ABSTRACT
Cholangiocarcinoma (CCA) is one of the most common hepatic and biliary malignancies. The overall five-year survival rate for cholangiocarcinoma is less than 15%. miR-28-5p has been reported to participate the development of various human cancer types. But whether miR-28-5p is associated with the clinical course of CCA patients has not been clarified. Herein, we observed that miR-28-5p was reduced in CCA tissues and predicts the poor prognosis of CCA patients. Treatment with the demethylating agent 5-aza-2’-deoxycytidine (5-AZA) restored miR-28-5p expression in CCA cell lines. Furthermore, up-regulated miR-28-5p inhibited CCA cells growth and metastasis. Mechanistically, miR-28-5p suppressed CCA cells growth and metastasis via directly targeting CD44 molecular. Specific CD44 special siRNA abrogated the discrepancy of the proliferation and metastasis capacity between miR-28-5p-overexpression CCA cells and their control cells, which further confirmed that CD44 was required in miR-28-5p-inhibited CCA cell growth and metastasis.
Introduction
Cholangiocarcinoma (CCA) is one of the most common primary hepatobiliary cancer with poor prognosis [Citation1]. More than half CCA patients are diagnosed at advanced stage and lost their operative chance [Citation2]. The recurrence and metastasis rate of CCA after surgery remains very high [Citation3]. The five-years survival rate for CCA is less than 15% [Citation4]. Despite these advances in treatment, deaths related to cholangiocarcinoma continue to increase [Citation5]. So, its urgent to explore the pathogenesis of cholangiocarcinoma, find new therapeutic targets and new therapeutic drugs.
miRNAs are non‑coding RNA molecules containing ~20 nucleotides in length that are major post‑transcriptional regulators [Citation6]. Despite lacking protein‐coding potential, they are also involved in the regulation of different types of cancers, including CCA. Aberrant expression of miRNAs is common in various human malignancies and may modulate cancer‑associated genomic regions or fragile sites [Citation7]. Emerging evidences have demonstrated that miR-28 is an important miRNA, including miR‑28‑5p and miR‑28‑3p. Abnormality of miR-28 expression has been found in numerous cancers, including colorectal cancer, B‑cell lymphoma, breast cancer, glioma and renal cell carcinoma [Citation8–12]. However, the potential role of miR-28 in human CCA remains to be elucidated.
In the present study, we for first identified miR-28-5p as a tumor suppressor that epigenetically silenced in CCA tissues. Analysis of patient cohorts demonstrated that the miR-28-5p predicts the prognosis of CCA patients. Biological function study demonstrated that miR-28-5p inhibited CCA cells proliferation and metastasis in vitro and in vivo. Further mechanism study revealed that CD44 was a direct target of miR-28-5p in CCA cells. In concluding, our results highlighted the importance of miR-28-5p in suppressing the proliferation and metastasis of CCA cells via CD44 pathway.
Materials and methods
Patients and samples
A total of 20 CCA tissues were randomly obtained from CCA patients who underwent curative resection, and 10 normal bile duct tissues were obtained from liver transplantation donators, following informed consent according to an established protocol approved by the Ethic Committee of Shanghai Seventh People’s Hospital (Shanghai, China). Tissue samples were collected from 84 CCA patients admitted in the Department of Hepatobiliary surgery, Shanghai Seventh People’s Hospital. The time between surgery and death was defined as overall survival (OS); the time between surgery and recurrence was defined disease-free survival (DFS); and non-HCC related death was scored as recurrence. If recurrence was not diagnosed, patients were censored on the date of the last follow-up. Detailed clinicopathological features of the patients in supplementary Table 1. The procedure of human sample collection was approved by the Ethic Committee of Shanghai Seventh People’s Hospital.
Cell lines and cell culture
CCA cells TFK-1, HuCCT-1, QBC939 and RBE were cultured with Dulbecco’s modified Eagle’s medium (DMEM) supplemented with 10% fetal bovine serum (FBS) and 2 mM L-glutamine, and 25 µg/ml of gentamicin, and maintained at 37°C in a 5% CO2 incubator. Normal biliary epithelial cells (HIBEpic) were grown in RPMI 1640 (Gibico, Carlsbad, CA, USA) medium supplemented with 10% FBS (Gibico, Carlsbad, CA, USA) and 1% penicillin/streptomycin (Beijing Solarbio Science & Technology Co., Beijing, China) at 37°C and 5% CO2.
