ABSTRACT
Osteosarcoma (OS) is one of the most prevalent and highly aggressive bone malignancies. The treatment strategies of OS is under standard regimens, including surgical resection, chemotherapy, and other adjuvant therapy. However, the 5-year survival rate is still unsatisfactory. Previous studies have demonstrated that the expression of miR-34a decreases in osteosarcoma, which is involved in regulating numerous genes directly or indirectly at the post-transcriptional level and other pathways. Thus, miR-34a plays an important role in mediating OS cell proliferation, differentiation, migration, and apoptosis, and might be a pivotal biomarker for OS with diagnostic and therapeutic potentials. In this review, we aim to summarize the relationship between miR-34a and OS, with an emphasis on the specific mechanisms in OS development referring to miR-34a. Moreover, the potential role of miR-34a as a diagnostic, prognostic, and therapeutic candidate for OS would be presented in detail. However, the molecular mechanisms related to miR-34a and OS remain elusive, and more investigations are needed to reach a comprehensive understanding.
Background
Osteosarcoma (OS) is the most common type of primary bone malignancy. It predominantly threatens the children and adolescent population, with a high occurrence in the metaphysis of long bones, especially around the knee joint [Citation1]. Previous studies have shown that its occurrence and aggressiveness might be attributed to unrestrained cell proliferation, preternatural differentiation, and aberrant apoptosis [Citation2,Citation3]. However, the detailed pathophysiological processes involved in OS development are still largely unexplored. The current treatment modality for OS consists of surgical removal, chemotherapy, radiation therapy, etc [Citation4]. However, the 5-years survival for OS patients with the localized lesion is still unsatisfactory [Citation5], which is worse for those with distant metastasis (less than 20%) or local recurrence (less than 15%) [Citation6,Citation7]. Accordingly, it is an urgent need to investigate the pathogenesis and identify an innovative treatment strategy for OS.
The microRNAs (miRNAs), a subtype of non-coding RNAs with 18 ~ 25 nucleotides (nts), participating in the regulation of mRNA degradation and translation [Citation8]. Besides, accumulating evidence suggests that miRNAs possess a large spectrum of functions including mediating cell death, differentiation, etc [Citation9,Citation10]. In addition, miRNAs are related to a variety of cancers [Citation11]. Particularly, miRNAs show the properties of pro- and anti-tumorigenesis in OS. For example, it is reported that miR-429 suppressed OS progression by targeting HOXA9 through the Wnt/β-catenin pathway [Citation12]. Besides, miR-205 targeted the runt-related transcription factor (RUNX2) and played a vital role in osteogenesis modulation [Citation11].
MiR-34 is a cluster of miRNAs involved in cancer occurrence and development [Citation13]. Inactivation of mature miR-34 has been reported in diverse cancer cells, as a result of lacking a 5′-phosphate [Citation14]. Hence, the miR-34 family plays an important role among several cancers [Citation15]. Of note, miR-34a, one of the most important members of the miR-34 family, is found directly regulated by p53 and thereby mediates numerous pathways [Citation16]. Recent studies have shown the abnormal expression of miR-34a in multiple cancers, including breast cancer, ovarian cancer, and hepatocellular carcinoma [Citation17]. In addition, miR-34a could prevent cancer cells from unrestricted growth and migration by multiple targets, such as MYC, mesenchymal-epithelial transition (MET), cyclin-dependent kinases 4 and 6 (CDK4/6), NOTCH1, B-cell lymphoma-2 (BCL2), and cluster of differentiation-44 (CD44) [Citation4]. In OS, miR-34a is markedly down-regulated [Citation18] and participates in the regulation of tumor development, invasion, and chemo-resistance [Citation19,Citation20]. Hence, miR-34a may act as an essential biomarker and therapeutic target for OS.
In this review, we have demonstrated the relationship between miR-34a and OS and revealed the specific mechanisms mediated by miR-34a. More significantly, we have envisioned the extensive application prospect of miR-34a for the treatment of OS.
Biogenesis and biological functions of miR-34 family
MiR-34 family comprises miR-34a, miR-34b and miR-34c [Citation21]. Nevertheless, they are encoded by different genes, and their expressions are identified in different tissues. In the human genome, miR-34b and miR-34c are expressed on a common transcript that occupies a fixed position on chromosome 11q23.1. Whereas, miR-34a possesses a unique transcript, which is situated on chromosome 1p36.22 [Citation22]. MiR-34a is widely expressed in various tissues, whereas miR-34b/c presents tissue specificity to the brain, lungs, testicles, and fallopian tubes [Citation23]. The first step of miR-34a transcription is producing a long hairpin molecule (pri-miRNA), and the following procedures are very intricate and involved in diverse proteins or their complexes (). For example, members of the Argonaute family, Pol II-dependent transcription, and the RNase IIIs Drosha and Dicer [Citation24].
Figure 1. Biogenesis and feedback loop of miR-34a. DNA damage induces the transcription of miR-34a by binding the promoter, CpG island. Firstly, miR-34a is transcribed as long hairpin molecule (pri-miRNA), which is processed by the human RNase Ill DROSHA to transform into pre-miRNAs. Then miR-34a is delivered into cytoplasm through Exportin-5 and subsequently is cut by another human RNaseIll, DICER. Lastly, the mature miR-34a strand generates after several steps and this mature strand is incorporated into the RNA-induced silencing complex (RISC). Like this, miR-34a could bind with the 3’-UTR of target mRNA, leading to the degradation or translation inhibition of mRNA. Besides, miR-34a could suppress SIRT1 to increase acetylation of p53, upregulating p53-dependent apoptosis.
