ABSTRACT
The yield efficiency of transgenic animal generation is relatively low[Citation1]. To improve its efficiency has become a priority task for researchers[Citation2]. Melatonin (N-acetyl-5-methoxytryptamine, MT) is a potent-free radical scavenger and antioxidant to protect mitochondria, lipids, protein and DNA from oxidative stress[Citation3]. In this study, we observed that improving the quality of both donor and recipient cells by giving physiological concentration (10−7 M) of MT significantly increase the sheep transgenic embryo development in the in vitro condition. MT promotes the donor cell viability, proliferation, efficiency of monoclonal formation and the electrotransferring efficiency of fetal fibroblast cells (FFCs). The mechanistic exploration indicates that MT has the capacity for the synchronization of cell division cycle, reduction of cellular oxidative stress, apoptosis, and the increase of mitochondrial number and function. All of these render MT’s ability to increase the efficiency of animal transgenic processes such as somatic cell nuclear transfer (SCNT) and electroporation. The outcomes are the increased cleavage rate and blastocyst rate of the transgenic sheep embryos after MT treatment. These beneficial effects of MT on transgenic embryo development are worth to be tested in the in vivo condition in the future.
1. Introduction
In 1996, a sheep was successfully cloned from highly differentiated embryonic cells [Citation4]. A year later, the same group had cloned the Sheep Dolly from adult somatic cells, an unprecedented success in the transgenic history [Citation5]. In the same year, another significant progress was made by using transfected fetal fibroblast cells (FFCs) for the generation of the first transgenic cloned sheep [Citation6]. Since then, somatic cell nuclear transfer (SCNT) technology has become an important part of transgenic animal breeding [Citation7]. The safety of the transgenic animals and the integration efficiency of exogenous genes are the two main concerning factors for this technology [Citation8]. In addition, this technique is very complicated and the success rate is considerably low [Citation9], for example, fewer than 3% of cloned embryos can develop to term in mice [Citation10].
A variety of methods have been used to improve the SCNT embryo development including optimizing physiological conditions of recipient oocytes or donor cells [Citation11,Citation12], using cells from different stages of the cell cycle [Citation13,Citation14], different kinds of donor cells [Citation15,Citation16], different strains of mice [Citation17], cells with different differentiation states [Citation18,Citation19], treatment of donor cells with a histone deacetylase inhibitor [Citation20], optimizing oocyte activation methods [Citation21], changing the timing of removal of the metaphase II chromosomes from recipient oocytes [Citation22], or increasing the cell numbers in SCNT embryos through aggregation [Citation23]. However, the cloning efficiency still cannot meet the real demand of the field [Citation15]. Usually, a specific virus is used as a victor to transfect DNA, RNA. Currently, the electroporation to transfect DNA, RNA has become the most popular means [Citation24]. However, this method will cause some damage to the cell membrane under the action of the electric field [Citation25]. During the process of electroporation, cell viability and electro-transfection efficiency (eTE) are determined by several experimental factors [Citation26] including electroporation procedure, cell type/density [Citation27], vector concentration and electroporation buffer properties [Citation28]. For instance, the cleavage rate and blastocyst rate of the reconstituted embryo are affected by the growth status of donor cells [Citation29]. If the donor cells are cultured in vitro for a relatively long period, it will cause oxidative stress and the failure of the recombinant embryos development [Citation30]. To reduce the donor cell oxidative stress and improve the electro-transfection efficiency, many antioxidants have been tested [Citation25,Citation31]. Melatonin (N-acetyl-5-methoxytryptamine, MT) is one of them. When the porcine fetal fibroblasts (PFFs) pretreated with MT are used as donor cells, the cell proliferation and blastocyst rate of the cloned embryos are significantly improved [Citation32].
MT is an indolamine that was originally found to lighten the skin of amphibians [Citation33]. MT is also referred as the chemical signal of dark and originally, it is suggested to be only produced by retinal photoreceptor cells and pinealocytes [Citation34]. Currently, it is found that almost all cells, tissues, and organs, including bone marrow, ovary, eyes, lymphocytes, gastric mucosa, and skin, have the ability to synthesize MT [Citation35].
MT is an amphiphilic substance and can easily cross the cellular membranes. It is a potent-free radical scavenger and antioxidant to protect mitochondria, lipids, protein, and DNA from oxidative stress [Citation36]. Different from the classic antioxidants, MT carries out an antioxidative cascade reaction, i.e. its secondary and tertiary metabolites still have potent antioxidant capacity [Citation37]. It is estimated that one MT molecule can eliminate up to 10 reactive oxygen species (ROS). MT can also participate in regulation of the circadian rhythm in animals. Its secretion levels, which reach the peak at night and become low during the day, are highly synchronized with light/dark cycle and naturally photoperiodic alterations. This characteristic makes MT an important signaling molecule of organisms [Citation38].
MT plays an important role in animal reproduction [Citation38]. It exhibits beneficial effects on nuclear transplantation cloning in many species [Citation39]. However, this effect has not been studied in sheep. In this study, we will test the effects of MT on SCNT embryo development in sheep as well as the potential mechanisms. The results will provide valuable information for the industrial scale of transgenic sheep production in the future.
2. Materials and methods
2.1. Cell viability assay
The sheep fetal fibroblast cells (FFCs) were obtained from the Key Laboratory of Animal Genetics and Breeding of the Ministry of Agricultura. Cell viability was measured by trypan blue staining. Simply, 0.1% trypan blue solution was added to the cell suspension at room temperature for 3–5 mins. Hemocytometer was used to count cells through microscope. The dead cells are light blue and swollen, dull. The living cells are not stained and maintain their normal shape, shiny. A total of 1000 cells were counted, and the cell viability was calculated by the use of the formula. Cell viability (%) = number of unstained cells/total number of cells observed×100%.