The lentivirus expressing miR-28-5p or control was purchased from Genechem Co., Ltd, Shanghai. TFK-1 and HuCCT1 cells were seed into six-well plate and then infected with miR-28-5p lentivirus and control virus as described previously [Citation13].
CCK8 assay
CCA cells were seeded in 96-well plates (3 × 103 cells per well). ATP activity was measured using a Cell Counting Kit-8 at indicated time points (0, 24, 48, 72, 96 hours). The procedure was as follows: The cell suspension (100 μl/well) was inoculated in a 96-well plate, and the plate was pre-incubated in a humidified incubator at 37°C for 1 h. This was followed by the addition of 10 μl of the CCK-8 solution to each well of the plate, and incubation of the plate for 1 h in the incubator. Finally, the absorbance was measured at 450 nm using a microplate reader (Synergy H1; BioTek Instruments, Inc., Winooski, VT, USA).
Colony formation assay
For colony formation assay, CCA cells were cultured in 12-well plates (3x103 cells/well). The cells were incubated at 37°C for 7 days and then fixed with 10% neutral formalin for more than 4 h. The cells were dyed with crystal violet (Beyotime, Haimen, China). The cells were photographed under a microscope (Olympus, Tokyo, Japan).
EdU immunofluorescence staining
For cell EdU immunofluorescence staining, CCA cells were seeded into 96-well plates and performed using the EdU Kit (RiboBio) at 48 hours. The results were quantified with a Zeiss axiophot photomicroscope (Carl Zeiss) and Image-Pro plus 6.0 software.
Animal models
BALB/c nude mice (male, 4 weeks old) were purchased from Chinese Academy of Sciences Slack Company (Shanghai, China). For xenograft formation assay, TFK-1 miR-28-5p and its control cells (2 × 106) were injected subcutaneously into nude mice (n = 6 each, randomized allocated). Nude mice were sacrificed six weeks post inoculation and tumors were collected and examined.
For pulmonary metastasis assay, TFK-1 miR-28-5p and its control cells (2 × 106) were injected into the tail vein of nude mice (n = 6 each, randomized allocated). The mice were sacrificed 12 weeks post inoculation and consecutive sections of the whole lung were subjected to hematoxylin and eosin (H&E) staining. All metastatic foci in the lung were calculated microscopically to evaluate the development of pulmonary metastasis. All animal experiments were approved by the Animal Care Committee of Shanghai Seventh People’s Hospital.
Cell migration assays
For cell migration experiments, 2 × 105 CCA cells were seeded into the upper chamber of a polycarbonate transwell in serum-free DMEM. The lower chamber was added with DMEM containing 20% FBS as chemoattractant. The cells were incubated for 24 hours and the chamber was fixed with 10% neutral formalin for more than 4 h. The cells were dyed with crystal violet (Beyotime, Haimen, China). Cell count is expressed as the average number of the cells in each field.
Cell invasion assays
For cell invasion experiments, 2 × 105 CCA cells were seeded into the upper chamber of a polycarbonate transwell in serum-free DMEM. The lower chamber was added with DMEM containing 20% FBS as chemoattractant. The cells were incubated for 36 hours and the chamber was fixed with 10% neutral formalin for more than 4 h. The cells were dyed with crystal violet (Beyotime, Haimen, China). Cell count is expressed as the average number of the cells in each field.
Real-time PCR assay
For detection of mature miR-28-5p, total RNA was subjected to reverse transcription using a TaqMan MicroRNA Reverse Transcription Kit (Applied Biosystems). qRT-PCR analysis of miR-28-5p expression was carried out using TaqMan MicroRNA assay kits (Applied Biosystems). Results were normalized to U6 snRNA using the comparative threshold cycle (Ct) method. The miR-28-5p forward primer, 5’-GCG GAA GGA GCT CAC AGT CT-3’; miR-28-5p reverse primer, 5’-TGGTGTCGTGGAGTCG-3’; U6 primer sequences were forward: 5’ ATTGGAACGATACAGAGAAGATT 3’.
