer was added to isolate cytoplasmic and nuclear fractions. Subsequently, the GLIDR level in cytoplasmic and nuclear fractions was respectively tested through RT-qPCR. GAPDH and U6 served as cytoplasmic and nuclear controls separately. Bio-repeats were conducted in triplicate.
FISH assay
RNA FISH probes specific to GLIDR were purchased from RiboBio. Subsequent to air drying, cells were cultivated with probes in hybridization buffer. Additionally, DAPI was applied for the counterstain of nuclei. Lastly, the fluorescence microscope (Olympus) was utilized for observation. Bio-repeats were conducted in triplicate.
RIP assay
On the basis of the supplier’s protocols, Magna RIP™ RNA-Binding Protein Immunoprecipitation Kit (Millipore, Billerica, MA, USA) was used for RIP assay. The transfected LUAD cells were rinsed in ice-cold PBS and then mixed with RIP lysis buffer. Then, cell lysates were incubated with Ago2 antibody conjugated with magnetic beads. IgG antibody served as NC. Finally, the enrichment was detected through RT-qPCR. Bio-repeats were conducted in triplicate.
RNA pull down assay
In accordance with the manufacturer’s suggestions, Pierce Magnetic RNA-Protein Pull-Down Kit (Thermo Scientific, Waltham, MA, USA) was utilized to carry out RNA pull down assay. In brief, protein extracts were cultivated with biotinylated miR-1270 probe (Bio-miR-1270-WT/Bio-miR-1270-Mut), followed by the addition of streptavidin magnetic beads. Next, RT-qPCR was utilized to analyze the enrichment of GLIDR or TCF12 in the pull-down complexes after RNA extraction using TRIzol. Bio-repeats were conducted in triplicate.
Luciferase reporter assay
The sequence of GLIDR full length or TCF12 3’UTR fragment containing the wild type and mutant miR-1270-binding sites were sub-cloned into pmirGLO luciferase vector to construct luciferase reporter vector (GLIDR-WT/GLIDR-Mut or TCF12-WT/TCF12-Mut). Then, luciferase reporter vector was co-transfected with miR-1270 mimics or NC mimics into LUAD cells for 48 h. The luciferase activity of reporter vector was detected with Luciferase expression is driven by the sequence of GLIDR or TCF12 3’UTR responsive to miR-1270 mimics. Dual-luciferase reporter assay system (Promega). Bio-repeats were conducted in triplicate.
Statistical analysis
All experiments in this study were repeated at least three times. The results were represented as mean ± standard deviation (SD). Data were analyzed with GraphPad PRISM 6 (GraphPad, La Jolla, CA, USA) and group difference was analyzed with Student’s t-test or one-way/two-way analysis of variance (ANOVA). The P value less than 0.05 was considered as statistically significant.
Results
GLIDR is high-expressed in LUAD cells and accelerates LUAD cell proliferation
Aberrant expression of lncRNA was considered to be the crucial element in the development of cancers. Thus, we detected GLIDR expression in LUAD cells. The outcomes of RT-qPCR showed that GLIDR possessed the high expression in LUAD cell lines (A549, NCI-H1975, NCI-H1568 and NCI-H2110) in comparison with that in the human bronchial epithelial cell (16HBE) (). It can be found that the expression in NCI-H1568 and NCI-H2110 cells was the highest. Hence, we selected these two cells to perform the following-up experiments for the detection of the functions of GLIDR in LUAD cells. We transfected the silencing plasmids against GLIDR into NCI-H1568 and NCI-H2110 cells and examined the interference efficiency of sh-GLIDR#1/2 through RT-qPCR (). Afterward, a series of functional assays were implemented for evaluating the influences of silenced GLIDR on LUAD cells. We firstly conducted the EdU staining, CCK-8 and colony formation experiments, finding that the EdU positive cells, OD value and the colonies were restrained by the insufficiency of GLIDR (–e). Then, it was detected by flow cytometry and JC-1 experiments that cell apoptotic capability was subjected to acceleration after GLIDR was knocked down (–g). Taken together, GLIDR is overexpressed in LUAD cells and accelerates LUAD cell proliferation.
