ABSTRACT
Gastric cancer is among the most common gastrointestinal malignancies. Recent studies have suggested that bone morphogenetic protein-2 (BMP2) is related to the development and progression of various cancers. Meanwhile, evidence suggests that BMP2 might lead to epigenetic changes in gastric cancer. Thus, we investigated whether BMP2 plays a role in the development of gastric cancer via epigenetic regulation. Cell viability, colony formation, and cell cycle assays were performed to assess the effect of recombinant human BMP2 (rhBMP2) in gastric cancer cells. LDN-193189 and Noggins were used as antagonists of the canonical BMP-SMAD signaling pathway. The protein levels were determined using a western blot analysis. Lentiviral vectors with EZH2 shRNA or EZH2 overexpression were used to mediate the role of EZH2 and the relationship between BMP2 and EZH2 in gastric cancer. We found that rhBMP2 inhibits cell proliferation by arresting the cell cycle in HGC-27 and SNU-216 gastric cancer cells. Neither LDN-193189 nor Noggins, antagonists of the canonical BMP-SMAD signaling pathway, can reverse the effect of rhBMP2 on gastric cancer. Molecularly, rhBMP2 downregulates the expression of EZH2 and H3K27me3, leading to increases in P16 and P21 and decreases in CDK2, CDK4, and CDK6. Altogether, in this study, we demonstrate that BMP2 serves as a tumor suppressor in gastric cancer cells by downregulating EZH2 and H3K27me3 through the non-SMAD BMP pathway, suggesting that BMP2 might be a new therapeutic target for gastric cancer treatment. Abbreviations: BMP: bone morphogenetic protein; TGF-β: transforming growth factor-beta; EZH2: enhancer of zeste homolog 2; H3K27me3: trimethylation histone H3 lysine 27; HRECs: human retinal endothelial cells; PcG: polycomb group; PRC: polycomb repressive complexes.
Introduction
Gastric cancer is among the most common gastrointestinal malignancies, ranking 4th in incidence and 3rd in fatality rate worldwide [Citation1,Citation2]. Unfortunately, most patients with gastric cancer are diagnosed at an advanced stage [Citation3]. The global 5-year survival rate of gastric cancer is less than 30% [Citation4,Citation5]. Due to the complex pathogenesis of gastric cancer and the great heterogeneity among patients, the current treatment methods are still far from ideal. Hence, there is a compelling demand to explore new therapeutic strategies for gastric cancer.
Bone morphogenetic proteins (BMPs), which are generally considered significant members of the transforming growth factor-beta (TGF-β) superfamily, function through the BMP-SMAD pathway in canonical theory and constitute a group of highly conserved functional proteins with similar structures [Citation6,Citation7]. BMPs were first discovered as proteins that induce ectopic bone formation [Citation6,Citation7]. However, BMPs have also been proven to play vital roles in cell differentiation, proliferation, and apoptosis in various cancers [Citation8,Citation9]. Recombinant human BMP2 (rhBMP2) is among the most extensively used bone morphogenetic proteins in clinics to induce cartilage and bone formation [Citation9–11]. BMP2 promotes the development and homeostasis of gastrointestinal organs [Citation12]. Changes in BMP2 expression are related to the development and progression of several solid tumors [Citation13]. For instance, Park et al. reported that BMP-2 seems to play a role in the invasion and metastasis of gastric cancer [Citation14]. Nevertheless, the effects of BMP2 on gastric cancer are still paradoxical [Citation8,Citation15–22]. The underlying mechanisms remain unclear.
Enhancer of zeste homolog 2 (EZH2) is a histone methyltransferase that can trimethylate histone H3 lysine 27 (H3K27) through its C-terminal SET domain to generate H3K27me3 [Citation23]. H3K27me3, which represses the translation of anti-oncogenes, is regarded as a crucial epigenetic regulator in various cancers [Citation24,Citation25]. Several studies have reported that BMP2 alters EZH2 expression [Citation15,Citation26,Citation27]. However, no studies explored whether and how BMP2 mediates this epigenetic regulation in gastric cancer. Our findings suggest that BMP2 serves as a tumor suppressor by downregulating EZH2 in gastric cancer, which may be exploited therapeutically.
Methods and materials
Cell culture and reagents
Human HGC-27 and SNU-216 cells were purchased from the Shanghai Institute of Cell Biology, Chinese Academy of Sciences (Shanghai, China). The cells were cultured in RPMI-1640 (MD Bio, China) medium with 10% fetal bovine serum (HyClone; GE Healthcare Life Sciences, Logan, UT, USA) and 1% penicillin and streptomycin (MD Bio, China) in a 5% CO2 atmosphere at 37°C.
rhBMP2, LDN-193189, and Noggin were purchased from Proteintech (Rosemont, IL, USA), Selleck Chemicals (Houston, TX, USA), and Novoprotein (Shanghai, China), respectively. The concentrations of LDN-193189 and Noggins were determined according to the manufacturer’s instructions.
