ABSTRACT
Increasing evidences have confirmed that long non-coding RNA LOXL1-AS1 functions in multiple human diseases. Here, we aim to explore the function and mechanism of LOXL1-AS1 in modulating oxidized low-density lipoprotein (ox-LDL)-induced angiogenesis of endothelial cells (ECs). Presently, we found that LOXL1-AS1 and KLF6 were upregulated in ECs treated by Ox-LDL in a dose- and time-dependent manner while miR-590-5p was downregulated. Overexpression of LOXL1-AS1 aggravated Ox-LDL mediated ECs proliferation and migration, and promoted angiogenesis both in vitro and in vivo. On the contrary, enhancing miR-590-5p or inhibiting LOXL1-AS1 level led to suppressive effects on the proliferation, migration and angiogenesis of ECs. Moreover, LOXL1-AS1 upregulation promoted the expression of vascular endothelial growth factor (VEGF), MMPs (including MMP2, MMP9 and MMP14) and also activated VEGF/VEGFR2/PI3K/Akt/eNOS pathway. Mechanistically, LOXL1-AS1 works as a competitive endogenous RNA (ceRNA) by sponging miR-590-5p, which targeted at the 3ʹ-untranslated region (3ʹUTR) of KLF6. Additionally, the proliferation, migration and angiogenesis of ECs were elevated following KLF6 upregulation. By detecting the expression of LOXL1-AS1 and miR-590-5p in the serum of healthy donors and atherosclerosis patients, it was found that LOXL1-AS1 was upregulated in atherosclerosis patients (compared with healthy donors) and had a negative relationship with miR-590-5p. Taken together, LOXL1-AS1 promoted Ox-LDL induced angiogenesis via regulating miR-590-5p-modulated KLF6/VEGF signaling pathway. The LOXL1-AS1-miR-590-5p axis exerts a novel role in the progression of atherosclerosis.
1 Background
Atherosclerosis (AS), as a chronic inflammatory vascular disease, often results in atherosclerotic plaque, which is a focal lesion located within the intima of large and medium-sized arteries [Citation1]. Atherosclerosis, as a typical pathological change in clinical, is one of the leading causes of various clinical events, including ischemic shock and myocardial infarction [Citation2]. During the development of AS, oxidized low-density lipoprotein (Ox-LDL) is involved in the development of AS through a variety of mechanisms, such as inducing endothelial cell activation and dysfunction, accelerating macrophage foam cell formation, promoting smooth muscle cell migration and proliferation, etc [Citation3,Citation4]. Vascular endothelial cells, which are attached to the inner wall of blood vessels, have phagocytose functions on foreign body, bacteria, necrosis and aged tissues, and participate in collective immune activities. Their damage and aberrant function are the initial factors contributes to AS [Citation5,Citation6]. Therefore, it is of considerable significance to maintain the integrity of endothelial cells and protect their normal functions for the treatment of AS to explore the way.
Long non-coding RNA, a kind of RNA more than 200 nucleotides in length, is identified in multiple cells. Mounting studies have indicated that lncRNAs take part in the fundamental processes of gene modulation, including chromatin modification and direct transcriptional regulation, as well as post-transcriptional events including splicing, editing, localization, transfection and degradation [Citation7,Citation8]. In recent years, many studies have proved that lncRNA exerts a significant effect on cardiovascular diseases, neurological diseases and immune-mediated diseases [Citation9–11], including in the development of AS [Citation12,Citation13]. LncRNA LOXL1 antisense RNA 1 (LOXL1‐AS1) has been identified by Hauser MA et al. [Citation14], it is located on human chromosome 15q24.1, which consists of 10,781 nucleotides and five exons. Studies have discovered that LOXL1-AS1 is upregulated in many tumors, such as ovarian cancer [Citation15], endometrial cancer [Citation16], and gastric cancer [Citation17], and it promotes tumor cell proliferation, migration, and invasion. Moreover, recent studies also proved that LOXL1-AS1 has a vital role in the non-tumor diseases. For instance, LOXL1-AS1 aggravates the proliferation and migration of trophoblast cells (JEG-3) [Citation18]; LOXL1-AS1 has abnormal high expression in patients with postmenopausal osteoporosis, and it inhibits the osteogenic differentiation of human bone marrow mesenchymal stem cells (hBMMSCs) but promotes adipocytic differentiation [19]. Thereby, we thought that LOXL1-AS1 plays a potential role in Ox-LDL-mediated dysfunctions of ECs.
Similar to lncRNA, microRNAs (miRNAs) are a class of non-coding RNAs widely found in cells. miRNAs are about 18–24 nucleotides in length, and they modulate gene expression at the post-transcriptional level by binding to the 3ʹUTR end of mRNA [Citation19]. Accumulating researches have indicated that miRNAs participate in regulating Ox-LDL-mediated dysfunction of vascular endothelial cells. Taking microRNA-338-3p as an example, it is upregulated in vascular endothelial cells after Ox-LDL treatment, and it mediates endothelial cell injury by targeting BAMBI and activating TGF-β/Smad Pathway [Citation20]. As a member of miRNAs, miR-590-5p takes part in mediating tumor growth and drug resistance [Citation21], and plays a therapeutic role in intestinal inflammation [Citation22] and brain injury caused by cerebral hemorrhage [Citation23]. Interestingly, previous studies have found that miR-590-5p attenuates Ox-LDL-mediated angiogenesis by targeting LOX-1 [Citation24], suggesting that miR-590-5p may exert a momentous effect in AS development.
KLF6 is one member of Krüppel-like factor family, and the zinc finger protein is a transcriptional activator, which works as a tumor suppressor. For this gene, multiple transcript variants encoding different isoforms are discovered, some of which have carcinogenic effect [Citation25]. Besides, KLF6 has been found to promote inflammatory response by inhibiting the expression of microRNA-223 (miR-223) in macrophages [Citation26] and to aggravate pathogenic myeloid cell activation in human and experimental inflammatory bowel disease (IBD) [Citation27]. Therefore, KFL6 is a pro-inflammatory transcription factor.
Here, we used the low concentration (5–20 μg/ml) Ox-LDL on human umbilical vein endothelial cells (HUVECs) and found that Ox-LDL significantly promoted the LOXL1-AS1 expression and suppressed miR-590-5p expression. Moreover, gain- and loss-of functional experiments confirmed that LOXL1-AS1 promoted Ox-LDL-mediated proliferation, migration and angiogenesis of HUVECs, while miR-590-5p had the opposite effects. What is more, we found that LOXL1-AS1 targeted miR-590-5p as a competitive endogenous RNA (ceRNA), while the miR-590-5p targeted KLF6. Therefore, we hypothesized that there was a regulatory network in Ox-LDL-mediated angiogenesis, namely the LOXL1-AS1-miR-590-5p-KLF6 axis.
2 Methods
2.1 Cells and treatments
HUVECs from Cell Bank of the Chinese Academy of Sciences (Shanghai, China) were cultured in a complete medium containing 10% fetal bovine serum (FBS, Gibco BRL, Gaithersburg, MD, USA) and 1% DEME-F12 (Thermo, Shanghai, China). The cell culture bottle/plate was put in a constant temperature incubator containing 5% CO2 at 37°C. When the cells were at the logarithmic growth phase, Ox-LDL (5–20 μg/ml) (Beijing Solarbio Science & Technology Co., Ltd.) was acted on HUVECs for a duration of time (0 h-72 h).
2.2 Cell culture and treatment
The pcDNA3.1 expression vectors, which contained full-length human LOXL1-AS1 (pcDNA-LOXL1-AS1) or KLF6 (pcDNA-KLF6), and their negative vectors were designed and synthesized by Shanghai Integrated Biotech Solutions (Shanghai, China). The small interference RNA (siRNA) targeting LOXL1-AS1 (si-LOXL1-AS1), miR-590-5p mimics and the corresponding negative controls were purchased from RiboBio (Guangzhou, China). The si-LOXL1-AS1 sequences include # 1 5ʹ- AUUCUUACCAUUAUUCCUGUCCAGGAAUAAUGGUAAGAAUUU-3ʹ, # 2 5ʹ- AGCAAAUAGACCAUUUAGGAGCCUAAAUGGUCUAUUUGCUCC-3ʹ, # 3 5ʹ- UUAUGAAGUAUUUUAGACGGUCGUCUAAAAUCUAU. HUVECs at the logarithmic growth stage were inoculated into 24-well plates. Then, Lipofectamine 3000 kit (Thermo, Shanghai, China) was used to co-transfected pcDNA-LOXL1-AS1, si-LOXL1-AS1, pcDNA-KLF6, miR-590-5p mimics and the corresponding negative control into the cells according to the instructions. Twenty-four hours after transfection, the expressions of LOXL1-AS1, miR-590-5p, or KLF6 were detected for confirming the transfection efficiency via RT-PCR or Western blot.