2.2. Cell proliferation assay
CCK-8 (Cell Counting Kit-8) Kit (Solarbio, Beijing, CA1210-100) was used to detect cell proliferation following the manufacturer’s instruction. Simply, 10 μL CCK-8 solution was added to the cell suspension and incubated for 2 hours in the incubator. The absorbance was measured at dual wavelength at 450 nm and 600 nm, respectively, with a microplate analyzer with a value greater.
2.3. Electrotransfection
The cells at the logarithmic growth phase were trypsinized and the plasmids were electrotransfected into the cells using an electrotransfer apparatus (Nucleofector II, Lonza, Koln, Germany) following the manufacturer’s instructions. Took 95% of the confluent cells observed under the inverted microscope for digest and centrifuge, and discarded the supernatant. Used the Lonza electroporation kit, added 100 μl electroporation solution and 10 ng GFP plasmid, mixed thoroughly by pipetting and transfer to the electroporation cup; put the electroporation cup into the electroporation instrument, and used A03 for electroporation; when it was done, gently aspirated the mixture in the electroporation cup. The transfected cells were cultured in DMEM/F12 (Gibco, USA, 11,320,033) containing 15% (v:v) FBS (Gibco, USA, 10099141C) for 48 h, then GFP expression was measured under the fluorenes microscopy (LX71; Olympus, Kawasaki, Japan). Samples were analyzed by flow cytometry. (NovoCyte 451,170,928,186; ACEA Biosciences, USA). The distribution of the cell GFP expression was analyzed by software named NovoExpress 1.2.5 .
2.4. Colony formation assay
Flow cytometry was used to measure the colony formation. The single cell with fluorescent signal was sorted into 96-well plates, and each well contained 200 μl of culture medium. After 14 day-culture, when the cell group growth reached 60% confluence, the cells were evaluated under microscope. The result was recorded as a single-cell clone group efficiency calculated by the formula, a single-cell clone group efficiency = the number of cell line/sorting single-cell total.
2.5. Cell cycle analysis
The cell cycle was measured using DNA Content Quantitation Assay (Cell Cycle) (Solarbio, Beijing, CA1510). The collected cells were fixed with pre-cooled 70% ethanol for 24 h, then washed with PBS (Gibco, USA, 10,010,002) twice and stained with PI working solution containing RNase at room temperature for 30 min. Then, the samples were analyzed by flow cytometry. The distribution of the cell cycle was analyzed by software named NovoExpress 1.2.5.
2.6. Apoptotic analysis
FFC cells were collected and stained using an Annexin V-Alexa Flour647/PI (Bioss, China, BA00103) staining kit. Samples were analyzed by flow cytometry. Apoptosis rate was calculated using a software called NovoExpress 1.2.5.
2.7. ROS analysis
FFC cells were collected and stained using a Reactive Oxygen Species Assay Kit (Solarbio, Beijing, CA1410) following the manufacture’s instructions. Samples were analyzed by flow cytometry. ROS level was analyzed by software named NovoExpress 1.2.5.
2.8. Mitochondrial density analysis
MitoTracker Red CMXRos Kit (Thermo Fisher Scientific, USA, M7512) was used for cell mitochondrial level detection. After trypsin digestion of adherent cells, the cells were centrifugated at 300 g for 5 mins at 4°C, washed with PBS (Gibco, USA, 10,010,002), centrifugated again, and discarded the supernatant. The cells was resuspended with MitoTracker Red CMXRos staining working solution, which is preheated at 37°C, and incubated the cells for 15–45 mins under normal cell culture condition. After staining, the cells were collected by centrifugation and the fluorescence intensity was recorded under a fluorescence microscope (LX71; Olympus, Kawasaki, Japan). Mitochondria were analyzed using imageJ software.
2.9. Ovary collection and cumulus–oocyte complex aspiration
Sheep ovaries were collected from local abattoir and transported to the laboratory in sterile saline solution supplemented with Penicillin-Streptomycin-Glutam (100 IU/mL) (Sigma-Aldrich, USA, 10,378,016) at 25–30°C within 3 h after slaughter, and the samples were washed in a sterile saline solution immediately after arrival. COCs were recovered from the ovary with a blade in a culture dish with a 90 mm diameter. Hepes-buffered tissue culture medium-199 (HTCM-199) (Gibco, USA, 11,150,059) supplemented with 0.1% polyvinyl pyrrolidine alcohol (PVA) (Sigma-Aldrich, USA, 341,584), 25 IU/mL heparin (Sigma-Aldrich, USA, H3149), and Penicillin-Streptomycin-Glutam (Sigma-Aldrich, USA, 10,378,016), was used for handling oocytes. Only aspirated COCs with compact and intact cumulus and homogeneous cytoplasm were selected under a stereomicroscope for subsequent experiments.
2.10. In Vitro maturation of oocytes
The tissue culture medium-199 (TCM-199) supplemented with Penicillin-Streptomycin-Glutam (100 IU/mL) (Sigma-Aldrich, USA, 10,378,016) 10% fetal bovine serum (Gibco, USA, 10099141C), and cysteamine (0.1 mM) (Sigma-Aldrich, USA, M9768) was used as the basal culture medium. Maturation medium was modified from basal culture medium by adding 100 ng/mL EGF (Sigma-Aldrich, USAm, SRP3027), 10 μg/mL FSH (Sansheng, China), 10 μg/mL LH (Sansheng, China), and 1 μg/mL estradiol-17β (Sigma-Aldrich, USA, E-060). MT (Sigma-Aldrich, USA, M5250) was dissolved in DMSO (Sigma-Aldrich, USA, 276,855) at concentration 0.1 M and stored at −20°C as the stock solution. COCs were cultured in groups of 90 in 700 μL droplets in maturation medium in 4-well plates at 38.5°C with 5% CO2 in humidified air for 24 h. The matured oocytes were evaluated by stereomicroscope.