Cell viability assay
A CCK-8 assay was applied to detect cell viability. Exponentially growing cells were seeded at a density of 2 × 104 cells/well in 96-well plates, incubated with 5% CO2 at 37°C for 12 h and then treated with different factors. In total, 10 µl CCK-8 solution (CK04; Dojindo Laboratories, Japan) were added to each well at 24 h, 48 h, 72 h, and 96 h, followed by incubation for 4 h with 5% CO2 at 37°C. The absorbance at 450 nm was measured by a microplate reader (Multiskan MK3, Thermo Fisher Scientific Inc., MA). The experiment was repeated three times.
Colony formation assay
HGC-27 and SNU-216 cells were seeded in 6-well cell culture plates at 400 cells per well. After two weeks, the cells were subsequently fixed with methyl alcohol for 20 mins and stained with 0.1% crystal violet for 15 mins. The colony formation calculation was performed using a light camera without magnification. The experiment was repeated three times.
Scratch wound healing assay
In total, 6 × 105 HGC-27 and SNU-216 cells were plated in each well of 6-well plates for 24 hours. Then, the monolayer was scraped perpendicularly in a straight line to create a “scratch” using a sterile 200 μl pipette tip. Subsequently, debris was removed, and a fresh medium containing 1% FBS was added. The cells were photographed using a digital camera system (Olympus Corporation, Tokyo, Japan) at 0 h, 12 h, and 24 h. The relative migration rate was calculated based on the cell relative migration area under each treatment. Each experiment was repeated three times.
Cell cycle assay
Following incubation with different factors for 48 h, HGC-27 and SNU-216 cells were collected after trypsinization and washed twice with cold PBS. Cycle analyses were performed with the following protocol. The cells were fixed in 75% cold ethanol at −20°C overnight. Subsequently, the cells were treated with RNase A for 30 min at 37°C and stained with propidium iodide (PI) in the dark at room temperature for 30 min. Next, the cell cycle was assessed using a FACSCalibur flow cytometer (BD Biosciences), and the data were analyzed using FlowJo 10.0 software (FlowJo LLC, Ashland, OR, USA). Each experiment was repeated three times.
Nude mouse xenograft assay
Logarithmic phase HGC-27cells or SNU-216 cells (5 × 106) were coinjected subcutaneously with 100 μl rhBMP2 (20 μg/ml) or PBS as a control into the right flanks of 5-week-old female BALB/c-nude mice (Shanghai SLAC Laboratory Animal Co. Ltd., Shanghai, China). Then, 100 μl rhBMP2 (20 μg/ml) or PBS were subcutaneously injected every 3 days after inoculating the xenograft tumors 7 times in total. Three weeks later, the mice were sacrificed, and the weight, length (a), and width (b) of the tumors were monitored using callipers. The tumor volume (V) was calculated as follows: V = ab2/2. The use of animals in this study was approved by the Institutional Animal Care and Use Committee of the First Affiliated Hospital of Xi’an Jiaotong University.
Lentiviral vectors and transfection
Short hairpin RNA (shRNA) against EZH2 and EZH2 overexpression were delivered with a lentivirus expressing vector. The target shRNA sequence was 5′- CCAACACAAGTCATCCCATTA −3′. All transfections were performed as previously described [Citation28]. The silencing of EZH2 in HGC-27 and SNU-216 cells and the ectopic expression of EZH2 in HGC-27 and SNU-216 cells were validated by a western blot analysis (Supplementary Figure S1).
RNA isolation and real-time PCR
TRIzol reagent (Invitrogen, Carlsbad, CA, USA) was used to isolate the total RNA from cells. A PrimeScript RT Reagent Kit (TaKaRa, Osaka, Japan) was used to synthesize complementary DNA (ctDNA). Real-time PCR was conducted on an IQ5 instrument (Bio-Rad, CA, USA) using SYBR Green fluorescence signal detection assays (TaKaRa, Osaka, Japan) with the EZH2 forward Primer 5′-AATCAGAGTACATGCGACTGAGA-3′ and reverse Primer 5′-GCTGTATCCTTCGCTGTTTCC-3′. The specific mRNA expression level was quantified by the 2 − ΔΔCT method. Each experiment was repeated three times.
Protein extraction and western blot analysis
The details of the antibodies are presented in Supplementary Table S1. All experimental procedures were based on our previous study [Citation28].