The total cells RNA was extracted by using Trizol reagent (Invitrogen, 15,596–018). Total cDNAs were synthesized by ThermoScript TM RT-PCR system (Invitrogen, 11,146–057). The total mRNA amount presented in the cells was measured by RT-PCR using the ABI PRISM 7300 sequence detector (Applied Biosystems). The CD44 primer sequences were forward: 5’ GAGCAGCACTTCAGGAGGTT 3’, reverse: 5’ TGGTTGCTGTCTCAGTTGCT 3’. The β-actin was used as reference for relative expression calculation and its primer sequences were forward: 5’ GGCCCAGAATGCAGTTCGCCTT 3’, reverse: 5’ AATGGCACCCTGCTCACGCA 3’.
Western blotting assays
The cells were collected with cell lysis buffer and then disposed as described previously [Citation14]. Twenty-five protein micrograms of the cell extracts were subjected to Western Blot with one of the antibodies against N-cadherin, Vimentin, E-cadherin, CD44 and GAPDH (Cell Signaling Technology, USA).
Luciferase reporter assays
The wide type CD44 3’-untranslated region (UTR) containing miR-28-5p targeting sequence and the mutated type was amplified and cloned into the luciferase reporter plasmid pGL4.13 vector (Promega, Madison, WI). All the constructs were verified by sequencing. Briefly, laryngocarcinoma cells were co-transfected with miR-28-5p sponge or miR-control and pMIR-reporter luciferase vector containing a specific sequence of wild-type or mutant CD44 fragment, using siRNA transfection (Invitrogen, NY, USA). Cells were collected and lysed for luciferase detection 48 h after transfection. The relative luciferase activity was normalized against to the Renilla luciferase activity [Citation15].
Transient transfection of small interference RNA
CCA cells were seeded into a six well plate until they reached 70%-80% confluence. Transfection of si-CD44 or its negative control was performed in each well in the absence of serum with small interfering RNA transfection reagent according to the manufacturer’s instructions (Polyplus, Illkirch, France). The sequence of siCD44 is as follows: 5’- GCTGACCTCTGCAAGGCTTTCAATAGCAC-3’. Then the cells subjected to CCK8 and migration assay.
Statistical analysis
The statistical software SPSS17.0 (SPSS Inc., Chicago, IL, USA) was introduced for analysis, with the measurement statistics presented as mean ± standard deviation (x ± s). T test was taken for comparison between two groups, and the analysis of variance was adopted to compare multiple means. *p < 0.05, a p value of less than 0.05 was considered significant.
Results
The expression of miR-28-5p is reduced in human CCA tissues and correlates with the poor prognosis of CCA patients
To explore the role of miR-28-5p in CCA progression, the expression of miR-28-5p in the human CCA tissues was detected. As shown in ), miR-28-5p expression was significantly downregulated in CCA tissues compared with that normal tissues. CCA patients always had a high rate of recurrence, and the recurrence was a prognostic factor of poor prognosis of the patients. As expected, the expression of miR-28-5p were dramatically reduced in the recurrence foci compared with the primary CCA tissues ()).
Figure 1. Aberrant CpG methylations associated with downregulation of miR-28-5p in CCA tissues. a. Real-time PCR analysis of miR-28-5p expression in CCA tissues (n = 20) and normal tissues (n = 10). b. Real-time PCR analysis of miR-28-5p expression in primary CCA tissues (n = 10) and recurrence CCA tissues (n = 10). c&d. RT-PCR and the expression of miR-28-5p were scoring in 84 human CCA samples. OS (p = 0.001) and DFS (p < 0.001) after surgery were compared between “low-miR-28-5p” group (n = 42) and “high-miR-28-5p” group (n = 42).
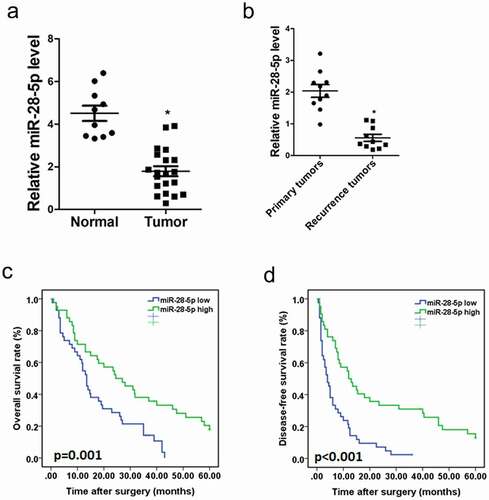
According to the miR-28-5p level, the 84 CCA patients were equally divided into a “high-miR-28-5p, n = 42” group and a “low-miR-28-5p, n = 42” group. The survival time in “low-miR-28-5p” group was shorter than that in “high-miR-28-5p” group ()). Collectively, miR-28-5p could be a good prognostic factor for CCA patients.