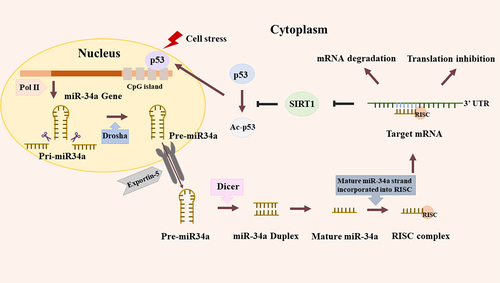
MiR-34 family is abnormally expressed and related to the diagnosis and prognosis in numerous tumors [Citation16]. For example, Roy et al. showed that expression of miR-34a/c was decreased in human colon cancer due to promoter hyper-methylation [Citation18]. Besides, several studies found that the miR-34 family was down-regulated in breast cancer and lung cancer. More importantly, downregulated expression of miR-34a/b was related to an unfavorable outcome in breast cancer [Citation25–27] (). Besides, miR-34 is the first member of miRNAs proved to be a direct transcriptional target of p53, and it is characterized by a large number of biological behaviors in a p53‑dependent manner under both physiological and pathological conditions, such as cell cycle arrest, senescence, ect [Citation21]. Furthermore, previous studies have revealed that miR-34 played a pivotal role in mediating gene expression at the post‑transcriptional level by aiming at the coding or non‑coding sequence directly or indirectly or other pathways [Citation28]. Particularly, the miR-34 family could bind to the 3’-UTRs of CDK4, CDK6, E2F transcription factor 5 (E2F5), Fra-1, etc., which participate in the regulation of biological behaviors [Citation29]. More importantly, many researches indicated miR-34a is involved in the regulation of many cellular physiological processes by targeting over 800 mRNAs and other molecules, such as the genes related to the cell proliferation (cyclins, cyclin-dependent kinases (CDKs), MYCN, NOTCH1, MDMX), apoptosis (BCL2, silencing information regulator 2 related enzyme 1 (SIRT1), Survivin (also named BIRC5)), senescence (E2F transcription factor 3 (E2F3), cancer stem-like cell phenotype (CD44, NANOG, sex determining region Y‑box 2 (SOX2)), motility (snail family transcriptional repressor 1 (SNAI1), MET, axis inhibition protein 2 (AXIN2)) or immune evasion (programmed death-ligand 1 (PD-L1), diacylglycerol kinase ζ (DGKζ)), etc [Citation30].
Table 1. Abnormal expression of the MiR-34a in various cancers.
MiR-34a was identified as a tumor suppressor and was down-regulated in multiple cancers, including lung cancer, colorectal cancer, hematological neoplasm, etc [Citation31–33]. Current evidence showed that miR-34a had an obvious suppressive effect in cervical carcinoma and choriocarcinoma cells by repressing NOTCH1 and Jagged1 [Citation31]. Additionally, miR-34a could suppress resistance to cisplatin on ovarian cancer via directly binding and down-regulating histone deacetylase 1 (HDAC1) gene expression [Citation34]. Given this situation and the ability of miR-34a in regulating many molecular mechanisms in various cancers, there is an increasing interest in exploring more about the complex signaling network where miR-34a is regarded as the nucleus. Therefore, miR-34a could open an avenue to the comprehensive therapeutic application in cancers.
Relationship between miR-34a and osteosarcoma
MiR-34a is a network hub that regulates a variety of signals in many diseases. In 2007, Welch et al firstly described miR-34a as a cancer-related miRNA in neuroblastoma [Citation35]. In OS, miR-34a was significantly decreased in tumors than those adjacent normal counterparts [Citation18]. Moreover, restoration of miR-34a expression could rescue the abnormal cellular processes in preclinical OS models [Citation36]. Therefore, miR-34a presented a vital role in regulating OS occurrence and development.
The interaction between miR-34a and P53 in OS
It was reported that novel TP53 gene arose in nearly 22% of OS patients, and 75% of OS patients have been detected the allelic deletion of chromosome 17p13 in the germline mutation test [Citation37]. As aforementioned, miR-34a was a direct target for p53 since it has a classic p53 binding site. Meanwhile, it participated in mediating the expression of p53 by several feedback loops () [Citation38]. Specifically, on the one hand, if DNA got damaged, there would be the initiation of p53-dependent miR-34a regulation to active DNA repair, or even prevent cell cycle arrest and apoptosis [Citation31]. On the other hand, miR-34a could upregulate p53-dependent apoptosis through SIRT1, which was related to the process of NAD-dependent deacetylation since it contained a well-conserved NAD-dependent sirtuin core domain. Moreover, suppression of SIRT1 by miR-34a increased acetylation of p53, therefore leading to an obvious strengthening effect on transcriptional activity and up-regulating the expression of numerous subsequent targets of p53, such as p21 and PUMA. Therefore, the p53-dependent modulation of miR-34a and the positive feedback loop played an important role to maintain the homeostasis of cells. If the expression of miR-34a decreased, there would be a series of serious consequences, for example, the occurrence and development of OS [Citation39].
The direct or indirect targets of miR-34a in OS
Many studies reported that plenty of anti-tumor activities of miR-34a without endogenous p53 in OS, indicating p53 was not an indispensable condition for miR-34a [Citation40,Citation41].