Statistical analysis
All data are presented as the mean ± SD (standard deviation) unless otherwise noted. Student’s t-test or one-way analysis of variance with Dunnett’s post hoc test was used to detect significant differences between different groups. All statistical analyses were performed using SPSS 20.0 software (SPSS, Inc., Chicago, IL, USA). P < 0.05 was considered indicative of a statistically significant difference.
Results
rhBMP2 suppresses cell proliferation by arresting the cell cycle in gastric cancer cells
A colony formation assay was performed to investigate the effects of rhBMP2 on gastric cancer cells, including HGC-27 and SNU-216 cells. Compared with the control group, the number of colonies of HGC-27 and SNU-216 cells decreased after the cells were cultured with 100 ng/ml rhBMP2 for 2 weeks (). Moreover, rhBMP2 significantly inhibited the viability of HGC-27 and SNU-216 cells as shown by CCK-8 assays ()). The effect of rhBMP2 on the cell cycle was also examined. After both gastric cancer cell lines were cultured with 100 ng/ml rhBMP2 for 48 h, the rate of G0/G1 phase cells in both cell lines was significantly higher than that in the control group (P < 0.05), with a decline in the rate of G0/G1 phase cells (P < 0.05). The results are presented in ). We also found that BMP2 did not affect the apoptotic rate (Supplementary Figure S2(a–f)) or scratch healing ability (Supplementary Figure S2(g-j)) of HGC-27 and SNU-216 cells. Here, we suggest that rhBMP2 might play an inhibitory role in gastric cancer by arresting the cell cycle.
Figure 1. Effect of rhBMP2 on HGC-27/SNU-216 proliferation, colony formation, and the cell cycle. (a) The colony formation capacity of HGC-27/SNU-216 cells was reduced after culture with rhBMP2 (100 ng/ml) for 2 weeks. (b) Cell viability was measured by a CCK8 assay, which showed a difference after incubation with rhBMP2 (100 ng/ml) for 4 days compared to the control group. (c) Flow cytometric analysis of the cell cycle shows cell cycle arrest after incubation with rhBMP2 (100 ng/ml) for 48 h (*P < 0.05, **P < 0.01).
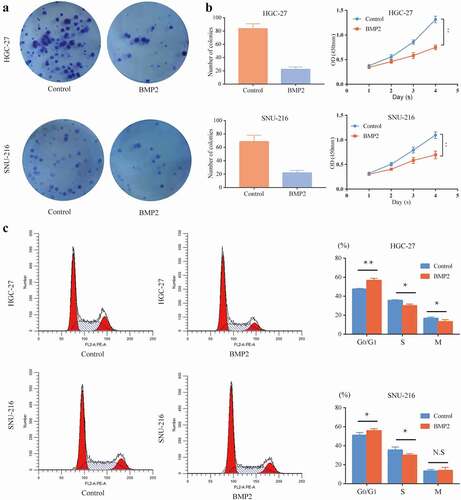
rhBMP2 inhibits gastric cancer independent of SMAD signaling
In our study, LDN-193189 (0.5 nM/ml) and Noggins (1 μg/ml) were used as antagonists of the canonical BMP signaling pathway, and HGC-27 or SNU-216 cells were cultured with rhBMP2 (100 ng/ml) for 2 weeks. However, we found that the inhibitory effect of rhBMP2 on colony formation ability did not vanish after the addition of the antagonists. Conversely, a further reduction in the number of colonies was observed ()). The viability of the two cell lines was found to be significantly inhibited after being cultured with rhBMP2 and LDN-193189 (0.5 nM/ml) or Noggins (1 μg/ml) for 4 days compared with that in the rhBMP2 group ()). Considering the above results, we speculate that rhBMP2 inhibits the proliferation of gastric cancer independent of SMAD signaling.
Figure 2. Effects of the BMP receptor antagonists LDN-193189 and Noggins on HGC-27/SNU-216.(a, c) The colony formation capacity of HGC-27/SNU-216 cells was reduced after culture with rhBMP2 (100 ng/ml) or rhBMP2 with LDN-193189 (0.5 nM/ml)/Noggins (1 μg/ml) for 2 weeks. (b, d) Cell viability of HGC-27/SNU-216 cell lines was measured by a CCK8 assay after culturing with rhBMP2 (100 ng/ml) or rhBMP2 with LDN-193189 (0.5 nM/ml)/Noggins (1 μg/ml) for 4 days, which showed significant differences between the control group and rhBMP2 group, rhBMP2 group and rhBMP2 with LDN-193189/Noggins group (*P < 0.05, **P < 0.01).