2.11. In vitro fertilization (IVF)
Following IVM, COCs were washed three times in IVF medium (Vitrolife, Swedish, 10,136), subsequently placed in 50 μL drops of IVF medium covered with mineral oil. Frozen semen from a ram was used for IVF. A single straw of semen was thawed at 37°C for 30 secs, spermatozoa were centrifuged at 500 g for 10 mins, washed twice. After washing, sperm concentration was adjusted as spermatozoa to 2 × 106/mL. The washed and suspended sperm cells were incubated with mature COCs for 18 h under 5% CO2 in humidified air at 38.5°C.
2.12. Embryo culture (IVC)
18 h after IVF, the samples were washed three times in IVC medium (Vitrolife, Sweden, 10,128). Groups of 15 to 20 zygotes were cultured in 30 μL IVC medium drops for 9 days at 38.5°C in a humidified incubator with 5% O2, 5% CO2, and 90% N2. The day of fertilization was considered as day 0. The stage of embryonic development was evaluated at day 3 and day 9 post-fertilization.
2.13. Somatic cell nuclear transfer and pregnancy diagnosis
The qualified oocytes in the holding medium were transferred to TCM199. After that, the oocytes with cumulus cells were incubated in TCM199 supplemented with 0.1% hyaluronidase (Sigma-Aldrich, USA, H3506) for 5 mins and the cumulus cells were removed by gently pipetting. The denuded oocytes were incubated in TCM199 containing 5 µg/mL Hoechst 33, 342 (Sigma-Aldrich, USA, 14, 533) and 5 µg/mL cytochalasin B (Sigma-Aldrich, USA, C6762) for 10 mins. The first polar body and chromatin plate were removed with an enucleation needle mounted on the micromanipulators (Narishige, Tokyo, Japan, MMN-1) under an inverted fluorescence microscope (Nikon, Tokyo, Japan, C. SHG1). Successful enucleation oocytes were served as the nuclear transplant recipient cytoplasms. The donor cells were trypsinized and an intact donor cell was injected into the perivitelline space and led it to contact with the recipient cytoplasm using micromanipulation. Cytoplast-cell couplets were equilibrated in an electrical fusion solution and were transferred to an electrofusion chamber containing the same medium. Cell fusion was induced by applying two direct current pulses of 2.0 kV/cm for 25 µs using an ECM2001 Electrocell Manipulator (BTX Inc, CA, USA, San Diego). Couplets were removed from the fusion chamber, washed, and incubated in TCM199 supplemented with 10% (v:v) FBS at 38.5°C in a humidified atmosphere of 5% CO2. At 30 mins after electrofusion, couplets’ fusion status was evaluated. If the fusion was not completed between the couplet, the second electrofusion would be applied. After electrical fusion, visual method was used to determine the fusion efficiency.
Then, the fused embryos were incubated in TCM199 containing 10 µg/mL cycloheximide (Sigma-Aldrich, USA, C7698) for 5 mins, and were transferred into TCM199 containing 5 µg/mL cytochalasin B for incubation for 5 h. 4 hours after chemical activation, the surviving reconstructed embryos were activated embryos. The activated embryos were incubated in TCM199 containing 10% (v:v) FBS supplemented with or without 10−7 M MT at 38. 5°C in a humidified atmosphere of 5% CO2 overnight. By adding MT into the development solution of reconstructed embryos, the cleavage efficiency of subsequent SCNT embryos could directly show us MT’s effect of promotion in this process .
2.14. Experimental group
Further studies show us the optimal concentration of MT in cell culture, oocyte and embryo development, help us to choose the optimal concentration for our experiment in those fields [Citation40,Citation41]. The concentrations of MT we choose for the experiment are 10−5, 10−7, 10−9 mol/L. However, there are few studies on the optimal concentration of MT for electroporation transfection, so it is necessary to expand the research range to find out the optimal concentration of melatonin for the area of electroporation transfection. The concentration(s) of MT we choose for the experiment are 10−3, 10−5, 10−7, 10−9, 10−11 mol/L.
In order to reduce random error, experiments were performed 3 times and 20 embryos were analyzed for each group at each stage, all experimental groups in this experiment were from same cell line, and cell-line culture was operated randomly. The experimental group contained more than 105 cells in each test, cultured by 60 mm and 90 mm of petri dishes, showed slow and abnormal growth, and turbid culture medium were excluded from the calculation. Only the lead author knows how they were grouped.
2.15. Statistical analysis
Statistical analyses were performed using the SPSS22.0 software. One-way analysis of variance was used. Results are presented as means ± SEM Significance was accepted at a level of p < 0.05.
3. Results
3.1. The timely dependent growth curve of cultured sheep fetal fibroblasts cells (FFCs)
. a showed that the typical morphology of the sheep FFCs that were adhesive together with a long spindle shape, uniform cell density, and typical fibroblast status. The cells grew vigorously with few heterotic and dead cells. The cell growth curve was made from 3 to 5 generations of FFCs by the methods of visual inspection and hemocytometer cell count (. b). The results showed a typical “S” shape growth curve of these cells (. b) and indicated that these cells are suitable for serving as transgenic donors.