Stathmin 1 (STMN1), also known as oncoprotein 18 (Op18), is a microtubule-destabilizing protein and is widely expressed in vertebrates. It could regulate the dimerization and polymerization of tubulins by binding β-tubulin [Citation42]. Vetter NS et al found that miR-34a interacted with the STMN1 mRNA 3′UTR and imposed a negative impact on STMN1 expression. Therefore, miR-34a could suppress OS cells growth by activating apoptosis via the miR-34a/STMN1/βIII-tubulin axis. Besides, The promoter region for βIII-tubulin contained multiple potential binding sequences for Sp1 transcription factor, which might be an indirect downstream effector of miR-34a [Citation43]. Moreover, several studies have demonstrated that up-regulation of many molecules in miR-34a-null OS cells, such as MET, CDK6, CDK44, etc., which were involved in the process of OS lung metastasis in vivo [Citation4]. Lopez CM et al found that miR-34a downregulated the expression of KruÈppel-like factor 4 (KLF4), Semaphorin 3E (SEMA3E), and vascular endothelial growth factor receptor A (VEGFA) in canine OS cell lines, inhibiting invasion and migration of OS cells to mediate the metastatic phenotype [Citation41,Citation44]. Zou Y et al. revealed that miR-34a acted as a post‑transcriptional regulatory factor of Sox-2, which was essential for OS cell self-renewal and tumorigenesis [Citation44,Citation45]. Besides, a remarkable study demonstrated that plasminogen activator inhibitor-1 (PAI-1) linked with miR-34a in the regulation of OS dedifferentiation, and miR-34a–PAI-1–Sox2 axis could be a pivotal target involved in OS [Citation46]. A study by Wen et al. demonstrated that miR-34a could block tumor invasion and metastasis in OS, and the mechanisms might be partly related to decreasing the level of BCL2 and cell inhibitor of apoptosis protein 2 (C-IAP2) directly or indirectly [Citation19,Citation46]. Collectively, miR-34a played a crucial role in the regulation network of OS (), but a thorough grasp of the specific pathways mediated by miR-34a required more studies.
Figure 2. MiR-34a is a core component in the regulation network of OS. MiR-34a regulated numerous downstream factors to directly or indirectly mediate apoptosis, proliferation, cell cycle arrest, migration, invasion and differentiation; the expression levels of miR-34a and its related targets could be interfered by a variety of lncRNAs, like SNHG7.
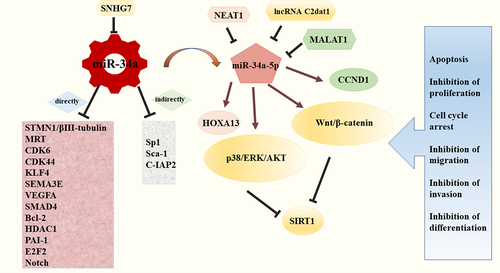
MiR-34a is regulated by lncRNAs in OS
Long noncoding RNAs (lncRNAs), non-coding RNAs with more than 200 nts, possess the ability to regulate the expression of various genes [Citation47]. They are defined as vital regulators in proliferation, differentiation, development, metastasis, and other physiologic processes in tumor cells. Specificlly, lncRNAs could mediate the deactivation or abnormal activation of tumor suppressor genes or oncogenes. Besides, lncRNAs could interact with kinds of RNAs and proteins to exert many functions [Citation19]. Rigorous studies have shown that lncRNAs were extensively involved in OS development. For instance, lncRNA-FGFR3-AS1 was reported to show a stimulatory effect in OS [Citation48]. LncRNA-TUG1 and LncRNA-CBR3-AS1 were significantly over-expressed in OS tissues and were closely related with tumor size, distant metastasis, histological grade, Enneking stage, and postoperative chemotherapy sensitivity [Citation49,Citation50]. More importantly, emerging evidence have indicated that many lncRNAs were dysregulated in OS and might participate in the regulation of miR-34a. For example, metastasis-associated lung adenocarcinoma transcript 1 (MALAT1) has been identified to be up-regulated in OS. Duan et al recently found that MALAT1 enhanced the suppression of miR-34a and then brought the negative effect of cyclin D1 (CCND1), indicating MALAT1 promoted OS via miR‑34a/CCND1 axis [Citation51]. Furthermore, the expression of lncRNA-SNHG7 (SNHG7) increased in a variety of cancers, which favored the tumor occurrence and development. Besides, Deng et al reported that SNHG7 had a strong antagonistic action against miR-34a and could induce the progression and epithelial-mesenchymal transition (EMT) phenotype in OS by regulating various related targets of miR-34a, including Notch1, BCL2, CDK6, and SMAD4 [Citation52]. LncRNA Nuclear Enriched Abundant Transcript 1 (NEAT1) possessed abundant functions in tumorigenesis [Citation53]. Ji et al found that the level of NEAT1 was elevated in OS. Besides, it was characterized as an endogenous competitor against miR‐34a‐5p, a derivative of miR‐34a, thereby mediating the expression of HOXA13 and exerting the positive feedback in the progression of OS [Citation54]. In addition, another recent study by Jia et al revealed that the suppression of lncRNA C2dat1 could impair cell growth and invasion in OS. This process was involved in the overexpression of miR-34a-5p, which could activate the p38/ERK/AKT and Wnt/β-catenin signaling pathways to suppress the expression of SIRT1 [Citation55].
In conclusion, lncRNAs exerted positive or negative effect in OS by regulating miR-34a or its related targets (). Thus, the interplay between lncRNA and miR-34a played an important role in various biological processes in OS.
MiR-34a in diagnosis and prognosis of OS
OS is a highly malignant and metastatic tumor [Citation56]. Over the past few decades, 70% of patients with OS have different degrees of lung metastasis when they are first diagnosed, which may attribute to poor survival outcomes [Citation57]. Therefore, a timely and accurate diagnosis of OS is an important guarantee for prognosis and improvement of the 5-year survival rate. Rigorous studies indicated effective biomarkers could contribute to improving diagnosis and predict prognosis of OS, which would help us to perform a risk assessment and select more effective treatment options [Citation58]. For instance, a study by Cong et al. revealed that miR-124 was significantly lower in serum from these patients with advanced or distant metastasis of OS. Besides, OS patients with lower serum miR-124 levels had a worse prognosis. Hence serum miR-124 could serve as diagnostic and prognostic biomarkers for OS [Citation59]. As aforementioned, several studies have shown a decreased level of miR-34a in OS, which contributed to mediating the invasive and migratory ability of OS cells [Citation60]. Therefore, the low expression of miR-34a was closely related to the poor prognosis of OS patients, which made possible new ways of prediction and early diagnosis of OS. The quantification of miR-34a in the blood circulation system and bone tissue might provide a basis for monitoring the progress of OS [Citation61–63]. Besides, direct and indirect targets of miR-34a were associated with the growth and aggressiveness of OS, which could be potential predictors. Recent research showed that the dysregulations of βIII-tubulin and STMN1 were significantly correlated to metastasis, resistance to chemotherapy, and recurrence of OS [Citation64]. As a result, elevated levels of the expressions of βIII-tubulin and STMN1 could be valuable markers for monitoring the infiltration and distant metastasis degree. Moreover, several reports indicated that C-IAP2 was implicated with the grade and Enneking stage of OS [Citation65] and it was suppressed in OS cells, hence it was an object worth exploring more as a maker in OS. Additionally, MALAT1 has already been verified to have a positive association with the progression of OS by linking to the enhancer of zeste homolog 2 to suppress the function of E‑cadherin [Citation66]. Therefore, the higher the MALAT1 level, the poorer prognosis was for the patients with OS [Citation67]. Besides, as aforementioned, diverse lncRNAs were closely associated with size, stage, infiltration, and distant metastasis degree of OS, which were regarded as valuable predictors of outcome for OS patients [Citation68]. For example, OS patients with highly expressed SNHG7 showed a shorter survival time [Citation69]. However, the relevance of these factors in the diagnosis and prognosis of OS remained to be further elucidated.