2.3 CCK8 method
The CCK8 assay was used for evaluating the proliferation of HUVECs using the CCK8 kit (Beyotime, Shanghai, China). HUVECs inoculated in 96-well plates (5 × 103 cells/well) were cultured for 24 hours. The previous medium was removed, and the CCK-8 and medium mixture (CCK-8 to medium ratio was 1:10) were added. After incubation in a standard incubator for 1 hour, the absorbance value of the culture plate at 450 nm was determined via ELISA. In this experiment, the same amount of CCK-8 and the medium mixture was taken as the blank control without cells. Five replicates were set up in each experiment, and the experiment was made three times.
2.4 Reverse transcription polymerase chain reaction (RT-PCR)
When the cell treatment was over, HUVECs were collected and lysed with TRIzol lysate (Invitrogen, Shanghai, China) on ice. Chloroform-isopropanol-75% ethanol method was used to extract the total RNA in the lysates, and double distilled water containing 1% DEPC (Thermo, Shanghai, China) was used to dissolve the total RNA. The ultraviolet RNA spectrophotometer was used to determine the total RNA concentration and purity. According to the operating procedure of the RevertAid First Strand cDNA Synthesis Kit (Thermo, Shanghai, China), reverse transcription reaction was conducted, and total RNA was reverted to cDNA. The reaction conditions were: 70°C, 10 min; 5 min on ice; 42°C, 60 min; 95°C, 5 min; 0°C, 5 min. Then, the obtained cDNA was amplified. The fluorescence quantitative PCR reaction system was 25 μL, containing 500 ng cDNA template, 250 nmol/L reverse and forward primers, and 12.5 μL of 2× SYBR Green PCR Master (Toyobo, Shanghai, China) mixture. The primers sequence of each molecule is shown in Table 1. U6 served as the endogenous control of miR-590-5p, and GAPDH as the endogenous control of VEGF, MMP2, MMP9, MMP14, LOXL1-AS1 and KLF6. The 2−ΔΔCt value indicates the relative expression of genes. LOXL1-AS1, Forward primer, 5ʹ-AATTGCCCTCTTGGGCTTAT-3ʹ, Reverse primer, 5ʹ- GTCTCACCTTCCCATTCCAA-3ʹ; VEGF, Forward primer, 5ʹ- GCCCACTGAGGAGTCCAAC −3ʹ, Reverse primer, 5ʹ- TGGTTCCCGAAACGCTGAG −3ʹ; MMP2, Forward primer, 5ʹ- TGATCTTGACCAGAATACCATCGA-3ʹ, Reverse primer, 5ʹ- GGCTTGCGAGGGAAGAAGTT-3ʹ; MMP9, Forward primer, 5ʹ- CCTGGAGACCTGAGAACCAATC-3ʹ, Reverse primer, 5ʹ- CCACCCGAGTGTAACCATAGC-3ʹ; MMP14, Forward primer, 5ʹ- GAGCTCAGGGCAGTGGATAG-3ʹ, Reverse primer, 5ʹ-GGTAGCCCGGTTCTACCTTC-3ʹ; KLF6, Forward primer, 5ʹ- GGCCAAGTTTACCTCCGACC −3ʹ, Reverse primer, 5ʹ- TAAGGCTTTTCTCCTTCCCTGG −3ʹ;GAPDH, Forward primer, 5ʹ- CATGAGAAGTATGACAACAGCCT −3ʹ, Reverse primer, 5ʹ- AGTCCTTCCACGATACCAAAGT −3ʹ; miR-590-5p, Forward primer, 5ʹ- TGTCCGCCGAGCTTATTCATAAA −3ʹ, Reverse primer, 5ʹ- CAGTGCAGGGTCCGAGGT −3ʹ; U6, Forward primer, 5ʹ- TGCGGGTGCTCGCTTCGGCAGC −3ʹ, Reverse primer, 5ʹ-CCAGTGCAGGGTCCGAGGT-3ʹ.
2.5 Western blot
When cell treatment was completed, the HUVECs were collected and lysed them on ice using RIPA lysate (biyun tian biotechnology co., LTD.). The cells were fully lysed for 30 minutes and then centrifuged at 14,000 rpm for 15 min in a 4°C centrifuge. A total of 5 μg total proteins were taken and electrophorezed for 2 h at 100 V with SDS-PAGE. Next, the separated proteins were electrically transferred to polyvinylidene fluoride (PVDF) membranes. The following procedure was to seal the membranes with 5% skim milk powder at room temperature for 1 h. Then, they underwent TBST wash (3 times, 10 min each) and overnight incubation with primary anti-VEGFA (Abcam, ab52917, 1:1500), anti-MMP2 (Abcam, ab97779, 1:1000), anti-MMP9 (Abcam, ab38898, 1:1200), anti-MMP14 (Abcam, ab51074, 1:1000), anti-VEGFR2 (Abcam, ab134191, 1:1200), anti-PI3K (Abcam, ab86714, 1:2000), anti-p-PI3K (Abcam, ab182651, 1:1500), anti-p-Akt (Abcam, ab38449, 1:1500), anti-Akt (Abcam, ab18785, 1:1000), anti-p-eNOS (Abcam, ab76199, 1:1000), anti-eNOS (Abcam, ab5589, 1:1000), anti-KLF6 (Santa Cruz Biotechnology, sc-20,884, 1:1000) at 4°C. After washing the membranes at TBST, we incubated them with horseradish peroxidase (HRP)-labeled anti-rabbit secondary antibody (concentration 1:300) (room temperature, 1 h). Next, TBST was used to wash the membrane 3 times, 10 min each. Finally, X-ray development was performed using Pierce ECL Western blot Substrate kit (Thermo, Shanghai, China), and the gray values of each protein were analyzed by Image J.
2.6 Dual luciferase viability experiment
The sequences of LOXL1-AS1 and KLF6 were amplified and transferred to the luciferase gene downstream of pmirGLO plasmid (Promega, Fitchburg, WI, USA), respectively, to obtain pmir-LOXL1-AS1-WT and pmiR-klf6-WT. Meanwhile, the LOXL1-AS1 fragment (pMIR-LOXL1-AS1-MUT) and KLF6 fragment (pMIR-KLF6-MUT) containing the mutant target was designed according to the predicted binding sites of LOXL1-AS1, KLF6 and miR-590-5p based on LncBase v.2 (http://carolina.imis.athena-innovation.gr/diana_tools/web/index.php?r=lncbasev2%2Findex-predicted) and Targetscan (http: //carolina.imis.athena -innovation.gr/diana_tools/web/index.php?r = lncbasev2%2Findex-predicted). PMIR-LOXL1-AS1-WT, pMIR-LOXL1-AS1-MUT, pMIR-KLF6-WT, pMIR-KLF6-MUT and miR-590-5p mimics and miR-NC were co-transfected into HUVECs using lipofectamine® 3000. Forty-eight hours after the transfection, the relative luciferase activity was evaluated via the Dual-Glo luciferase assay kit (Promega, Fitchburg, WI, USA).
2.7 RNA immunoprecipitation
The Magna RIP™ RNA-Binding Protein Immunoprecipitation Kit (Millipore, Burlington, MA, USA) was employed to conduct RIP experiment. In short, HUVECs were harvested and lysed in RIP lysis buffer. Next, RNA was immunoprecipitated with antibody against Ago2 (Abcam, ab32381,1:500) or negative control IgG (EMD Millipore, cat. no. 12–371, Burlington, MA, USA). Following that, the RNA in the lysates were extracted by TRIzol, and PCR was used to measure the enrichments of LOXL1-AS1 and miR-590-5p.
2.8 Dual-color fluorescence in situ hybridization (FISH)
RNA FISH assay was used for determining the co-location of LOXL1-AS1 and miR-590-5p in HUVECs. The DNA oligo probes of LOXL1-AS1 (labeled with FAM) and miR-590-5p (labeled by Cy5) were purchased from GenePharma (Shanghai, China). HUVECs were cultured in 24-well plates (with 1 × 105 cells per well). The cells were fixed by 4% Paraformaldehyde (at room temperature for 15 min). Next, the cells were washed with PBS for 3 times (5 min each time) and blocked with 5% goat serum for 1 hour at room temperature. Followed by that, the cells were incubated with the LOXL1-AS1 and miR-509-5p probes overnight. The nuclei were stained by 4, 6-diamidino- 2-phenylindole (DAPI) (Beyotime, Shanghai, China). Finally, the fluorescence signal was observed using the Leica SP5 confocal microscope (Leica Microsystems, Mannheim, Germany).
