ABSTRACT
Preeclampsia is a serious pregnancy disorder affecting both maternal and fetal health. However, the pathogenesis of preeclampsia has not been fully understood. This study aimed to investigate the key microRNAs (miRNAs) in the development of preeclampsia. A high-throughput miRNA sequencing analysis for the placental tissues from patients with preeclampsia and healthy controls was conducted, followed by investigation of differentially expressed miRNAs (DEMs) and functional enrichment analysis. Moreover, the expression of a key DEM, named miR-200b-3p, in the preeclampsia patients was validated, and the effects of miR-200b-3p overexpression on the proliferation, migration, and apoptosis of HTR8 trophoblast cells were investigated in vitro. Furthermore, the target gene of miR-200b-3p was investigated based on gene expression profile GSE177049 and miRWalk 2.0 database. The target relationship between miR-200b-3p and profilin 2 (PFN2) was investigated in vitro. A total of 12 DEMs including miR-200b-3p were identified between preeclampsia placental tissues and control placental tissues, which were significantly enriched in several pathways, such as cell adhesion molecules (CAMs) and tight junction. Moreover, increased expression of miR-200b-3p was revealed in the placental tissues of preeclampsia patients, and overexpression of miR-200b-3p suppressed cell proliferation and migration but promoted apoptosis of trophoblast cells. Furthermore, PFN2 was confirmed as a target of miR-200b-3p, and overexpression of PFN2 reversed the inhibitory effects of miR-200b-3p overexpression on trophoblast cell migration. Our findings reveal that miR-200b-3p is upregulated in the placental tissues of patients with preeclampsia and promotes preeclampsia development via PFN2. miR-200b-3p may serve as a promising therapeutic target against preeclampsia.
Introduction
Preeclampsia is an obstetric disorder characterized by new-onset hypertension and proteinuria after 20 weeks of gestation [Citation1]. It affects 2% to 8% of pregnancies worldwide [Citation2] and is a major cause of both maternal and fetal morbidity and mortality [Citation3]. Moreover, preeclampsia is associated with an increased risk of developing hypertension, diabetes mellitus, and cardiovascular complications later in life for both the mother and her child [Citation4]. Currently, few effective treatments are available for patients with preeclampsia in clinics. The delivery of the fetus and placenta is considered as the only available cure for preeclampsia, which reduces proinflammatory substances in the maternal cardiovasculature [Citation5,Citation6]. However, the pathogenesis of preeclampsia remains elusive.
MicroRNAs (miRNAs) are small (about 18–24 nucleotides in length) noncoding single-stranded RNAs responsible for regulating 40% to 60% of gene expression at the posttranscriptional level [Citation7]. Growing studies have indicated that miRNAs perform critical roles in the pathobiology of preeclampsia [Citation8–10]. For instance, overexpression of miR-30a-3p in the placentas is associated with the pathogenesis of preeclampsia [Citation11]; miR-655-3p contributes to the development of preeclampsia by regulating biological features of trophoblasts [Citation12]. Moreover, differentially expressed miRNAs (DEMs) may be implicated in preeclampsia development, since the biological processes involving these miRNAs have been shown to be similar to those involved in preeclampsia [Citation13–15]. A very recent study also reveals that several miRNAs have exhibited diagnostic potential as biomarkers in the detection of preeclampsia [Citation16]. Taken together, the identification of key miRNAs underlying preeclampsia will foster the development of diagnostic biomarkers or effective therapeutic strategies. Nevertheless, the crucial miRNAs involved in preeclampsia development have not yet been fully investigated.
In the present study, we performed a high-throughput miRNA sequencing analysis to investigate DEMs and their enriched pathways in patients with preeclampsia. We then validated the expression of a key DEM, named miR-200b-3p, in patients with preeclampsia and investigated the role of overexpression of miR-200b-3p in proliferation, migration, and apoptosis of trophoblast cells in vitro. Furthermore, the target gene of miR-200b-3p was explored to elucidate its regulatory mechanism. Our findings may expand our understanding regarding the pathogenesis of preeclampsia and provide a potential biomarker for the prevention and treatment of this disease.
Materials and methods
Tissue specimens
The study was approved by the Ethics Committee of Shandong Provincial Hospital (SWYX: NO. 2021–352), and the need to obtain informed consent was waived.
Fifteen pregnant women who were diagnosed with preeclampsia and gave birth by cesarean section in our hospital were included. According to the diagnostic criteria for preeclampsia (referred to the 9th edition of Obstetrics and Gynecology [Citation17]), pregnant women with normal blood pressure developed hypertension after ≥20 weeks of gestation, accompanied by at least one of the following new conditions: (1) proteinuria; (2) function insufficiency of other maternal organs, including acute kidney injury, liver involvement with or without epigastric pain, neurological symptoms, and hematological involvement; and (3) uterine-placental dysfunctions, such as fetal growth restriction, abnormal umbilical artery flow or intrauterine death. All subtypes of preeclampsia were enrolled, including early-onset preeclampsia (gestational age <34 weeks + 0); late-onset preeclampsia (gestational age ≥34 weeks + 0); and chronic hypertension with superimposed preeclampsia. Fifteen pregnant women who gave birth by cesarean section due to breech position and scar uterus were included as controls. Exclusion criteria: premature rupture of membranes; conception with assisted reproductive technology; teratogenic or stillbirth; multiple pregnancies (≥2 births); and people who were addicted to alcohol, drugs, and drug abuse.