MiR-34a in treatment of OS
Treatment of OS covers surgical resection, chemotherapy, etc [Citation70]. However, some patients may experience drug resistance and relapse after receiving the multimodal therapy, having extremely poor survival outcomes. Epidemiologically, the 5-year survival rate of OS has not been obviously improved in the last decades [Citation71]. Therefore, further breakthroughs and novel approaches for the treatment of OS are in great need. Given that restoration of the expression of miR-34a had an anti-tumor effect, many researchers envisaged treatments in the rescue of miR-34a in OS cells to put the cell cycle, growth, and apoptosis back to normal. In 2013, a clinical trial (NCT01829971) showed that the therapeutic benefit of MRX34, a special amphoteric lipid nanoparticle filled with miR-34 mimics, provided a broad avenue of miR-34-associated therapeutic application on cancer therapy. Recently, Yu et al designed an unprecedented miR-34a prodrug (a tRNA/miR-34a chimera) by removing some unnecessary chemical modifications, which were selectively processed intra-cellularly to obtain full function. It could target various vital genes to depress the malignant biological behavior of OS. Besides, this drug was applied in an orthotopic human OS xenograft mouse with a satisfactory result of suppressing tumor progression and promoting tissue damage, especially, without liver and kidney toxicity [Citation72]. Additionally, Alegre F et al reported the chimera had an inhibition growth effect on all canine OS cell lines, in a concentration- and time-dependent manner [Citation73,Citation74]. More importantly, there was an interesting find that HMPOS cells (p53 mutant) were the least sensitive to tRNA/miR-34a prodrug while D-17 cells (p53 wild-type) were the most sensitive [Citation75], which was consistent with the interaction of mir-34a and p53. Likewise, supplementary exogenous miR-34a such as synthetic miR-34a mimics and viral vectors could significantly impede the migration of OS cells in vitro and/or in vivo of humans [Citation45] and/or mice [Citation74].
Nowadays, some studies revealed there was a synergistic effect on the treatment of a combined regimen of miR-34a and chemotherapy. For example, a study by Zhao Y et al showed bioengineered miR-34a pro-drug combined with doxorubicin had more advantages for preventing OS in an orthotopic xenograft tumor mouse model. Because there was a stronger lethality for cells by promoting apoptosis, necrosis, and G2 cell cycle arrest and targeting miR-34a-related molecules more significantly [Citation76]. Li et al also found elevated level of miR-34a could increase the sensitivity of U2OS to cisplatin by activation of the c-Myc-dependent Bim pathway [Citation77].
Several studies showed that miR-34a-5p was related to multi-chemoresistance of OS through targeting Angiotensin II Receptor Type 1 (AGTR1) gene [Citation22], CD117 gene [Citation20], and Delta-like 1 (DLL1) gene [Citation78], which brought insight in applying the combined usage of the medicine for OS patients. More importantly, strong evidence supported miR-34a-related molecules could be therapeutic targets for OS. For instance, some studies indicated using ribozymes, siRNA targeting, and miRNAs to silence STMN1 might be effective strategies to inhibit cell division [Citation79–81]. Besides, a recent study showed that PAI-1 participates in the p53–miR-34a pathway to mediate the dedifferentiation of OS cells, providing a direction for clinical application of PAI-1-related therapy in OS [Citation82]. Meanwhile, PAI-039, an indole oxoacetic acid PAI-1 inhibitor, could reduce tumor angiogenesis and cell proliferation and promote cell apoptosis [Citation83,Citation84]. Besides, PAI-039 combined with doxorubicin exerted an obvious synergistic effect in blocking the unlimited cell division in OS (). Therefore, this could be speculated as a possible targeted treatment strategy [Citation46].
Conclusions and prospects
Though a lot of researchers are committed to detecting the accurate mechanisms of occurrence and development in OS, we still have not got a thorough comprehension of this malignancy so far. Besides, the tendency toward relapse and chemo-resistant remain high, which is the main cause of terrible outcomes for OS patients [Citation85]. And over the past thirty years, we have not made a breakthrough in the treatment of OS. However, it is worth mentioning that the essential member of miRNAs, miR-34a, exerted anti-tumor properties in cell culture experiments and some animal studies. It was universally down-regulated in OS and played a central role in the network for OS regulation. Besides, emerging evidence suggested that miR-34a was a valuable biomarker for the diagnosis, prognosis, and treatment of OS. Many studies indicated that miR-34a could suppress diverse biological behaviors of OS cells, such as growth, proliferation, migration, through being involved in a complex regulation system as follows: (i) the interaction between miR-34a and p53 provided obvious inhibitory effect in OS; (ii) miR-34a mediated the expression of numerous downstream factors to promote cell cycle arrest and apoptosis directly or indirectly; (iii) the expression levels of miR-34a and its related targets could be interfered by a variety of lncRNAs, highlighting these upstream regulators played an important role in OS.