3.2. Effects of MT on the quality of FFCs
FFCs were incubated with varied concentrations of MT at different time points. Cell viability was evaluated with trypan blue and counted by hemocytometer. The results showed that MT at all concentrations (10−5, 10−7and 10−9M) significantly increased cell viability compared with the control group at the different time points (), the proliferation ability of cells was measured at 48 hours after MT treatment with Cell Counting Kit-8. Different concentrations of MT were added to the culture medium of FFCs, and the cells were collected at 1, 2 or 3 days, respectively, after MT treatment. The results indicated that MT at all concentrations (10−5, 10−7and 10−9M) significantly improved the cell proliferation compared with the control group (. a).
Table 1. Effect of different concentrations of MT on cell viability at different culture time points (Cell viability/%)
The flow cytometry was used to test the cloning efficiency of MT on the FFCs after electroporation procedure. The monoclonals were counted at 10 days after different concentrations of MT treatment. The results showed that MT at the concentration of 10−7M significantly increased the cloning efficiency of FFCs after electroporation compared with the control group while MT at the concentrations of 10−5 and 10−9M exhibited no significant effect on this parameter (. b).
Figure 2. The effect of MT treatment on cell proliferation and cloning of FFCs: (a) Cell proliferation was analyzed by CCK8 assay. The value of absorbance was positively related to the number of live cells; (b) The cloning efficiency was measured by flow cytometry. The number of monoclonal cells were counted. Con, control. Values were mean ± standard error of the mean of 3 times independent experiments *P < 0. 05 vs other groups.

3.3. Effects of MT on electrotransferring efficiency of FFCs
The electroporation program A03 was used to electrotransfer the plasmid with GFP fluorescence into the sheep FFCs. The electrotransfected cells were visualized under a fluorescent inverted microscope shown in a–b. The cells with uniform bright green fluorescence suggested that the transfection was successful and the target gene was indicated to integrate into the cell for expression. The flow cytometer measurement confirmed that MT at all tested concentrations greatly improved the electroporation transfection efficiency compared with the group without MT ( c – d). In accordance with the improved transfection efficiency, MT at the concentrations of 10−5, 10−7 and 10−9M also significantly reduced the cell apoptosis which is a side effect of electroporation. Con was the sheep FFCs which was not electrotransfected ().
Figure 3. Effects of MT on electrotransferring efficiency. (a) Photo of positive transfected FFCs at 48 h after electrotransfection, scale bars: 100 μm. (b) Map of plasmid expressing GFP fluorescence. (c) Flow cytometry profiles. The fixed cells were analyzed on a flow cytometer until 20, 000 cells were counted to calculate. (d) The statistical analysis on the data obtain from (c). (f) Flow cytometry profiles. The fixed cells were analyzed on a flow cytometer until 20, 000 cells were counted to calculate. (e) The apoptotic rate; The electro-transfection efficiency distribution was analyzed using Modfit software. The statistical analysis on the data obtain from (f)The data were expressed as mean ± standard error of 3 times independent experiments, * P < 0. 05 vs. Control (electroporation alone group).
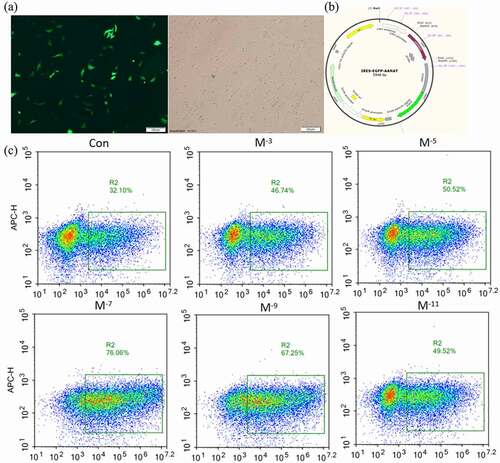
3.4. The effect of MT treatment on cell synchronization at different cell cycle phases
In the study, we found that the electroporation hindered the cell cycle synchronization with less cell entering the G1/G0 phase. However, MT at the concentration of 10−7 and 10−9M normalized the cell cycle which was impeded by the electroporation and allowed more cell entering G1/G0 stage compared with the group without MT while MT in other concentrations had no such effect. In addition, MT at the concentration of 10−5, 10−7, and 10−9M reduced the cells at the S phase compared with the group without MT (.a-b). Con was the sheep FFCs which was not electrotransfected (.a-b)
Figure 4. The effect of MT treatment on FFCs synchronization at different cell cycle phases. (a) DNA histograms, by flow cytometry, the difference in the count collected did not affect the experimental results. (b) Ratio of cells in different cell cycle phases for each group of cultured. Con, control; Data were expressed as e mean ± standard error of the mean of 3 times independent experiments. * P < 0. 05 vs. control group.
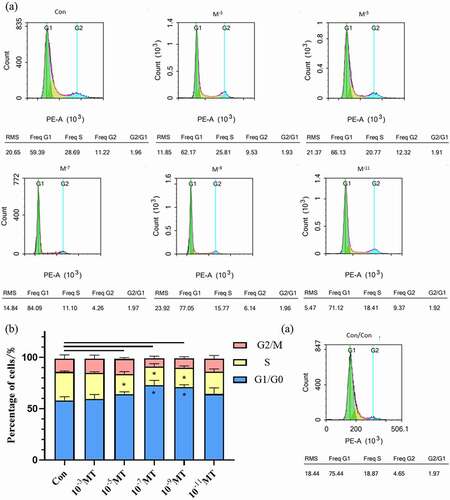
3.5. Effects of MT on ROS production and mitochondrial numbers of FFCs
The fluorescent probe DCFH-DA and flow cytometry were used for staining and measuring the intracellular ROS levels, respectively. The results showed that MT at different concentrations significantly reduced the intracellular ROS levels compared with the group without MT. Among these MT concentrations, the most effective one is 10−7M (. a,b). MitoTracker Red CMXRos was used to measure the mitochondrial density of FFCs. MT at the concentration of 10−5, 10−7, and 10−9M significantly increased the mitochondrial density in the FFCs compared with the group without MT. (. c – d).