2.9 EdU experiment
The viability of the cells was detected by the EdU method. After treatment, HUVECs was added with 200 μL EdU medium (50 μmol/L, Guangzhou RiboBio Co., LTD) and cultured 2 h in the incubation. Then, we discarded the complete medium and washed the cells with PBS for 3 times, added 4% paraformaldehyde and secured it at 4°C for 24 h. Subsequently, the fixation fluid was discarded, and the cells were rinse with PBS 3 times (5 minutes each). Next, these cells were sealed with 5% bovine serum albumin (BSA) (1 hour at room temperature), added with 100 μL 1× Apollo® staining solution in the dark and went through decolorized incubation on a shaking bed (30 min, room temperature). Afterward, we washed the cells three times with PBS (5 min each time) and stained the nuclei 5 min with DAPI (Beyotime, Shanghai, China). Finally, EdU positive cells were observed and photographed using an Olympus fluorescence microscope. Image J was taken to count EdU positive cells. The ratio of EdU positive cell number to the total cell number in the field at high magnification was randomly selected as the EdU positive rate. The mean values of the three replicates were taken to represent the proliferation capacity of tumor cells.
2.10 Cell scratch test
HUVECs were inoculated in a 6-well plate (5 × 106/ well). When HUVECs were fully laid out, a straight scratch was formed in the middle of the confluence monolith by marking vertically with the tips of sterile 200 μL tubes. Then, the wounded cell monolayer was gently washed 3 times with serum-free medium. Healing was done in a complete culture medium. At 0 h and 24 h after the scratch formation, the width of the scratch under the microscope was photographed and calculated. Cell migration was evaluated through percent closure determination. Each experiment was repeated three times with three measurements each.
2.11 Tube formation assay
The capability of HUVECs to form capillary tube-like structures was assessed by the Matrigel-based tube formation assay, as previously described [Citation28]. Briefly, a 24-well plate was polymerized by Matrigel (BD Biosciences, Bedford, MA) for 30 min at 37°C. HUVECs (2 × 104) were incubated in 200 μl conditioned medium (CM) for 12 h before image taking. The capillary tubes were quantified under a 100× bright-field microscope by measuring the total lengths of the completed tubule structure. Three independent experiments were required for each treatment.
2.12 Matrigel plug assay
Forty BALB/c-nude mice (male, 6-wk-old) were purchased from the Animal Laboratory of Zhengzhou University. Three mice were used for the implantation of Matrigel plugs as previously described [Citation28]. In brief, ice-cold Matrigel (BD Biosciences, San Jose, CA, USA) (200 μL per plug) was mixed with 50 μL PBS or Ox-LDL (5 μg/ml) and HUVEC transfected with pcDNA-LOXL1-AS1, si-LOXL1-AS1, or the corresponding negative controls (1 × 106 cells in 50 μL PBS) and then injected subcutaneously into the dorsal region of mice (each mouse received three plugs in total from the different groups). One week after implantation, Matrigel plugs were excised for hematoxylin-eosin staining and immunohistochemical staining. All experimental protocols were approved by the Animal Care and Experiment Committee of the First Affiliated Hospital of Zhengzhou University.
2.13 Immunohistochemical staining
The Matrigel plugs were embedded in paraffin. Then the sections were dewaxed (by xylene), hydrated (using gradient ethanol), dipped in citrate buffer (pH 6.0) and boiled in a pressure cooker at 121°C for 4 min for antigen retrieval. After being blocked by 5% v/ v normal goat serum, the sections were incubated with anti-CD31 (1:200; ab28364; Abcam) at 4°C overnight and stained with secondary antibody of anti-rabbit IgG goat IgG-HRP (Abcam). The immunohistochemical staining was photographed under an optical microscope. This experiment was repeated for 3 times and five rats in each group were used for testing.
2.14 Clinical specimen collection and processing
The blood specimen was collected from patients diagnosed with atherosclerosis and healthy donors in the First Affiliated Hospital of Zhengzhou University. A total of 15 clinical samples were obtained from atherosclerosis patients and 15 healthy donors. After removing the cells in the blood samples through low-speed centrifugation, and the serum was collected and stored at −80°C in a freezer. Then RT-PCR was used to detect LOXL1-AS1 and miR-590-5p in the serum. This study had been approved by the medical ethics committee of the First Affiliated Hospital of Zhengzhou University. All patients consented to be involved in the study and signed the consent form.
2.15 Data analysis
SPSS 17.0 statistical software was taken for data process. Measurement data were presented as mean ± variance (x ± s). One-way ANOVA and LSD tests were performed for comparison of mean differences between multiple groups, and t-test was employed for comparison of mean differences between two groups. The correlation between miR-590-5p and LOXL1-AS1 was analyzed by Pearson linear regression. All experiments were repeated at least for 3 times, and each time has three repeats. P < 0.05 was taken as statistically valuable. 0
3 Results
3.1 LOXL1-AS1 promoted Ox-LDL-mediated angiogenesis
HUVECs were treated with different concentrations of Ox-LDL (2.5–20 μg/ml) to explore the effect of LOXL1-AS1 on Ox-LDL-mediated angiogenesis. It turned out that Ox-LDL promoted LOXL1-AS1 expression in HUVECs in a dose- and time-dependent manner ( A-B). Then, the overexpression and knockdown of LOXL1-AS1 cell models were constructed in HUVECs ( C-D). The proliferation and cell viability of HUVECs were detected by CCK8 and EdU methods. The results showed that Ox-LDL (5 μg/ml) notably promoted cell proliferation and viability (compared with the control group) ( E-H). However, after overexpression of LOXL1-AS1, the proliferative capacity and cell viability were considerably increased (compared with the Ox-LDL group) ( E and G), while the downregulation of LOXL1-AS1 inhibited the cell proliferation and viability of HUVECs ( F and H). Next, we examined the mobility and tube formation ability of HUVECs. The results revealed that Ox-LDL (5 μg/ml) promoted HUVECs movement and angiogenesis, while LOXL1-AS1 upregulation promoted HUVECs’ movement and angiogenesis, and the knockdown of LOXL1-AS1 had the opposite effects (compared with the Ox-LDL group) ( I and J). Moreover, the Matrigel plug assay was performed to investigate the role of Ox-LDL and LOXL1-AS1 in regulating the angiogenesis of HUVECs in vivo. We found that HUVECs treated with Ox-LDL had enhanced angiogenesis and upregulated CD31 compared with the control group ( K-L). Transfection with LOXL1-AS1 overexpression plasmids markedly enhanced Ox-LDL-induced angiogenesis and CD31 expression (compared with Ox-LDL+vector group), while LOXL1-AS1 knockdown obviously reduced angiogenesis and CD31 expression (compared with Ox-LDL+si-NC group) ( K-L). Therefore, the above statistics manifested that LOXL1-AS1 is increased after the treatment of Ox-LDL, and upregulated LOXL1-AS1 promotes HUVECs’ proliferation, migration and angiogenesis.