Moreover, the low expression of miR-34a might indicate a poor outcome for OS patients, and some other related molecules that participated in the pathway could also be potential biomarkers for the diagnosis and prognosis of OS. More importantly, miR-34a could offer notable protection against OS, providing us with a new strategy for treating OS. A previous study showed that restoration of the miR-34a expression may have an inhibitory action in OS. Collectively, miR-34a is a network hub in regulating OS cell proliferation, survival, and resistance to therapy and could be a potential diagnostic biomarker and therapeutic target for OS.
List of abbreviations
AGTR1 | = | Angiotensin II Receptor Type 1 |
AXIN2 | = | axis inhibition protein 2 |
BCL2 | = | B-cell lymphoma-2 |
CCND1 | = | cyclin D1 |
CD44 | = | cluster of differentiation-44 |
CDK4 | = | cyclin-dependent kinases 4 |
CDK6 | = | cyclin-dependent kinases 6 |
CDKs | = | cyclin-dependent kinases |
C-IAP2 | = | cell inhibitor of apoptosis protein 2 |
DGKζ | = | diacylglycerol kinase ζ |
DLL1 | = | Delta-like 1 |
E2F3 | = | E2F transcription factor 3 |
E2F5 | = | E2F5: E2F transcription factor 5 |
EMT | = | epithelial-mesenchymal transition |
HDAC1 | = | histone deacetylase 1 |
KLF4 | = | KruÈppel-like factor 4 |
lncRNAs | = | Long noncoding RNAs |
MALAT1 | = | metastasis-associated lung adenocarcinoma transcript 1 |
MET | = | mesenchymal-epithelial transition |
miRNAs | = | microRNAs |
NEAT1 | = | Nuclear Enriched Abundant Transcript 1 |
OS | = | osteosarcoma |
PAI-1 | = | plasminogen activator inhibitor-1 |
PD-L1 | = | programmed death-ligand 1 |
RUNX2 | = | runt-related transcription factor |
SEMA3E | = | Semaphorin 3E |
SIRT1 | = | silencing information regulator 2 related enzyme 1 |
SNAI1 | = | snail family transcriptional repressor 1 |
SNHG7 | = | lncRNA-SNHG7 |
SOX2 | = | sex determining region Y‑box 2 |
STMN1 | = | Stathmin 1 |
VEGFA | = | vascular endothelial growth factor receptor A |
Authors’ contributions
Zhimin Yang: Writing- Original Draft& Editing. Tang Liu: Review & Editing. Xiaolei Ren: Review & Funding acquisition. Mei Yang: Review & Editing. Chao Tu: Writing- Review, Revision, Supervision, & Funding acquisition. Zhihong Li: Writing- Review & Editing, Supervision. All authors knew the content of this manuscript, and approved its submission.
Disclosure statement
The authors declare that they have no known competing financial interests or personal relationships that could have appeared to influence the work reported in this paper.
Additional information
Funding
References
- Luetke A, Meyers PA, Lewis I, et al. Osteosarcoma treatment - where do we stand? A state of the art review. Cancer Treat Rev. 2014;40(4):523–532.
- Valenti MT, Dalle Carbonare L, Mottes M. Role of microRNAs in progenitor cell commitment and osteogenic differentiation in health and disease (Review). Int J Mol Med. 2018;41(5):2441–2449.
- Zhang C, He J, Qi L, et al. Diagnostic and prognostic significance of dysregulated expression of circular RNAs in osteosarcoma. Expert Rev Mol Diagn. 2021;21(2):235–244.
- Agostini M, Knight RA. miR-34: from bench to bedside. Oncotarget. 2014;5(4):872–881.
- Harrison DJ, Geller DS, Gill JD, et al. Current and future therapeutic approaches for osteosarcoma. Expert Rev Anticancer Ther. 2018;18(1):39–50.
- Friebele JC, Peck J, Pan X, et al. Osteosarcoma: a meta-analysis and review of the literature. Am J Orthop (Belle Mead NJ). 2015;44(12):547–553.
- Bielack S, Jürgens H, Jundt G, et al. Osteosarcoma: the COSS experience. Cancer Treat Res. 2009;152:289–308.
- Bartel DP. MicroRNAs: genomics, biogenesis, mechanism, and function. Cell. 2004;116(2):281–297.
- Farooqi AA, Tabassum S, Ahmad A. MicroRNA-34a: a versatile regulator of myriads of targets in different cancers. Int J Mol Sci. 2017;18(10):2089.
- Zhang W, He L, Liu Z, et al. Multifaceted functions and novel insight into the regulatory role of RNA N(6)-Methyladenosine modification in musculoskeletal disorders. Front Cell Dev Biol. 2020;8:870.
- Ambros V. The functions of animal microRNAs. Nature. 2004;431(7006):350–355.
- Sun L, Wang L, Luan S, et al. miR-429 inhibits osteosarcoma progression by targeting HOXA9 through suppressing Wnt/β-catenin signaling pathway. Oncol Lett. 2020;20(3):2447–2455.
- Cortez MA, Ivan C, Valdecanas D, et al. PDL1 regulation by p53 via miR-34. J Natl Cancer Inst. 2016;108(1). DOI:10.1093/jnci/djv303.
- Rupaimoole R, Slack FJ. MicroRNA therapeutics: towards a new era for the management of cancer and other diseases. Nat Rev Drug Discov. 2017;16(3):203–222.
- Zhang C, Long F, Wan J, et al. MicroRNA-205 acts as a tumor suppressor in osteosarcoma via targeting RUNX2. Oncol Rep. 2016;35(6):3275–3284.
- Naghizadeh S, Mohammadi A, Duijf PHG, et al. The role of miR-34 in cancer drug resistance. J Cell Physiol. 2020;235(10):6424–6440.