Figure 5. Effects of MT on ROS production during electroporation and mitochondrial density. (a) Flow cytometry profiles of intracellular ROS staining. (b) The statistical analysis of the data from A. (c) Representative images of mitochondrial level in FFCs, the right picture scale bars: 100 μm; (d) The statistical analysis of the data from C based on the intracellular relative fluorescence intensity of mitochondria. Data were expressed as mean ± standard error of the mean of 3 times independent experiments. * P < 0. 05 vs. control (electroporation alone group).
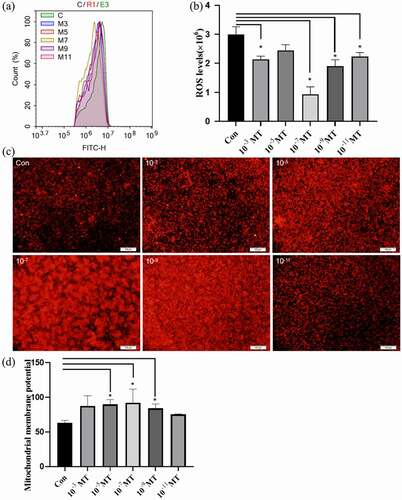
3.6. Effects of MT on the maturation, fertilization, and early embryo development efficiency in cultured sheep oocyte
The collected normal oocytes were cultured in IVM medium with or without MT (10−7M). The 1153 oocytes were the control group and 3245 oocytes were treated with MT. Firstly, we observed that after 24 h MT incubation, the oocytes became maturation, the expansion of cumulus granulosa cells and polar body excretion were all significantly improved compared with the control group (. a-d). Then, the matured oocytes which were previously treated or untreated with MT were fertilized. These embryos were continuously incubated with or without MT. The results indicated that the MT treated embryos derived from the MT treated oocytes (MT+MT) or the embryos derived from MT treated oocytes but without continuously MT treatment (MT+Control) both exhibited significantly higher cleavage rate than that in the Control (oocytes without MT treatment) + Control (embryo without MT treatment) and Control (oocytes without MT treatment) + MT (embryo with MT treatment) groups (. e), while all combinations of MT treated groups had the significantly higher blastocyst rates than that in the control + control group (. f).
Figure 6. Effects of MT on the maturation, fertilization and early embryo development efficiency in cultured of sheep oocytes: (a) The morphology of normal sheep oocyte. The upper and lower two are different experimental groups. The picture on the right is enlarged and observed more clearly, the right picture scale bars: 200 μm; (b) Expansion of cumulus cell after in vitro maturation. The picture on the right is enlarged and observed more clearly, the right picture scale bars: 200 μm; (c) Photo of polar body excretion. The left picture enlarges the polar body, the right picture of scale bars: 200 μm; (d) The statistical analysis of the data obtained from C; (e) The cleavage rate of sheep oocytes after in vitro fertilization; (f) The blastocyst rate of sheep oocytes after in vitro fertilization, MT/CON before “+“ means adding MT/control group during IVM, MT/CON after ”+” means adding MT/control group during IVC. Data were expressed as mean ± standard error of the mean of 3 times oocytes or embryos. * P < 0. 05 vs. respective groups.

3.7. Effects of MT on SCNT embryo development
When MT was added during the process of SCNT followed by electrofusion the fusion rate was significantly increased compared with the control group (. a) and the embryo activation rate was also enhanced but it failed to reach the statistical significance (. b). Most importantly, MT treatments with different combinations including MT + MT, MT + Control and Control + MT significantly promoted the cleavage rate at the different degrees compared with the control + control group (. c).
Figure 7. Effects of MT on SCNT embryo development: (a) The fusion rate of sheep embryo after SCNT; (b) The stimulating rate of sheep embryo after SCNT; (c) The Cleaved rate of sheep embryo after SCNT, MT: MT. Before “+” indicated the IVM stage and after “+” indicated IVC stage. Data were expressed as mean ± standard error of the mean of 3 times samples. * P < 0. 05 vs. respective groups.** P < 0. 01 vs. respective groups.

4. Discussion
SCNT is an important method used to generate transgenic animals. The method can be used for medical studies, agricultural animal production or development of other biological resource. It is also an important tool for improvement of food quality and meat production. However, the relatively low yield efficiency of these methods limits their industrial-scale utility and to improve their yield efficiency becomes the primary task of researchers [Citation42]. The purpose of current study attempts to modify the current method and thus, to improve the yield efficiency of transgenic animal process. Based on our previous studies, we focus on the effects of MT on the SCNT embryo development after electroporation. It is well documented that SCNT and electroporation cause embryo oxidative stress and hinders its development [Citation43,Citation44]. MT plays a role as a potent antioxidant that may overcome these shortcomings and promote the efficiency of transgenic animal yield.
MT not only directly scavenges the reactive oxygen species (ROS) but also improves the activities of several antioxidant enzymes as well as their gene expressions [Citation45]. These include glutathione peroxidase (GSH-Px), glutathione reductase (GSH-RD), catalase (CAT), superoxide dismutase (SOD), and glucose-6-phosphate dehydrogenase (G6PD) [Citation36]. In addition, MT can also inhibit the activities of some prooxidant enzymes, such as inducible nitric oxide synthase (iNOS), xanthine oxidase (XO), and HO-1 [Citation46]. In this study, a variety of MT concentrations (10−3 to 10−11M) were selected to test their effects on sheep FFCs, oocytes, and the embryo development. This selection is based on the previous study which shows that melatonin serum levels in different breeds of sheep are around 20–200 pg/ml, that is, 9 × 10−8 to 9 × 10−7M [Citation47]. Thus, the melatonin 10−7M used in this study is considered as the physiological concentration.
