ABSTRACT
Epidermal growth factor (EGF) has many important biological functions. It plays an important role in regulating the growth, survival, migration, apoptosis, proliferation, and differentiation of intestinal tissues and cells. However, until now, the effect of inflammation on the biological activity of EGF in intestinal cells or tissues is still unclear. For this reason, in the current research, we have conducted a detailed study on this issue. Using the rat small intestinal crypt epithelial cell line (IEC6) was used as an in vitro model, and Confocal laser scanning microscope (CLSM), Flow cytometry (FCM), Indirect immunofluorescence assay (IFA), Western-blotting (WB), and Quantitative real-time RT-PCR (QRT-PCR) methods were used to explore the effects of inflammation on EGF/EGFR biological activity and signal transduction profiles. We found that the EGF/EGFR nuclear signal almost disappeared in the inflammatory state, and the phosphorylation levels of EGFR, AKT, and STAT3 were all significantly down-regulated. In addition, we also studied the effect of β-carotene on the biological activity of EGF, and found that when cells were pretreated with β-carotene, the cellular behavior, biological activity, and nuclear signal of EGF/EGFR under inflammation stimulation were partially restored. In summary, the current study shows that inflammation can disrupt EGF/EGFR-mediated signaling in IEC6 cells, suggesting that inflammation negatively regulates the biological activity of EGF/EGFR. Furthermore, we found that β-carotene not only attenuated lipopolysaccharide (LPS)-induced inflammation but also partially restored the biological activity of EGF in IEC6 cells, laying a solid foundation for studying the biological functions of EGF and β-carotene.
KEYWORDS:
1. Introduction
Epidermal growth factor (EGF) is a prototype and founding member of the epidermis growth factor receptor (EGFR) ligand family and was first discovered in 1962 in jaw gland extract [Citation1]. Its structure consists of three disulfur bridges, containing 53 amino acids, which is an effective cell protection peptide. It has important regulatory effect on intestinal mucosa [Citation2]. As an effective filamentous growth factor, EGF is involved in the process of cell growth, differentiation, proliferation, metabolism, and tumor occurrence [Citation3]. EGFR is a typical trans-membrane receptor, which starts the signal cascade through the dimerization stimulated by EGF or other ligands, and activates various signal pathways mediating cell proliferation, migration, invasion and metastasis. The main signal pathways include MAPK (Mitogen-activated protein kinase), PI3K/Akt (Phosphatidylinositol-3-kinase/protein kinase B) and JAK/STAT (Janus kinase/Signal tranfducersand activators of transcription), which exhibit the important biological functions in early embryonic development, skin Liver, and intestine cell renewal [Citation4,Citation5].
EGF/EGFR plays an important biological role in enhancing intestinal immune function. Torres et al. reported that EGF supplementation throughout lactation will enhance the immune system function of the whole body and intestine [Citation6]. Grases et al. reported that EGF can increase intestinal permeability and epithelial barrier integrity in rats [Citation7]. EGF/EGFR also plays an important role in promoting cell proliferation and anti-apoptosis. Klingensmith et al. reported that EGF can reduce the apoptosis of mouse intestinal epithelial cells by reducing the ratio of Bax/Bcl-2 [Citation8]. Suzuki et al. reported that EGF can promote the proliferation and reduce the apoptosis of immature cells in mice [Citation9]. Dominguez et al. found that overexpression of epidermal growth factor in mouse intestinal cells could prevent pneumonia-induced apoptosis of intestinal epithelial cells [Citation10]. Furthermore, Clark et al. demonstrated that EGF/EGFR can also protect the intestinal integrity of septic mice [Citation11]. Previous study has showed that that EGF has significant nutritional effects on the stomach, small intestine, and colon, indicated EGF plays an extremely important role in maintaining intestinal health [Citation12].
Intestinal diseases have been affecting animal intestinal health, and intestinal injury caused by inflammation is the most common [Citation13]. Inflammation is the bodys response to tissue damage, which is mainly caused by tissue damage and microbial/virus infection, such as immune response induced by cell wall proteoglycan, pathogenic nucleic acid and toxin [Citation14,Citation15]. NF-κB, JAK/STAT, and MAPK are signaling pathways related to inflammation [Citation16–18]. The effect of inflammation on EGF/EGFR gene and protein expression in the intestine has also been reported in recent years. Cheng et al. found that the expression urokinase-type Plas-minogen Activator (uPA, PLAU) and its receptor(uPAR, PLAUR) was significantly increased in living tissues of mice with inflammatory bowel disease (IBD) and when the lack of uPAR in intestinal epithelial cells led to enhanced EGF/EGFR signaling [Citation19]. Tang et al. reported that EGFR protein expression was significantly downregulated in the gastrointestinal tissue of capsaicin-treated mice [Citation20]. However, the effect of inflammation on EGF/EGFR biological activity and signaling pathways in intestinal cells remains unclear.
β-carotene is one of the most abundant natural pigments in food. It exists in a large number of fruits and vegetables and is widely used as antioxidant and natural colorant in food [Citation21]. It has antioxidant and anti-inflammatory activities, and is involved in protecting animals from free radicals and singlet oxygen active substances. It has been reported that β-carotene can block the nuclear translocation of NF-κB to alleviates inflammation [Citation22]. Previous research from our team showed that β-carotene may play a relieve inflammation role by regulating JAK2/STAT3 and JNK/p38 MAPK signaling pathway [Citation23].