- Welponer H, Tsibulak I, Wieser V, et al. The miR-34 family and its clinical significance in ovarian cancer. J Cancer. 2020;11(6):1446–1456.
- Rokavec M, Li H, Jiang L, et al. The p53/miR-34 axis in development and disease. Journal of Molecular Cell Biology. 2014;6(3):214–230.
- Wen J, Zhao YK, Liu Y, et al. MicroRNA-34a inhibits tumor invasion and metastasis in osteosarcoma partly by effecting C-IAP2 and Bcl-2. Tumour Biol. 2017;39(6):1010428317705761.
- Pu Y, Zhao F, Wang H, et al. MiR-34a-5p promotes the multi-drug resistance of osteosarcoma by targeting the CD117 gene. Oncotarget. 2016;7(19):28420–28434.
- Jiang L, Hermeking H. miR-34a and miR-34b/c suppress intestinal tumorigenesis. Cancer Res. 2017;77(10):2746–2758.
- Pu Y, Zhao F, Li Y, et al. The miR-34a-5p promotes the multi-chemoresistance of osteosarcoma via repression of the AGTR1 gene. BMC Cancer. 2017;17(1):45.
- Maroof H, Salajegheh A, Smith RA, et al. Role of microRNA-34 family in cancer with particular reference to cancer angiogenesis. Exp Mol Pathol. 2014;97(2):298–304.
- Liang Y, Ridzon D, Wong L, et al. Characterization of microRNA expression profiles in normal human tissues. BMC Genomics. 2007;8(1):166.
- Roy S, Levi E, Majumdar AP, et al. Expression of miR-34 is lost in colon cancer which can be re-expressed by a novel agent CDF. J Hematol Oncol. 2012;5(1):58.
- Wang B, Li D, Kovalchuk I, et al. miR-34a directly targets tRNA(i)(Met) precursors and affects cellular proliferation, cell cycle, and apoptosis. Proc Natl Acad Sci U S A. 2018;115(28):7392–7397.
- Zhang L, Wang L, Dong D, et al. MiR-34b/c-5p and the neurokinin-1 receptor regulate breast cancer cell proliferation and apoptosis. Cell Prolif. 2019;52(1):e12527.
- Wang L, Yu J, Xu J, et al. The analysis of microRNA-34 family expression in human cancer studies comparing cancer tissues with corresponding pericarcinous tissues. Gene. 2015;554(1):1–8.
- Lewis BP, Burge CB, Bartel DP. Conserved seed pairing, often flanked by adenosines, indicates that thousands of human genes are microRNA targets. Cell. 2005;120(1):15–20.
- Xiong S, Hu M, Li C, et al. Role of miR‑34 in gastric cancer: from bench to bedside (Review). Oncol Rep. 2019;42(5):1635–1646.
- Slabáková E, Culig Z, Remšík J, et al. Alternative mechanisms of miR-34a regulation in cancer. Cell Death Dis. 2017;8(10):e3100.
- Lv T, Song K, Zhang L, et al. miRNA-34a decreases ovarian cancer cell proliferation and chemoresistance by targeting HDAC1. Biochem Cell Biol. 2018;96(5):663–671.
- Welch C, Chen Y, Stallings RL. MicroRNA-34a functions as a potential tumor suppressor by inducing apoptosis in neuroblastoma cells. Oncogene. 2007;26(34):5017–5022.
- Pang RT, Leung CO, Ye TM, et al. MicroRNA-34a suppresses invasion through downregulation of Notch1 and Jagged1 in cervical carcinoma and choriocarcinoma cells. Carcinogenesis. 2010;31(6):1037–1044.
- Chandrasekaran KS, Sathyanarayanan A, Karunagaran D. Downregulation of HMGB1 by miR-34a is sufficient to suppress proliferation, migration and invasion of human cervical and colorectal cancer cells. Tumour Biol. 2016;37(10):13155–13166.
- Zhang L, Liao Y, Tang L. MicroRNA-34 family: a potential tumor suppressor and therapeutic candidate in cancer. J Exp Clin Cancer Res. 2019;38(1):53.
- Yan K, Gao J, Yang T, et al. MicroRNA-34a inhibits the proliferation and metastasis of osteosarcoma cells both in vitro and in vivo. PLoS One. 2012;7(3):e33778.
- Shi X, Kaller M, Rokavec M, et al. Characterization of a p53/miR-34a/CSF1R/STAT3 feedback loop in colorectal cancer. Cell Mol Gastroenterol Hepatol. 2020;10(2):391–418.
- He C, Xiong J, Xu X, et al. Functional elucidation of MiR-34 in osteosarcoma cells and primary tumor samples. Biochem Biophys Res Commun. 2009;388(1):35–40.
- Misso G, Di Martino MT, De Rosa G, et al. Mir-34: a new weapon against cancer? Mol Ther Nucleic Acids. 2014;3(9):e194.
- Wang Y, Jia LS, Yuan W, et al. Low miR-34a and miR-192 are associated with unfavorable prognosis in patients suffering from osteosarcoma. Am J Transl Res. 2015;7(1):111–119.
- Zhang R, Gao X, Zuo J, et al. STMN1 upregulation mediates hepatocellular carcinoma and hepatic stellate cell crosstalk to aggravate cancer by triggering the MET pathway. Cancer Sci. 2020;111(2):406–417.
- Vetter NS, Kolb EA, Mills CC, et al. The microtubule network and cell death are regulated by an miR-34a/Stathmin 1/βIII-Tubulin axis. Mol Cancer Res. 2017;15(7):953–964.
- Lopez CM, Yu PY, Zhang X, et al. MiR-34a regulates the invasive capacity of canine osteosarcoma cell lines. PLoS One. 2018;13(1):e0190086.
- Zou Y, Huang Y, Yang J, et al. miR-34a is downregulated in human osteosarcoma stem-like cells and promotes invasion, tumorigenic ability and self-renewal capacity. Mol Med Rep. 2017;15(4):1631–1637.