During the cesarean section, within 5 min of delivery of the placenta, placental tissues with a size of 1 × 1 cm were cut from the center of the vertical section of the placenta where the umbilical cord was attached, to avoid infarction, hemorrhage, and calcification. The placental tissues were rinsing with the ice-code normal saline to remove blood, cut into pieces, frozen in liquid nitrogen, and then stored at a − 80°C refrigerator.
High-throughput sequencing
Six placental tissues, including three placental tissue samples (SPE1, SPE2, and SPE3) from patients with preeclampsia and three control samples (Con1, Con2, and Con3) from healthy controls, were subjected to high-throughput sequencing. Total RNA was extracted from the placental tissues using RNAzol RT RNA Isolation Reagent (GeneCopoela, Rockville, MD, USA). The quantity and integrality of the total RNA was detected by Agilent2100 (Agilent, USA). After polyacrylamide gel electrophoresis purification of small RNA molecules (18–30 nt) and 3’- and 5’-end ligation, the small RNA molecules were amplified and used for library construction. The quality and quantity of the established library was tested using an Agilent 2100 Bioanalyzer and ABI StepOnePlus Real-Time PCR System. A small RNA sequencing analysis was then conducted using an Illumina HiSeqTM2500 system (Illumina, CA, USA).
Data filtering and sequence alignment
To ensure the reliability of the results, data filtering was performed. In detail, the reads with low sequencing quality, with unknown base N content greater than 5%, with contaminated 5’ adapter sequence or no insertion fragments, and without 3’ adapter sequence were removed. Moreover, the reads containing polyA and shorter than 18 nt were excluded. After data filtering, the clean reads were saved in FASTQ format. Blast software was used to compare the sequencing tags with miRBase database to identify known miRNAs. To obtain more miRNAs, the novel miRNAs were predicted by mapping the clean reads to the human reference genome using SOAPaligner software (soap2.21; http://soap.genomics.org.cn/soapaligner.html).
Differential expression analysis of miRNAs
The DEMs between preeclampsia samples and control samples were analyzed using the DEseq2 package [Citation18]. The cutoff value was set as |log2 fold change (FC)| > 1 and p < 0.05. To visualize the DEMs, volcano plot and hierarchical clustering of miRNAs were conducted.
Functional enrichment analysis of target genes of DEMs
To understand the function of DEMs, the target genes of DEMs were predicted using the miRanda [Citation19], and targetscan [Citation20] databases, respectively. The overlapping target genes from the two datasets were subjected to Gene Ontology (GO) and Kyoto Encyclopedia of Genes and Genomes (KEGG) pathway enrichment analyses using phyper function in R. The p value was subjected to false discovery rate (FDR) adjustment, and FDR ≤ 0.01 indicated a significant enrichment result.
Prediction of target genes of miR-200b-3p
To further investigate the target genes of miR-200b-3p, the gene expression profile GSE177049 was downloaded from Gene Expression Omnibus database. In this dataset, the RNA sequencing data for placenta samples from five early-onset preeclampsia (EO-PE) cases and five preterm birth (PTB) controls were used. The differentially expressed genes (DEGs) between EO-PE cases and PTB controls were analyzed using limma package [Citation21] in R. The cutoff value was set as |logFC| > 0.585 and p < 0.05. Volcano plot and hierarchical clustering were also conducted to visualize the DEGs. Based on miRWalk 2.0 database, the target genes of miR-200b-3p was predicted. The predicted target genes should be presented in at least seven of the following databases, including miRWalk, Microt4, miRanda, mirbridge, miRDB, miRMap, miRNAMap, Pictar2, PITA, RNA22, RNAhybrid, and Targetscan. Other prediction parameters are database defaults. By further intersection analysis of the above-obtained DEGs and predicted target genes, the overlapping genes were regarded as the target genes of miR-200b-3p in the development of preeclampsia.
Quantitative real-time polymerase chain reaction (qPCR)
Total RNA was prepared from clinical placental tissues from patients with preeclampsia and healthy controls using Trizol reagent (CW Bio Co., Ltd., Beijing, China) according to the manufacturer’s instructions. Subsequently, total RNA was reverse transcribed into cDNA using the HiFiScript cDNA Synthesis kit (CW Bio Co., Ltd.). qPCR was performed using specific primers and SYBR Master Mixture Taq kit (CW Bio Co., Ltd.) with an ABI 7500 Real-time PCR system (Applied Biosystems; Thermo Fisher Scientific, Inc., Waltham, MA, USA). The sequences of the primers were as follows: miR-200b-3p, 5’-GCGCGTAATACTGCCTGGTAA-3’ (forward) and 5’-AGTGCAGGGTCCGAGGTATT-3’ (reverse); U6, 5’-GTGCTCGCTTCGGCAGCACATAT-3’ (forward) and 5’-AGTGCAGGGTCCGAGGTATT-3’ (reverse); profilin 2 (PFN2), 5’-CAACGGTTTGACTCTTGGCG-3’ (forward) and 5’-TGCCGACAGCCACATTGTAT-3’ (reverse); and actin, 5’-AGACCTGTACGCCAACACAG-3’ (forward) and 5’-CGGACTCGTCATACTCCTGC-3’ (reverse). U6 and actin were used as the internal controls for miR-200b-3 and PFN2, respectively. The relative expression level was calculated using the 2−ΔΔCt method.