However, the effect of inflammation on EGF/EGFR bioactivity and signaling in intestinal cells has remained unknown until now. To this end, in this current study, we modeled cellular inflammation with LPS-stimulated IEC6 cells and found that LPS-induced inflammation inhibits EGF/EGFR signaling and intracellular transport. Next, we evaluated the effect of β-carotene on EGF activity in the LPS-induced inflammation model of IEC6 cells. We found that the biological function of EGF/EGFR was partially restored by β-carotene treatment. Based on the fact that β-carotene is anti-inflammatory, we speculate that β-carotene may partially restore the biological function of EGF/EGFR by inhibiting the inflammatory process, laying a theoretical foundation for studying the biological functions of β-carotene and EGF. More in vivo and ex-vivo experiments are needed to further explore and study the mechanism of action. It is also interesting that further experiments showed that EGF and β-carotene have synergistic effects on anti-inflammation and anti-apoptosis.
2. Materials and methods
2.1 Antibodies and reagents
Lipopolysaccharide (LPS), β-carotene (purity > 93%), Hoechst 33,258, 4% paraformaldehyde, Triton X-100, and FITC were obtained from Sigma-Aldrich (USA). EGF was purchased from Peprotech (Rocky Hill, NJ, USA). RPMI 1640, fetal bovine serum (FBS), Trypsin/EDTA and antibiotics (Penicillin-Streptomycin for cell Culture) were from GIBCO (USA). The BCA kits, Cell lysis buffer (RIPA kit), Enhanced chemiluminescence (ECL), and Difco skim milk were obtained from Pierce (USA). The IL-10, TGF-β1, TNF-α, and IL-1β ELISA kits were purchased from R&D Systems (Minneapolis, MN, USA). Anti-EGFR (catalog no. ab52894, 1:500 dilution), anti-phospho-EGFR (catalog no. ab32086, 1:1,000 dilution), anti-STAT3 (catalog no. ab68153, 1:1,000 dilution) and anti-phospho-STAT3 (catalog no. ab267373, 1:1,000 dilution) were purchased from Abcam (Cambridge, UK).The primary antibodies against AKT (catalog no. 4691, 1:1,000 dilution), P-AKT (catalog no. 4060, 1:2,000 dilution), Bax (catalog no. A20227, 1:1,000 dilution), Bcl-2 (catalog no. A19693, 1:500 dilution), Caspase3 (catalog no. A11021, 1:1500 dilution), Caspase9 (catalog no. A11910, 1:500 dilution), p53 (catalog no. A19583, 1:1,000 dilution), Fas (catalog no. A19582, 1:1,000 dilution), β-actin (catalog no. AC026, 1:50,000 dilution), and the secondary antibody Goat Anti-Rabbit IgG/HRP (catalog no. AS014, 1:2,000 dilution) used in Western blot analyses were all purchased from Cell Signaling Technology (USA). Unless otherwise stated, other reagents were obtained from Sigma-Aldrich (USA).
2.2. Cell culture
Rat small intestinal crypt epithelial cell line (IEC-6) was stored in our laboratory [Citation24]. IEC-6 cells were cultured in RPMI-1640 medium supplemented with 10% FBS, streptomycin (0.05 mg/mL), and penicillin (0.1 mg/mL) (Biofluids, Rockville, MD, USA), and maintained at 37°C in a humidified atmosphere of 5% CO2.
2.3. MTT assay
Cell proliferation was evaluated using MTT analysis. IEC6 cells were seeded into 96 well plates (1 × 105/well) and cultured in RPMI 1640 with 10% FBS and 1% antibiotics for 24 hours. The cells were treated with EGF at concentrations of 0, 20, 40, 60, 80, 100 ng/mL and incubated for 24 hours. After incubation, 100 μL MTT solution (1 mg/mL) was added to each well and incubated in 5% CO2 at 37°C incubated for After incubation, the supernatant was removed and 100 μL dimethy sulfoxide (DMSO) was added to each well and incubated for 15 minutes to dissolve the crystals, the optical density (OD) was measured at 490 nm by Microplate reader (Thermo Scientific, multiskan FC). The experiment was repeated three times.
Effect of LPS stimulation on EGF-induced proliferation. IEC6 cells were inoculated on 96 well plates (1 × 105/well), cultured in RPMI 1640 with 10% FBS for 24 hours. Treatment with EGF (40 ng/mL) or β-carotene (50 μmol/L) in the presence of LPS (l00 ng/mL) for 24 hours. After incubation, optical density (OD) was measured at 490 nm by Microplate reader (Thermo Scientific, multiskan FC). The experiment was repeated three times.
2.4 Indirect immunofluorescence assay (IFA)
Evaluate the expression of EGFR in IEC6 cells through indirect immunofluorescence assay (IFA). The IEC6 cells were cultured in serum-free medium for 2 h. The cells were washed three times with PBS and stimulated with EGF for 45 minutes. After washing three times, the cells were fixed with 4% paraformaldehyde for 30 minutes. Then, seal with 5% skimmed milk powder for 1 hour. The cells were washed three times with PBS and treated with anti-EGFR antibody overnight at 4°C. Wash the cells and incubate with fluorescent-conjugated secondary antibody for 1 h at 37°C in the dark. After washing three times with PBS for 5 minutes, the cells were observed using a confocal laser scanning microscope (CLSM, Olympus FV1200). The values shown were measured intriplicate and are representative of three independent experiments.