- Zhang Y, Pan Y, Xie C, et al. miR-34a exerts as a key regulator in the dedifferentiation of osteosarcoma via PAI-1-Sox2 axis. Cell Death Dis. 2018;9(7):777.
- Tu C, Yang K, Wan L, et al. The crosstalk between lncRNAs and the hippo signalling pathway in cancer progression. Cell Prolif. 2020;53(9):e12887.
- Li P, Huang R, Huang T, et al. Long non-coding RNA NEAT1 promotes proliferation, migration and invasion of human osteosarcoma cells. Int J Med Sci. 2018;15(11):1227–1234.
- Sun J, Wang X, Fu C, et al. Long noncoding RNA FGFR3-AS1 promotes osteosarcoma growth through regulating its natural antisense transcript FGFR3. Mol Biol Rep. 2016;43(5):427–436.
- Ma B, Li M, Zhang L, et al. Upregulation of long non-coding RNA TUG1 correlates with poor prognosis and disease status in osteosarcoma. Tumour Biol. 2016;37(4):4445–4455.
- Zhang Y, Meng W, Cui H. LncRNA CBR3-AS1 predicts unfavorable prognosis and promotes tumorigenesis in osteosarcoma. Biomed Pharmacother. 2018;102:169–174.
- Duan G, Zhang C, Xu C, et al. Knockdown of MALAT1 inhibits osteosarcoma progression via regulating the miR‑34a/cyclin D1 axis. Int J Oncol. 2019;54(1):17–28.
- Deng Y, Zhao F, Zhang Z, et al. Long noncoding RNA SNHG7 promotes the tumor growth and epithelial-to-mesenchymal transition via regulation of miR-34a signals in osteosarcoma. Cancer Biother Radiopharm. 2018;33(9):365–372.
- Ghafouri-Fard S, Taheri M. Nuclear enriched abundant transcript 1 (NEAT1): a long non-coding RNA with diverse functions in tumorigenesis. Biomed Pharmacother. 2019;111:51–59.
- Ji S, Wang S, Zhao X, et al. Long noncoding RNA NEAT1 regulates the development of osteosarcoma through sponging miR-34a-5p to mediate HOXA13 expression as a competitive endogenous RNA. Mol Genet Genomic Med. 2019;7(6):e673.
- Tu C, He J, Qi L, et al. Emerging landscape of circular RNAs as biomarkers and pivotal regulators in osteosarcoma. J Cell Physiol. 2020;235(12):9037–9058.
- Jia D, Niu Y, Li D, et al. lncRNA C2dat1 promotes cell proliferation, migration, and invasion by targeting miR-34a-5p in osteosarcoma cells. Oncol Res. 2018;26(5):753–764.
- Tu C, He J, Chen R, et al. The emerging role of exosomal non-coding RNAs in musculoskeletal diseases. Curr Pharm Des. 2019;25(42):4523–4535.
- Hayes J, Peruzzi PP, Lawler S. MicroRNAs in cancer: biomarkers, functions and therapy. Trends Mol Med. 2014;20(8):460–469.
- Cong C, Wang W, Tian J, et al. Identification of serum miR-124 as a biomarker for diagnosis and prognosis in osteosarcoma. Cancer Biomark. 2018;21(2):449–454.
- Zhao H, Ma B, Wang Y, et al. miR-34a inhibits the metastasis of osteosarcoma cells by repressing the expression of CD44. Oncol Rep. 2013;29(3):1027–1036.
- Sampson VB, Yoo S, Kumar A, et al. MicroRNAs and potential targets in osteosarcoma: review. Front Pediatr. 2015;3:69.
- Zhou G, Shi X, Zhang J, et al. MicroRNAs in osteosarcoma: from biological players to clinical contributors, a review. J Int Med Res. 2013;41(1):1–12.
- Chang L, Shrestha S, LaChaud G, et al. Review of microRNA in osteosarcoma and chondrosarcoma. Med Oncol. 2015;32(6):613.
- Su D, Smith SM, Preti M, et al. Stathmin and tubulin expression and survival of ovarian cancer patients receiving platinum treatment with and without paclitaxel. Cancer. 2009;115(11):2453–2463.
- de Almagro MC, Vucic D. The inhibitor of apoptosis (IAP) proteins are critical regulators of signaling pathways and targets for anti-cancer therapy. Exp Oncol. 2012;34(3):200–211.
- Li Q, Pan X, Wang X, et al. Long noncoding RNA MALAT1 promotes cell proliferation through suppressing miR-205 and promoting SMAD4 expression in osteosarcoma. Oncotarget. 2017;8(63):106648–106660.
- Huo Y, Li Q, Wang X, et al. MALAT1 predicts poor survival in osteosarcoma patients and promotes cell metastasis through associating with EZH2. Oncotarget. 2017;8(29):46993–47006.
- Zhou Y, Tian B, Tang J, et al. SNHG7: a novel vital oncogenic lncRNA in human cancers. Biomed Pharmacother. 2020;124:109921.
- Kansara M, Teng MW, Smyth MJ, et al. Translational biology of osteosarcoma. Nat Rev Cancer. 2014;14(11):722–735.
- Ritter J, Bielack SS. Osteosarcoma. Ann Oncol. 2010;21(Suppl 7):vii320–325.
- Yang Y, Wang S, Li T. Altered long non-coding RNAs predict worse outcome in osteosarcoma patients: evidence from a meta-analysis. Oncotarget. 2017;8(21):35234–35243.
- Alegre F, Ormonde AR, Godinez DR, et al. The interaction between RUNX2 and core binding factor beta as a potential therapeutic target in canine osteosarcoma. Vet Comp Oncol. 2020;18(1):52–63.
- Alegre F, Ormonde AR, Snider KM, et al. A genetically engineered microRNA-34a prodrug demonstrates anti-tumor activity in a canine model of osteosarcoma. PLoS One. 2018;13(12):e0209941.