It is generally believed that the status of donor cells is important in the subsequent embryonic development of SCNT, in which the cell cycle stage of donor cells is the key factor. The first SCNT animal (Dolly) was produced by using a G0 phase donor cell [Citation4]. When the nuclei of donor cells at G0 [Citation47] or G1 [Citation48] phase are transplanted into enucleated metaphase II (MII) oocytes, it will increase the transgenic efficiency. The commonly used methods for synchronization at G0/G1 phase of the cell cycle are serum starvation [Citation49] and contact inhibition [Citation50]. In this study, MT was found to increase the G1 phase and decrease S phase during the electrotransfection process. In this study, contrary to MT’s effect of promoting proliferation, it was found that MT resulted in cells’ increase in G1 phase and decrease in S phase in electrotransfection. we have known that the shock made the cell cycle abnormal. This result may be caused by cell damage and cell-line difference during electric transfer, and by MT’s important role played in making the MT-treated cell cycle more similar to those unshocked cells compared with the control group. This is a novel mechanism that melatonin increases the transgenic efficiency by synchronization of G0/G1 phase of the cell cycle.
During the process of transgenic animal generation, after the homologous recombination and clonal selection, a considerably large quantity of transfected cells are required for recombination analysis as well as cryopreservation, which will be used for subsequent SCNT experiments [Citation43]. Thus, improving the proliferation of the transfected cells is a key step to increase the transgenic efficiency [Citation51]. It has been well known that the medium composition is critical for transfected cell proliferation. In this study, different concentrations of MT were added into the culture medium. To test the effects of MT on proliferation of the transfected cells, the plasmid encoding green fluorescent protein was used as the indicator. We found that adding MT to the electroporation solution significantly increased the electroporation efficiency. The mechanistic study indicates that MT reduces the cellular oxidative stress and apoptosis caused by the electroporation [Citation52,Citation53] and also increases the numbers of mitochondria in transfected cells. Studies have shown that ROS levels in synchronized cells are significantly reduced, and indications ROS is related to cell proliferation [Citation54]. Excessive concentration of melatonin takes a negative effect on cells, while the concentration could be too low to eliminate the effects of oxidative stress. More mitochondria proliferation indicates more energy supply for the cell proliferation. All of these positively contribute to the MT’s effects on the increased in transfected cells.
MT is presented in follicular fluid of bovine [Citation55] and swine [Citation56]. This suggests the regulatory action of MT on oocyte maturation in the in vivo condition. Several studies have shown that MT supplementation promotes oocyte maturation in vitro and improves oocyte development in different species including human, mouse, bovine, and swine [Citation57–59,Citation60]. This is consistent with our observation in which, MT at the concentration of 10−7M (which is considered as the physiological concentration) promoted sheep oocyte maturation as well as the development of both cloned and fertilized embryos in vitro. As we have realized that the beneficial results of melatonin on the development of sheep transgenic cloned embryos are from the in vitro study. Whether these beneficial effects can be repeated in vivo condition is not clear since both have different microenvironments, which decide the embryo developments greatly. Our next study is to explore the effects of melatonin treatment on the in vivo embryo developments.
In conclusion, MT in different concentrations, especially in the physiological concentration of 10−7M has the capacity to promote the donor cell viability, transfected cell proliferation, monoclonal efficiency and the electrotransfer efficiency in sheep FFCs. The mechanisms are multiple including synchronization of cell division cycle, reduction of cellular oxidative stress and apoptosis and increase in mitochondrial number and function. All of these render MT’s ability increase the efficiency of animal transgenic technology such as SCNT and electroporation. This has been finally manifested by the increased cleavage rate and blastocyst rate of the transfected embryos under the physiological concentration of MT treatment. MT is easily available with low cost and the method is simple. Our future project is to use this method in the in vivo situation for generation of transgenic sheep.
Institutional review board statement
All experimental protocols concerning the handling of animals were performed in accordance with the requirements of the Institutional Animal Care and Use Committee at the China Agricultural University (permission number: AW20151203-01).
Author contributions
Y.Y., G.L., S.D., L.Z. and G.L. conceived and designed the experiments; H.W., H.Y., W.M., D.L., Y.F., T.Z. and P.J. performed the experiments; X.T. and Z.L. contributed the materials and executed the work in the experiment; Y.Y. wrote the manuscript and Y.Y. and A.Y. analyzed the data. All the authors approved the final manuscript. All authors have read and agreed to the published version of the manuscript.
Disclosure statement
No potential conflict of interest was reported by the author(s).
Data availability statement
The data used to support the findings of this study are available from the corresponding author upon request.
Additional information
Funding
References
- Challa AK, Stanford D, Allen A, et al. Validation of gene editing efficiency with CRISPR-Cas9 system directly in rat zygotes using electroporation mediated delivery and embryo culture[J]. MethodsX. 2021;8:101419.
- Ashry M, Yang C, Rajput SK, et al. Follistatin supplementation induces changes in CDX2 CpG methylation and improves in vitro development of bovine SCNT preimplantation embryos[J]. Reprod Biol Endocrinol. 2021;19(1):141.
- Kruk J, Aboul-Enein BH, Duchnik E. Exercise-induced oxidative stress and melatonin supplementation: current evidence[J]. J Physiol Sci. 2021;71(1):27.