Figure 1. GLIDR is high-expression in LUAD cells and accelerates cell proliferation. (a) The relative expression of GLIDR, normalized to GAPDH, was detected through RT-qPCR in LUAD cell lines and 16HBE cell line. (b) The interference efficiency of GLIDR was measured via RT-qPCR, with sh-NC as control. (c–e) EdU staining, CCK-8 and colony formation experiments were conducted to test the influence of silenced GLIDR on cell proliferation, with sh-NC as control. (f–g) Flow cytometry and JC-1 experiments were implemented to test cell apoptosis when GLIDR was silenced, with sh-NC as control. *P < 0.05, **P < 0.01
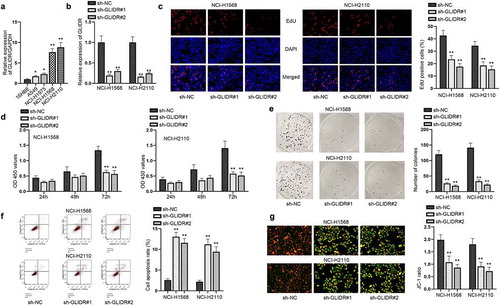
GLIDR directly combines with miR-1270 in LUAD cells
After evaluating the functions of GLIDR in LUAD cells, we investigated the regulatory mechanisms of GLIDR. Firstly, we examined the distribution of GLIDR in NCI-H1568 and NCI-H2110 cells through subcellular fractionation experiments and FISH experiments (–b). The results pointed out that GLIDR was mostly located in the cytoplasm of NCI-H1568 and NCI-H2110 cells, suggesting that GLIDR may function as a ceRNA to regulate the mRNA expression through sponging miRNAs. Hence, we utilized the ENCORI (http://starbase.sysu.edu.cn/index.php) to seek out the potential target miRNAs. Through the bioinformatics tool, we discovered 11 miRNAs that could combine with GLIDR; and then we conducted RT-qPCR analysis to detect their expressions in LUAD cells (). It was evidently observed that the expression of miR-1270 was the lowest. Hence, we carried out pull down experiments to evaluate the relationship of miR-1270 and GLIDR (). The outcomes indicated that GLIDR was enriched in the biotinylated miR-1270-WT group, indicating that GLIDR could combine with miR-1270. For further validation, we found the binding sites of them through ENCORI (). After the verification of efficiency of miR-1270 mimics (), we conducted the luciferase report experiment in NCI-H1568 and NCI-H2110 cells after the co-transfection of GLIDR-WT/Mut and miR-1270 mimics (). Overexpressed miR-1270 could hamper the luciferase activity of GLIDR-WT, which further confirmed their combination. Following, we investigated the functions of miR-1270 in LUAD cells. Through EdU, CCK-8 and colony formation experiments, we discovered that the proliferative ability of NCI-H1568 and NCI-H2110 cells was hampered when miR-1270 was up-regulated (–j). Meanwhile, flow cytometry and JC-1 experiments demonstrated that overexpression of miR-1270 facilitated cell apoptosis (–l). The abovementioned results unveiled that GLIDR directly combines with miR-1270 and overexpressed miR-1270 suppresses cell proliferation in LUAD cells.