Figure 1. LOXL1-AS1 promoted Ox-LDL-mediated angiogenesis. A: Different concentrations of Ox-LDL (2.5–20 μg/ml) were applied to treat HUVECs for 24 hours, and LOXL1-AS1 expression was detected via RT-PCR; B: Ox-LDL (5 μg/ml) was applied to HUVECs for different times (0–72 h), and RT-PCR was performed to measure the expression of LOXL1-AS1 in the HUVECs, ns p > 0.05, *** p < 0.001 vs. control group. N = 3; C-D: Overexpression (c) and low expression (d) of LOXL1-AS1 cell model were constructed in HUVECs; Ox-LDL (5 μg/ml) were applied to treat HUVECs with different level of LOXL1-AS1 for 24 hours. E-F: CCK8 was carried out to examine the proliferation of HUVECs; G-H: The cell viability of HUVECs was evaluated via EdU method; I: HUVECs’ migration was examined by cell scratch test; J. HUVECs’ angiogenic ability was detected via the tube formation assay. K-L: matrigel plug assay was used to evaluate the angiogenesis of HUVECs in vivo. the matrigel plugs were harvested at 1-wk post-implantation and then subjected to hematoxylin-eosin staining and immunohistochemical staining (for detecting CD31). *, **, *** represents p < 0.05, p < 0.01 and p < 0.001, respectively. N = 3
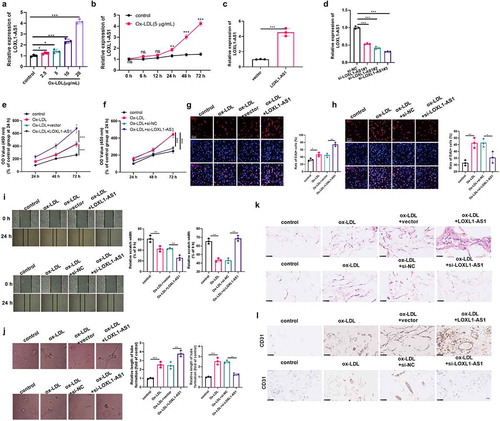
3.2 LOXL1 AS1 promoted the activation of the VEGF/VEGFR2/PI3K/Akt/eNOS pathway
To explore the underlying mechanism of LOXL1-AS1 in regulating the angiogenesis of vascular endothelial cell, we detected the expression of VEGF and matrix metalloproteinases (MMPs). The results illustrated that Ox-LDL (5 μg/ml) promoted the mRNA and protein expressions of VEGF, MMP2, MMP9, MMP14 and the activation of VEGF/VEGFR2/PI3K/Akt/eNOS pathway ( A-F). After the upregulation of LOXL1-AS1, the expressions of VEGF, MMP2, MMP9 and MMP14 were raised significantly, and the activation of the VEGF/VEGFR2/PI3K/Akt/eNOS pathway was more significant (compared with the Ox-LDL+vector group) ( A-B, E). On the contrary, after LOXL1-AS1 knockdown, the expressions of VEGF, MMP2, MMP9 and MMP14 in HUVECs were remarkably decreased, and the VEGF/VEGFR2/PI3K/Akt/eNOS pathway was notably inhibited (compared with the OxLDL+si-NC group) ( C-D, F). Thus, the up-regulation of LOXL1-AS1 promotes the activation of the Ox-LDL-mediated VEGF pathway, while the down-regulation of LOXL1-AS1 reverses the activation of the Ox-LDL-mediated VEGF pathway.
Figure 2. LOXL1-AS1 promoted the activation of VEGF/VEGFR2/PI3K/Akt/eNOS pathway. A-B: HUVECs were transfected with LOXL1-AS1 overexpression plasmid or negative vector, and cells were treated with Ox-LDL (5 μg/ml) for 24 hours. RT-PCR and western blot were used to detect the expressions of VEGF, MMP2, MMP9 and MMP14. C-D: HUVECs were transfected with si-LOXL1-AS1 or si-NC, and cells were treated with Ox-LDL (5 μg/ml). RT-PCR and western blot were carried out to detect the expressions of VEGF, MMP2, MMP9 and MMP14. E-F. western blot was taken to detect the activation of VEGF/VEGFR2/PI3K/Akt/eNOS pathway in HUVECs transfected with LOXL1-AS1 overexpression plasmids or si-LOXL1-AS1 or their negative controls. *, **, *** represents p < 0.05, p < 0.01 and p < 0.001, respectfully. N = 3
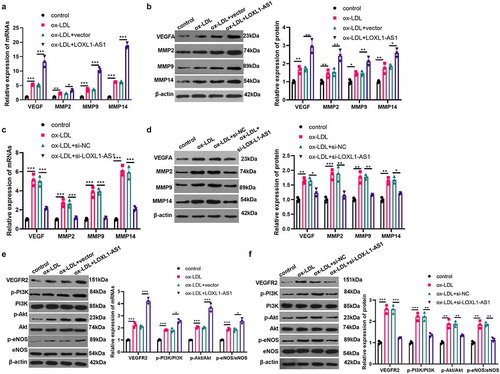
3.3 MiR-590-5p is a competitive endogenous miRNA of LOXL1-AS1
The potential miRNAs regulated by LOXL1-AS1 were searched through LncBase v.2 and Starbase databases. A total of 19 miRNAs (including hsa-miR-3622b-5p, hsa-miR-4735-3p, hsa-miR-1224-5p, hsa-miR-382-3p, hsa-miR-374a-5p hsa-miR-28-5p, hsa-miR-552-5p, hsa-miR-708-5p, hsa-miR-2278, hsa-miR-4428, hsa-miR-5590-3p, hsa-miR-423-5p, hsa-miR-5094, hsa-miR-590-5p, hsa-miR-374b-5p hsa-miR-3126-5p, hsa-miR-1913, hsa-miR-6875-5p, hsa-miR-3139) were shared by the LncBase V2 and Starbase v2.0 through Venny diagram analysis (). Interestingly, miR-590-5p contains binding sites that pair with LOXL1-AS1 (). To explore the targeting relationship between miR-590-5p and LOXL1-AS1, the dual luciferase activity experiment and the RIP experiment were carried out. It was found that miR-590-5p inhibited luciferase activity in cells transfected with luciferase reporter gene vector LOXL1-AS1-WT, but had no significant effect on luciferase activity in cells transfected with LOXL1-AS1-MUT vector (). Meanwhile, in the RIP experiment, increased LOXL1-AS1 and miR-590-5p were enriched in the anti-Ago2 group (vs. anti-IgG group, ). Further, miR-590-5p expression after selective regulation of LOXL1-AS1 was detected. The outcomes revealed that LOXL1-AS1 upregulation inhibited miR-590-5p expression, while down-regulation of LOXL1-AS1 promoted its expression (). The RNA FISH assay showed that both of LOXL1-AS1 and miR-590-5p were mainly located in the cytoplasm of HUVECs, and they were partly co-located (). Then, we measured the expression level of miR-590-5p after Ox-LDL (2.5–20 μg/ml) treatment. The results illustrated that Ox-LDL dose-dependently suppressed miR-590-5p expression (). Meanwhile, with the extension of Ox-LDL action time, the expression of miR-590-5p was notably decreased (), which was contrary to the trend of LOXL1-AS1 under the action of Ox-LDL. Collectively, LOXL1-AS1 was confirmed to target miR-590-5p.
Figure 3. MiR-590-5p is a competitive endogenous miRNA for LOXL1-AS1. A-B: through LncBase v.2 and starbase databases, we searched for potential miRNAs regulated by LOXL1-AS1.venny’s diagram was used to analyzed the shared miRNAs in the two databases. among the 19 shared miRNAs, miR-590-5p contains sites that pair with LOXL1-AS1. C: the dual luciferase activity experiment was performed to verify the targeting relationship between miR-590-5p and LOXL1-AS1. D: RT-PCR was performed to detect the enrichment level of LOXL1-AS1 and miR-590-5p in the lysate of RIP experiment. E: RT-PCR was applied to detect the expression of miR-590-5p after selective regulation of LOXL1-AS1. ns, **, *** represents p > 0.05, p < 0.01 and p < 0.001, respectfully. F: RNA FISH assay was used for determining the cellular localization of LOXL1-AS1 (red) and miR-590-5p (green) in HUVECs. G. different concentrations of Ox-LDL (2.5–20 μg/ml) were applied to HUVECs for 24 hours, and miR-590-5p expression was detected via RT-PCR; H: Ox-LDL (5 μg/ml) was applied to HUVECs for different time (0–72 h), miR-590-5p expression was measured by RT-PCR, ns p > 0.05, ** * p < 0.001 vs. control group
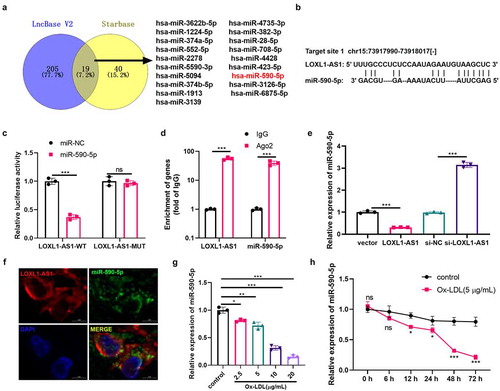
3.4 MiR-590-5p inhibited Ox-LDL-mediated angiogenesis and was inhibited by LOXL1-AS1
Due to the expression characteristics of miR-590-5p and LOXL1-AS1 after Ox-LDL action, we were very curious about their roles in Ox-LDL-mediated angiogenesis. We transfected HUVECs with miR-590-5p mimics and/or LOXL1-AS1 overexpressed plasmids. The results revealed that after transfection with miR-590-5p, the level of miR-590-5p in HUVECs was upregulated, while miR-590-5p expression was down-regulated after the transfection of LOXL1-AS1 overexpressed plasmids (). Next, we examined the proliferation, viability, migration, and angiogenesis of HUVECs with Ox-LDL (5 μg/ml) treatment. Compared with the Ox-LDL group, the proliferation, viability, migration and tube formation ability of HUVECs were decreased after the overexpression of miR-590-5p ()). However, after supplementing the LOXL1-AS1 overexpressed plasmid, the inhibitory effects of miR-590-5p on the proliferation, viability, migration and tube formation ability of HUVECs were reduced ()). Then, we tested the expressions of VEGF, MMP2, MMP9, MMP14 and the activation of the VEGF/VEGFR2/PI3K/Akt/eNOS pathway. The results illustrated that miR-590-5p upregulation inhibited the mRNA and protein expressions of VEGF, MMP2, MMP9, MMP14 and the VEGF/VEGFR2/PI3K/Akt/eNOS pathway activation, and overexpression of LOXL1-AS1 reversed this effect ()). Therefore, the upregulation of miR-590-5p attenuated Ox-LDL-mediated angiogenesis, while the upregulation of LOXL1-AS1 inhibited the miR-590-5p-mediated effects.