Methylated miR-28-5p gene is increased in human CCA tissues
Next, we found that miR-28-5p expression in four CCA cell lines was markedly lower than the normal cell line ()). Increasing evidence showed that DNA methylation or histone acetylation is a major cause of tumor suppressor silencing in cancer, CCA cells and normal biliary epithelial cells were treated with different concentrations of DNA methyltransferase inhibitor 5-aza-2’-deoxycytidine (5-Aza-dC) or TSA to determine whether miR-28-5p downregulation was associated with DNA methylation or histone acetylation in CCA. Treatment of CCA cells and normal biliary epithelial cells with TSA did not change the level of miR-28-5p, while 5-Aza-dC led to a dose-dependent restoration of miR-28-5p expression, suggesting that there was a negative correlation between DNA methylation and miR-28-5p expression ()).
Figure 2. Aberrant CpG methylations associated with downregulation of miR-28-5p in CCA tissues. a. The expression of miR-28-5p in HIBEpic, QBC939, RBE, HuCCT1 and TFK-1 cells was determined by real-time PCR assay. b. HIBEpic, QBC939, RBE, HuCCT1 and TFK-1 cells were treated with TSA (2 μM) for 24 hours, and then subjected to real-time PCR assay. c. HIBEpic, QBC939, RBE, HuCCT1 and TFK-1 cells were treated with 5-aza-dC (5 μM) for 24 hours, and then subjected to real-time PCR assay. All results are presented as the mean ± SD, and statistical significance was assessed using a two-tailed Student t test. *p < 0.05 (vs. Control or NC-in group).
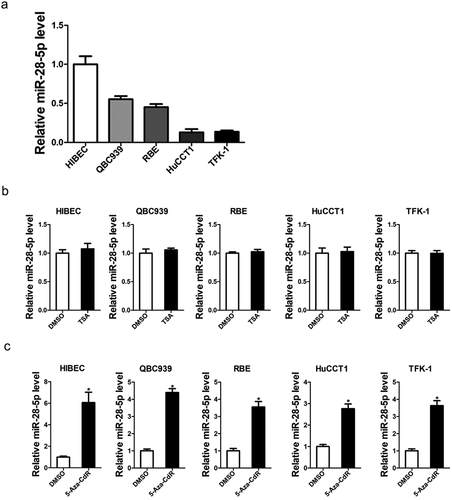
miR-28-5p suppresses CCA cells proliferation
To further elucidate the biological function of miR-28-5p in CCA cells progression, we stably overexpressed miR-28-5p in TFK-1 and HuCCT-1 cells. The overexpressing effect was confirmed by real-time PCR assay ()). As expected, CCK8 assay showed that miR-28-5p overexpression inhibited the proliferation of CCA cells compared with control CCA cells ()). Consistently, miR-28-5p overexpression CCA cells formed less clones compared with control CCA cells ()). Moreover, 5-ethynyl-2’-deoxyuridine (EdU) staining showed that miR-28-5p overexpression suppressed CCA cells proliferation ()). Flow cytometry data showed a decreased G1/S transition and a marked G2/M arrest in miR-28-5p overexpression CCA cells ()). More importantly, the in vivo experiments also confirmed that miR-28-5p overexpression inhibited CCA cells growth ()). Taken together, our data showed that miR-28-5p inhibited CCA cells proliferation.