Figure 2. GLIDR directly combines with miR-1270 in LUAD cells. (a–b) The location of GLIDR in LUAD cells were estimated by subcellular fractionation assays (GAPDH and U6 served as controls) and FISH assays. (c) MiRNAs expressions were detected via RT-qPCR in LUAD cells normalized to 16HBE. (d) RNA pull down experiments were conducted to test the combined situation of miR-1270 and GLIDR, with Bio-NC as control. (e) The binding site of GLIDR and miR-1270 was predicted by ENCORI. (f) The overexpression efficiency of miR-1270 mimics was measured through RT-qPCR with NC mimics as control. (g) Luciferase report assay was to prove the binding situation of GLIDR and miR-1270 with NC mimics as control. (h–j) The influence of overexpressing miR-1270 on cell proliferation was estimated by EdU staining, CCK-8 and colony formation experiments with NC mimics as control. (k–l) Cell apoptotic ability was tested by flow cytometry and JC-1 experiments with NC mimics as control. **P < 0.01
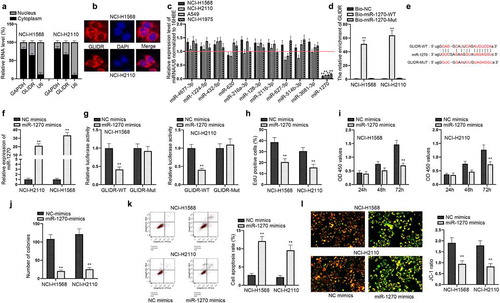
TCF12 is a target of miR-1270 in LUAD cells
In order to further investigate the regulator mechanisms in LUAD, we explored the downstream target gene of miR-1270. Then, we utilized ENCORI to screen out the possible mRNAs binding to miR-1270. Based on the predictions of PITA, miRmap, microT and RNA22, we identified 14 mRNAs (). Only two mRNAs (TCF12, CNOT6) could be down-regulated under the condition of silencing GLIDR and overexpressing miR-1270 simultaneously through RT-qPCR (). The RT-qPCR was conducted again to evaluate their expressions in LUAD cells (). We discovered that TCF12 was highly expressed in LUAD cells, while CNOT6 was evenly expressed in LUAD and normal cells. Thus, we selected TCF12 for further verification. We performed western blot to detect the level of TCF12 after the overexpression of miR-1270, finding that miR-1270 was negatively correlated with TCF12 at the protein level (Figure S1A). The binding sites between TCF12 and miR-1270 were discovered by ENCORI and displayed in . Subsequently, luciferase reporter assay depicted that the luciferase activity of TCF12-WT was inhibited by miR-1270 overexpression but that of TCF12-Mut was not subjected to influences, indicating that TCF12 interacted with miR-1270 via the binding site (). Later, RIP experiments displayed that TCF12 and miR-1270 were enriched in Ago2 group, indicating coexistence of TCF12 and miR-1270 in RISC complex (). RNA pull down experiments further proved the correlation of TCF12 with miR-1270 (). All of these results demonstrated that TCF12 acted as a target of miR-1270 in LUAD cells. For the sake of investigating the functions of TCF12 in LUAD cells, we knocked down TCF12 expression and conducted several functional experiments. RT-qPCR analysis displayed that TCF12 expression could be inhibited after the transfection of sh-TCF12#1/2 (). Through EdU, CCK-8 and colony formation experiments, we discovered that silenced TCF12 hampered cell proliferation (–k). At the same time, cell apoptosis was accelerated by inhibition of TCF12 in accordance with the results of flow cytometry and JC-1 assays (–m). To further verify the functions of TCF12, we performed functional experiments in 16HBE cells. The overexpression efficiency of pcDNA3.1-TCF12 in 16HBE cells was assessed by RT-qPCR (Figure S1B). According to the results of EdU and flow cytometry assays, cell proliferation was promoted and cell apoptosis was inhibited by the overexpression of TCF12 (Figure S1C–D). Taken together, TCF12 is a target of miR-1270 in LUAD cells.