Figure 4. MiR-590-5p inhibited Ox-LDL-mediated angiogenesis and was suppressed by LOXL1-AS1. A: we transfected miR-590-5p mimics and LOXL1-AS1 overexpression plasmids into HUVECs and detected the expression of miR-590-5p by RT-PCR. HUVECs were transfected with miR-590-5p mimics and LOXL1-AS1 overexpression plasmids, and cells were treated with Ox-LDL (5 μg/ml) for 24 hours; B: CCK8 was used to examine the proliferation of HUVECs; C: the cell viability of HUVECs was detected by EdU method; D: the migration of HUVECs was detected by cell scratch test; E. the tube formation ability of HUVECs was detected by tube formation assay. *, **, *** represents p < 0.05, p < 0.01 and p < 0.001, respectively. N = 3; F-G: The expressions of VEGF, MMP2, MMP9, MMP14 were detected via RT-PCR and western blot, respectively (f-g), and the activation of VEGFR2/PI3K/Akt/eNOS pathway was detected by western blot (h). *, **, *** represents p < 0.05, p < 0.01 and p < 0.001, respectively. N = 3
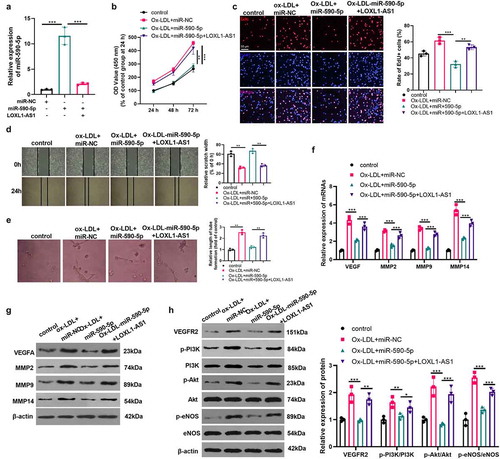
3.5 KLF6 is a target of miR-590-5p and is upregulated by Ox-LDL
By browsing Targetscan, miRanda and Pictar databases, we analyzed the downstream regulatory genes of miR-590-5p. Interestingly, the 3ʹUTR of KLF6 mRNA had a base site binding to miR-590-5p (). Subsequently, we confirmed that miR-590-5p targeted the 3ʹUTRof KLF6 via dual luciferase activity assay (). Next, we examined the regulatory effects of LOXL1-AS1 and miR-590-5p on KLF6. The results showed that both of Ox-LDL and LOXL1-AS1 overexpression increased KLF6 mRNA and protein expression. And LOXL1-AS1 had more significant effects in promoting KLF6 compared with Ox-LDL (). Moreover, the upregulation of miR-590-5p inhibited KLF6 expression, which was reversed by LOXL1-AS1 overexpression ()). The KLF6 mRNA and protein levels in HUVECs treated with Ox-LDL (2.5–20 μg/ml) were determined. As the data showed, Ox-LDL upregulated the mRNA and protein levels of KLF6, with dose-dependent and time-dependent effects ()). The above statistics indicated that the LOXL1-AS1/miR-590-5p axis regulates Ox-LDL-mediated angiogenesis potentially via regulating KLF6.
Figure 5. KLF6 is the target of miR-590-5p and was upregulated by Ox-LDL. A-B. through the targetscan, miranda and pictar databases, we analyzed the downstream regulatory genes of miR-590-5p. among the 124 potential gens, the 3ʹUTR of KLF6 mRNA has a base site that binds to miR-590-5p; C: the dual luciferase activity experiment was used to confirm the targeting relationship between miR-590-5p and KLF6; D-E: we performed RT-PCR (d) and western blot (e) to measure the expression of KLF6 mRNA and protein in HUVECs treated with Ox-LDL (5 μg/ml) or with selective regulation of LOXL1-AS1; F-G: RT-PCR (f) and western blot (g) were applied to examine the regulation effect of LOXL1-AS1 and miR-590-5p on KLF6; H-I: different concentrations of Ox-LDL (2.5–20 μg/ml) were applied to HUVECs for 24 hours, and the expression of KLF6 was evaluated via RT-PCR (h) and western blot (i). Ns, *, **, *** represents p > 0.05, p < 0.05, p < 0.01 and p < 0.001, respectively. N = 3; J-K: Ox-LDL (5 μg/ml) was applied to HUVECs for different time (0–72 h), RT- PCR (j) and western blot (k) were taken to examine the expression of KLF6, ns, *, **, *** represents p > 0.05, p < 0.05, p < 0.01 and p < 0.001 vs. 0 h group, respectively. N = 3
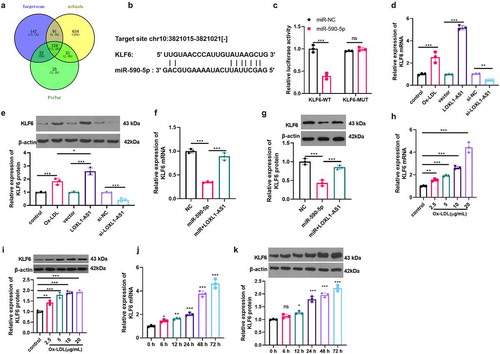
3.6 KLF6 promoted Ox-LDL-mediated angiogenesis
The data has confirmed that KLF6 may make a great contribution to the regulation of Ox-LDL-mediated angiogenesis. Hence, we constructed a cell model of KLF6 overexpression (). Next, we examined the proliferation, activity, migration, and angiogenesis of HUVECs induced by Ox-LDL (5 μg/ml). The results presented that the proliferation, viability, migration and tube formation ability of HUVECs were considerably enhanced after overexpression of KLF6 (compared with Ox-LDL+vector group) () (compared with the Ox-LDL group). Then, we tested the mRNA and protein expressions of VEGF, MMP2, MMP9, MMP and the activation levels of VEGF/VEGFR2/PI3K/Akt/eNOS pathway. The results showed that forced upregulation of KLF6 significantly promoted VEGF, MMP2, MMP9, MMP14 expressions and the VEGF/VEGFR2/PI3K/Akt/eNOS pathway activation ()). Therefore, the up-regulation of KLF6 promotes Ox-LDL-mediated angiogenesis.
Figure 6. KLF6 promoted Ox-LDL-mediated angiogenesis. A. HUVECs were transfected with KLF6 overexpression plasmid, and western blot was used to detect the expression of KLF6. Ox-LDL (5 μg/ml) were used to treat KLF6 over-expressed cells for 24 hours; B: CCK8 was used to detect the proliferation of HUVECs; C: the cell viability of HUVECs was measured by EdU method; D: the migration of HUVECs was detected via cell scratch test; E: the blood vessel formation ability of HUVECs was detected by tube formation assay. *, **, *** represents p < 0.05, p < 0.01 and p < 0.001, respectively. N = 3; F-G: the expressions of VEGF, MMP2, MMP9, MMP14 were detected via RT-PCR (f) and western blot (g). H: the activation of VEGFR2/PI3K/Akt/eNOS pathway was measured by western blot (h). *, **, *** represents p < 0.05, p < 0.01 and p < 0.001, respectively. N = 3
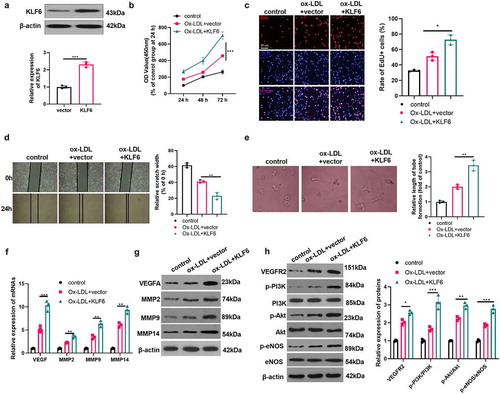
3.7 LOXL1-AS1 was upregulated in the serum of atherosclerosis patients
To further investigate the functions of LOXL1-AS1 and miR-590-5p in atherosclerosis, we collected 15 serum samples of atherosclerosis patients as well as 15 serum samples of healthy donors. Then RT-PCR was performed to detect LOXL1-AS1 and miR-590-5p in the clinical samples. It was found that LOXL1-AS1 was upregulated in AS patients () while miR-590-5p was downregulated in the serum of AS patients compared with healthy donors (). In addition, the linear regression analysis showed that LOXL1-AS1 and miR-590-5p had a negative correlation in the serum of atherosclerosis patients (). Therefore, we believed that both of LOXL1-AS1 and miR-590-5p exert a role in atherosclerosis progression.
