ABSTRACT
Cancers continue to have high incidence and mortality rates worldwide. Therefore, cancer control remains the main public health goal. Growing research evidence suggests that phospholysine phosphohistidine inorganic pyrophosphate phosphatase (LHPP) plays an important role in inhibiting tumor cell progression. It has been reported in the literature that LHPP is expressed at low levels in tumor tissues and cells and that patients with low LHPP expression have a poorer prognosis. Functional studies have shown that LHPP can inhibit tumor cell proliferation, metastasis, and apoptosis by affecting different target genes. In addition, researchers have used iDPP nanoparticles to deliver LHPP plasmids to treat tumors, demonstrating the great potential of LHPP plasmids for cancer therapy. In our review, we highlight the biological functions and important downstream target genes of LHPP in tumors, providing a theoretical basis for the treatment of human cancers. Although not thoroughly studied in terms of tumor mechanisms, LHPP still represents a promising and effective anticancer drug target.
KEYWORDS:
1. Background
Phosphorylation of proteins is regulated by protein kinases and phosphatases and is involved in various functions, such as cell growth and metabolism. Protein phosphorylation occurs on amino acid residues such as serine (Ser), threonine (Thr), tyrosine (Tyr), aspartic acid, glutamic acid, cysteine, histidine, lysine and arginine. Histidine phosphorylase was first reported by Boyer in 1962. Researchers first identified this enzyme as succinyl coenzyme A synthase, a key enzyme of the tricarboxylic acid cycle. Phosphorylation of the imidazole nitrogen atom of histidine forms a high-energy amino-phosphate bond, while phosphorylation of Ser, Thr, and Tyr forms a more stable phosphate ester bond [Citation1]. Due to its chemical instability, histidine phosphorylation has been less studied in the last half century. In recent years, with the development of biological techniques, histidine phosphorylation has been found to be involved in cell signaling processes as a reversible regulatory modification in cells. In tumor cells, histidine kinases and phosphatases participate in cancer development by regulating the phosphorylation of histidine-containing proteins.
1.1 Histidine kinases
The histone kinases mainly include histone H4 histidine kinase (HHK) and nucleoside diphosphate kinase (NDPK). Chen et al. first identified HHK in the nuclei of regenerating rat hepatocytes. Increased HHK activity was associated with liver progenitor cell proliferation and differentiation, suggesting that HHK may be involved in cancer development and progression. HHK activity was significantly higher in hepatocellular carcinoma tissues than in paraneoplastic and normal liver tissues. The expression pattern of HHK was similar to that of tumor developmental markers, such as methemoglobin and pyruvate kinase isoenzymes [Citation2].
NDPK is a nucleotide-metabolizing enzyme encoded by NME genes (involved in neoplasm metastasis), of which NME1 has the highest sequence homology with NME2 and is therefore the most widely studied [Citation3]. Overexpression of NME1 in melanoma, breast cancer and ovarian cancer was shown to inhibit tumor metastasis and increase the overall survival of patients [Citation4]. However, NME1 promotes tumor metastasis in hepatocellular carcinoma, acute myeloid leukemia and neuroblastoma [Citation5]. The dual role of NME1 in tumors may be related to the differences in various biochemical characteristics of NME1 in different tissues, such as NDPK activity and histidine protein kinase activity. NME2 can also function as a metastasis regulator to regulate the occurrence and development of tumors in different cellular environments. NME2 mediates the phosphorylation of Ser5 at the c-terminus of RNA polymerase II by interacting with RNA polymerase II and RNA polymerase II-related protein 2 and promotes the transcription of anti-apoptosis-related genes, thereby inhibiting tumor growth in vivo [Citation6].
1.2 Histidine phosphatases
The known histidine phosphatases are phosphohistidine phosphatase 1 (PHPT1), phospholysine phosphohistidine inorganic pyrophosphate phosphatase (LHPP), and phosphoglycerate mutase 5 (PGAM5) [Citation7]. Histidine phosphatases play a critical role in cell signaling, and their expression and dysfunction play an important role in tumor progression. PHPT1 was the first protein histidine phosphatase to be discovered in vertebrates and humans, and it dephosphorylates the β-subunit of ATP-citrate lyase and G proteins in vitro [Citation8–11]. Studies on the mechanisms associated with tumor progression revealed that higher PHPT1 expression was associated with enhanced invasion of tumor cells; in addition, both in vivo and in vitro studies revealed the important role of PHPT1 in regulating lung cancer cell migration [Citation12,Citation13]. In renal clear cell carcinoma and hepatocellular carcinoma, PHPT1 plays a role in tumorigenesis and development by regulating the proliferation and apoptosis of tumor cells [Citation14,Citation15]. As a cytosolic Ser/Thr phosphatase, PGAM5 is involved in several functions that affect mitochondrial homeostasis. Ma et al. showed that PGAM5 interacts with the BCL-xL and FUNDC1 proteins to modify the mediated molecular switch that regulates mitochondrial autophagy and apoptosis to promote tumor progression [Citation16,Citation17]. In colon cancer cells and cervical cancer cells, PGAM5 induces cell death by regulating mitochondrial fission and necrosis [Citation18]. In addition, some studies have reported that elevated PGAM5 expression leads to a poorer prognosis for patients. Knockdown of PGAM5 inhibits tumor cell proliferation and enhances the chemotherapy sensitivity of tumor cells [Citation19]. In this article, we focus on the newly discovered histidine phosphatase LHPP and its mechanism as a tumor suppressor gene in inhibiting cancer cell progression.
2. Introduction to LHPP
The LHPP gene encodes an enzyme called lysine phosphate histidine inorganic pyrophosphatase (LHPP); LHPP was first isolated from porcine brain tissue, is expressed at high levels in the brain, and is a member of the halogen dehydrogenase structural domain (HDHD) gene family [Citation20]. LHPP is also a novel inorganic pyrophosphatase that is closely associated with cell growth and polarization [Citation21]. LHPP polymorphisms are related to thyroid hormone (TH) levels and are believed to mediate functional modification in patients with major depressive disorder (MDD) [Citation22]. Cui et al. showed that a single nucleotide polymorphism in the LHPP gene (rs35936514) was associated with MDD in a genome-wide association study [Citation23]. LHPP gene is located on q26.13 of chromosome 10 [Citation24]. In addition, it was revealed that LHPP is mainly located in the nucleus and cytoplasm [Citation25,Citation26]. In 2018, researchers demonstrated that LHPP is a protein histidine phosphatase and tumor suppressor and that it acts extensively on N3 phosphorylated proteins [Citation27]. In our review, we concentrate on the features of LHPP in important cancers and its regulatory molecules. In addition, we explored the potential application value of LHPP as a therapeutic target.