Figure 3. TCF12 is a target of miR-1270 in LUAD cells. (a) Venn diagram showed the target genes of miR-1270 predicted by ENCORI. (b) The utilization of RT-qPCR was to pick out the potential mRNAs that could be regulated by silenced GLIDR and overexpressed miR-1270 at the same time. (c) RT-qPCR was implemented to detect the expressions of mRNAs in LUAD cells, normalized to GAPDH. (d) The binding site of miR-1270 and TCF12 was predicted. (e–g) Luciferase report (NC mimics as control), RIP (IgG as control) and pull down experiments (Bio-NC as control) were implemented to prove the interaction of miR-1270 and TCF12. (h) RT-qPCR was utilized to estimate knockdown efficiency of TCF12 with sh-NC as control. (i–k) Silenced TCF12 was transfected into cells to detect cell proliferation via EdU staining, CCK-8 and colony formation assays with sh-NC as control. (l–m) Cell apoptotic ability was evaluated by flow cytometry and JC-1 experiments when TCF12 was inhibited, with sh-NC as control. *P < 0.05, **P < 0.01
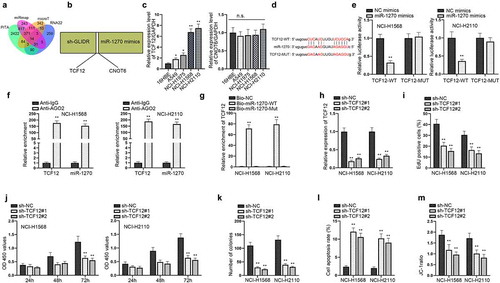
GLIDR/miR-1270/TCF12 axis modulates the progression of LUAD cells
We carried out rescue experiments to prove whether the regulatory mechanism of GLIDR/miR-1270/TCF12 axis could influence the development of LUAD. First of all, we detected the knockdown efficiency of miR-1270 and the overexpression efficiency of TCF12 by utilizing RT-qPCR (–b). We conducted western blot and found that the inhibitory effect of ablated GLIDR on TCF12 protein level could be countervailed by miR-1270 depletion or TCF12 up-regulation (Figure S1E). Afterward, the rescue experiments combined with EdU staining, CCK-8 and colony formation assays were implemented, and the outcomes disclosed that the inhibitory effect of silencing GLIDR on cell proliferation could be offset by miR-1270 depletion or TCF12 up-regulation (–e). Following, through flow cytometry and JC-1 experiments, we observed that cell apoptotic capability could be accelerated by silenced GLIDR, but then recovered by knockdown of miR-1270 or overexpression of TCF12 (–g). Overall, GLIDR could accelerate the progression of LUAD cells by regulating miR-1270/TCF12 axis.
Figure 4. GLIDR/miR-1270/TCF12 axis modulates cell growth in LUAD cells. (a–b) RT-qPCR was utilized to detect the interference efficiency of miR-1270 inhibitor and the overexpression efficiency of pcDNA3.1-TCF12, with NC inhibitor and pcDNA3.1-NC as control, respectively. (c–e) EdU staining, CCK-8 and colony formation experiments were implemented to evaluate the influence of silenced miR-1270 or overexpressed TCF12 on the inhibited cell proliferation induced by silenced GLIDR, with sh-NC as control. (f–g) The influence of inhibited miR-1270 or up-regulated TCF12 on cell apoptosis which was accelerated by GLIDR depletion was measured by flow cytometry and JC-1 experiments with sh-NC as control. **P < 0.01
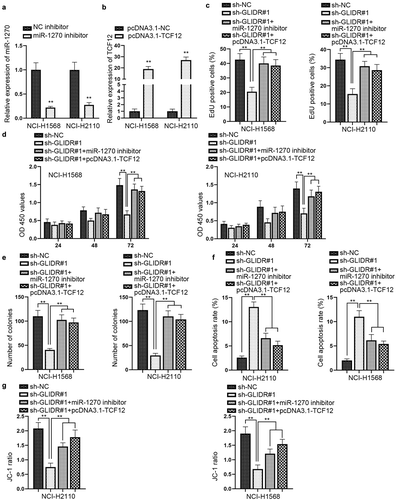
Discussion
Lung adenocarcinoma (LUAD) is a kind of malignant tumor with high incidence and mortality all over the world, which threatens human health. Several researches have proven that lncRNAs can exert the regulatory functions in the process of cancers, including LUAD. For example, LINC00857 predicts bad prognosis and facilitates tumor growth in LUAD [Citation16]. Overexpressed CCAT1 can expedite invasion and migration in LUAD cells [Citation17]. Linc00665 acts as a ceRNA to accelerate the development of LUAD through sponging miR-98 [Citation18]. However, the latent roles of GLIDR in LUAD remain to be explored. In our current research, we found that GLIDR was highly expressed in LUAD cells according to the outcomes of RT-qPCR. The functional experiments indicated that GLIDR depletion could hamper cell proliferation. However, the cell apoptosis could be enhanced by the inhibition of GLIDR. All of these results demonstrated that GLIDR exerted the carcinogenic effect in LUAD cells.