- Campbell KHS, McWhir J, Ritchie WA, et al. Sheep cloned by nuclear transfer from a cultured cell line. Nature. 1996;380(6569):64–66.
- Wilmut I, Schnieke AE, McWhir J, et al. Viable offspring derived from fetal and adult mammalian cells. Cloning Stem Cells. 2007;9(1):3–7.
- Schnieke AE, Kind AJ, Ritchie WA, et al. Human factor IX transgenic sheep produced by transfer of nuclei from transfected fetal fibroblasts. Science. 1997;278(5346):2130–2133.
- Menchaca A, Dos Santos-neto PC, Mulet AP, et al. CRISPR in livestock: from editing to printing[J]. Theriogenology. 2020;150:247–254.
- Keefer CL. Artificial cloning of domestic animals[J]. Proc Natl Acad Sci U S A. 2015;112(29):8874–8878.
- Yoo JG, Kim B-W, Park M-R, et al. Influences of somatic donor cell sex on in vitro and in vivo embryo development following somatic cell nuclear transfer in pigs[J]. Asian-Australas J Anim Sci. 2017;30(4):585–592.
- Thuan NV, Kishigami S, Wakayama T. How to improve the success rate of mouse cloning technology. Jpn J Anim Reprod. 2010;56(1):20.
- Wakayama T, Yanagimachi R. Mouse cloning with nucleus donor cells of different age and type. Molecular Reproduction and Development. 2001;58(4):376–383.
- Hiiragi T, Solter D. Reprogramming is essential in nuclear transfer. Mol Reprod Dev. 2005;70(4):417–421.
- Amano T, Tani T, Kato Y, et al. Mouse cloned from embryonic stem (ES) cells synchronized in metaphase with nocodazole. J. Exp. Zool. 2001;289(2):139–145.
- Tani T, Kato Y, Tsunoda Y. Reprogramming of bovine somatic cell nuclei is not directly regulated by maturation promoting factor or mitogen-activated protein kinase activity. Biol Reprod. 2003;69(6):1890–1894.
- Li Z, Shi J, Liu D, et al. Effects of donor fibroblast cell type and transferred cloned embryo number on the efficiency of pig cloning. Cell Reprogram. 2013;15(1):35–42.
- Pan S, Chen W, Liu X, et al. Application of a novel population of multipotent stem cells derived from skin fibroblasts as donor cells in bovine SCNT. Plos One. 2015;10(1):e0114423.
- Saito M, Saga A, Matsuoka H. Production of a cloned mouse by nuclear transfer from a fetal fibroblast cell of a mouse closed colony strain. Exp Anim. 2004;53(5):467.
- Mizutani E, Ohta H, Kishigami S, et al. Developmental ability of cloned embryos from neural stem cells. Reproduction. 2006;132(6):849–857.
- Inoue K, Ogonuki N, Miki H, et al. Inefficient reprogramming of the hematopoietic stem cell genome following nuclear transfer. J Cell Sci. 2006;119(10):1985–1991.
- Zhao J, Hao Y, Ross JW, et al. Histone deacetylase inhibitors improve in vitro and in vivo developmental competence of somatic cell nuclear transfer porcine embryos. Cell Reprogram. 2010;12(1):75–83.
- Wakayama T, Yanagimachi R. Effect of cytokinesis inhibitors, DMSO and the timing of oocyte activation on mouse cloning using cumulus cell nuclei. Reproduction. 2001;122(1):49–60.
- Wakayama S, Cibelli JB, Wakayama T. Effect of timing of the removal of oocyte chromosomes before or after injection of somatic nucleus on development of NT embryos. Cloning and Stem Cells. 2003;5(3):181–189.
- Boiani M, Eckardt S, Leu NA, et al. Pluripotency deficit in clones overcome by clone-clone aggregation: epigenetic complementation? Embo J. 2003;22(19):5304–5312.
- Roth TL, Puig-Saus C, Yu R, et al. Reprogramming human T cell function and specificity with non-viral genome targeting. Nature. 2018;559(7714):405–409.
- Igawa K, Ohara N, Kawakubo A, et al. D-glucosamine promotes transfection efficiency during electroporation. Biomed Res Int. 2014;2014:485867.
- Zhang G, Sun QY, Chen DY. In vitro development of mouse somatic nuclear transfer embryos: effects of donorcell passages and electrofusion. Zygote. 2008;16(3):223–227.
- Lei L, Liu Z-H, Wang H, et al. The effects of different donor cells and passages on development of reconstructed embryos. Yi Chuan Xue Bao. 2003;30(3):215–220.
- Sherba JJ, Hogquist S, Lin H, et al. The effects of electroporation buffer composition on cell viability and electro-transfection efficiency. Sci Rep. 2020;10(1):3053.
- Roh S, Shim H, Hwang W-S, et al. In vitro development of green fluorescent protein (GFP) transgenic bovine embryos after nuclear transfer using different cell cycles and passages of fetal fibroblasts. Reprod Fertil Dev. 2000;12(1–2):1–6.
- Hill JR, Winger QA, Long CR, et al. Development rates of male bovine nuclear transfer embryos derived from adult and fetal cells. Biol Reprod. 2000;62(5):1135–1140.
- Markelc B, Tevz C, Cemazar M, et al. Muscle gene electrotransfer is increased by the antioxidant tempol in mice. Gene Ther. 2012;19(3):312–320.
- Pang YW, An L, Wang P, et al. Treatment of porcine donor cells and reconstructed embryos with the antioxidant melatonin enhances cloning efficiency. J Pineal Res. 2013;54(4):389–397.
- Lerner AB, Case JD. Pigment cell regulatory factors. J Invest Dermatol. 1959;32(2, Part 2):211–221.