3. The function of LHPP in tumors
Because LHPP has been shown to be a tumor suppressor, it has become a highly researched topic in the field of tumor research, and many experiments have demonstrated that LHPP plays a key role in various tumor progression processes, including proliferation, metastasis, and apoptosis [Citation26,Citation28–34]. LHPP is mainly expressed in brain and thyroid tissue, and its expression in different tissues is shown in Figure S1. In addition, it was found in the tumor immune estimation resource (TIMER) data that LHPP expression was significantly lower in BLCA (bladder urothelial carcinoma), CHOL (cholangiocarcinoma), COAD (colon adenocarcinoma), KICH (kidney chromophobe), KIRC (kidney renal clear cell carcinoma), PRAD (prostate adenocarcinoma), READ (rectum adenocarcinoma), and STAD (stomach adenocarcinoma) compared with normal tissues. However, LHPP expression was significantly higher in LUAD (lung adenocarcinoma) compared with normal tissues (). Further studies revealed that LHPP overexpression attenuated the proliferation and metastasis of human hepatocellular carcinoma (HCC) cells by inhibiting the expression of Cyclin B1 (CCNB1), Pyruvate Kinase M2 (PKM2), Matrix metallo proteinase 7/9 (MMP7/9) [Citation31]. In addition, in colorectal cancer (CRC), LHPP inhibited the growth and proliferation and promoted the apoptosis of CRC cells by affecting the phosphatidylinositol-3-kinase/protein kinase B (PI3K/AKT) and cell cycle signaling pathways [Citation35]. All these results suggest that LHPP plays an important role in inhibiting tumor progression. This article mainly summarizes the existing evidence on the aberrant expression, molecular mechanisms and clinical relevance of LHPP.
Figure 1. Human LHPP expression levels in different tumor types from The Cancer Genome Atlas database were determined by TIMER (https://cistrome.shinyapps.io/timer/), and the differential expression was evaluated by Wilcoxon test. TPM:Transcripts Per Million. Red: Tumor, Blue: Normal. (*P < 0.05, **P < 0.01, ***P < 0.001).
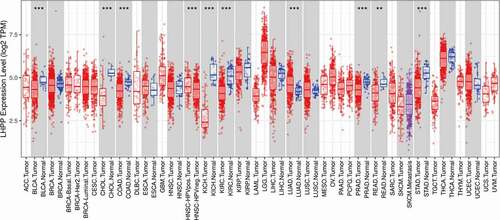
–: Not reported in literature
–: Not reported in literature
3.1 LHPP and liver cancer
Liver cancer has a poor prognosis due to its high proliferation and metastatic capacity [Citation36]. Therefore, it is crucial to understand the potential mechanisms of proliferation and metastasis in hepatocellular carcinoma (HCC) for treatment. Recent studies have shown that nearly half of patients with HCC show abnormal PI3K-AKT-mTOR signaling, mainly including loss of the tumor suppressors Phosphatase and tensin homolog (PTEN), Tuberous Sclerosis 1/2 (TSC1/2). Hindupur et al. successfully constructed a mouse HCC model by knocking out both the PTEN and TSC1 genes [Citation37,Citation38]. Decreased LHPP expression was correlated with tumor progression but not with mTOR activity. The researchers also found that overexpression of LHPP in CB1 and SNU449 cells inhibited cell proliferation and significantly reduced hepatosphere formation in CB1 cells. A reduction in LHPP expression in human HCC leads to enhanced histidine phosphorylation and reduced survival. LHPP inactivation was shown to be a key factor for tumorigenesis in HCC [Citation27]. Liao et al. found that LHPP was decreased in HCC patients. The increased expression of LHPP may be related to the overall survival and disease-free survival of HCC. In addition, LHPP expression was reduced in HCC cell lines compared to normal cell lines. Further studies showed that LHPP overexpression attenuated the proliferation and metastasis of human HCC cells by inhibiting the expression of CCNB1, PKM2, MMP7, and MMP9 [Citation31]. Another study related to HCC showed that miR-765 levels in HCC tissues and miR-765 levels in HCC serum were significantly negatively correlated with LHPP in HCC tissues. Studies of hepatocellular carcinoma cell lines revealed that miR-765 directly targets and binds LHPP at the 3’ untranslated region (3ʹUTR) sequence. MiR-765 overexpression promoted epithelial−mesenchymal transition (EMT) and proliferation through downregulation of LHPP expression and inhibited apoptosis in HepG2 cells [Citation39]. Similar studies have also shown that miR-363-5p and miR-765 bind directly to LHPP to play a targeted regulatory role [Citation40].
Another study by Wang et al. demonstrated the role of LHPP in intrahepatic cholangiocarcinoma (ICC). The investigators found that LHPP was downregulated in tumor tissue and that low levels of LHPP predicted poor survival. Low LHPP expression predicts better overall survival in HCC patients. In vivo and in vitro experiments found that LHPP inhibited ICC cell growth, invasion, and EMT. Further mechanistic studies revealed that LHPP inactivated the transforming growth factor-β (TGFβ) signaling pathway and that interference with LHPP led to enhanced TGFβ expression and phosphorylation of smad2/3. In addition, restoration of normal pathway function reversed the biological function of LHPP [Citation33].
This evidence suggests that aberrant expression of LHPP is related to the advancement of liver cancer. LHPP may be a potential oncogene in liver cancer. These results provide new insights into the mechanisms involved in the development of liver cancer.