Figure 3. miR-28-5p suppressed CCA cells proliferation. a. HuCCT1 and TFK-1 cells were infected with miR-28-5p mimic lenti-virus or vector virus, and the stable transfectants were determined by real-time PCR assay. b. Cell proliferation was measured using CCK-8 assays in miR-28-5p mimic CCA cells and control CCA cells. c. miR-28-5p mimic CCA cells and control CCA cells were performed colony formation. d. Cell proliferation was assessed using EdU immunofluorescence staining (red) in miR-28-5p mimic CCA cells and control CCA cells. Nuclei were stained with Hoechst 33,342 (blue). e. Cell cycle was assessed by flow cytometry in miR-28-5p mimic CCA cells and control CCA cells. f&g. TFK-1 miR-28-5p mimic and its control cells were implanted subcutaneously to induce xenograft tumor in nude mice (n = 6). Mice were sacrificed at 6th weeks after inoculation; tumor was excised, pictured and weighed. Cisplatin (1 μg/ml) used as a positive control. All results are presented as the mean ± SD, and statistical significance was assessed using a two-tailed Student t test. *p < 0.05 (vs. Control or NC-in group).
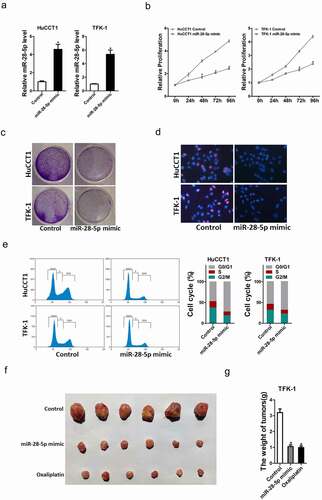
miR-28-5p inhibits CCA cells metastasis
Next, we also explore whether miR-28-5p influence the metastasis of CCA cells. Transwell and Matrigel invasion assay were used to compare the metastasis ability between miR-28-5p overexpression CCA cells and control CCA cells. As shown in ), transwell assay showed that the migration ability was impaired in miR-28-5p-overexpressed CCA cells. Consistently, the invasion capacity was also destroyed in miR-28-5p-overexpressed CCA cells ()). Moreover, the EMT protein N-cadherin, vimentin was downregulated and E-cadherin was upregulated in miR-28-5p overexpression CCA cells ()). Furthermore, the in vivo lung metastasis experiment was performed. The results showed that no difference in lung morphology between miR-28-5p mimic CCA cells and control cells, while the H&E staining of nude mice showed much less lung metastasis foci in miR-28-5p mimic CCA cells ()). Collectively, our results demonstrate that miR-28-5p suppressed metastatic potential of CCA cells.
Figure 4. miR-28-5p inhibited CCA cells metastasis. a. The migration ability of HuCCT1 miR-28-5p mimic and its control cells were analyzed by using transwell assay. b. The migration ability of TFK-1 miR-28-5p mimic and its control cells were analyzed by using transwell assay. c. The invasive properties of HuCCT1 miR-28-5p mimic and its control cells were analyzed using Matrigel-coated Boyden chamber. d. The invasive properties of TFK-1 miR-28-5p mimic and its control cells were analyzed using Matrigel-coated Boyden chamber. e. The EMT protein N-cadherin, vimentin and E-cadherin in miR-28-5p overexpression CCA cells and control CCA cells were checked by western blot assay. f. The number of lung metastatic foci in each group (n = 6) were also calculated, p < 0.05. All results are presented as the mean ± SD, and statistical significance was assessed using a two-tailed Student t test. *p < 0.05 (vs. Control or NC-in group).