MiRNAs are small non-coding RNAs and the length of them was about 22 ~ 24 nucleotides. Like lncRNAs, miRNAs lack the ability to code proteins as well [Citation19]. MiRNAs have been proven that they serve as the vital regulatory factors in cancers. For example, miR-125a hinders tumor growth in cervical cancer through targeting STAT3 [Citation20]. And miR-17-5p accelerates tumorigenesis in nasopharyngeal carcinoma by targeting p21 [Citation21]. Furthermore, miR-1270 was confirmed to be down-regulated in glioblastoma cells and overexpressed miR-1270 can inhibit cell proliferation and migration [Citation22], which means that miR-1270 serves as the tumor suppressor gene in glioblastoma. In ceRNA network, miRNA can be sponged by lncRNA, so as to regulate cancer development. Thus, our research investigated the relationships of miR-1270 and GLIDR in LUAD cells. Through subcellular fractionation experiments and FISH experiments, we affirmed that GLIDR was a cytoplasm lncRNA, indicating that it exerts the function at the post-transcription level. As ceRNA mode is a post-transcription regulation, we speculated that GLIDR functions as a ceRNA to sponge miRNA. Thus, we identified miR-1270 through ENCORI. After conducting luciferase report and pull down assays, we confirmed that GLIDR directly combines with miR-1270 in LUAD cells. Moreover, functional experiments demonstrated that miR-1270 could suppress cell growth in LUAD cells.
TCF12 is a member of HLH protein family, which has been proven to be closely related to cancer process. For example, miR-211 accelerates the development of oral carcinoma through inhibiting TCF12 [Citation23]. And overexpressed TCF12 expedites gastric cancer progression [Citation24]. In our research, TCF12 was highly expressed in LUAD cells. We proved that TCF12 was a target of miR-1270 in LUAD cells via mechanism experiments. In addition, the rescue experiments demonstrated that up-regulated TCF12 could reverse the inhibition of silenced GLIDR on the progression of LUAD cells, suggesting that GLIDR acted as the oncogene in LUAD cells via TCF12.
In conclusion, GLIDR could accelerate the tumorigenesis of LUAD by sponging miR-1270 to target TCF12, which offers a new sight into the investigation of LUAD therapies.
Supplemental Material
Download Zip (17.3 KB)Acknowledgments
We thank all the participants.
Data availability statement
The data that support the findings of this study are available from the corresponding author upon reasonable request.
Disclosure statement
No potential conflict of interest was reported by the author(s).
Supplementary material
Supplemental data for this article can be accessed here.
Additional information
Funding
References
- Wistuba II. Genetics of preneoplasia: lessons from lung cancer. Curr Mol Med. 2007;7(1):3–14.
- de Groot P, Munden RF. Lung cancer epidemiology, risk factors, and prevention. Radiol Clin North Am. 2012;50(5):863–876.
- Meng Q, Ren M, Li Y, et al. LncRNA-RMRP acts as an oncogene in lung cancer. PloS One. 2016;11(12):e0164845.
- Li P, Zhang G, Li J, et al. Long noncoding RNA RGMB-AS1 indicates a poor prognosis and modulates cell proliferation, migration and invasion in lung adenocarcinoma. PloS One. 2016;11(3):e0150790.
- Li J, Li Z, Zheng W, et al. LncRNA-ATB: an indispensable cancer-related long noncoding RNA. Cell Prolif. 2017;50(6):e12381.
- Jarroux J, Morillon A, Pinskaya M. History, discovery, and classification of lncRNAs. Adv Exp Med Biol. 2017;1008:1–46.