2.5 Confocal Laser Scanning Microscopy (CLSM)
IEC6 cells were seeded on a 6-well plate (1 × 105 /well) and cultured with RPMI 1640 supplemented with 10% FBS and 1% antibiotic. After treatment with EGF (40 ng/mL) or β-carotene (50 μmol/L) in the presence of LPS (l00 ng/mL) for 24 h. The detail grouping was described in the figure legends. When the cells reach 80% confluence, the cell were washed and incubated with FITC-EGF for 0–90 min. The cells were washed with PBS for 3 times for 5 minutes each time. The cells were then fixed with 4% paraformaldehyde for 20 min. After washing, the cells were washed with PBS for 3 times, then stained with Hoechst for 15 min. After final three washes with PBS, the cells were with 50% glycerol. The cell samples were observed by laser confocal microscope (CLSM, Olympus FV 3000). The values shown were measured intriplicate and are representative of three independent experiments.
2.6 Flow cytometry (FCM)
The apoptosis rate of IEC-6 cells was detected by flow cytometry. After treatment with EGF (40 ng/mL) or β-carotene (50 μmol/L) in the presence of LPS (l00 ng/mL) for 24 h. The specific grouping was described in the figure legends. After incubation for 24 h, the cells were collected with fluorescein isothiocyanate (FITC)-conjugated annexin V at 4 conjugated annexinugated annexinescein is othiocyanatetes before the computer test. Finally, the cell suspension was movinto the tube and immediately detected by flow cytometry. The values shown were measured intriplicate and are representative of three independent experiments.
2.7 Enzyme Linked Immune Sorbent Assay (ELISA)
The IEC-6 cells (1 × 105/well) were seeded into a 6-well plate and incubated under different conditions. Detailed grouping was described in the figure legends. The cell culture supernatant was collected to determine the secretion. According to the ELISA kit instructions, the secretion of TNF-α, IL-10, IL-6, and TGF-β1 in IEC6 cells was determined. The experiment was repeated three times.
2.8 Quantitative real-time RT-PCR (QRT-PCR)
After the IEC6 cells were grouped and incubated as shown in the figure legends. After incubation for 24 h, the total RNA of each group of cells was extracted and purified with Trizol reagent according to the protocol provided by the manufacturer, and then the RNA sample was reverse transcribed into cDNA with primescript RT Kit (Takara). Real-time polymerase-chain reaction was performed in SYBR premix ex Taq II (tlirnasheh plus) (Takara). The experiment was repeated three times. The primer sequences used are as .
Table 1. Primers used for Quantitative Reverse Transcription PCR.
2.9 Western Blot Analysis (WB)
After the IEC6 cells were treated as indicated in the figure legends. The cells in the 6-well plate were washed with cold PBS for 3 times, and then the IEC6 cells were lysed with the Ripa lysis buffer. BCA protein detection kit was used to determine the protein concentration of each group. The protein samples were separated by 10% SDS-PAGE gel and transferred to the PVDF membrane. After blocking with 5% skimmed milk powder for 1 hour at 37°C, incubate with the designated primary antibody at 4°C overnight; then wash 5 times with TBST for 5 minutes each, then incubate with IgG-HRP goat anti-rabbit IgG at 37°C for 1 hour, and finally use TBST Wash 5 times for 5 minutes each time, and use chemiluminescence kit to develop PVDF membrane. The values shown were measured intriplicate and are representative of three independent experiments.
2.10 Statistical Analysis
All data are represented as mean values ± standard deviation (SD). Statistical analysis was performed using ANOVA and Duncan’s multiple comparisons test with of SPSS statistics 18 (SPSS, Chicago, Illinois, USA). p-value less than 0.05 was considered statistically significant. Different letters indicate a significant difference (P< 0.05). The values shown were measured intriplicate and are representative of three independent experiments. Same letters are statistically similar.
3. Results
3.1 Presence of epidermal growth factor receptor on IEC6 cells
Here, We firstly evaluated the EGFR expression on IEC6 cells using IFA. The anti-EGFR was used to detect the EGFR expression. As shown in , it can be found that that EGFR mainly localized plasma membrane of IEC6 cell, and a small amount of fluorescent signal was found to be located in the cytoplasm. Thus, indicating that EGFR was also expressed in the cytoplasm. The control antibody show little or no fluorescent signal (data not shown).
3.2 EGF has a proliferative effect on IEC6 cells
The proliferation effect of EGF on IEC6 cells was measured by MTT assay. The growth of IEC6 cells is shown in . The results shown in indicated that after EGF treatment for 24 hours, compared with the control group, the number of cells in the 20 ng/mL, 40 ng/mL, 60 ng/mL, 80 ng/mL and 100 ng/mL epidermal growth factor groups increased significantly (p< 0.05). Therefore, 40 ng/mL EGF and cells cultured for 24 hours were selected for subsequent experiments. In order to explore the effect of LPS on the proliferation of EGF, IEC6 cells were treated with 40 ng/mL EGF and 100 ng/mL LPS for 24 hours, as shown in . The results show that inflammation inhibits the proliferation of cells by EGF under LPS stimulation, the effect of EGF on cell proliferation was restored by β-carotene. In addition, β-carotene has no significant effect on cell proliferation.
Figure 2. Effects of EGF on IEC6 cell growth.