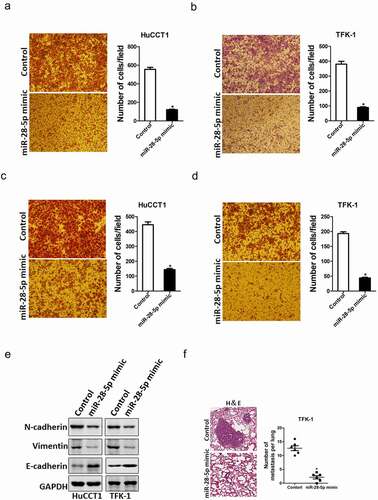
miR-28-5p inhibits CCA cells progression by directly targeting CD44
Next, we attempted to explore the potential target molecular of miR-28-5p in CCA cells. Bioinformatics analysis found that miR-28-5p has a putative binding site in CD44 mRNA 3’-UTR ()). To further explore whether miR-28-5p directly regulates CD44 expression via interaction with its 3’-UTR, the wild-type or mutant CD44 3’-UTR reporter plasmids were transfected into miR-28-5p overexpression CCA cells and control CCA cells. The luciferase activity of wild-type reporter was markedly downregulated in miR-28-5p overexpression CCA cells ()). However, miR-28-5p-mediated repression of the reporter expression was abolished by mutation of the miR-28-5p binding site in the CD44 3’-UTR. Moreover, CD44 mRNA and protein expression was also decreased in miR-28-5p overexpression CCA cells ()). There was a significant negative correlation between miR-28-5p and CD44 mRNA expression in human CCA tissues ()). To further confirm whether CD44 was required for miR-28-5p mediated CCA cells proliferation and metastasis, the special CD44 siRNA was used ()). CD44 siRNA abrogated the distinct growth capacity between miR-28-5p overexpression CCA cells and control CCA cells ()), and diminished the discrepancy of metastasis between miR-28-5p overexpression CCA cells and control CCA cells ()), which suggesting that miR-28-5p inhibited CCA cells progression by directly targeting CD44 molecular.
Figure 5. miR-28-5p directly targeted CD44 in CCA cells. a. A potential target site for miR-28-5p in the 3’-UTR of human CD44 mRNA, as predicted by the program Targetscan. To disrupt the interaction between miR-28-5p and CD44 mRNA, the target site was mutated. b. Luciferase reporter assays performed in miR-28-5p mimic CCA cells and their control cells transfected with wild-type or mutant CD44 3’-UTR constructs. c. The mRNA expression of CD44 was checked in HuCCT1 miR-28-5p mimic or TFK-1 miR-28-5p mimic and their control cells by real-time PCR. d. The protein expression of CD44 was determined in HuCCT1 miR-28-5p mimic or TFK-1 miR-28-5p mimic and their control cells by western blot. E. Significant correlation was observed between miR-28-5p and CD44 expression in human CCA tissues (n = 20). f. HuCCT1 miR-28-5p mimic or TFK-1 miR-28-5p mimic and their control cells were transfected with siCD44 or negative control, followed by Western bolt assay. g. HuCCT1 miR-28-5p mimic or TFK-1 miR-28-5p mimic and their control cells were transfected with siCD44 or negative control, respectively, followed by CCK8 assay. h. HuCCT1 miR-28-5p mimic or TFK-1 miR-28-5p mimic and their control cells were transfected with siCD44 or negative control, respectively, followed by Migration assay. All results are presented as the mean ± SD, and statistical significance was assessed using a two-tailed Student t test. *p < 0.05 (vs. Control or NC-in group).
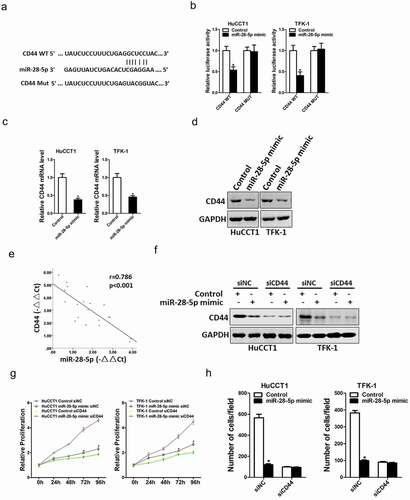
Discussion
Although current treatments for cholangiocarcinoma have improved, increasing prevalence and poor prognosis make this incurable disease a global health challenge [Citation16,Citation17]. It suggests that we still don’t know much about the disease. Therefore, more effort is required to explore the in-depth mechanism driving the initiation and progression of CCA. In the present study, for the first time, we clarify that miR-28-5p functioned as tumor suppressor to inhibit CCA growth and migration via regulation of CD44 signals.
Increasing evidence showed that miRNAs are probably of great value in cancer diagnosis and treatment, which have attracted considerable attention [Citation18]. For instance, miR-429 is overexpressed in HCC tissues and promotes liver tumor-initiating cells expansion via targeting Rb binding protein 4 [Citation19]. miR‑378 is upregulated in CCA tissues and was significantly associated with significantly associated with TNM stage, lymph node metastasis and prognosis [Citation20]. miR-876 is downregulated in CCA tissues and inhibits CCA cells growth via targeting BCL-XL [Citation21]. We here identified that miR-28-5p was also functioned as tumor suppressor during the development of CCA. Overexpressing miR-28-5p inhibited CCA cells proliferation and metastasis in vivo and in vitro.