Cell culture
Human trophoblast HTR8 cell line was purchased from Cell Bank of Shanghai Institutes for Biological Sciences, Chinese Academy of Sciences. HTR8 cell were cultured in Dulbecco’s modified Eagle’s medium (DMEM, Gibco, USA) containing 10% heat-inactivated fetal bovine serum (FBS, Gibco, USA), and then maintained in a 37°C humidified incubator with 5% CO2.
Cell transfection
HTR8 cells were plated into 6-well plates and grown until 80% confluency. HTR8 cells were then transfected with miR-200b-3p mimics and their negative control (NC) using Lipofectamine 2000 (Invitrogen, Carlsbad, CA). After 48 h of transfection, cells were harvested for the subsequent experiments.
A PFN2 overexpression vector (OE-PFN2) was constructed by cloning the full-length wild-type PFN2 coding sequence into pEGFP-N1 vector. The empty construct pEGFP-N1 was used as the negative control. Cells were transfected with lentivirus for OE-PFN2 and OE-NC at an appropriate multiplicity of infection (MOI). Transfection was also performed using Lipofectamine 2000 (Invitrogen, Carlsbad, CA). After 48 h of transfection, cells were harvested for the subsequent experiments.
CCK8 assay
After transfection, HTR8 cells (2000 cells) were seeded onto 96-well plates. At the indicated time points (0, 24, 48, 72, and 96 h), 10 μl of CCK-8 reagent (Cat# HY-K0301, Med Chem Express, NJ, USA) was added to each well and continued to incubate the cells for 1–4 h at 37°C. The optical density (OD) value of each well was detected with a microplate reader (Tecan Infinite F50, Tecan, Männedorf, Switzerland).
Transwell migration assay
The Transwell inserts (8-μm pore size, #3422, Corning, USA) were added into 24-well plates, and 100 μl serum-free media was added to incubate the inserts for 1–2 h. The cells at the logarithmic growth stage in different groups were trypsin digested, suspended in serum-free media, and then seeded into the upper Transwell inserts. The 600 μL medium containing 30%FBS was then added into the lower Transwell inserts. After incubation for 24 h, cells in the upper Transwell inserts were wiped with cotton swab. The Transwell inserts were stained with 0.1% crystal violet for 20 min. After rinsing several times, the migrated cells in the lower Transwell inserts were imaged and counted using a fluorescent microscope (Olympus, Tokyo, Japan).
Scratch wound-healing assay
A marker pen was used to draw lines on the back of dishes. The HTR8 cells (5 × 105 cells/well) were plated in culture dishes. Cells were cultured overnight and formed a confluent monolayer. Then, a linear scratch wound was created in a straight line with a pipet tip. Scratched wells were rinsed three times with PBS to remove the debris. Afterward, the serum-free media were added to culture cells for 24 h at 37°C in a humidified incubator with 5% CO2. At 0 and 24 h after scratch, wound closure was photographed and analyzed.
Flow cytometry for detection of cell apoptosis
Cell apoptosis of different groups was detected by the FITC-Annexin V Apoptosis Detection Kit (BD Biosciences) using flow cytometry. Briefly, HTR8 cells in different groups were starved in a serum-free medium for 24 h. Cell (1 × 106 cells/ml) were then harvested, followed by a double staining with 5 μl FITC-Annexin V and 10 μl Propidium iodide (PI). After incubation at room temperature and in the dark for 5 min, the percentage of apoptotic cells was detected with a flow cytometry (FACScan; BD Biosciences) and analyzed using Flowjo software (TreeStar).
Dual-luciferase reporter gene assay
The wild-type (WT) or mutated-type (MUT) PFN2-3ʹUTR was, respectively, cloned into the psiCHECKTM-2 plasmids (Promega, Madison, Wisconsin, USA) to construct dual-luciferase reporter vectors, named PFN2-WT or PFN2-MUT luciferase reporter vectors. HEK293 T cells (2 × 104 cells/well) were seeded into 96-well plates and cotransfected with the PFN2-WT or PFN2-MUT luciferase reporter vectors and miR-200b-3p mimics or mimic NC using Lipofectamine 2000 (Invitrogen). At 48 h after transfection, the luciferase activity of the cells was detected in the dual-Luciferase Reporter Assay System (E1910, Promega). Renilla luciferase activity was standardized for firefly activity, and the relative luciferase activity was calculated.