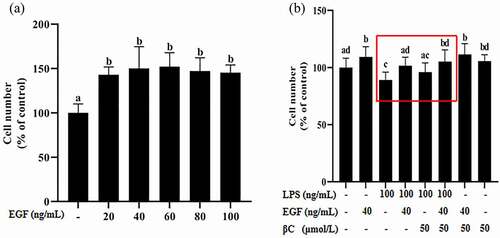
3.3 Inflammation inhibits EGF/EGFR internalization in IEC6 cells
To investigate the internalization kinetics of EGF in IEC6 cell, the cells were incubated with FITC-EGF for the indicated time points (0–90 min). After incubation, the cell samples were observed by CLSM, as shown in , it can be seen that EGF internalized into the IEC6 cells with a time-dependent manner. At 0–5 min, EGF mainly localized in the cell membrane; at 5–15 min, FITC-EGF (green signal) mainly localized in the cytoplasm; 30 min after EGF stimulation, the fluorescence signal within the cell reaches the maximum, after which, the fluorescent signal inside the cell started to decline.
Figure 3. Analysis of EGF/EGFR internalization kinetics in IEC6 cells by CLSM.
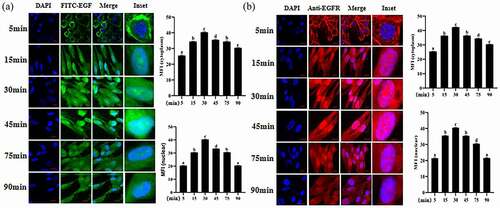
The internalization kinetics of EGFR was also analyzed by CLSM under EGF stimulation. We also found that EGFR also transported into cell nuclei in a time-dependent manner. The nuclear fluorescence signal of EGFR increased with time increasing (), the nuclear fluorescence signal reached a peak after EGF treatment for 30 min. After EGF stimulation for 60 min, the fluorescence signal of EGFR in the cell nuclei decreased slightly.
3.4 EGF-induced intracellular signaling was impaired under the inflammation induced by LPS
In the above-mentioned study, we investigated the cellular behavior of EGF/EGFR under normal physiological conditions. Here, we further explored the cellular behavior of EGF/EGFR in the inflammation induced by LPS, and found that inflammation led to the loss of nuclear signal of EGF/EGFR (). It can be seen that EGF could not induce EGFR to transport into the cell nuclei of intestinal cells in the state of inflammation, suggesting that inflammation led to the change of the cell behavior of EGF/EGFR system, suggesting that inflammation leads to the loss of nuclear signal of EGF. However, when β-carotine was used to pre-treat the cell, the cellular behavior of EGF/EGFR was restored (at least in partially) (). On this basis, we further analyzed the changes of EGF/EGFR signal profile in the inflammatory state (). The results showed that tyrosine phosphorylation of EGFR, STAT3 and AKT were triggered in a time-dependent manner in the EGF (alone) treatment group. The expression levels of p-EGFR, p-STAT3 and p-Akt peaked when the duration of EGF action was at 30 min. However, the intensity of tyrosine phosphorylation of EGFR, STAT3 and AKT was significantly reduced under inflammation. Interestingly, the inflammation-induced signaling imbalance (p-EGFR, p-STAT3 and p-Akt) was found to be partially restored when β-carotene treatment was administered.
Figure 4. Internalization kinetics of EGF/EGFR in IEC6 cells under inflammatory state analyzed by CLSM.
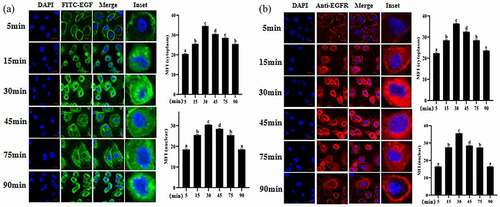
Figure 5. Effect of β-carotene on EGF/EGFR internalization kinetics in IEC6 cells in inflammatory states.
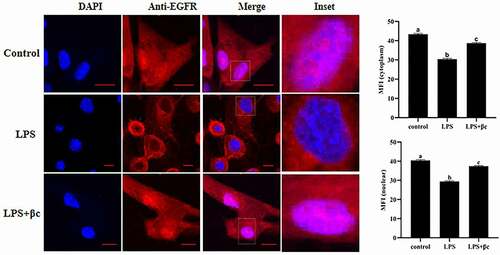
Figure 6. EGF induces phosphorylation of EGFR, AKT and STAT3 (time-dependent assay) in IEC6 cells.
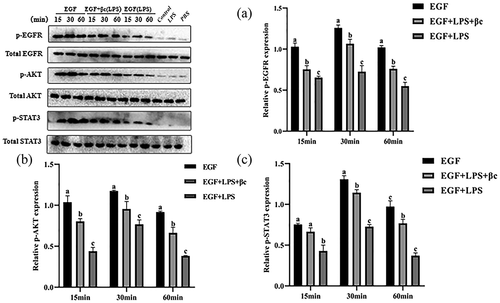
3.5 LPS-induced inflammation was inhibited by the synergistic effect of EGF and β-carotene
The secretion of TNF-α, IL-6, IL-10, and TGF-β1 was measured by ELISA assays, and the results showed that LPS significantly induced IEC6 cells to secrete TNF-α, IL-6, IL-10 and TGF-β1 (). However, the secretion of TNF-α ()) and IL-6 () induced by LPS was attenuated by EGF treatment, and the production of IL-10 () and TGF-β1 () was enhanced at the same time. Previous studies have reported that both EGF and β-carotene alone can relieve inflammation [Citation2,Citation25]. Our results also confirm this conclusion. EGF and β-carotene can reduce LPS-induced inflammation by increasing the expression of IL-10 and TGF-β1, and reducing the expression of TNF-α and IL-6. In addition, we found that the combined effect of EGF and β-carotene was significantly lower than that of single use, the expression of TNF-α and IL-6 was significantly reduced, and the expression of IL-10 and TGF-β1 was significantly increased. We believe that β-carotene and EGF may have a synergistic effect in anti-inflammatory.