3.2 LHPP and CRC
Globally, CRC is one of the most common malignancies in humans and the leading cause of tumor-related deaths [Citation41,Citation42]. Therefore, it is crucial to find new therapeutic targets for CRC. The PI3K/AKT signaling pathway plays an extremely important role in cellular activities, including cell proliferation, migration, autophagy, and other functions. Moreover, an increasing number of studies have shown that PI3K/AKT inhibitors are promising in the treatment of CRC [Citation43,Citation44]. IIn another study, similar results also showed that the LHPP protein inhibits CRC cell growth by suppressing the PI3K/AKT signaling pathway and that overexpression of the LHPP protein suppresses the expression of the EGFR, cyclin-D1, and NF-κB proteins in HCT-116 cells [Citation45]. Aberrant expression of tumor suppressor genes enhances the expression of antiapoptotic proteins and attenuates the expression of proapoptotic proteins; this mechanism underlies colorectal carcinogenesis [Citation46,Citation47]. Hu et al. determined that purpurin strongly interacts with LHPP by binding to it through hydrophobic and hydrophilic bonds. Furthermore, purpurin promoted the expression of the apoptotic genes Bax, caspase-9, and caspase-3 in HCT-116 cells by inducing the expression of LHPP [Citation45]. Hou et al. showed that LHPP expression was reduced in CRC tissues compared to normal colorectal tissues and that increased expression of LHPP may be related to tumor size, TNM stage and lymphatic metastasis of CRC. However, bioinformatics studies found that LHPP was not associated with patient prognosis. These differences are likely due to the limited sample size, slightly different genetic mutations in different countries, and differences in surgeon surgical skills, which play have a crucial effect on the outcome of CRC patients. Mechanistic studies in vivo and in vitro have shown that LHPP can inhibit the growth and proliferation of CRC cells and promote their apoptosis through the PI3K/AKT and cell cycle signaling pathways [Citation35]. Mechanistic studies have shown that LHPP inhibits CRC cell proliferation and metastasis through the TGF-β/Smad signaling pathway [Citation48]. Therefore, LHPP may suppress CRC progression. This idea suggests that low expression of LHPP leads to poor prognostic survival of CRC and that elevated expression of LHPP inhibits proliferation and metastasis and promotes apoptosis of CRC cells.
3.3 LHPP and PCa
PCa, one of the most common types of cancer in men, still has a poor survival rate and prognosis, despite advances in medical technology that have further improved its diagnosis and treatment [Citation49–51]. Therefore, to improve the diagnosis and treatment of PCa, we urgently need to find new potential therapeutic targets. YTHDF2, a, important cytoplasmic m6A reader, degrades N6-methyladenosine (m6A)-modified mRNA by binding to m6A sites and promotes tumor progression in multiple cancers [Citation52–55]. Li et al. found that YTHDF2 enhances AKT phosphorylation in PCa by targeting the tumor suppressor LHPP for degradation. The researchers also found that LHPP is a novel tumor suppressor that inhibits the proliferation and metastasis of PCa cells by inhibiting the phosphorylation of vehicle AKT. Investigators have shown through in vitro and in vivo studies that LHPP can mediate mRNA degradation in an m6A-dependent manner via upstream YTHDF2 to regulate PCa tumor progression [Citation56]. Lin et al. found that LHPP expression was downregulated in PCa samples compared to normal prostate samples. Interestingly, LHPP expression was downregulated in N1 grade PCa patients compared to N0 grade patients. In vivo studies revealed that silencing LHPP inhibited the proliferation and metastatic capacity of 22Rv1 and PC-3 cells via E-cadherin and vimentin. These data suggest that LHPP inhibits EMT progression in PCa. Further study of the mechanism showed that upregulation of the circular RNA DDX17 promoted LHPP mRNA and protein expression, and miR-346 could bind directly to the 3ʹUTR of LHPP. These results suggest that circDDX17 can promote LHPP expression by binding miR-346 [Citation57]. The experimental results of the abovementioned studies suggest that LHPP can regulate the progression of PCa and provide a theoretical basis for LHPP as a potential therapeutic target in PCa.
3.4 LHPP and glioblastoma
Glioblastoma (GBM) is one of the most common and fatal malignancies in the nervous system and has some of the highest rates of morbidity and mortality. Despite advances in modern medical technology, the highly proliferative and aggressive cellular characteristics of GBM lead to poor treatment outcomes and prognosis [Citation58,Citation59]. Therefore, we need to further explore the specific progression of GBM tumors and develop new therapeutic targets. Wnt/β-catenin signaling is widely involved in the progression of many cancers, including GBM [Citation60,Citation61]. It has been reported that LHPP expression is downregulated in GBM. In addition, LHPP inhibits the proliferation and metastasis of GBM through the Akt and Wnt/β-catenin signaling pathways, and reactivation of Wnt/β-catenin signaling reverses the tumor suppressive effect of LHPP [Citation29]. Another study also found that LHPP was abnormally reduced in GBM and that patients with lower expression of LHPP had a poorer prognosis. Mechanistic studies have shown that LHPP inhibits glycolysis in tumor cells by inducing ubiquitin-mediated PKM2 degradation. In addition, increasing PKM2 expression effectively reversed the growth inhibition of cancer cells caused by LHPP in GBM [Citation28]. The results related to the effects of LHPP in GBM suggest that it may be a potential therapeutic target.
3.5 LHPP and cervical cancer
In addition to being the most common gynecologic malignancy, cervical cancer also has the highest mortality rate among cancers [Citation62,Citation63]. Although its incidence and mortality have decreased significantly in recent decades with the development of techniques for diagnosis and treatment, the prognosis of patients with advanced-stage disease remains poor [Citation64,Citation65]. Zheng et al. found that LHPP expression was downregulated in clinical cervical cancer tumor tissues and cell lines and that patients with greater levels of LHPP expression showed a better prognosis. Increased expression of LHPP may be related to tumor size and lymphatic metastasis of cervical cancer. Researchers have also found that LHPP inhibits the proliferation and metastasis of cervical cancer cells through MMP-2, MMP-9, TGF-β1, fibronectin, Neuronal cadherin (N-cadherin), and vimentin and promotes apoptosis by inhibiting AKT activation [Citation66]. In addition, inhibition of the cyclin D1/CDK4 pathway plays a key role in regulating tumor progression, and LHPP significantly suppresses the expression of cyclin D1 and CDK4 [Citation67]. P27 and p53 perform significant functions in inhibiting tumor development. Some investigators have found that LHPP significantly increases the protein levels of p53 and p27 and inhibits the growth of cervical cancer [Citation66,Citation68]. These results suggest that LHPP is a prospective target for therapy development in the fight against cervical cancer.