- Cai P, Li H, Huo W, et al. Aberrant expression of LncRNA-MIR31HG regulates cell migration and proliferation by affecting miR-31 and miR-31* in Hirschsprung’s disease. J Cell Biochem. 2018;119(10):8195–8203.
- Li D, Zhang J, Li X, et al. Insights into lncRNAs in Alzheimer’s disease mechanisms. RNA Biol. 202118(7):1037-1047.
- He F, Huang L, Xu Q, et al. Microarray profiling of differentially expressed lncRNAs and mRNAs in lung adenocarcinomas and bioinformatics analysis. Cancer Med. 2020;9(20):7717–7728.
- Qiu M, Xu Y, Wang J, et al. A novel lncRNA, LUADT1, promotes lung adenocarcinoma proliferation via the epigenetic suppression of p27. Cell Death Dis. 2015;6(8):e1858.
- Lu X, Huang C, He X, et al. A novel long non-coding RNA, SOX21-AS1, indicates a poor prognosis and promotes lung adenocarcinoma proliferation. Cell Physiol Biochem. 2017;42(5):1857–1869.
- Qi X, Zhang DH, Wu N, et al. ceRNA in cancer: possible functions and clinical implications. J Med Genet. 2015;52(10):710–718.
- Cai Y, Sheng Z, Chen Y, et al. LncRNA HMMR-AS1 promotes proliferation and metastasis of lung adenocarcinoma by regulating MiR-138/sirt6 axis. Aging (Albany NY). 2019;11(10):3041–3054.
- Zhang Y, Kong Z, Zhang Y, et al. Increased expression of long non-coding RNA GLIDR in prostate cancer. Cancer Biomark. 2017;19(2):145–150.
- Rieger AM, Nelson KL, Konowalchuk JD, et al. Modified annexin V/propidium iodide apoptosis assay for accurate assessment of cell death. JoVE. 2011;(50). DOI: https://doi.org/10.3791/2597.
- Wang L, He Y, Liu W, et al. Non-coding RNA LINC00857 is predictive of poor patient survival and promotes tumor progression via cell cycle regulation in lung cancer. Oncotarget. 2016;7(10):11487–11499.
- Lin H, Cheng W, Yan H, et al. Overexpression of the long noncoding RNA CCAT1 promotes metastasis via epithelial-to-mesenchymal transition in lung adenocarcinoma. Oncol Lett. 2018;16(2):1809–1814.
- Cong Z, Diao Y, Xu Y, et al. Long non-coding RNA linc00665 promotes lung adenocarcinoma progression and functions as ceRNA to regulate AKR1B10-ERK signaling by sponging miR-98. Cell Death Dis. 2019;10(2):84.
- Guo Z, Zhou H, Zhang W. [Progress in research on genetic variations in miRNA regulatory pathway]. Zhonghua Yi Xue Yi Chuan Xue Za Zhi. 2015;32(1):109–112.
- Fan Z, Cui H, Xu X, et al. MiR-125a suppresses tumor growth, invasion and metastasis in cervical cancer by targeting STAT3. Oncotarget. 2015;6(28):25266–25280.
- Chen C, Lu Z, Yang J, et al. MiR-17-5p promotes cancer cell proliferation and tumorigenesis in nasopharyngeal carcinoma by targeting p21. Cancer Med. 2016;5(12):3489–3499.
- Wei L, Li P, Zhao C, et al. Upregulation of microRNA-1270 suppressed human glioblastoma cancer cell proliferation migration and tumorigenesis by acting through WT1. Oncol Targets Ther. 2019;12:4839–4848.
- Chen YF, Yang CC, Kao SY, et al. MicroRNA-211 enhances the oncogenicity of carcinogen-induced oral carcinoma by repressing TCF12 and increasing antioxidant activity. Cancer Res. 2016;76(16):4872–4886.
- Wang X, Gao S, Xie F, et al. Retracted: high expression of TCF12 contributes to gastric cancer development via being target regulated by miR-183 and activating PI3K/AKT pathway. J Cell Biochem. 2019;120(8):13903–13911.