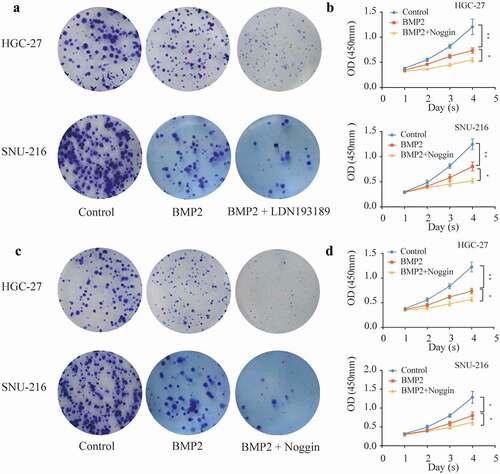
rhBMP2 inhibits the proliferation of gastric cancer by repressing EZH2
A previous gene microarray implied that the mRNA expression of EZH2 is inversely correlated with BMP2 [Citation15]. Herein, we investigated the effect of rhBMP2 on the expression of EZH2, H3K27me3, and BMI1 in HGC-27 and SNU-216 cell lines. We found that the levels of EZH2 and H3K27me3 were significantly decreased ()). Therefore, we speculate that rhBMP2 might inhibit the expression of EZH2 and further change the phenotype of tumor cells by inhibiting H3K27me3. Furthermore, we found that the knockdown of EZH2 repressed the viability and colony formation ability of HGC-27 and SNU-216 cells ()). Meanwhile, the depletion of EZH2 arrested HGC-27 and SNU-216 cells in the G0/G1 phase ()) as determined by the increased levels of P16 and P21 and decreased levels of CDK2, CDK4, and CDK6 ()) in the western blot analysis.
Figure 3. BMP2 regulates the expression of EZH2. (a, b) EZH2, H3K27me3, and BMI1 were measured by western blot analyses of HGC-27 and SNU-216 cells after the treatment with rhBMP-2 for 48 h, and α-Tubulin was used as an internal control. (c) Stable knockdown of EZH2 inhibited the proliferation of HGC-27 and SNU-216 cells as measured by a CCK-8 assay. (d, e) EZH2, p16, p21, CDK2, CDK4, and CDK6 were measured by a western blot analysis of HGC-27 and SNU-216 cells and both the EZH2 knockdown and NC groups. (f) Stable knockdown of EZH2 in HGC-27 and SNU-216 cells inhibited colony formation. (g) Stable knockdown of EZH2 arrested the cell cycle as measured by a flow cytometric analysis (*P < 0.05, **P < 0.01).
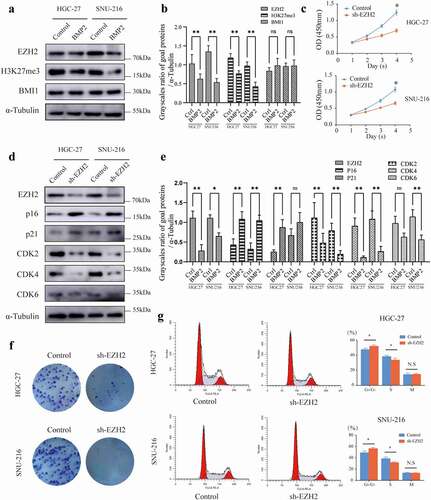
To further determine whether EZH2 is involved in the rhBMP2-mediated inhibition of the cell cycle and proliferation in gastric cancer, we upregulated EZH2 and then investigated its impact on the functions of rhBMP2. The inhibition of proliferation in HGC27 and SNU-216 caused by rhBMP2 could be remarkably attenuated by the upregulation of EZH2 in vitro ()). Meanwhile, the rhBMP2-induced H3K27me3 increase and P16 and P21 reduction could be attenuated by overexpressing EZH2 ()). To explore the function of rhBMP2 in vivo, HGC-27 and SNU-216 cells were coinjected subcutaneously with 100 μl rhBMP2 (20 μg/ml) or PBS as negative control into the right flanks of nude mice. In the following 3 weeks, 100 μl rhBMP2 (20 μg/ml) or PBS were injected into the xenograft tumors every 3 days 7 times in total. Three weeks later, we measured the weight of the xenograft tumors and found that rhBMP2 significantly inhibited tumor growth ()). Therefore, rhBMP2 inhibits the proliferation of gastric cancer by repressing EZH2.