3.6 LHPP and thyroid cancer
Thyroid carcinoma (TC) is the most commonly occurring malignant tumor of the thyroid gland and accounts for approximately 1% of all malignant tumors in the body. In recent decades, the incidence of TC has ranked eighth among cancers in China, and TC has been a heavy economic burden on society [Citation69–71]. Studies of TC revealed that LHPP expression was downregulated in TC tissues and cell lines [Citation32]. Cellular autophagy is an evolutionarily conserved and important process in eukaryotes for the turnover of intracellular material and participates in a number of physiological and pathophysiological processes in tumors [Citation72,Citation73] LHPP enhances autophagy in TC cells in response to the regulation of BECN1 and LC3BI/II. The PI3K/AKT/mTOR signaling pathway is engaged in the progression of many cancers and plays an important role in the development of TC. Sun et al. showed that LHPP inhibits cell proliferation, migration, and invasion by regulating the AKT/AMPK/mTOR signaling pathway. Increased expression of LHPP may be related to the disease-free survival of TC. It was further shown that LHPP enhances autophagy in TC by regulating the AKT/AMPK/mTOR signaling pathway [Citation32,Citation74]. These results show that LHPP inhibits the progression of TC cells and indicate that LHPP may be a promising treatment target.
3.7 LHPP and bladder cancer
Bladder cancer (BC) ranks fourth and ninth in incidence among malignancies in men and women, respectively. In recent decades, the morbidity of BC has been increasing [Citation75–77]. Thus, it is essential to improve the understanding of the pathogenesis of and reveal new treatments for BC tumors. It has been reported that the protein and mRNA expression of LHPP in BC tissues is reduced [Citation30]. Aerobic glycolysis, represented by the Warburg effect, is a metabolic marker of tumors [Citation78,Citation79]. In a recent study, knockdown of LHPP was found to lead to increased glucose consumption and lactate production in BC cells. Functional studies have demonstrated that LHPP inhibits the proliferation, metastasis, and invasion of BC cells through the regulation of the cell cycle proteins p21, p27, and cyclin B. The majority of research indicates that p65 activation enhances cancer progression. Some studies have shown that downregulation of LHPP activates p65 by enhancing AKT signaling [Citation30,Citation80,Citation81]. These studies highlight the novel role of LHPP in suppressing BC cell function, and LHPP may serve as a powerful diagnostic and therapeutic target for BC.
3.8 LHPP and other malignant tumors
With the demonstration of LHPP as a tumor suppressor, many researchers have conducted in-depth studies of its regulatory mechanism and found aberrant expression of LHPP in melanoma, renal cell carcinoma (RCC), pancreatic cancer (PaCa), non-small-cell lung cancer (NSCLC), and laryngeal cancer (LC). In LC, LHPP expression is reduced in tumor tissues, and the prognosis of patients with high LHPP expression is significantly better. A gene set enrichment analysis (GSEA) suggested that LHPP may regulate glycolysis to inhibit the progression of LC [Citation82]. Wu et al. found that LHPP was expressed at low levels in PaCa tissues and cell lines. Analysis of the clinical features suggests that the decrease in LHPP in PaCa may be associated with increased invasion of lymphatic tissue by cancer cells. Cell function studies show that LHPP inhibits the proliferation, migration, and invasion of PaCa cells. These effects may be related to the inhibition of snail, laminin-5, L1CAM, N-cadherin, and vimentin mRNA expression by LHPP. In addition, LHPP promotes PaCa cell apoptosis, which may be related to the regulation of the target proteins cIAP1, cleaved PARP, and cleaved Casp3 [Citation34]. Increased expression of LHPP may be related to lymphatic metastasis of PaCa. Zhang et al. revealed that LHPP was also significantly downregulated in RCC tissues and cell lines and that LHPP was positively related to tumor size and metastasis in RCC patients. In addition, LHPP significantly suppressed the growth and metastasis of RCC cells. However, inhibition of LHPP led to opposite effects [Citation26].. Further studies showed that miRNA-765, miRNA-21, and miRNA-144 can promote the proliferation, EMT, and invasion of RCC cells by downregulating LHPP [Citation83]. Yang et al. showed that LHPP suppresses cell EMT, proliferation, and invasion via vimentin and E-cadherin in NSCLC cells. Further mechanistic studies revealed that miR-217 directly binds to the LHPP 3ʹUTR and that GAS5 directly binds miR-217 to regulate LHPP. These studies suggest that GAS5 enhances LHPP expression by directly binding to the miR-217 binding site in NSCLC [Citation84]. The prognosis of tumor patients with low GAS5 expression is poor, and GAS5 expression is also associated with drug resistance [Citation85–88]. MiR-217 gene expression promotes the proliferation and metastasis of tumor cells [Citation89]. LHPP inhibits the proliferation of melanoma B16-F10 cells by arresting the cell cycle at the G0/G1 transition. In addition, LHPP promotes apoptosis in melanoma cells through upregulation of the caspase 3 and fas genes. Further study of the mechanism revealed that LHPP overexpression suppresses the transcription of the H2afv, EZH2 and CDK8 genes in melanoma [Citation90]. The H2AFV gene is potentially oncogenic and plays an important role in the tumor cell cycle and EMT [Citation91]. Studies have shown that EZH2 is essential for the biological function of cancer cell lines, and CDK8 is a target and biomarker for cancer therapy [Citation92,Citation93]. In addition, investigators have used iDPP nanoparticles delivering LHPP plasmids to treat melanoma in vivo and in vitro. The results showed that the LHPP nanocomplex inhibits the proliferation and promotes the apoptosis of melanoma cells and causes minimal systemic toxicity [Citation90].