- Wang WP, Ho PY, Chen QX, et al. Bioengineering novel chimeric microRNA-34a for prodrug cancer therapy: high-Yield expression and purification, and structural and functional characterization. J Pharmacol Exp Ther. 2015;354(2):131–141.
- Zhao Y, Tu MJ, Yu YF, et al. Combination therapy with bioengineered miR-34a prodrug and doxorubicin synergistically suppresses osteosarcoma growth. Biochem Pharmacol. 2015;98(4):602–613.
- Li QC, Xu H, Wang X, et al. miR-34a increases cisplatin sensitivity of osteosarcoma cells in vitro through up-regulation of c-Myc and Bim signal. Cancer Biomark. 2017;21(1):135–144.
- Pu Y, Zhao F, Wang H, et al. MiR-34a-5p promotes multi-chemoresistance of osteosarcoma through down-regulation of the DLL1 gene. Sci Rep. 2017;7(1):44218.
- Li X, Yao R, Yue L, et al. FOXM1 mediates resistance to docetaxel in gastric cancer via up-regulating Stathmin. J Cell Mol Med. 2014;18(5):811–823.
- Hassan MK, Watari H, Mitamura T, et al. P18/Stathmin1 is regulated by miR-31 in ovarian cancer in response to taxane. Oncoscience. 2015;2(3):294–308.
- Barve M, Wang Z, Kumar P, et al. Phase 1 trial of Bi-shRNA STMN1 BIV in refractory cancer. Mol Ther. 2015;23(6):1123–1130.
- Tsuge M, Osaki M, Sasaki R, et al. SK-216, a novel inhibitor of plasminogen activator inhibitor-1, suppresses lung metastasis of human osteosarcoma. Int J Mol Sci. 2018;19(3):736.
- Gomes-Giacoia E, Miyake M, Goodison S, et al. Targeting plasminogen activator inhibitor-1 inhibits angiogenesis and tumor growth in a human cancer xenograft model. Mol Cancer Ther. 2013;12(12):2697–2708.
- Placencio VR, DeClerck YA. Plasminogen activator inhibitor-1 in cancer: rationale and insight for future therapeutic testing. Cancer Res. 2015;75(15):2969–2974.
- Kofman AV, Kim J, Park SY, et al. microRNA-34a promotes DNA damage and mitotic catastrophe. Cell Cycle. 2013;12(22):3500–3511.
- Tafsiri E, Darbouy M, Shadmehr MB, et al. Expression of miRNAs in non-small-cell lung carcinomas and their association with clinicopathological features. Tumour Biol. 2015;36(3):1603–1612.
- Chen J, Hongting L, Shaoping L, et al. MiR-34-a acts as a suppressor in neuroblastoma progression by targeting CD44. J Pak Med Assoc. 2017;67(10):1524–1531.
- Wang Y, Wang L. miR-34a attenuates glioma cells progression and chemoresistance via targeting PD-L1. Biotechnol Lett. 2017;39(10):1485–1492.
- Metheetrairut C, Chotigavanich C, Amornpichetkul K, et al. Expression levels of miR-34-family microRNAs are associated with TP53 mutation status in head and neck squamous cell carcinoma. Eur Arch Otorhinolaryngol. 2019;276(2):521–533.
- Shabani N, Razaviyan J, Paryan M, et al. Evaluation of miRNAs expression in medullary thyroid carcinoma tissue samples: miR-34a and miR-144 as promising overexpressed markers in MTC. Hum Pathol. 2018;79:212–221.
- Kim YH, Lee WK, Lee EB, et al. Combined effect of metastasis-related MicroRNA, miR-34 and miR-124 family, methylation on prognosis of non-small-cell Lung Cancer. Clin Lung Cancer. 2017;18(1):e13–e20.
- Mizuno K, Mataki H, Arai T, et al. The microRNA expression signature of small cell lung cancer: tumor suppressors of miR-27a-5p and miR-34b-3p and their targeted oncogenes. J Hum Genet. 2017;62(7):671–678.
- Tanaka N, Toyooka S, Soh J, et al. Frequent methylation and oncogenic role of microRNA-34b/c in small-cell lung cancer. Lung Cancer. 2012;76(1):32–38.
- Schmid G, Notaro S, Reimer D, et al. Expression and promotor hypermethylation of miR-34a in the various histological subtypes of ovarian cancer. BMC Cancer. 2016;16(1):102.
- Lin X, Chen W, Wei F, et al. Nanoparticle delivery of miR-34a eradicates long-term-cultured breast cancer stem cells via targeting C22ORF28 directly. Theranostics. 2017;7(19):4805–4824.
- Fang LL, Sun BF, Huang LR, et al. Potent inhibition of miR-34b on migration and invasion in metastatic prostate cancer cells by regulating the TGF-β pathway. Int J Mol Sci. 2017;18(12):2762.
- Yamamura S, Saini S, Majid S, et al. MicroRNA-34a modulates c-Myc transcriptional complexes to suppress malignancy in human prostate cancer cells. PLoS One. 2012;7(1):e29722.
- Yu G, Xu K, Xu S, et al. [MicroRNA-34a regulates cell cycle by targeting CD44 in human bladder carcinoma cells]. Nan Fang Yi Ke Da Xue Xue Bao. 2015;35(7):935–940.
- Dang Y, Luo D, Rong M, et al. Underexpression of miR-34a in hepatocellular carcinoma and its contribution towards enhancement of proliferating inhibitory effects of agents targeting c-MET. PLoS One. 2013;8(4):e61054.
- Fritz HK, Gustafsson A, Ljungberg B, et al. The Axl-regulating tumor suppressor miR-34a Is increased in ccRCC but does not correlate with Axl mRNA or Axl protein levels. PLoS One. 2015;10(8):e0135991.
- Lu H, Hao L, Yang H, et al. miRNA-34a suppresses colon carcinoma proliferation and induces cell apoptosis by targeting SYT1. Int J Clin Exp Pathol. 2019;12(8):2887–2897.