Western blot assay
Cells in different treatment groups were lysed with 1 × RIPA lysis buffer (Beyotime, Haimen, China) on ice for 10–15 min and then centrifuged at 12,000 g for 5 min. Total proteins were collected, followed by detection of their concentration using a BCA method. The protein (20 μg) was separated on 10% SDS-polyacrylamide gels and transferred onto polyvinylidene fluoride (PVDF) membranes (Millipore, Billerica, MA, USA). The membranes were blocked with 5% skimmed milk, probed with rabbit anti-PFN2 antibody (1:1000, ABclonal, Cambridge, MA, USA) and mouse anti-GAPDH antibody (1:5000, Proteintech, Inc., CA, USA) at 4°C overnight, and incubated with secondary antibodies (HRP Goat Anti-Rabbit IgG (H + L) or HRP Goat Anti-Mouse IgG (H + L), 1:5000, ABclonal) at room temperature for 1 h. After rinsing, the protein blots were visualized using ECL method (Pierce, Thermo Scientific, Waltham, USA) and analyzed using Image J software.
Statistical analysis
Measurement data were analyzed using the Shapiro–Wilk test to determine the normal distribution of all variables. Data conforming to the normal distribution are expressed as the mean ± standard deviation (SD). T test was used to analyze the differences between the two groups and a one-way ANOVA following by Tukey’s post hoc test was applied to compare differences among multiple groups. Otherwise, data were presented as median (interquartile range) and compared with the Wilcoxon rank sum test. Statistical analyses were accomplished using the SPSS 25.0 software (SPSS, Chicago, IL). P < 0.05 indicated a statistically significant result.
Results
High-throughput sequencing data
To explore key miRNAs in the development of preeclampsia, the miRNA sequencing for placental tissues from three patients with preeclampsia and three healthy controls was conducted using the Illumina sequencing technology. shows the clinical characteristics of the study population for miRNA sequencing.
Table 1. Clinical characteristics of the study population for miRNA sequencing.
After data preprocessing, 31,314,934, 31,072,695, 32,138,922, 30,087,385, 30,899,838, and 32,902,495 clean reads were obtained from Con1, Con2, Con3, SPE1, SPE2, and SPE3 samples. After sequence alignment to the human reference genome, the mapped rates of all samples were up to 79%, and the unique mapped rates were about 30% (). As results, 1814 known miRNAs and 1617 novel miRNAs were obtained from all samples.
Table 2. The sequence alignment results.
DEMs analysis
With a cutoff value of |log2FC| >1 and p < 0.05, 12 DEMs were identified between preeclampsia samples and control samples. Among these, seven miRNAs, namely, hsa-miR-200b-3p, hsa-miR-708-5p, hsa-miR-10b-5p, hsa-miR-549a-5p, hsa-miR-124-3p, hsa-miR-2113, and hsa-miR-200a-3p, were significantly upregulated in the preeclampsia samples, while the other five miRNAs, including novel-m0267-5p, novel-m0224-3p, hsa-miR-2682-3p, hsa-miR-137-3p, and hsa-miR-4664-5p, were remarkably downregulated. A volcano plot of DEMs is shown in . Moreover, the results of hierarchical clustering of miRNAs showed that preeclampsia samples and control samples could be distinguished by these DEMs ().
Functional enrichment analysis of the target genes of DEMs
Based on the information of miRanda and targetscan databases, a total of 119, 709, 690, 311, 1511, 124, 460, 1745, 204, 756, 21, and 1246 overlapping target genes of hsa-miR-200b-3p, hsa-miR-708-5p, hsa-miR-10b-5p, hsa-miR-549a-5p, hsa-miR-124-3p, hsa-miR-2113, hsa-miR-200a-3p, novel-m0267-5p, novel-m0224-3p, hsa-miR-2682-3p, hsa-miR-137-3p, and hsa-miR-4664-5p were obtained from the two databases, respectively. To understand the function of DEMs, GO functions in different GO categories (biological process, cellular component, and molecular function) and KEGG pathways were enriched by the target genes of DEMs. The results showed that DEMs were significantly enriched in GO-biological process functions, such as cellular process, biological regulation, and metabolic process; GO-cellular component functions, like cell, cell part, and organelle; and GO-molecular functions, such as binding, catalytic activity, and molecular function regulator (). Moreover, the significantly enriched pathways of target genes of DEMs mainly included cell adhesion molecules (CAMs), tight junction, and focal adhesion ().
miR-200b-3p was upregulated in the placental tissues of patients with preeclampsia
Based on the results of miRNA sequencing data, we proposed that miR-200b-3p participated in the development of preeclampsia. Hence, qPCR was conducted to verify the expression of miR-200b-3p in 15 patients with preeclampsia and 15 healthy controls. The clinical characteristics of patients with preeclampsia and healthy controls are summarized in . There was no significant difference in maternal age and number of nulliparous between the two groups. Compared to healthy controls, patients with preeclampsia had higher BMI, systolic/diastolic blood pressure, and proteinuria, and lower Gestational age at delivery and birth weight of newborns (P < 0.05). The results of qPCR showed that miR-200b-3p expression was significantly upregulated in the placental tissues of patients with preeclampsia compared to that in the placental tissues of controls (P < 0.01, ), in line with the result of miRNA sequencing analysis.