Figure 7. LOXL1-AS1 was upregulated in the serum of atherosclerosis patients 15 serum samples of atherosclerosis patients as well as 15 serum samples of healthy donors were collected. RT-PCR was performed to detect LOXL1-AS1 and miR-590-5p in the clinical samples (a-b). C: linear regression analysis was used to analyze the correlation of LOXL1-AS1 and miR-590-5p in the serum of atherosclerosis patients. N = 3
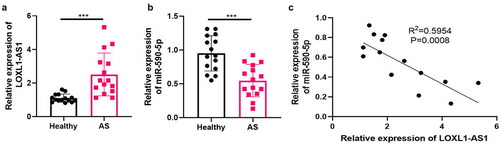
4 Discussion
This study explored the effect of the LOXL1-AS1/miR-590-5p/KLF6 axis in Ox-LDL-mediated abnormal proliferation, migration and angiogenesis. Our results demonstrated that Ox-LDL remarkably upregulated the expression of LOXL1-AS1, which promoted Ox-LDL-mediated angiogenesis by inhibiting miR-590-5p and upregulating KLF6.
As an important barrier to the inner wall of blood vessels, vascular endothelial cells exert a great effect on protecting blood vessels [Citation29]. In atherosclerotic lesions, the expression of angiogenic factors (VEGF, PDGF, TGFβ), the generation of lipid mediators (lysophosphatidic acid, S1P, prostaglandins) and the formation of oxidized lipids were stimulated by local oxidative stress and hypoxia caused by intimal hyperplasia [Citation30,Citation31]. In the atherosclerosis patients, dysregulated Ox-LDL mediates vascular dysfunction, and on the surface of vascular endothelial cells, several scavenger receptors that mediate the cytotoxic effects of Ox-LDL are expressed [Citation32]. Unregulated Ox-LDL caused dual roles on the proliferation, migration and angiogenesis of vascular cells. On the one hand, Ox-LDL leads to proinflammatory responses and accelerates cell migration and proliferation. On the other hand, higher concentration of Ox-LDL represses cell growth and induces apoptosis [Citation33]. These unbalanced endothelial cell migration and proliferation lead to the formation of neocapillaries issued from adventitial vasa vasorum and invading the intima [Citation34]. In the initial stage, the neovascularization provides blood cells, nutrients and oxygen that promote the growth of the atherosclerotic plaque. However, these leaky fragile neocapillaries increase the risk of plaque destabilization as they promote iron deposition and intraplaque hemorrhages [Citation35–38]. In this study, we found that Ox-LDL (5 μg/ml) markedly promoted the proliferation, migration and tube formation ability.
Lectin-like oxidation of low-density lipoprotein receptor 1 (LOX-1) is the main Ox-LDL receptor in endothelial cells and is expressed in macrophages and smooth muscle cells. LOX-1 is upregulated after exposure to several pro-inflammatory and pro-atherogenic stimuli, and aggravates the injury of vascular endothelial cells by activating multiple inflammatory response signaling pathways [Citation39,Citation40]. Additionally, there are researches showing that Ox-LDL mediates angiogenesis, which is a complex process that involves the cell effort, migration, basement be degradation, and neo-vessel organization and maturation [Citation41]. This differential effect of Ox-LDL is most likely due to different concentrations of Ox-LDL. As shown in previous studies, low concentrations of ox-LDL accelerate angiogenesis in human endothelial cells, thereby making a contribution to plaque vulnerability and intravascular thrombosis [Citation42,Citation43]. Here, we found that under a relatively low concentration of Ox-LDL (5 μg/ml) treatment, the proliferation, viability, migration and angiogenesis of HUVECs were notably promoted, which further confirmed the above conclusion.
LncRNA is one of the research hotspots in recent years, and it plays an important role in regulating cell proliferation, migration and apoptosis [Citation44]. What is worth noting is that lncRNA takes part in Ox-LDL-mediated dysfunction of endothelial cells, macrophages, and vascular smooth muscle cells. Taking lncRNA NORAD as an example, its downregulation exacerbated Ox-LDL-mediated endothelial cell apoptosis, reactive oxygen species, malondialdehyde, and p-IKBα expression levels and NF-κB nuclear translocation [Citation45]. LncRNA MALAT1 can maintain autophagic levels in Ox-LDL-acting macrophages by regulating the SIRT1/MAPK/NF-κB Pathway [Citation46]. Besides, Ox-LDL dose-dependently promotes the proliferation of vascular smooth muscle cells, and regulates vascular smooth muscle cell proliferation and colony formation ability by raising lncRNA urothelial cancer-associated 1 (UCA1) [Citation47]. As a lncRNA, LOXL1-AS1 has been proven to promote the proliferation and metastasis of tumor cells [Citation48,Citation49]. Therefore, we were curious about the function of LOXL1-AS1 in Ox-LDL-mediated vascular endothelial cells. Our results manifested that low concentration of Ox-LDL promoted the expression of LOXL1-AS1, while LOXL1-AS1 accelerated Ox-LDL-mediated proliferation, migration and angiogenesis of vascular endothelial cells. This fact revealed the potential effect of LOXL1-AS1 on the diagnosis and treatment of AS.
Abundant miRNAs have previously been implicated in the regulation of Ox-LDL in vascular endothelial cell injury and angiogenesis. For instance, up-regulation of miR-140-5p can inhibit Ox-LDL-mediated vascular endothelial cell apoptosis and oxidative stress by targeting the toll like receptor 4 (TLR4) [Citation50]. Similarly, miR-381 attenuates the damage of Ox-LDL-mediated HUVECs inflammation by targeting CXCR4 [Citation51]. Besides, Ox-LDL-stimulated human monocyte-derived macrophages (HMDMs) -derived extracellular vesicles (EVs) inhibited the proliferation, migration, and angiogenesis abilities of human coronary artery vascular endothelial cells (HCAECs) in vitro. Further exploration on the mechanism discovered that EV-shuttled miR-4306 upregulates the Akt/nuclear factor kappa B signaling pathway [Citation52]. MiR-590-5p is a multifunctional miRNA that plays a significant role in the regulation of tumor progression, inflammatory response, central nervous system damage, and angiogenesis [Citation21–24]. Moreover, previous studies have found that lncRNA TUG1, as the upstream modulator of miR-590-5p, inhibits the expression of miR-590-5p and promotes the proliferation and migration of ASMCs [Citation53]. Interestingly, in the present study, we found that miR-590-5p was considerably inhibited by Ox-LDL as a ceRNA molecule of LOXL1-AS1. We further explored the effect of miR-590-5p on Ox-LDL-mediated HUVECs. The results illustrated that miR-590-5p upregulation significantly attenuated Ox-LDL-mediated proliferation, migration, and angiogenesis of HUVECs. Meanwhile, overexpression of LOXL1-AS1 limited the expression of miR-590-5p and the effect of miR-590-5p. Therefore, under the action of Ox-LDL, upregulated LOXL1-AS1 promotes Ox-LDL-mediated angiogenesis by inhibiting miR-590-5p.
Recently, accumulating evidences have suggested that KLFs play important roles in vascular wall biology, which further affect vascular diseases by in vitro, in vivo, and genetic epidemiology studies. Specifically, multiple functional aspects are regulated by KLFs through modulating various signaling pathways [Citation54]. Those functions include cell growth, differentiation, activation, development, angiogenesis, inflammation and so on [Citation55,Citation56]. As one of the vital members of KLFs, KLF6 also exerts a key regulator of angiogenesis and vascular remodeling. For instance, during the process of vascular injury, KLF6 expression is elevated and it translocates into the nucleus to specifically enhance the transcriptional activity of endoglin and activin receptor-like kinase 1 (ALK1) [Citation57]. Additionally, KLF6 also regulates a pool of genes involved in motility and invasion during vascular remodeling and angiogenesis including collagen a1, E-cadherin, MMP9, MMP14 and VEGF [Citation58,Citation59]. Here, our data illustrated that low concentration of Ox-LDL promoted the expression of KLF6, while overexpression of KLF6 promoted Ox-LDL-mediated HUVECs proliferation, migration and angiogenesis, as well as the expressions of VEGF, MMP2, MMP9 and MMP14, which further verified the learn-promoting tubular effect of KLF6. Interestingly, we found that miR-590-5p targeted the 3ʹUTR end of KLF6 and inhibited the expression of KLF6, while LOXL1-AS1 promoted the expression of KLF6. Therefore, the LOXL1-AS1/miR-590-5p axis can promote Ox-LDL-mediated angiogenesis by upregulation of KLF6.