Figure 7. Effect of EGF and β-carotene on LPS-induced inflammation.
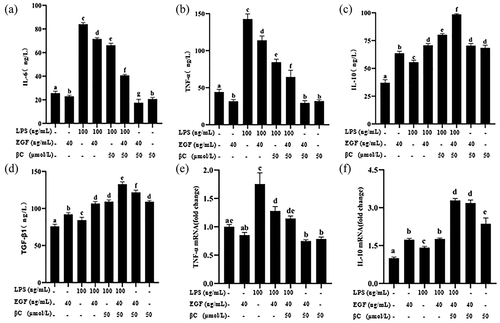
QRT-PCR detected the levels of pro-inflammatory gene TNF-α and anti-inflammatory gene IL-10 mRNA in IEC6 cells, and the results showed that the expression of pro-inflammatory gene TNF-α was significantly increased after LPS (p< 0.05) (), the expression of anti-inflammatory gene IL-10 () decreased significantly (p< 0.05). After treatment with EGF, IL-10 was significantly up-regulated, and TNF-α was significantly reduced. However, compared with EGF alone, the combined application of EGF and β-carotene significantly up-regulated the expression of IL-10, and the change of TNF-α was not significant. These results indicate that EGF can reduce LPS-induced cell inflammation by up-regulating IL-10 and down-regulating TNF-α. In addition, EGF and β-carotene may have a synergistic effect on anti-inflammatory.
3.6 LPS-induced IEC6 cell apoptosis was alleviated by EGF and β-carotene
To evaluate the effects of EGF and β-carotene on the apoptosis of IEC6 cells induced by LPS. Apoptosis rate and mitochondrial damage were detected by flow cytometry. As shown in (A), we found that 20 ng/mL and 40 ng/mL EGF can significantly promote cell proliferation for 24 hours, and 40 ng/mL has a stronger effect on cell proliferation, so we choose 40 ng/mL EGF for subsequent experiments. The apoptotic rate of IEC6 cells was significantly increased by LPS, and EGF treatment (40 ng/ml) reduced the rate of apoptosis induced by LPS (). The cell apoptosis rate after EGF and β-carotene treatment was significantly reduced compared with the ratio of EGF alone (). The results of mitochondrial damage () were the same as the results of apoptosis. Therefore, we conclude that LPS-induced apoptos was alleviated by EGF. In addition, we found that under the induction of LPS, the EGF+β-carotene group had better anti-apoptotic effects, but whether the two have a synergistic effect on anti-apoptosis still needs further study.
Figure 8. Effect of EGF and β-carotene on LPS-induced apoptosis.
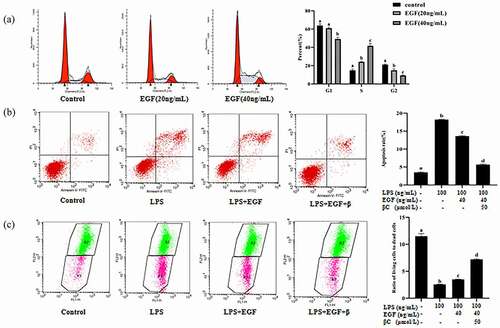
Next, detect the pro-apoptotic genes (P53, Bax, Cascase 3, Cascase 9) and the anti-apoptotic gene bcl-2. The results showed that the pro-apoptotic genes Bax (), P53 (), Cascase 3 () and Cascase 9 () were significantly up-regulated (p< 0.05) after LPS stimulation, and the anti-apoptotic gene Bcl-2 was significantly down-regulated () (p< 0.05). The expression of pro-apoptotic genes was significantly down-regulated after EGF, and the expression of anti-apoptotic gene Bcl-2 was significantly up-regulated. After the combined effect of EGF and β-carotene, Cascase 9 was significantly down-regulated, and the other pro-apoptotic genes showed a downward trend but not significant. The anti-apoptotic gene Bcl-2 showed an upward trend, but it was not significant. The results suggest that EGF reduces LPS-induced apoptosis by up-regulating anti-apoptotic genes and down-regulating pro-apoptotic genes. In IEC6 cells induced by LPS, the combined use of EGF and β-carotene has a stronger anti-apoptotic effect at the gene level, but whether the two have a synergistic anti-apoptotic effect remains to be further studied.
Figure 9. Effects of EGF and β-carotene on pro-apoptotic and anti-apoptotic genes expression induced by LPS.
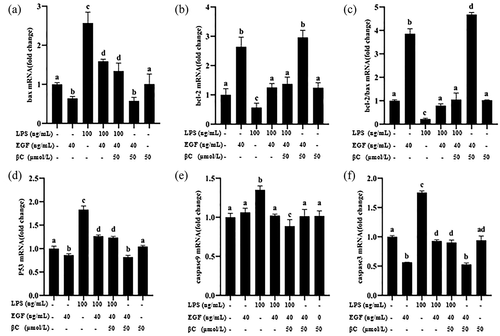
Similarly, Western blot analysis also confirmed that EGF can effectively regulate LPS-induced apoptosis. As shown in , LPS significantly (p< 0.05) caused the expression of Bax, Caspase3, Caspase9, P53 and Fas to increase, and the expression of Bcl-2 to decrease. When the cells were treated by LPS, the expressions of P53 (), Fas (), Bax (), Caspase3 () and Caspase9 () were significantly inhibited by EGF (p< 0.05), but the Bcl-2 protein was enhanced (). At the protein level, the combined effect of β-carotene and EGF is better than EGF alone in anti-apoptotic effects, but it is not significant. Whether β-carotene and EGF have a synergistic effect on anti-apoptosis remains to be explored in the future.