Table 3. Clinical characteristics of the study population for quantitative real-time polymerase chain reaction (qPCR) validation.
miR-200b-3p inhibited trophoblast cell proliferation and migration while promoted cell apoptosis
To further investigate the function of miR-200b-3p in preeclampsia development, miR-200b-3p was overexpressed in HTR8 cells by transfection. The cell proliferation was monitored by CCK-8 assay, and the results indicated that compared with NC group, overexpression of miR-200b-3p remarkably decreased the cell viability of HTR-8 cells after 48 h of transfection (P < 0.05, ). Moreover, both Transwell migration assay and scratch wound healing assay revealed that overexpression of miR-200b-3p significantly inhibited the migration of HTR-8 cells (P < 0.01, ). Furthermore, the results of flow cytometry revealed that the overexpression of miR-200b-3p dramatically promoted the apoptosis of HTR-8 cells (P < 0.001, ). Our data demonstrated that overexpression of miR-200b-3p might promote the development of preeclampsia.
Figure 4. The effects of miR-200b-3p on the viability, migration and apoptosis of trophoblast cells in vitro. A: CCK-8 assay showed cell proliferation in different groups. B: Transwell migration assay showed cell migration in different groups. C: Scratch wound healing assay showed cell migration in different groups. D: Flow cytometry showed cell apoptosis in different groups. Significant differences compared with negative control (NC) group: * indicated P < 0.05, ** indicated P < 0.01, and *** indicated P < 0.001.
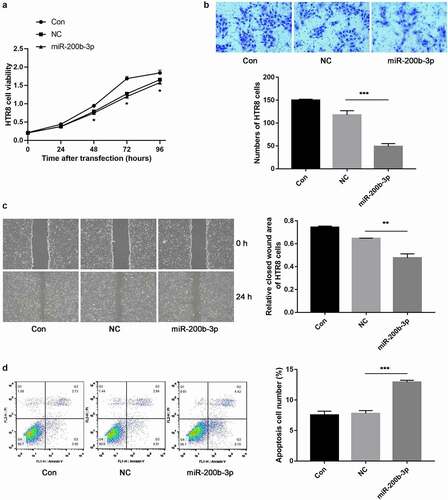
PFN2 was a functional target of miR-200b-3p and overexpression of PFN2 reversed the inhibitory effects of miR-200b-3p overexpression on trophoblast cell migration
Based on GSE177049, a total of 194 DEGs between EO-PE cases and PTB controls were obtained, including 71 up-regulated genes and 123 down-regulated ones. The volcano plot and hierarchical clustering heatmap of DEGs are shown in . Moreover, 1198 target genes of miR-200b-3p was predicted using miRWalk 2.0 tool. By intersection analysis of the above-obtained DEGs and predicted target genes, 16 overlapping genes, including PFN2 were obtained (), which were regarded as the target genes of miR-200b-3p in the development of preeclampsia. The expression level of PFN2 was found to be significantly down-regulated in EO-PE cases compared to that in PTB controls based on GSE177049 ().
Figure 5. PFN2 was predicted as a potential target of miR-200b-3p. A: A volcano plot of differentially expressed genes (DEGs) between early-onset preeclampsia (EO-PE) cases and 5 preterm birth (PTB) controls based on gene expression profile GSE177049. B: Hierarchical clustering heatmap of DEGs. C: Venn plot of DEGs and predicted target genes by miRWalk 2.0 database. D: The expression level of PFN2 in EO-PE cases and PTB controls based on GSE177049. * indicated P < 0.05.
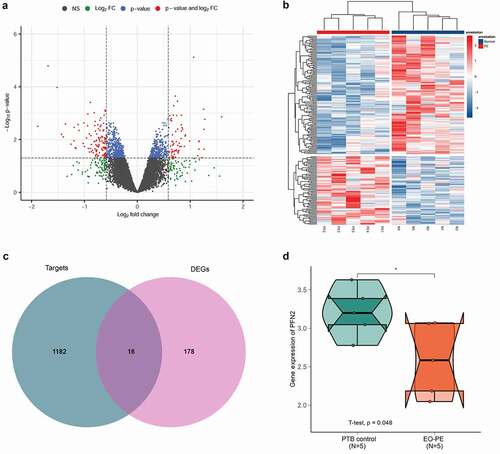
The relationship between miR-200b-3p and PFN2 was validated by in vitro experiments. The results showed that the expression level of PFN2 protein was significantly down-regulated in HTR-8 cells after overexpression of miR-200b-3p (P < 0.05, ). The results of luciferase activity assay showed that only the luciferase activity of PFN2-WT was significantly inhibited after co-transfection with miR-200b-3p (P < 0.05, ). To further investigate whether miR-200b-3p contribute to preeclampsia via targeting PFN2, we overexpressed the expression of PFN2 by transfection. The results of qPCR () and western blot assay () showed that the expression levels of PFN2 mRNA and protein were all significantly increased after transfection (P < 0.001), suggesting the high transfection efficiency. Furthermore, both Transwell migration assay () and scratch wound healing assay () revealed that the inhibitory effect of miR-200b-3p overexpression on the migration of HTR-8 cells was significantly reversed after overexpression of miR-200b-3p and PFN2 at the same time (all P < 0.01).