4. Regulatory mechanisms of LHPP in cancers
The role of LHPP in the signaling pathways involved in cancer development has been comprehensively explored. As shown in , Although the mechanism of LHPP is relatively less explored at present, LHPP seems to affect tumor progression in many cancer types through the regulation of the PI3K-Akt pathway. Therefore, we mainly discuss the mechanisms related to the effects of LHPP on the PI3K-Akt pathway. The PI3K/Akt signaling pathway plays an important role in tumor progression, including processes such as cell proliferation, apoptosis and metastasis. LHPP plays an important role in tumor metastasis by regulating the expression of its upstream and downstream genes. Mechanistic studies have shown that LHPP inhibits the phosphorylation of smad2/3 through the TGF-β1/PI3K/Akt signaling pathway [Citation33]. Experiments in HCT-116 cells showed that the LHPP protein inhibits CRC cell growth by suppressing the PI3K/AKT signaling pathway and that overexpression of the LHPP protein suppresses the expression of the EGFR, cyclin-D1, and NF-κB proteins in HCT-116 cells [Citation45]. In another paper, similar results also showed that LHPP can inhibit the growth and proliferation of CRC cells and promote their apoptosis through the PI3K/AKT signaling pathway [Citation35]. Li et al. found that YTHDF2 enhances AKT phosphorylation in PCa by targeting the tumor suppressor LHPP for degradation. The researchers also found that LHPP is a novel tumor suppressor that inhibits the proliferation and metastasis of PCa cells by inhibiting the phosphorylation of vehicle AKT [Citation56]. In addition, LHPP inhibits the proliferation and metastasis of GBM cells through the Akt signaling pathway [Citation29]. Further mechanistic studies showed that LHPP inhibits the expression of MMP-2, MMP-9, TGF-β1, fibronectin, N-cadherin, and vimentin through the AKT pathway in cervical cancer cells [Citation66]. Sun et al. showed that LHPP inhibits cell proliferation, migration, and invasion by regulating the AKT/AMPK/mTOR signaling pathway. It was further shown that LHPP enhances autophagy in TC by regulating the AKT/AMPK/mTOR signaling pathway [Citation32,Citation74]. Some studies have shown that downregulation of LHPP activates p65 by enhancing AKT signaling [Citation30,Citation80,Citation81]. Wu et al. found that LHPP inhibited the mRNA expression of snail, laminin-5, L1CAM, N-cadherin, and vimentin through the AKT signaling pathway. In addition, LHPP can also regulate the target proteins cIAP1, cleaved PARP, and cleaved Casp3 through the AKT signaling pathway [Citation34]. In conclusion, LHPP can regulate the PI3K/Akt signaling pathway, greatly affecting the signal transduction of cancer cells.
Table 1. Effect of LHPP upregulation in tumor cells
Table 2. Effect of LHPP downregulation in tumor cells
5. Conclusion
LHPP has been shown to be a tumor suppressor [Citation27]. As a novel histidine phosphatase, LHPP is downregulated in various cancers and associated with clinical and pathological characteristics, including tumor stage, TNM stages, lymph node metastasis, Tumor size, and survival of cancer patients (). Functional studies have also shown that LHPP is highly expressed in tumors, and LHPP can inhibit tumor cell proliferation, metastasis, and glycolysis and promote apoptosis to regulate cancer progression. The proliferation and metastasis of tumor cells are important factors affecting cancer progression. Mechanistic studies have shown that LHPP competitively binds with microRNAs (such as miR-363-5p and miR-765), resulting in the abnormal expression of their downstream target genes (such as PKM2), and modulation of some classical signaling pathways (such as PI3K-AKT pathway) (). Although many studies have shown that LHPP can inhibit tumorigenesis, the mechanism by which it inhibits tumor progression has not been studied in depth. In addition, although LHPP-loaded nanocarriers can inhibit the progression of melanoma cells, the toxicity and unpredictable side effects of nanoparticles still need to be further explored. LHPP can be used in combination with existing chemoradiotherapy to increase tumor sensitivity to existing therapies. The key challenges in this regard will be to find an effective method of targeting LHPP and an effective in vivo delivery method. To date, siRNA and other nucleic acid therapies have become increasingly mature and can successfully knock out the expression of LHPP in cells. Clinical trials have shown that combining small molecules to promote LHPP expression in cancer can be used as an effective strategy. With an increasing number of genomic studies on their roles in cancer pathogenesis and the improved success rate of nucleic acid therapy, LHPP and other genes will soon become viable therapeutic targets for cancer treatment. Therefore, researchers need to develop a comprehensive understanding of the mechanism of LHPP, explore how it regulates tumor progression, and provide further evidence to support the use of LHPP as a therapeutic target. Regardless, LHPP remains a promising cancer therapeutic target. Although the application of LHPP in vivo is currently relatively lacking, its therapeutic effect on tumors still deserves further exploration.
Table 3. Clinical characteristics of LHPP in various tumors
Ethics approval and consent to participate
Not applicable.
Consent for publication
Not applicable.
Availability of data and materials
The datasets generated during and/or analysed during the current study are available in the [TIMER] repository.
Authors’ contributions
FH Wu and HW Ma analyzed and interpreted the data, and were major contributor in writing the manuscript. XL Wang, HZ Wei, W Zhang provided technical support. YC Zhang, who provided ideas and financial support, was our corresponding author. All authors read and approved the final manuscript.
Supplemental Material
Download MS Word (1.9 MB)Disclosure statement
No potential conflict of interest was reported by the author(s).
Supplementary material
Supplemental data for this article can be accessed here.
Additional information
Funding
References
- Boyer PD, DeLuca M, Ebner KE, et al. Identification of phosphohistidine in digests from a probable intermediate of oxidative phosphorylation. J Biol Chem. 1962;237(10): Pc3306–pc3308.
- Tan E, Besant PG, and Zu XL, et al. Histone H4 histidine kinase displays the expression pattern of a liver oncodevelopmental marker. Carcinogenesis. 2004;25(11):2083–2088.
- Attwood PV, Muimo R. The actions of NME1/NDPK-A and NME2/NDPK-B as protein kinases. Lab Invest. 2018;98(3):283–290.
- Boissan M, Schlattner U, Lacombe M-L. The NDPK/NME superfamily: state of the art. Lab Invest. 2018;98(2):164–174.