Figure 10. Effects of EGF β-carotene on pro-apoptotic and anti-apoptotic proteins expression induced by LPS.
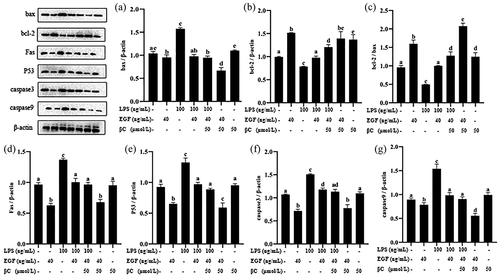
4. Discussion
A series of studies have shown that EGF has important biological functions on the intestine [Citation3–5]. EGF is a cytoprotective peptide that plays an important role in regulating cell growth, survival, migration, apoptosis, proliferation, and differentiation of intestinal tissues and cells [Citation5]. In addition to enhancing cell proliferation and differentiation, EGF also participates in intestinal maturation and maintenance of small intestinal epithelial cell homeostasis, promotes intestinal development, reduces autophagy and inhibits cell apoptosis [Citation26]. The biological effects of EGF are exhibited by EGFR-mediated introcellular signaling pathway, including MAPK/ERK, PI3K/AKT and STAT3 pathways, which are involved in the regulation of cell proliferation, movement, apoptosis protection and differentiation [Citation27]. As we all know, the gastrointestinal tract is not only an important organ for digestion, absorption, and metabolism of dietary nutrients but also serves as a barrier against harmful and toxic substances [Citation24,Citation25]. However, washabau et al reported that acute intestinal inflammation can cause vomiting or diarrhea in animals, loss of appetite, weight loss and performance loss, which may eventually lead to death [Citation28]. It has also been reported that inflammation destroys the integrity of the intestinal mucosal barrier, and the integrity and repair of the epithelial barrier is one of the pathogenesis mechanisms leading to inflammatory bowel disease (IBD) [Citation29]. There are many reports on the impact of inflammation on animal intestinal health. However, until now, the effect of inflammation on the biological activity of EGF in intestinal cells or tissues is still unclear. In the current research, we have carried out a detailed research on this issue. First of all our results indicated the presence of EGFR on IEC6 cells. EGF/EGFR underwent intracellular (IEC6 cells) translocation in a time-dependent manner in the control group. The fluorescence intensity expression level of EGF/EGFR in the nucleus reached a peak when the duration of EGF action was 30 min. However in the inflammatory state, there is little or no nuclear fluorescent signal of EGF/EGFR, thus affecting the biological function of EGF/EGFR in the cells. Interestingly when cells were pretreated with β-carotene, the cellular behavior and biological activity of EGF/EGFR were partially restored.
Our current study showed that inflammation could inhibit EGFR to transport into the nucleus of intestinal cells, resulting in the loss of EGF/EGFR nuclear signal. The traditional view is that growth factors only play a physiological role on the cell membrane; however, some studies have shown that internalized growth factors can transport into cell nuclei to perform more important physiological functions [Citation30]. Accumulating evidence has shown that EGFR has three key nuclear functions: (a) gene regulation (b) kinase function that leads to tyrosine phosphorylation of target proteins (c) protein interaction leads to DNA repair [Citation31]. Shiaw et al. reported that EGFR in the nucleus is related to the high proliferative activity of tissues and the cyclin D1 promoter region in vivo [Citation32,Citation33].Yang et al. reported that EGF/EGFR signal transduction is involved in mediating bone homeostasis, promoting the proliferation and differentiation of osteoblasts and accelerating bone formation [Citation31]. EGF/EGFR mediates the intracellular signaling pathways MAPK/ERK, PI3K/AKT, and STAT3 pathways, and participates in the regulation of cell proliferation, movement regulation, apoptosis protection and differentiation [Citation26]. Studies have reported that the activation of EGFR/AKT signaling is involved in protecting retinal pigment epithelial (RPE) cells from oxidative damage [Citation34]. On the basis of finding that inflammation affects EGF/EGFR cell behavior, we further analyzed the changes of EGF/EGFR signal profile under the inflammation condition. The results showed that the EGFR-mediated intracellular signals AKT and STAT3 phosphorylation levels in the EGF (alone) treatment were stronger, but in the LPS-induced inflammation group, EGF/EGFR-mediated intracellular signals AKT and STAT3 phosphorylation levels were significant significantly down-regulated, indicating that inflammation destroys EGFR-mediated intracellular signaling pathway. But it is interesting is that the addition of β-carotene can alleviate the signal imbalance caused by inflammation and patially restore the biological function of EGF/EGFR.