Figure 6. miR-200b-3p could target PFN2 and overexpression of PFN2 reversed the inhibitory effects of miR-200b-3p overexpression on trophoblast cell migration in vitro. A: Western blot assay showed the expression level of PFN2 protein in HTR-8 cells after overexpression of miR-200b-3p. B: Dual luciferase activity assay indicated that miR-200b-3p could target PFN2. C: qPCR showed the expression levels of PFN2 mRNA after transfection. D: Western blot assay showed the expression levels of PFN2 protein after transfection. E: Transwell migration assay showed cell migration in different groups. F: Scratch wound healing assay showed cell migration in different groups. Significant differences compared with corresponding control group: * indicated P < 0.05, and *** indicated P < 0.001.
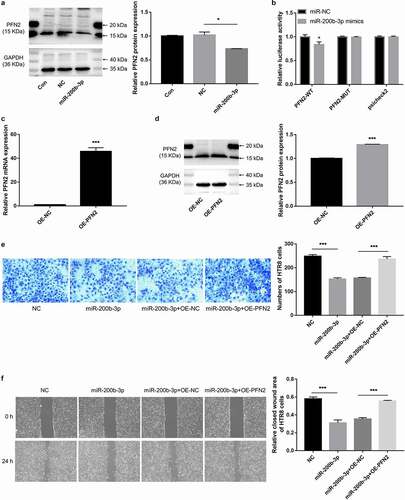
Discussion
The present study demonstrated that 12 DEMs including miR-200b-3p were identified between preeclampsia placental tissues and control placental tissues, which were significantly enriched in several pathways, such as CAM and tight junction. Moreover, qPCR confirmed the increased expression of miR-200b-3p in the placental tissues from patients with preeclampsia, and in vitro experiments revealed that overexpression of miR-200b-3p could suppress the viability and migration but promoted the apoptosis of trophoblast cells. Furthermore, PFN2 was confirmed as a functional target of miR-200b-3p and overexpression of PFN2 reversed the inhibitory effects of miR-200b-3p overexpression on trophoblast cell migration. Collectively, these findings suggested that miR-200b-3p may represent a potential therapeutic target against preeclampsia.
Preeclampsia is considered to be a pregnancy complication of placental origin since the clinical symptoms disappear rapidly after the placenta is delivered [Citation22]. The trophoblast cells, the major cell type in the placenta, have been shown to play pivotal roles in placental development [Citation23], and dysfunction of the trophoblast cells is associated with preeclampsia [Citation24]. Accumulating studies have reported that miRNAs are aberrantly expressed in the placenta tissues of patients with preeclampsia and take part in several pathophysiological processes, including the migration and apoptosis of trophoblast cells. For instance, miR-142-3p is upregulated expressed in preeclampsia tissues, whose overexpression inhibits trophoblast cell growth and migration [Citation25]; miR-491-5p expression is increased in the placental tissues from patients with preeclampsia, and its upregulation suppresses the migration and invasion of trophoblast cells [Citation26]; miR-124-3p expression is increased in the placental tissues of preeclampsia patients, and miR-124-3p overexpression in trophoblastic cells significantly decreased cell migration and invasion but increased cell apoptosis [Citation27]; and miR-200a-3p is found to be upregulated in human preeclamptic plasma and placenta and contribute to preeclampsia development via targeting transthyretin [Citation28]. Consistent with previous findings, miR-124-3p and miR-200a-3p were also identified as DEMs in our study, suggesting an association between DEMs and preeclampsia.
Notably, our study revealed that miR-200b-3p expression was increased in the placental tissues from patients with preeclampsia, and overexpression of miR-200b-3p could suppress the viability and migration and promoted the apoptosis of trophoblast cells. Previous studies have demonstrated that miR-200b-3p is a key regulator of tumor cell functions, such as cell proliferation, migration, and apoptosis [Citation29–31]. Notably, a recent microarray analysis of preterm preeclampsia has suggested that miR-200b-3p might be involved in the development of preterm preeclampsia; however, no experimental validation was conducted on the expression and function of miR-200b-3p in the disease [Citation32]. Moreover, PFN2 was identified as a functional target of miR-200b-3p. PFN2 acts as an actin cytoskeleton regulator and plays a significant role in cell motility [Citation33]. PFN2 has been reported to participate in a variety of cancers, such as head and neck cancer [Citation34] and triple negative breast cancer [Citation35]. Miao et al. demonstrated that PFN2 was down-regulated in patients with chronic thromboembolic pulmonary hypertension [Citation36], suggesting the potential role of PFN2 in this disease. However, there was no study reporting the role of PFN2 in preeclampsia. Our study revealed that overexpression of PFN2 reversed the inhibitory effects of miR-200b-3p overexpression on trophoblast cell migration, hinting that PFN2 was also a key player in preeclampsia development. Taken together, we speculate that the increased expression of miR-200b-3p may promote the development of preeclampsia via targeting PFN2.