- Andolfo I, De Martino D, Liguori L, et al. Correlation of NM23-H1 cytoplasmic expression with metastatic stage in human prostate cancer tissue. Naunyn Schmiedebergs Arch Pharmacol. 2011;384(4–5):489–498.
- Gong Y, Yang G, Wang Q, et al. NME2 Is a Master Suppressor of Apoptosis in Gastric Cancer Cells via Transcriptional Regulation of miR-100 and Other Survival Factors. Mol Cancer Res. 2020;18(2):287–299.
- Fuhs SR, Hunter T. pHisphorylation: the emergence of histidine phosphorylation as a reversible regulatory modification. Curr Opin Cell Biol. 2017;45:8–16.
- Ek P, Pettersson G, Ek B, et al. Identification and characterization of a mammalian 14-kDa phosphohistidine phosphatase. Eur J Biochem. 2002;269(20):5016–5023.
- Klumpp S, Bechmann G, Mäurer A, et al. ATP-citrate lyase as a substrate of protein histidine phosphatase in vertebrates. Biochem Biophys Res Commun. 2003;306(1):110–115.
- Klumpp S, Hermesmeier J, Selke D, et al. Protein histidine phosphatase: a novel enzyme with potency for neuronal signaling. J Cereb Blood Flow Metab. 2002;22(12):1420–1424.
- Mäurer A, Wieland T, Meissl F, et al. The beta-subunit of G proteins is a substrate of protein histidine phosphatase. Biochem Biophys Res Commun. 2005;334(4):1115–1120.
- Tian T, Hao J, Xu A, et al. Determination of metastasis-associated proteins in non-small cell lung cancer by comparative proteomic analysis. Cancer Sci. 2007;98(8):1265–1274.
- Xu A, Hao J, Zhang Z, et al. 14-kDa phosphohistidine phosphatase and its role in human lung cancer cell migration and invasion. Lung Cancer. 2010;67(1):48–56.
- Han SX, Wang L-J, Zhao J, et al. 14-kDa Phosphohistidine phosphatase plays an important role in hepatocellular carcinoma cell proliferation. Oncol Lett. 2012;4(4):658–664.
- Shen H, Yang P, Liu Q, et al. Nuclear expression and clinical significance of phosphohistidine phosphatase 1 in clear-cell renal cell carcinoma. J Int Med Res. 2015;43(6):747–757.
- Lu W, Karuppagounder SS, Springer DA, et al. Genetic deficiency of the mitochondrial protein PGAM5 causes a Parkinson’s-like movement disorder. Nat Commun. 2014;5(1):4930.
- Ma K, Zhang Z, Chang R, et al. Dynamic PGAM5 multimers dephosphorylate BCL-xL or FUNDC1 to regulate mitochondrial and cellular fate. Cell Death Differ. 2020;27(3):1036–1051.
- Wang Z, Jiang H, Chen S, et al. The mitochondrial phosphatase PGAM5 functions at the convergence point of multiple necrotic death pathways. Cell. 2012;148(1–2):228–243.
- Cheng J, Qian D, Ding X, et al. High PGAM5 expression induces chemoresistance by enhancing Bcl-xL-mediated anti-apoptotic signaling and predicts poor prognosis in hepatocellular carcinoma patients. Cell Death Dis. 2018;9(10):991.
- Cui L, Gong X, Tang Y, et al. Relationship between the LHPP Gene Polymorphism and Resting-State Brain Activity in Major Depressive Disorder. Neural Plast. 2016;2016:9162590.
- Seal US, Binkley F. An inorganic pyrophosphatase of swine brain. J Biol Chem. 1957;228(1):193–199.
- Peng R, Li Y. Low serum thyroid-stimulating hormone levels are associated with lipid profile in depressive patients with long symptom duration. J Affect Disord. 2017;217:99–104.
- Cui L, Gong X, Chang M, et al. Association of LHPP genetic variation (rs35936514) with structural and functional connectivity of hippocampal-corticolimbic neural circuitry. Brain Imaging and Behavior. 2020;14(4):1025–1033.
- Knowles EEM, Kent JW, McKay DR, et al. Genome-wide linkage on chromosome 10q26 for a dimensional scale of major depression. J Affect Disord. 2016;191:123–131.
- Chao X, Zhang W, Wu J, et al. Downregulation of LHPP Expression Associated with AFP Acts as a Good Prognostic Factor in Human Hepatocellular Carcinoma. Biomed Res Int. 2021;2021:1971048.
- Zhang X, Kang H, Xiao J, et al. LHPP Inhibits the Proliferation and Metastasis of Renal Cell Carcinoma. Biomed Res Int. 2020;2020:7020924.
- Hindupur SK, Colombi M, Fuhs SR, et al. The protein histidine phosphatase LHPP is a tumour suppressor. Nature. 2018;555(7698):678–682.
- Chen W-J, Chen L-H, Wang J, et al. LHPP impedes energy metabolism by inducing ubiquitin-mediated degradation of PKM2 in glioblastoma. Am J Cancer Res. 2021;11(4):1369–1390.
- Li C, Yang J, Wang W, et al. LHPP exerts a tumor-inhibiting role in glioblastoma via the downregulation of Akt and Wnt/β-catenin signaling. J Bioenerg Biomembr. 2021;53(1):61–71.
- Li Y, Zhang X, and Zhou X, et al. LHPP suppresses bladder cancer cell proliferation and growth via inactivating AKT/p65 signaling pathway. Biosci Rep. 2019;39(7). DOI:https://doi.org/10.1042/BSR20182270
- Liao L, Duan D, Liu Y, et al. LHPP inhibits hepatocellular carcinoma cell growth and metastasis. Cell Cycle. 2020;19(14):1846–1854.
- Sun W, Qian K, Guo K, et al. LHPP inhibits cell growth and migration and triggers autophagy in papillary thyroid cancer by regulating the AKT/AMPK/mTOR signaling pathway. Acta Biochim Biophys Sin (Shanghai). 2020;52(4):382–389.
- Wang D, Ning Z, Zhu Z, et al. LHPP suppresses tumorigenesis of intrahepatic cholangiocarcinoma by inhibiting the TGFβ/smad signaling pathway. Int J Biochem Cell Biol. 2021;132:105845.