VEGF, as a classical cytokine, can further promote angiogenesis by activating the downstream PI3K/Akt/eNOS through VEGFR2. This effect is widely recognized in both tumor and non-tumor diseases [Citation60,Citation61]. Targeting the regulation of VEGFR2/PI3K/Akt/eNOS has a significant therapeutic effect on angiogenesis in AS. For example, Triptolide inhibits the angiogenesis of microvascular endothelial cells through upregulating miR-92-a, which then targets ITGA5 mediated VEGF signaling [Citation62]. In this study, we found that Ox-LDL promoted the activation of VEGF signal and its downstream VEGFR2/PI3K/Akt/eNOS pathway, and the up-regulation of LOXL1-AS1 promoted the effect of Ox-LDL, while miR-590-5p had an opposite effect. In addition, KLF6 was found to promote activation of the VEGFR2/PI3K/Akt/eNOS pathway. In connection with the promoting effect of KLF6 on VEGF, we hypothesized that KLF6 mediated by the LOXL1-AS1/miR-590-5p axis could promote the activation of VEGFR2/PI3K/Akt/eNOS pathway by upregulating VEGF.
Collectively, this study revealed a new regulatory pathway, namely the effect of the LOXL1-AS1/miR-590-5p/KLF6 axis in low-concentration Ox-LDL-mediated angiogenesis. Under the action of Ox-LDL, the upregulated LOXL1-AS1 inhibited the expression of miR-590-5p, leading to the up-regulation of KLF6 and the activation of VEGFR2/PI3K/Akt/eNOS pathway. However, there are still some problems to be solved in the following research: 1) how KLF6 upregulates the expression of VEGFR; 2) under the action of high concentration of Ox-LDL, whether the effect of LOXL1-AS1 /miR-590-5p/KLF6 axis remains unchanged; 3) the effect of the LOXL1-AS1 /miR-590-5p/KLF6 axis in the atherosclerosis animal model remains to be explored. In conclusion, this study explored the molecular mechanism of vascular endothelial cell angiogenesis during atherosclerosis, which is expected to provide a new theoretical basis for the diagnosis and treatment of atherosclerosis patients.
4 HIGHTS
1) LncRNA LOXL1-AS1 were upregulated in Ox-LDL induced HUVECs and atherosclerosis patients;
2) LOXL1-AS1 overexpression promoted Ox-LDL mediated angiogenesis both in vivo and in vitro;
3) LOXL1-AS1 works as a competitive endogenous RNA (ceRNA) by sponging miR-590-5p;
4) LOXL1-AS1 promoted Ox-LDL induced angiogenesis via modulating miR-590-5p targeted KLF6/VEGF signaling pathway.
Authors’ contributions
Conceived and designed the experiments: Yajun Lian;
Performed the experiments: Xuan Cheng;
Statistical analysis: Zhiwei Liu;
Wrote the paper: Xuan Cheng.
All authors read and approved the final manuscript.
Ethical approval
Our study was approved by the Ethics Review Board of the First Affiliated Hospital of Zhengzhou University.
Disclosure statement
No potential conflict of interest was reported by the author(s).
Data availability statement for Basic Data Sharing Policy
The data sets used and analyzed during the current study are available from the corresponding author on reasonable request. http://carolina.imis.athena-innovation.gr/diana_tools/web/index.php?r=lncbasev2%2Findex-predicted
Additional information
Funding
References
- Schaftenaar F, Frodermann V, Kuiper J, et al. Atherosclerosis: the interplay between lipids and immune cells. Curr Opin Lipidol. 2016;27(3):209–215.
- Zehr KR, Walker MK. Omega-3 polyunsaturated fatty acids improve endothelial function in humans at risk for atherosclerosis: a review. Prostaglandins Other Lipid Mediat. 2018;134:131–140.
- Ishigaki Y, Katagiri H, Gao J, et al. Impact of plasma oxidized low-density lipoprotein removal on atherosclerosis. Circulation. 2008;118(1):75–83.
- Mitra S, Deshmukh A, Sachdeva R, et al. Oxidized low-density lipoprotein and atherosclerosis implications in antioxidant therapy. Am J Med Sci. 2011;342(2):135–142.
- Gimbrone MA Jr, García-Cardeña G. Endothelial Cell Dysfunction and the Pathobiology of Atherosclerosis. Circ Res. 2016;118(4):620–636.
- Gao F, Chen J, Zhu H. A potential strategy for treating atherosclerosis: improving endothelial function via AMP-activated protein kinase. Sci China Life Sci. 2018;61(9):1024–1029.
- Ferrè F, Colantoni A, Helmer-Citterich M. Revealing protein-lncRNA interaction. Brief Bioinform. 2016;17(1):106–116.
- Kopp F, Mendell JT, Classification F. Experimental Dissection of Long Noncoding RNAs. Cell. 2018;172(3):393–407.
- Peng WX, Koirala P, Mo YY. LncRNA-mediated regulation of cell signaling in cancer. Oncogene. 2017;36(41):5661–5667.
- Marques-Rocha JL, Samblas M, Milagro FI, et al. Noncoding RNAs, cytokines, and inflammation-related diseases. FASEB J. 2015;29(9):3595–3611.
- Wang S, Yu W, Chen J, et al. LncRNA MALAT1 sponges miR-203 to promote inflammation in myocardial ischemia-reperfusion injury. Int J Cardiol. 2018;268:245.
- Pan JX. LncRNA H19 promotes atherosclerosis by regulating MAPK and NF-kB signaling pathway. Eur Rev Med Pharmacol Sci. 2017;21(2):322–328.
- Chen L, Yang W, Guo Y, et al. Exosomal lncRNA GAS5 regulates the apoptosis of macrophages and vascular endothelial cells in atherosclerosis. PLoS One. 2017;12(9):e0185406.
- Hauser MA, Aboobakar IF, Liu Y, et al. Genetic variants and cellular stressors associated with exfoliation syndrome modulate promoter activity of a lncRNA within the LOXL1 locus. Hum Mol Genet. 2015;24(22):6552–6563.
- Xue F, Xu YH, Shen CC, et al. Non-coding RNA LOXL1-AS1 exhibits oncogenic activity in ovarian cancer via regulation of miR-18b-5p/VMA21 axis. Biomed Pharmacother. 2020;125:109568.
- Yang X, Xing G, Liu S, et al. LncRNA LOXL1-AS1 promotes endometrial cancer progression by sponging miR-28-5p to upregulate RAP1B expression. Biomed Pharmacother. 2020;125:109839.
- Li M, Cai O, Tan S. LOXL1-AS1 drives the progression of gastric cancer via regulating miR-142-5p/PIK3CA Axis. Onco Targets Ther. 2019;12:11345–11357.
- Zhang L, Wan Q, Zhou H. Targeted-regulating of miR-515-5p by LncRNA LOXL1-AS1 on the proliferation and migration of trophoblast cells [published online ahead of print, 2020 Dec 2]. Exp Mol Pathol. 2020;118:104588.[19] Zhang L, Xie H, Li S. LncRNA LOXL1-AS1 controls osteogenic and adipocytic differentiation of bone marrow mesenchymal stem cells in postmenopausal osteoporosis through regulating the miR-196a-5p/Hmga2 axis. J Bone Miner Metab. 2020;38(6):794–805.
- Catalanotto C, Cogoni C, Zardo G. MicroRNA in control of gene expression: an overview of nuclear functions. Int J Mol Sci. 2016;17(10):1712.
- Yin J, Hou X, Yang S. microRNA-338-3p promotes ox-LDL-induced endothelial cell injury through targeting BAMBI and activating TGF-β/Smad pathway. J Cell Physiol. 2019;234(7):11577–11586.
- Jia G, Tang Y, Deng G, et al. miR-590-5p promotes liver cancer growth and chemotherapy resistance through directly targeting FOXO1. Am J Transl Res. 2019;11(4):2181–2193.
- Yu M, Luo Y, Cong Z, et al. MicroRNA-590-5p inhibits intestinal inflammation by targeting YAP. J Crohns Colitis. 2018;12(8):993–1004.
- Guo Q, Su H, He JB, et al. MiR-590-5p alleviates intracerebral hemorrhage-induced brain injury through targeting Peli1 gene expression. Biochem Biophys Res Commun. 2018;504(1):61–67.