β-carotene is one of the most abundant natural pigments in foods and has multiple biological functions [Citation21]. Cho et al. reported that β-carotene can inhibit the activation of NF-κB and STAT3 induced by oxidative stress, thereby preventing and inhibiting inflammation [Citation35]. Li et al. also reported that β-carotene can reduce LPS-induced inflammation of RAW264.7 cells and peritoneal macrophages by inhibiting JAK2/STAT3, NF-κB and JNK/p38 MAPK [Citation36]. Chen et al. reported that epidermal growth factor can improve intestinal integrity, colonization of the large intestine, and decrease intestinal permeability by reducing the apoptosis rate of intestinal epithelial cells in rats, thereby improving the inflammatory response of ethanol feeding [Citation2]. In our research, the anti-inflammatory effect of EFG and β-carotene were also confirmed. In addition, we also found that the combined use of β-carotene and EGF shows a better anti-inflammatory effect than that of EGF alone or β-carotene alone. Therefore, it is speculated that β-carotene and EGF display a synergistic effect on anti-inflammation.
Apoptosis is a “programmed” mode of cell death, which usually occurs during development and aging [Citation36]. The mechanism of apoptosis is very complex. So far, studies have shown that mammals have two main apoptosis pathways: the exogenous or death receptor pathway and the endogenous or mitochondrial pathway [Citation36]. In this study, in IEC6 cells stimulated by LPS, the expression of pro-apoptotic genes (such as P53, Bax, caspase-3 and caspase-9) increased, and the expression of the anti-apoptotic gene bcl-2 decreased, indicating that mitochondria-dependent cells apoptosis occurred. The gene expression of P53, Bax, caspase-3 and caspase-9 was down-regulated after EGF treatment. Similarly, Western blot analysis also confirmed that EGF can effectively relieve LPS-induced apoptosis. When cells were stimulated by LPS, EGF significantly inhibited the expression of Fas, Bax, caspase 3, and caspase-9, and promoted the expression of Bcl2 protein. It can be considered that epidermal growth factor can reduce the apoptosis of IEC6 cells induced by LPS by inhibiting the Fas-dependent pathway and promoting the expression of Bcl-2. In addition, studies have found that β-carotene combined with EGF displays a better anti-apoptotic effect.
5. Conclusion
In conclusion, this study found that inflammation destroyed EGF/EGFR-mediated intracellular signal transduction in IEC6 cells, indicating that inflammation has a negative regulatory effect on the biological activity of EGF/EGFR in IEC6 cells. On this basis, further studies have found that β-carotene can not only reduce the inflammatory response of intestinal cells IEC6 induced by LPS but also partially restore the biological activity of EGF in IEC6 cells.
Author contributions
Guoxia Wang: Resources; Writing-original draft. Yu Yang: Writing-original draft. Shuai Zhang: Writing-original draft. Hainan Lan: Writing-original draft. Xin Zheng: Writing-original draft.
Ethical approval
All applicable international, national, and/or institutional guidelines for the care and use of animals were followed.
Consent for publication
All authors read and approved the final manuscript.
Disclosure statement
No potential conflict of interest was reported by the author(s).
Data availability statement
All data and materials are available for publication. Data related to the paper can be obtained from the corresponding author, based on reasonable requirements.
Additional information
Funding
References
- Singh B, Carpenter G, Coffey RJ. EGF receptor ligands: recent advances. F1000Research. 2016;5:2270. F1000Res. 5.
- Chen YL, Peng HC, Hsieh YC, et al. Epidermal growth factor improved alcohol-induced inflammation in rats. Alcohol. 2014;487(7):701–706.
- Zeng F, Harris RC. Epidermal growth factor, from gene organization to bedside. Semin Cell Dev Biol. 2014;28:2–11.
- Ellina MI, Bouris P, Kletsas D, et al. EGF/EGFR signaling axis is a significant regulator of the proteasome expression and activity in colon cancer cells. Semin Cell Dev Biol; 2014.
- Mao Y, Ma J, Xia Y, et al. The Overexpression of Epidermal Growth Factor (EGF) in HaCaT Cells Promotes the Proliferation, Migration, Invasion and Transdifferentiation to Epidermal Stem Cell Immunophenotyping of Adipose-Derived Stem Cells (ADSCs). Int J Stem Cells. 2020;131(1):93–103.
- Torres-Castro P, Abril-Gil M, Rodríguez-Lagunas MJ, et al. TGF-β2, EGF, and FGF21 Growth Factors Present in Breast Milk Promote Mesenteric Lymph Node Lymphocytes Maturation in Suckling Rats. Nutrients. 2018;10(9):1171.
- Grases-Pintó B, Torres-Castro P, Marín-Morote L, et al. Leptin and EGF Supplementation Enhance the Immune System Maturation in Preterm Suckling Rats. Nutrients. 2019;1110(10):2380.
- Klingensmith NJ, Yoseph BP, Liang Z, et al. Epidermal Growth Factor Improves Intestinal Integrity and Survival in Murine Sepsis Following Chronic Alcohol Ingestion. Shock. 2017;472(2):184–192.
- Suzuki A, Sekiya S, Gunshima E, et al. EGF signaling activates proliferation and blocks apoptosis of mouse and human intestinal stem/progenitor cells in long-term monolayer cell culture. Lab Invest. 2010;9010(10):1425–1436.
- Dominguez JA, Vithayathil PJ, Khailova L, et al. Epidermal growth factor improves survival and prevents intestinal injury in a murine model of pseudomonas aeruginosa pneumonia. Shock. 2011;364(4):381–389.
- Clark JA, Clark AT, Hotchkiss RS, et al. Epidermal growth factor treatment decreases mortality and is associated with improved gut integrity in sepsis. Shock. 2008;301:36–42.