Previous studies demonstrated that loss of miRNAs expression was due to gene promoter methylation in gastric, ovarian, lung, esophageal and pancreatic cancers [Citation22–24]. Nevertheless, how miR-28-5p was downregulated in CCA remains unclear. In the current study, 5-aza-CdR treatment of CCA cell lines with low miR-28-5p expression levels led to elevated miR-28-5p levels, indicating that there is a reverse correlation between DNA methylation and miR-28-5p expression.
Through a software predictions and functional studies, we identified CD44 as a functional downstream target of miR-28-5p in CCA cells. CD44 is an oncoprotein that promotes the growth of several types of cancer cells in vitro and in vivo [Citation25–27]. Previous studies have elucidated that CD44 plays essential roles in CCA cells proliferation and metastasis and predicted the poor prognosis of CCA patients [Citation28–30]. We hereby revealed that CD44 is a direct target of miR-28-5p in CCA cells. miR-552 overexpression reduced CD44 mRNA and protein expression in CCA cells. Moreover, we also found that miR-28-5p directly regulates CD44 expression via interaction with its 3’-UTR. More importantly, CD44 siRNA could abolished the distinct growth capacity or metastasis ability between miR-28-5p overexpression CCA cells and control cells.
Here, we first showed that miR-28-5p was downregulated in CCA tissues, which in turn inhibited the growth and metastasis of CCA cells, accompanied by directly targeted CD44 signaling. Analysis of patient cohorts demonstrated that the miR-28-5p predicts the prognosis of CCA patients. These findings not only shed a new light on the mechanism of CCA progression but suggest a potential prognostic marker and a possible therapeutic target against CCA.
Availability of data and materials:
The data in the current study are available from the corresponding authors upon reasonable request.
Supplemental Material
Download MS Word (18.3 KB)Disclosure statement
No potential conflict of interest was reported by the author(s).
Supplementary material
Supplemental data for this article can be accessed online at https://doi.org/10.1080/15384101.2022.2085359
Additional information
Funding
References
- Nakamura H, Arai Y, Totoki Y, et al. Genomic spectra of biliary tract cancer. Nat Genet. 2015;47(9):1003–1010.
- Khan SA, Davidson BR, Goldin RD, et al. Guidelines for the diagnosis and treatment of cholangiocarcinoma: an update. Gut. 2012;61(12):1657–1669.
- Razumilava N, Gores GJ. Cholangiocarcinoma. Lancet. 2014;383(9935):2168–2179.
- Morise Z, Sugioka A, Hoshimoto S, et al. Patient with advanced intrahepatic cholangiocarcinoma with long-term survival successfully treated with a combination of surgery and chemotherapy. J Hepatobiliary Pancreat Surg. 2008;15(5):545–548.
- Doherty B, Nambudiri VE, Palmer WC. Update on the diagnosis and treatment of Cholangiocarcinoma. Curr Gastroenterol Rep. 2017;19(1):2.
- Bartel DP. MicroRNAs: target recognition and regulatory functions. Cell. 2009;136(2):215–233.
- Zhang B, Pan X, Cobb GP. Anderson TA. microRNAs as oncogenes and tumor suppressors. Dev Biol. 2007;302(1):1–12.
- Tsiakanikas P, Kontos CK, Kerimis D, et al. High microRNA-28-5p expression in colorectal adenocarcinoma predicts short-term relapse of node-negative patients and poor overall survival of patients with non-metastatic disease. Clin Chem Lab Med. 2018;56(6):990–1000.
- Yang M, Yao Y, Eades G, et al. MiR-28 regulates Nrf2 expression through a Keap1-independent mechanism. Breast Cancer Res Treat. 2011;129(3):983–991.
- Bartolome-Izquierdo N, de Yebenes VG, Alvarez-Prado AF, et al. miR-28 regulates the germinal center reaction and blocks tumor growth in preclinical models of non-Hodgkin lymphoma. Blood. 2017;129(17):2408–2419.