In addition, dysfunction of the placenta plays a key role in the initiation and progression of preeclampsia [Citation37,Citation38]. Placentation can be regulated by several processes, such as CAMs and tight junctions, and some of molecules in these processes can modulate the trophoblast proliferation, fusion, migration, invasion, and trophoblast-endothelium adhesion during placentation [Citation39]. Adhesion molecules have been revealed to participate in the pathophysiology of preeclampsia through reducing invasion by the trophoblast and increasing vascular endothelial damage [Citation40]. Tight junction sustains normal placental barrier function, whose dysregulation under hypoxia is shown to participate in preeclampsia development. In the present study, DEMs were significantly enriched in several pathways, such as CAMs and tight junction. It can therefore be conducted that dysregulation of key pathways such as CAMs and tight junction may be a key mechanism by which DEMs contribute to the development of preeclampsia.
The strengths of our study include the identification of key DEMs (such as miR-200b-3p) and their enriched pathways (like CAMs and tight junction) as participants in preeclampsia development. Moreover, we for the first time confirmed the target relationship between miR-200b-3p and PFN2. These data provide us with a broader perspective for elucidating the pathobiology of preeclampsia. Despite these, our study still has some limitations that merit further consideration. First, the sample size was small. Second, the key pathways involving DEMs were not validated by experiments. Further studies with large sample size and more experiments are required to confirm our findings.
In conclusion, our findings reveal that miR-200b-3p is upregulated in the placental tissues from patients with preeclampsia and promotes preeclampsia development via targeting PFN2. Dysregulation of key pathways such as CAMs and tight junction contribute to the development of preeclampsia. miR-200b-3p may serve as a promising therapeutic target against preeclampsia.
Author contributions
All authors contributed to the study conception and design. Material preparation, data collection, and analysis were performed by [Huijun liu] and [Xietong Wang]. The first draft of the manuscript was written by [Huijun liu] and all authors commented on previous versions of the manuscript. All authors read and approved the final manuscript.
Availability of data and materials
The dataset supporting the conclusions of this manuscript is included within the manuscript.
Ethical approval
The study was approved by the Ethics Committee of Shandong Provincial Hospital (SWYX: NO. 2021-352), and the need to obtain informed consent was waived.
Disclosure statement
No potential conflict of interest was reported by the author(s).
Additional information
Funding
References
- Fox R, Kitt J, Leeson P, et al. Preeclampsia: risk factors, diagnosis, management, and the cardiovascular impact on the offspring. J Clin Med. 2019;8:1625.
- Ives CW, Sinkey R, Rajapreyar I, et al. Preeclampsia-pathophysiology and clinical presentations: JACC state-of-the-art review. J Am Coll Cardiol. 2020;76:1690–1702.
- Gatford KL, Andraweera PH, Roberts CT, et al. Animal models of preeclampsia: causes, consequences, and interventions. Hypertension. 2020;75:1363–1381.
- Gathiram P, Moodley J. Pre-eclampsia: its pathogenesis and pathophysiolgy. Cardiovasc J Afr. 2016;27:71–78.
- Brown MA, Magee LA, Kenny LC, et al. The hypertensive disorders of pregnancy: ISSHP classification, diagnosis & management recommendations for international practice. Pregnancy Hypertens. 2018;13:291–310.
- Staff AC. The two-stage placental model of preeclampsia: an update. J Reprod Immunol. 2019;134-135:1–10.
- Benmoussa A, Provost P. Milk microRNAs in health and disease. Compr Rev Food Sci Food Saf. 2019;18(3):703–722.
- Bounds KR, Chiasson VL, Pan LJ, et al. MicroRNAs: new players in the pathobiology of preeclampsia. Front Cardiovasc Med. 2017;4:60.
- Skalis G, Katsi V, Miliou A, et al. MicroRNAs in preeclampsia. Microrna. 2019;8(1):28–35.
- Lykoudi A, Kolialexi A, Lambrou GI, et al. Dysregulated placental microRNAs in early and late onset preeclampsia. Placenta. 2018;61:24–32.
- Niu Z-R, Han T, Sun X-L, et al. MicroRNA-30a-3p is overexpressed in the placentas of patients with preeclampsia and affects trophoblast invasion and apoptosis by its effects on IGF-1. Am J Obstet Gynecol. 2018;218:249.e1–.e12.
- Song H, Wang X, Li JC, et al. MiR-655-3p inhibits growth and invasiveness of trophoblasts via targeting PBX3 and thus deteriorates preeclampsia. Eur Rev Med Pharmacol Sci. 2020;24:10346–10351.
- Zhong Y, Zhu F, Ding Y. Differential microRNA expression profile in the plasma of preeclampsia and normal pregnancies. Exp Ther Med. 2019;18:826–832.
- Lv Y, Lu C, Ji X, et al. Roles of microRNAs in preeclampsia. J Cell Physiol. 2019;234:1052–1061.