- Sasaki M, FitzGerald AJ, Mandir N, et al. Keratinocyte growth factor and epidermal growth factor can reverse the intestinal atrophy associated with elemental diets in mice. Exp Physiol. 2003;88(882):261–267.
- Dong N, Xu X, Xue C, et al. Ethyl pyruvate inhibits LPS induced IPEC-J2 inflammation and apoptosis through p38 and ERK1/2 pathways. Cell Cycle. 2019;1820(20):2614–2628.
- Singh N, Baby D, Rajguru JP, et al. Inflammation and cancer. Ann Afr Med. 2019;183(3):121–126.
- Kasza A. IL-1 and EGF regulate expression of genes important in inflammation and cancer. Cytokine. 2013;621(1):22–33.
- Lawrence T. The nuclear factor NF-kappaB pathway in inflammation. Cold Spring Harb Perspect Biol. 2009;16:a001651.
- Habbel J, Arnold L, Chen Y, et al. Inflammation-driven activation of JAK/STAT signaling reversibly accelerates acute myeloid leukemia in vitro. Blood Adv. 2020;413(13):3000–3010.
- Dong Q, Jie Y, Ma J, et al. Renal tubular cell death and inflammation response are regulated by the MAPK-ERK-CREB signaling pathway under hypoxia-reoxygenation injury. J Recept Signal Transduct Res. 2019;395-6(5–6):383–391.
- Cheng Y, Hall TR, Xu X, et al. Targeting uPA-uPAR interaction to improve intestinal epithelial barrier integrity in inflammatory bowel disease. EbioMedicine. 2022;75:103758.
- Tang T, Song J, Wang H, et al. Qingke β-glucan synergizes with a β-glucan-utilizing Lactobacillus strain to relieve capsaicin-induced gastrointestinal injury in mice. Int J Biol Macromol. 2021;174:289–299.
- Zhao G, Zhang X, Wang H, et al. Beta carotene protects H9c2 cardiomyocytes from advanced glycation end product-induced endoplasmic reticulum stress, apoptosis, and autophagy via the PI3K/Akt/mTOR signaling pathway. Ann Transl Med. 2020;810(10):647.
- Bai SK, Lee SJ, Na HJ, et al. beta-Carotene inhibits inflammatory gene expression in lipopolysaccharide-stimulated macrophages by suppressing redox-based NF-kappaB activation. Exp Mol Med. 2005;374(4):323–334.
- Yang Y, Li R, Hui J, et al. β-Carotene attenuates LPS-induced rat intestinal inflammation via modulating autophagy and regulating the JAK2/STAT3 and JNK/p38 MAPK signaling pathways. J Food Biochem. 2021;451:e13544.
- Stewart AS, Pratt-Phillips S, Gonzalez LM. Alterations in Intestinal Permeability: the Role of the “Leaky Gut” in Health and Disease. J Equine Vet Sci. 2017;52:10–22.
- Camilleri M, Madsen K, Spiller R, et al. Intestinal barrier function in health and gastrointestinal disease. Neurogastroenterol Motil. 2012;246(6):503–512.
- Arda-Pirincci P, Bolkent S. The role of epidermal growth factor in prevention of oxidative injury and apoptosis induced by intestinal ischemia/reperfusion in rats. Acta Histochem. 2014;1161(1):167–175.
- Kitade M, Factor VM, Andersen JB, et al. Specific fate decisions in adult hepatic progenitor cells driven by MET and EGFR signaling. Genes Dev. 2013;2715(15):1706–1717.
- Washabau RJ, Day MJ, Willard MD, et al. Endoscopic, biopsy, and histopathologic guidelines for the evaluation of gastrointestinal inflammation in companion animals. J Vet Intern Med. 2010;241:10–26.
- Leppkes M, Roulis M, Neurath MF, et al. Pleiotropic functions of TNF-α in the regulation of the intestinal epithelial response to inflammation. Int Immunol. 2014;269(9):509–515.
- Chunhui Y, Wenjun C, Hui W, et al. Pilose antler peptide protects osteoblasts from inflammatory and oxidative injury through EGF/EGFR signaling. Int J Biol Macromol. 2017;99:15–20.
- Lo HW. Nuclear mode of the EGFR signaling network: biology, prognostic value, and therapeutic implications. Discov Med. 2010;1050:44–51.
- Lin SY, Makino K, Xia W, et al. Nuclear localization of EGF receptor and its potential new role as a transcription factor. Nat Cell Biol. 2001;39(9):802–808.
- Cheng LB, Chen CM, Zhong H, et al. Squamosamide derivative FLZ protects retinal pigment epithelium cells from oxidative stress through activation of epidermal growth factor receptor (EGFR)-AKT signaling. Int J Mol Sci. 2014;15(10):18762–18775.
- Cho SO, Kim MH, Kim H. β-Carotene Inhibits Activation of NF-κB, Activator Protein-1, and STAT3 and Regulates Abnormal Expression of Some Adipokines in 3T3-L1 Adipocytes. J Cancer Prev. 2018;231(1):37–43.
- Li R, Li L, Hong P, et al. β-Carotene prevents weaning-induced intestinal inflammation by modulating gut microbiota in piglets. Asian-Australas J Anim Sci. 2019;34(7):1221–1234.
- Elmore S. Apoptosis: a review of programmed cell death. Toxicol Pathol. 2007;354(4):495–516.