- Dai Y, Zhang Z, Cao Y, et al. MiR-590-5p Inhibits Oxidized- LDL Induced Angiogenesis by Targeting LOX-1. Sci Rep. 2016;6(1):22607.
- DiFeo A, Martignetti JA, Narla G. The role of KLF6 and its splice variants in cancer therapy. Drug Resist Updat. 2009;12(1–2):1–7.
- Kim GD, Ng HP, Patel N, et al. Kruppel-like factor 6 and miR-223 signaling axis regulates macrophage-mediated inflammation. FASEB J. 2019;33(10):10902–10915.
- Goodman WA, Omenetti S, Date D, et al. KLF6 contributes to myeloid cell plasticity in the pathogenesis of intestinal inflammation. Mucosal Immunol. 2016;9(5):1250–1262.
- Yu F, Witman N, Yan D, et al. Human adipose-derived stem cells enriched with VEGF-modified mRNA promote angiogenesis and long-term graft survival in a fat graft transplantation model. Stem Cell Res Ther. 2020;11(1):490.
- Naito H, Iba T, Takakura N. Mechanisms of new blood-vessel formation and proliferative heterogeneity of endothelial cells. Int Immunol. 2020;32(5):295–305.
- Carmeliet P, Jain RK. Molecular mechanisms and clinical applications of angiogenesis. Nature. 2011;473(7347):298–307.
- Kwon TG, Lerman LO, Lerman A. The vasa vasorum in atherosclerosis: the vessel within the vascular wall. J Am Coll Cardiol. 2015;65(23):2478–2480.
- Tang Y, Zhao J, Shen L, et al. ox-LDL induces endothelial dysfunction by promoting Arp2/3 complex expression. Biochem Biophys Res Commun. 2016;475(2):182–188.
- Nègre-Salvayre A, Augé N, Camaré C, et al. Dual signaling evoked by oxidized LDLs in vascular cells. Free Radic Biol Med. 2017;106:118–133.
- Chen PY, Qin L, Li G, et al. Endothelial TGF-β signalling drives vascular inflammation and atherosclerosis. Nat Metab. 2019;1(9):912–926.
- Moreno PR, Purushothaman KR, Sirol M, et al. Neovascularization in human atherosclerosis. Circulation. 2006;113(18):2245–2252.
- Subbotin VM. Neovascularization of coronary tunica intima (DIT) is the cause of coronary atherosclerosis. Lipoproteins invade coronary intima via neovascularization from adventitial vasa vasorum, but not from the arterial lumen: a hypothesis. Theor Biol Med Model. 2012;9(1):11.
- Ho-Tin-Noé B, Michel JB. Initiation of angiogenesis in atherosclerosis: smooth muscle cells as mediators of the angiogenic response to atheroma formation. Trends Cardiovasc Med. 2011;21(7):183–187.
- Gao L, Chen Q, Zhou X, et al. The role of hypoxia-inducible factor 1 in atherosclerosis. J Clin Pathol. 2012;65(10):872–876.
- Kattoor AJ, Kanuri SH, Mehta JL. Role of Ox-LDL and LOX-1 in Atherogenesis. Curr Med Chem. 2019;26(9):1693–1700.
- Singh S, Gautam AS. Upregulated LOX-1 Receptor: key player of the pathogenesis of atherosclerosis. Curr Atheroscler Rep. 2019;21(10):38.
- Yuan R, Shi W, Xin Q, et al. Tetramethylpyrazine and Paeoniflorin Inhibit Oxidized LDL-Induced Angiogenesis in Human Umbilical Vein Endothelial Cells via VEGF and Notch Pathways. Evid Based Complement Alternat Med. 2018;2018:3082507.
- Qiu J, Peng Q, Zheng Y, et al. OxLDL stimulates Id1 nucleocytoplasmic shuttling in endothelial cell angiogenesis via PI3K Pathway. Biochimica Et Biophysica Acta (BBA) - Mol Cell Biol Lipids. 2012;1821(10):1361–1369.
- Wang L, Li G, Chen Q, et al. Octanoylated ghrelin attenuates angiogenesis induced by oxLDL in human coronary artery endothelial cells via the GHSR1a-mediated NF-κB pathway. Metab Clin Exp. 2015;64(10):1262–1271.
- Zhao YH, Liu YL, Fei KL, et al. Long non-coding RNA HOTAIR modulates the progression of preeclampsia through inhibiting miR-106 in an EZH2-dependent manner [published online ahead of print, 2020 Apr 19]. Life Sci. 2020;253:117668.
- Bian W, Jing X, Yang Z, et al. Downregulation of LncRNA NORAD promotes Ox-LDL-induced vascular endothelial cell injury and atherosclerosis. Aging (Albany NY). 2020;12(7):6385–6400.
- Yang J, Lin X, Wang LA, et al. LncRNA MALAT1 Enhances ox-LDL-Induced Autophagy through the SIRT1/MAPK/NF-κB Pathway in Macrophages [published online ahead of print, 2020 Mar 17]. Curr Vasc Pharmacol. 2020;10:2174/1570161118666200317153124.
- Zheng HK, Dong MH, Liu GH, et al. Dysregulation of the Urothelial Cancer Associated 1 Long Noncoding RNA Promotes Proliferation of Vascular Smooth Muscle Cells by Modulating Expression of P27KIP1/CDK2. Genet Test Mol Biomarkers. 2020;24(4):204–211.
- Xie N, Fei X, Liu S, et al. LOXL1-AS1 promotes invasion and proliferation of non-small-cell lung cancer through targeting miR-324-3p. Am J Transl Res. 2019;11(10):6403–6412.
- Gao R, Zhang R, Zhang C, et al. LncRNA LOXL1-AS1 Promotes the Proliferation and Metastasis of Medulloblastoma by Activating the PI3K/AKT Pathway. Anal Cell Pathol (Amst). 2018;2018:9275685.
- Liu H, Mao Z, Zhu J, et al. MiR-140-5p inhibits oxidized low-density lipoprotein-induced oxidative stress and cell apoptosis via targeting toll-like receptor 4 [published online ahead of print, 2020 Mar 12]. Gene Ther. 2020. DOI:https://doi.org/10.1038/s41434-020-0139-7.
- Li Y, Huang J, Yan H, et al. Protective effect of microRNA-381 against inflammatory damage of endothelial cells during coronary heart disease by targeting CXCR4. Mol Med Rep. 2020;21(3):1439–1448.
- Yang Y, Luo H, Zhou C, et al. Regulation of capillary tubules and lipid formation in vascular endothelial cells and macrophages via extracellular vesicle-mediated microRNA-4306 transfer. J Int Med Res. 2019;47(1):453–469.
- Lin J, Feng X, Zhang J, et al. Long noncoding RNA TUG1 promotes airway smooth muscle cells proliferation and migration via sponging miR-590-5p/FGF1 in asthma. Am J Transl Res. 2019;11(5):3159–3166.
- Chang E, Nayak L, Jain MK. Krüppel-like factors in endothelial cell biology. Curr Opin Hematol. 2017;24(3):224–229.
- Fan Y, Lu H, Liang W, et al. Krüppel-like factors and vascular wall homeostasis. J Mol Cell Biol. 2017;9(5):352–363.
- Hamik A, Lin Z, Kumar A, et al. Kruppel-like factor 4 regulates endothelial inflammation. J Biol Chem. 2007;282(18):13769–13779.
- Garrido-Martín EM, Blanco FJ, Roquè M, et al. Vascular injury triggers Krüppel-like factor 6 mobilization and cooperation with specificity protein 1 to promote endothelial activation through upregulation of the activin receptor-like kinase 1 gene. Circ Res. 2013;112(1):113–127.
- Gallardo-Vara E, Blanco FJ, Roqué M, et al. Transcription factor KLF6 upregulates expression of metalloprotease MMP14 and subsequent release of soluble endoglin during vascular injury. Angiogenesis. 2016;19(2):155–171.
- Atkins GB, Jain MK. Role of Krüppel-like transcription factors in endothelial biology. Circ Res. 2007;100(12):1686–1695.
- Pan WK, Li P, Guo ZT, et al. Propranolol induces regression of hemangioma cells via the down-regulation of the PI3K/Akt/eNOS/VEGF pathway. Pediatr Blood Cancer. 2015;62(8):1414–1420.
- Nasirzadeh M, Rasmi Y, Rahbarghazi R, et al. Crocetin promotes angiogenesis in human endothelial cells through PI3K-Akt-eNOS signaling pathway. EXCLI J. 2019;18:936–949. Published 2019 Oct 21
- Xu X, Tian L, Zhang Z. Triptolide inhibits angiogenesis in microvascular endothelial cells through regulation of miR-92a. J Physiol Biochem. 2019;75(4):573–583.