- Wu F, Chen Y, and Zhu J. LHPP suppresses proliferation, migration, and invasion and promotes apoptosis in pancreatic cancer. Biosci Rep. 2020;40(3). DOI:https://doi.org/10.1042/BSR20194142
- Hou B, Li W, Li J, et al. Tumor suppressor LHPP regulates the proliferation of colorectal cancer cells via the PI3K/AKT pathway. Oncol Rep. 2020;43(2):536–548.
- Yu T, Han C, Zhu G, et al. Prognostic value of Notch receptors in postsurgical patients with hepatitis B virus-related hepatocellular carcinoma. Cancer Med. 2017;6(7):1587–1600.
- Llovet JM, Zucman-Rossi J, Pikarsky E, et al. Hepatocellular carcinoma. Nat Rev Dis Primers. 2016;2:16018.
- Schulze K, Imbeaud S, Letouzé E, et al. Exome sequencing of hepatocellular carcinomas identifies new mutational signatures and potential therapeutic targets. Nat Genet. 2015;47(5):505–511.
- Yan J, He L, Li G, et al. Clinical Value of Serum LHPP-associated miR-765 in the Prognosis of Laparoscopic or Open Hepatectomy for Hepatocellular Carcinoma. Surgical Laparoscopy, Endoscopy & Percutaneous Techniques. 2020;30(5):395–402.
- Tian Z, Yu T, Wei H, et al. Clinical value of LHPP-associated microRNAs combined with protein induced by vitamin K deficiency or antagonist-II in the diagnosis of alpha-fetoprotein-negative hepatocellular carcinoma. J Clin Lab Anal. 2020;34(2):e23071.
- Brenner H, Kloor M, Pox CP. Colorectal cancer. Lancet. 2014;383(9927):1490–1502.
- Siegel RL, Miller KD, Jemal A. Cancer statistics, 2019. CA Cancer J Clin. 2019;69(1):7–34.
- Bahrami A, Khazaei M, Hasanzadeh M, et al. Therapeutic Potential of Targeting PI3K/AKT Pathway in Treatment of Colorectal Cancer: rational and Progress. J Cell Biochem. 2018;119(3):2460–2469.
- Noorolyai S, Shajari N, Baghbani E, et al. The relation between PI3K/AKT signalling pathway and cancer. Gene. 2019;698:120–128.
- Li Z, Zhou X, Zhu H, et al. Purpurin binding interacts with LHPP protein that inhibits PI3K/AKT phosphorylation and induces apoptosis in colon cancer cells HCT-116. J Biochem Mol Toxicol. 2021;35(3):e22665.
- Pan M-H, Lai C-S, Wu J-C, et al. Molecular mechanisms for chemoprevention of colorectal cancer by natural dietary compounds. Mol Nutr Food Res. 2011;55(1):32–45.
- Perše M. Oxidative stress in the pathogenesis of colorectal cancer: cause or consequence? Biomed Res Int. 2013;2013:725710.
- Hou B, Li W, Xia P, et al. LHPP suppresses colorectal cancer cell migration and invasion in vitro and in vivo by inhibiting Smad3 phosphorylation in the TGF-β pathway. Cell Death Discov. 2021;7(1):273.
- Chen R, Sheng L, Zhang H-J, et al. miR-15b-5p facilitates the tumorigenicity by targeting RECK and predicts tumour recurrence in prostate cancer. J Cell Mol Med. 2018;22(3):1855–1863.
- Ferlay J, Shin H-R, Bray F, et al. Estimates of worldwide burden of cancer in 2008: GLOBOCAN 2008. Int J Cancer. 2010;127(12):2893–2917.
- Poorthuis MHF, Vernooij RWM, van Moorselaar RJA, et al. Second-line therapy in patients with metastatic castration-resistant prostate cancer with progression after or under docetaxel: a systematic review of nine randomized controlled trials. Semin Oncol. 2017;44(5):358–371.
- Du H, Zhao Y, He J, et al. YTHDF2 destabilizes m6A-containing RNA through direct recruitment of the CCR4–NOT deadenylase complex. Nat Commun. 2016;7(1):12626.
- Wang H, Zuo H, Liu J, et al. Loss of YTHDF2-mediated m6A-dependent mRNA clearance facilitates hematopoietic stem cell regeneration. Cell Res. 2018;28(10):1035–1038.
- Wang X, Lu Z, Gomez A, et al. N6-methyladenosine-dependent regulation of messenger RNA stability. Nature. 2014;505(7481):117–120.
- Yang Z, Li J, Feng G, et al. MicroRNA-145 Modulates N6-Methyladenosine Levels by Targeting the 3′-Untranslated mRNA Region of the N6-Methyladenosine Binding YTH Domain Family 2 Protein. J Biol Chem. 2017;292(9):3614–3623.
- Li J, Xie H, Ying Y, et al. YTHDF2 mediates the mRNA degradation of the tumor suppressors to induce AKT phosphorylation in N6-methyladenosine-dependent way in prostate cancer. Mol Cancer. 2020;19(1):152.
- Lin Q, Cai J, Wang -Q-Q. The Significance of Circular RNA DDX17 in Prostate Cancer. Biomed Res Int. 2020;2020:1878431.
- Boele FW, Given CW, Given BA, et al. Family caregivers‘ level of mastery predicts survival of patients with glioblastoma: a preliminary report. Cancer. 2017;123(5):832–840.
- Ostrom QT, Cioffi G, Gittleman H, et al. CBTRUS Statistical Report: primary Brain and Other Central Nervous System Tumors Diagnosed in the United States in 2012–2016. Neuro Oncol. 2019;21(Suppl Supplement_5):v1–v100.
- McCord M, Mukouyama Y-S, Gilbert MR, et al. Targeting WNT Signaling for Multifaceted Glioblastoma Therapy. Front Cell Neurosci. 2017;11:318.
- Zhong Z, Yu J, Virshup DM, et al. Wnts and the hallmarks of cancer. Cancer Metastasis Rev. 2020;39(3):625–645.
- Tewari KS, Sill MW, Long HJ, et al. Improved survival with bevacizumab in advanced cervical cancer. N Engl J Med. 2014;370(8):734–743.