- Awamleh Z, Gloor GB, Han VK. Placental microRNAs in pregnancies with early onset intrauterine growth restriction and preeclampsia: potential impact on gene expression and pathophysiology. BMC Med Genomics. 2019;12:1–10.
- Hornakova A, Kolkova Z, Holubekova V, et al. Diagnostic potential of MicroRNAs as biomarkers in the detection of preeclampsia. Genet Test Mol Biomarkers. 2020;24:321–327.
- Xie X, Kong B, Duan T. Obstetrics and Gynecology. 9th ed. China: People’s Medical Publishing House; 2018.
- Love MI, Huber W, Anders S. Moderated estimation of fold change and dispersion for RNA-seq data with DESeq2. Genome Biol. 2014;15(12):1–21.
- Enright A, John B, Gaul U, et al. MicroRNA targets in Drosophila. Genome Biol. 2003;4:1–27.
- Lewis BP, Shih I-H, Jones-Rhoades MW, et al. Prediction of mammalian microRNA targets. Cell. 2003;115:787–798.
- Smyth GK Limma: linear models for microarray data. In: Bioinformatics and computational biology solutions using R and bioconductor. NewYork (USA): Springer; 2005. p. 397–420.
- Phipps E, Prasanna D, Brima W, et al. Preeclampsia: updates in pathogenesis, definitions, and guidelines. Clin J Am Soc Nephrol. 2016;11:1102–1113.
- Staud F, Karahoda R. Trophoblast: the central unit of fetal growth, protection and programming. Int J Biochem Cell Biol. 2018;105:35–40.
- Chen H, Zhou X, Han T-L, et al. Decreased IL-33 production contributes to trophoblast cell dysfunction in pregnancies with preeclampsia. Mediators Inflamm. 2018;2018:9787239.
- Mao Y, Hou B, Shan L, et al. Aberrantly up-regulated miR-142-3p inhibited the proliferation and invasion of trophoblast cells by regulating FOXM1. Placenta. 2021;104:253–260.
- Liu E, Zhou Y, Li J, et al. MicroRNA‑491‑5p inhibits trophoblast cell migration and invasion through targeting matrix metalloproteinase‑9 in preeclampsia. Mol Med Rep. 2020;22:5033–5040.
- Tao J, Xia LZ, Liang L, et al. MiR-124-3p promotes trophoblast cell HTR-8/SVneo pyroptosis by targeting placental growth factor. Placenta. 2020;101:176–184.
- Cao G, Cui R, Liu C, et al. MicroRNA regulation of transthyretin in trophoblast biofunction and preeclampsia. Arch Biochem Biophys. 2019;676:108129.
- Zhou WJ, Wang HY, Zhang J, et al. NEAT1/miR-200b-3p/SMAD2 axis promotes progression of melanoma. Aging (Albany NY). 2020;12:22759–22775.
- Liu K, Zhang W, Tan J, et al. MiR-200b-3p functions as an oncogene by targeting ABCA1 in lung adenocarcinoma. Technol Cancer Res Treat. 2019;18:1533033819892590.
- Chen L, Wang X, Zhu Y, et al. miR‑200b‑3p inhibits proliferation and induces apoptosis in colorectal cancer by targeting Wnt1. Mol Med Rep. 2018;18:2571–2580.
- Liu H, Yu J, Yuan W, et al. Microarray analysis of preterm preeclampsia. Cell Mol Biol. 2020;66:59–64.
- Zhang H, Yang W, Yan J, et al. Loss of profilin 2 contributes to enhanced epithelial-mesenchymal transition and metastasis of colorectal cancer. Int J Oncol. 2018;53:1118–1128.
- Zhou K, Chen J, Wu J, et al. Profilin 2 promotes proliferation and metastasis of head and neck cancer cells by regulating PI3K/AKT/β-catenin signaling pathway. Oncol Res. 2019;27:1079.
- Ling Y, Cao Q, Liu Y, et al. Profilin 2 (PFN2) promotes the proliferation, migration, invasion and epithelial-to-mesenchymal transition of triple negative breast cancer cells. Breast Cancer. 2021;28:368–378.
- Miao R, Dong X, Gong J, et al. Possible immune regulation mechanisms for the progression of chronic thromboembolic pulmonary hypertension. Thromb Res. 2021;198:122–131.
- Fisher SJ, McMaster M, Roberts JM The placenta in normal pregnancy and preeclampsia. In: Chesley’s hypertensive disorders in pregnancy. Amsterdam (Netherlands): Elsevier; 2015. p. 81–112.
- Turner RJ, Bloemenkamp KW, Bruijn JA, et al. Loss of thrombomodulin in placental dysfunction in preeclampsia. Arterioscler Thromb Vasc Biol. 2016;36:728–735.
- Adu-Gyamfi EA, Czika A, Gorleku PN, et al. The involvement of cell adhesion molecules, tight junctions, and gap junctions in human placentation. Reprod Sci. 2021;28:305–320.
- Haram K, Mortensen JH, Myking O, et al. The role of oxidative stress, adhesion molecules and antioxidants in preeclampsia. Curr Hypertens Rev. 2019;15:105–112.