- Chen HS, Lu AQ, Yang PY, et al. MicroRNA-28-5p regulates glioma cell proliferation, invasion and migration by targeting SphK1. Eur Rev Med Pharmacol Sci. 2019;23(15):6621–6628.
- Wang C, Wu C, Yang Q, et al. miR-28-5p acts as a tumor suppressor in renal cell carcinoma for multiple antitumor effects by targeting RAP1B. Oncotarget. 2016;7(45):73888–73902.
- Xiang DM, Sun W, Zhou T, et al. Oncofetal HLF transactivates c-Jun to promote hepatocellular carcinoma development and sorafenib resistance. Gut. 2019;68(10):1858–1871.
- Xiang D, Cheng Z, Liu H, et al. Shp2 promotes liver cancer stem cell expansion by augmenting beta-catenin signaling and predicts chemotherapeutic response of patients. Hepatology. 2017;65(5):1566–1580.
- Xiang DM, Sun W, Ning BF, et al. The HLF/IL-6/STAT3 feedforward circuit drives hepatic stellate cell activation to promote liver fibrosis. Gut. 2017;67(9):1704–1715.
- Liu S, Jiang J, Huang L, et al. iNOS is associated with tumorigenicity as an independent prognosticator in human intrahepatic cholangiocarcinoma. Cancer Manag Res. 2019;11:8005–8022.
- Peng DZ, Lu J, Li B, et al. A simple scoring system to predict early recurrence of Bismuth-Corlette type IV perihilar cholangiocarcinoma. Gastroenterol Rep (Oxf). 2019;7(5):345–353.
- Lee H, Han S, Kwon CS, et al. Biogenesis and regulation of the let-7 miRNAs and their functional implications. Protein Cell. 2016;7(2):100–113.
- Li L, Tang J, Zhang B, et al. Epigenetic modification of MiR-429 promotes liver tumour-initiating cell properties by targeting Rb binding protein 4. Gut. 2015;64(1):156–167.
- Zhou Z, Ma J. miR-378 serves as a prognostic biomarker in cholangiocarcinoma and promotes tumor proliferation, migration, and invasion. Cancer Biomark. 2019;24(2):173–181.
- Ursu S, Majid S, Garger C, et al. Novel tumor suppressor role of miRNA-876 in cholangiocarcinoma. Oncogenesis. 2019;8(8):42.
- Chhabra R. miRNA and methylation: a multifaceted liaison. Chembiochem. 2015;16(2):195–203.
- Holubekova V, Mendelova A, Jasek K, et al. Epigenetic regulation by DNA methylation and miRNA molecules in cancer. Future Oncol. 2017;13(25):2217–2222.
- Li YS, Zou Y, Dai DQ. MicroRNA-320a suppresses tumor progression by targeting PBX3 in gastric cancer and is downregulated by DNA methylation. World J Gastrointest Oncol. 2019;11(10):842–856.
- Ghuwalewala S, Ghatak D, Das P, et al. CD44(high)CD24(low) molecular signature determines the cancer stem cell and EMT phenotype in oral squamous cell carcinoma. Stem Cell Res. 2016;16(2):405–417.
- Nagaya T, Nakamura Y, Okuyama S, et al. Syngeneic mouse models of oral cancer are effectively targeted by anti-CD44-based NIR-PIT. Mol Cancer Res. 2017;15(12):1667–1677.
- Vega FM, Colmenero-Repiso A, Gomez-Munoz MA, et al. CD44-high neural crest stem-like cells are associated with tumour aggressiveness and poor survival in neuroblastoma tumours. EBioMedicine. 2019;49:82–95.
- Morine Y, Imura S, Ikemoto T, et al. CD44 expression is a prognostic factor in patients with intrahepatic cholangiocarcinoma after surgical resection. Anticancer Res. 2017;37(10):5701–5705.
- Thanee M, Loilome W, Techasen A, et al. CD44 variant-dependent redox status regulation in liver fluke-associated cholangiocarcinoma: a target for cholangiocarcinoma treatment. Cancer Sci. 2016;107(7):991–1000.
- Suwannakul N, Ma N, Thanan R, et al. Overexpression of CD44 variant 9: a novel cancer stem cell marker in human cholangiocarcinoma in relation to inflammation. Mediators Inflamm. 2018;2018:4867234.