- Wiechmann AF, Sherry DM. Role of melatonin and its receptors in the vertebrate retina. Int Rev Cell Mol Biol. 2013;300:211–242.
- Acuña-Castroviejo D, Escames G, Venegas C, et al. Extrapineal melatonin: sources, regulation, and potential functions. Cell Mol Life Sci. 2014;71(16):2997–3025.
- Abbaszadeh A, Haddadi GH, Haddadi Z. Melatonin role in ameliorating radiation-induced skin damage: from theory to practice (A review of literature). J Biomed Phys Eng. 2017;7(2):127–136.
- Tan D-X, Manchester L, Esteban-Zubero E, et al. Melatonin as a potent and inducible endogenous antioxidant: synthesis and metabolism. Molecules. 2015;20(10):18886–18906.
- Dragojevic Dikic S, Jovanovic AM, Dikic S, et al. Melatonin: a ”Higgs boson” in human reproduction. Gynecol Endocrinol. 2015;31(2):92–101.
- Qu P, Shen C, Du Y, et al. Melatonin Protects Rabbit Somatic Cell Nuclear Transfer (SCNT) Embryos from Electrofusion Damage. Sci Rep. 2020;10(1):2186.
- An Q, Peng W, Cheng Y, et al. Melatonin supplementation during in vitro maturation of oocyte enhances subsequent development of bovine cloned embryos. J Cell Physiol. 2019;234(10):17370–17381.
- He C, Wang J, Zhang Z, et al. Mitochondria synthesize melatonin to ameliorate its function and improve mice oocyte’s quality under in vitro conditions. Int J Mol Sci. 2016;17(6):939.
- Mizutani E, Wakayama S, Wakayama T. Treatment of donor cell/embryo with different approaches to improve development after nuclear transfer. Methods Mol Biol. 2015;1222:101–111.
- Hwang IS, Bae HK, Cheong HT. Mitochondrial and DNA damage in bovine somatic cell nuclear transfer embryos. J Vet Sci. 2013;14(3):235–240.
- You J, Kim J, Lim J, et al. Anthocyanin stimulates in vitro development of cloned pig embryos by increasing the intracellular glutathione level and inhibiting reactive oxygen species. Theriogenology. 2010;74(5):777–785.
- Galano A, Tan DX, Reiter RJ. Melatonin: a versatile protector against oxidative DNA damage. Molecules. 2018;23(3):530.
- Reiter RJ, Mayo JC, Tan DX, et al. Melatonin as an antioxidant: under promises but over delivers. J Pineal Res. 2016;61(3):253–78.
- Tao J, Yang M, Wu H, et al. Effects of AANAT overexpression on the inflammatory responses and autophagy activity in the cellular and transgenic animal levels. Autophagy. 2018;14(11):1850–1869.
- Wells DN, Misica PM, Tervit HR. Production of cloned calves following nuclear transfer with cultured adult mural granulosa cells. Biol Reprod. 1999;60(4):996–1005.
- Zou X, Wang Y, Cheng Y, et al. Generation of cloned goats (Capra hircus) from transfected foetal fibroblast cells, the effect of donor cell cycle. Mol Reprod Dev. 2002;61(2):164–172.
- Yin XJ, Lee H-S, Kim L-H, et al. Effect of serum starvation on the efficiency of nuclear transfer using odd-eyed white cat fibroblasts. Theriogenology. 2007;67(4):816–823.
- Sun, Xiuzhu X, Wang S, et al. Cell-cycle synchronization of fibroblasts derived from transgenic cloned cattle ear skin: effects of serum starvation, roscovitine and contact inhibition. Zygote. 2008;16(2):111–116.
- Brielmeier M, Polack A, Bornkamm GW, et al. Improving stable transfection efficiency: antioxidants dramatically improve the outgrowth of clones under dominant marker selection. Nucleic Acids Res. 1998;26(9):2082–2085.
- Meraviglia V, Azzimato V, Colussi C, et al. Acetylation mediates Cx43 reduction caused by electrical stimulation. J Mol Cell Cardiol. 2015;87:54–64.
- Yoo J, Lee E, Kim HY, et al. Electromagnetized gold nanoparticles mediate direct lineage reprogramming into induced dopamine neurons in vivo for Parkinson’s disease therapy. Nat Nanotechnol. 2017;12(10):1006–1014.
- Du X, Fu X, Yao K, et al. Bcl-2 delays cell cycle through mitochondrial ATP and ROS. Cell Cycle. 2017;16(7):707–713.
- Tian X, Wang F, He C, et al. Beneficial effects of melatonin on bovine oocytes maturation: a mechanistic approach. J Pineal Res. 2014;57(3):239–247.
- Shi JM, Tian XZ, Zhou GB, et al. Melatonin exists in porcine follicular fluid and improves in vitro maturation and parthenogenetic development of porcine oocytes. J Pineal Res. 2009;47(4):318–323.
- Lin T, Lee JE, Kang JW, et al. Melatonin supplementation during prolonged in vitro maturation improves the quality and development of poor-quality porcine oocytes via anti-oxidative and anti-apoptotic effects. Mol Reprod Dev. 2018;85(8–9):665–681.
- Mondal P, Hasan KN, Pal PK, et al. Influences of exogenous melatonin on the oocyte growth and oxidative status of ovary during different reproductive phases of an annual cycle in carp Catla catla. Theriogenology. 2017;87:349–359.
- Park HJ, Park J-Y, Kim J-W, et al. Melatonin improves the meiotic maturation of porcine oocytes by reducing endoplasmic reticulum stress during in vitro maturation. J Pineal Res. 2018;64(2):e12458.