- Cancer Genome Atlas Research Network. Integrated genomic and molecular characterization of cervical cancer. Nature. 2017;543(7645):378–384. DOI:https://doi.org/10.1038/nature21386
- Siegel RL, Miller KD, Jemal A. Cancer statistics, 2016. CA Cancer J Clin. 2016;66(1):7–30.
- Zhu L, Zhu L, Shi H, et al. Evaluating early response of cervical cancer under concurrent chemo-radiotherapy by intravoxel incoherent motion MR imaging. BMC Cancer. 2016;16(1):79.
- Zheng J, Dai X, Chen H, et al. Down-regulation of LHPP in cervical cancer influences cell proliferation, metastasis and apoptosis by modulating AKT. Biochem Biophys Res Commun. 2018;503(2):1108–1114.
- Peurala E, Koivunen P, Haapasaari K-M, et al. The prognostic significance and value of cyclin D1, CDK4 and p16 in human breast cancer. Breast Cancer Res. 2013;15(1):R5.
- Yadav V, Sultana S, Yadav J, et al. Gatifloxacin induces S and G2-phase cell cycle arrest in pancreatic cancer cells via p21/p27/p53. PLoS One. 2012;7(10):e47796.
- Chen W, Zheng R, Baade PD, et al. Cancer statistics in China, 2015. CA Cancer J Clin. 2016;66(2):115–132.
- Pellegriti G, Frasca F, Regalbuto C, et al. Worldwide increasing incidence of thyroid cancer: update on epidemiology and risk factors. J Cancer Epidemiol. 2013;2013:965212.
- Siegel RL, Miller KD, Jemal A. Cancer statistics, 2015. CA Cancer J Clin. 2015;65(1):5–29.
- Lei Y, Zhang D, Yu J, et al. Targeting autophagy in cancer stem cells as an anticancer therapy. Cancer Lett. 2017;393:33–39.
- Levy JMM, Towers CG, Thorburn A. Targeting autophagy in cancer. Nat Rev Cancer. 2017;17(9):528–542.
- Feng Z. p53 regulation of the IGF-1/AKT/mTOR pathways and the endosomal compartment. Cold Spring Harb Perspect Biol. 2010;2(2):a001057.
- Pollard C, Smith SC, Theodorescu D. Molecular genesis of non-muscle-invasive urothelial carcinoma (NMIUC). Expert Rev Mol Med. 2010;12:e10.
- Rosenberg JE, Hahn WC. Bladder cancer: modeling and translation: table 1. Genes Dev. 2009;23(6):655–659.
- Sanli O, Dobruch J, Knowles MA, et al. Bladder cancer. Nat Rev Dis Primers. 2017;3:17022.
- Hanahan D, Weinberg RA. Hallmarks of cancer: the next generation. Cell. 2011;144(5):646–674.
- Hsu PP, Sabatini DM. Cancer cell metabolism: warburg and beyond. Cell. 2008;134(5):703–707.
- Maeda S, Kamata H, Luo J-L, et al. IKKβ Couples Hepatocyte Death to Cytokine-Driven Compensatory Proliferation that Promotes Chemical Hepatocarcinogenesis. Cell. 2005;121(7):977–990.
- Taniguchi K, Karin M. NF-κB, inflammation, immunity and cancer: coming of age. Nat Rev Immunol. 2018;18(5):309–324.
- Ding Z, Yu D, Li H, et al. Prognostic Correlation of Glycolysis-Related Gene Signature in Patients with Laryngeal Cancer. Am J Med Sci. 2021;362(2):161–172.
- Meng K, Li Z, Cui X. Three LHPP gene-targeting co-expressed microRNAs (microRNA-765, microRNA-21, and microRNA-144) promote proliferation, epithelial-mesenchymal transition, invasion, and are independent prognostic biomarkers in renal cell carcinomas patients. J Clin Lab Anal. 2021;35(12):e24077.
- Yang X, Meng L, Zhong Y, et al. The long intergenic noncoding RNA GAS5 reduces cisplatin-resistance in non-small cell lung cancer through the miR-217/LHPP axis. Aging (Albany NY). 2021;13(2):2864–2884.
- Li J, Huang H, Li Y, et al. Decreased expression of long non-coding RNA GAS5 promotes cell proliferation, migration and invasion, and indicates a poor prognosis in ovarian cancer. Oncol Rep. 2016;36(6):3241–3250.
- Tan Q, Zuo J, Qiu S, et al. Identification of circulating long non-coding RNA GAS5 as a potential biomarker for non-small cell lung cancer diagnosisnon-small cell lung cancer, long non-coding RNA, plasma, GAS5, biomarker. Int J Oncol. 2017;50(5):1729–1738.
- Wang T-H, Chan C-W, Fang J-Y, et al. 2-O-Methylmagnolol upregulates the long non-coding RNA, GAS5, and enhances apoptosis in skin cancer cells. Cell Death Dis. 2017;8(3):e2638.
- Xue Y, Ni T, Jiang Y, et al. Long Noncoding RNA GAS5 Inhibits Tumorigenesis and Enhances Radiosensitivity by Suppressing miR-135b Expression in Non-Small Cell Lung Cancer. Oncol Res. 2017;25(8):1305–1316.
- Bai M, Zhang M, Long F, et al. MiR-217 promotes cutaneous squamous cell carcinoma progression by targeting PTRF. Am J Transl Res. 2017;9(2):647–655.
- Zhang Q, Xiong M, Liu J, et al. <p>Targeted nanoparticle-mediated LHPP for melanoma treatment. Int J Nanomedicine. 2019;14:3455–3468.
- Yang HD, Kim P-J, Eun JW, et al. Oncogenic potential of histone-variant H2A.Z.1 and its regulatory role in cell cycle and epithelial-mesenchymal transition in liver cancer. Oncotarget. 2016;7(10):11412–11423.
- Chou J, Quigley DA, Robinson TM, et al. Transcription-Associated Cyclin-Dependent Kinases as Targets and Biomarkers for Cancer Therapy. Cancer Discov. 2020;10(3):351–370.
- Kim KH, Roberts CWM. Targeting EZH2 in cancer. Nat Med. 2016;22(2):128–134.