Figure 4. Upregulation of EZH2 reduces the inhibition of HGC-27/SNU-216 by rhBMP2. (a, b) Cell viability was measured by a CCK8 assay after the upregulation of EZH2 in HGC-27 and SNU-216 cells compared with the control group with or without the rhBMP2 treatment. (c) Colony formation capacity of HGC-27/HGC-27-EZH2 and SNU-216/SNU-216-EZH2 cell lines after culture with rhBMP2 (100 ng/ml) or PBS for 2 weeks. (d, e, f, g) Expression of H3K27me3, P16, and P21 was measured by a western blot analysis of EZH2-overexpressing cells compared with control cells with or without the rhBMP2 treatment. α-Tubulin was used as an internal control. (h, i) Images and weights of xenograft tumors 21 days after the inoculation of HGC-27 or SNU-216 cells treated with 100 μl rhBMP2 (20 μg/ml) or PBS.
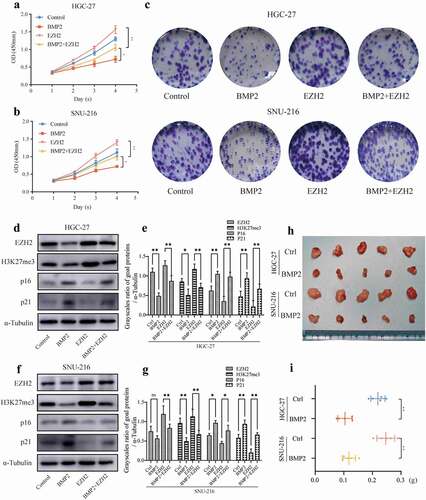
Discussion
rhBMP2 has been used clinically after orthopedic surgery as a stimulator of bone healing and connective tissue repair for nearly two decades [Citation29]; however, in recent years, the safety of rhBMP2 has been widely discussed. Research accomplished by Carragee et al. showed that a high dose of rhBMP-2 in lumbar spinal arthrodesis was associated with a higher risk of cancers [Citation30]. BMP2 has also been reported to influence the biological behavior of tumors in vitro. However, the roles of BMP2 in gastric cancer remain controversial. In several studies, BMP2 appeared to induce the epithelial-mesenchymal transition (EMT) [Citation16,Citation17] and promote invasion/migration [Citation14,Citation19,Citation31]. In contrast, BMP2 had the opposite effect in other studies [Citation15,Citation18,Citation20–22]. To investigate this issue, we added recombinant human BMP2 at a concentration of 100 ng/ml to in vitro cultured gastric cancer cells and 20 μg/ml to in vivo xenograft tumors, which have been reported in several studies to have a significant effect on gastrointestinal cancer cells [Citation18,Citation32,Citation33]. Our study showed that rhBMP2 inhibited the proliferation of gastric cancer cells, including HGC-27 and SNU-216 cells, by arresting the cell cycle in the G0/G1 phase, which is similar to previous studies using the gastric cancer cell lines BGC823, SGC7901, MKN74, and OUMS37 [Citation18,Citation22]. However, BMP2 did not increase their apoptotic rate, and we did not find a BMP2-induced acceleration of migration in gastric cancer cells in contrast to the research by Kang et al [Citation19]. We found that a possible reason is that the sources of BMP2 differed, which had a certain influence on the experimental results. According to our research, we are inclined to agree with the view that BMP2 inhibits gastric cancer. Hence, we mainly focused on the effect of BMP2 on the ability of cancer cells to proliferate by arresting the cell cycle.
As essential members of the TGF-β superfamily, BMPs work through the canonical BMP-SMAD pathway or noncanonical pathway. Canonical BMP-SMAD signaling acts by combining BMPs and type II receptors, which can bind and phosphorylate type I receptors. After they recruit and phosphorylate downstream Smad1/5/8, they form a SMAD complex with SMAD4. Furthermore, the complex translocates into the nucleus and directly regulates the transcription of downstream target genes. In addition to canonical SMAD-mediated signaling activation, BMPs can activate various noncanonical BMP signaling pathways [Citation34]. For instance, BMP2 can activate the p38/NFκB pathway in human retinal endothelial cells (HRECs), which causes the upregulation of VEGF and the disruption of HRECs [Citation35]. The study by Saxena et al. showed that dendritic branch formation in neurons could be more strongly affected by LIMK-mediated noncanonical BMP signaling than canonical BMP signaling [Citation36]. In addition, Ohta et al. reported that BMP2 regulates Hmx3 in vestibular formation through the noncanonical pathway by increasing cAMP-dependent protein kinase A activity and the GLI3R to GLI3A ratio [Citation37]. However, the mechanism of these noncanonical pathways remains unclear. To study whether canonical BMP-SMAD signaling is the cause of the inhibition of gastric cancer, we chose the wild-type SMAD4 cell line HGC-27 and the mutant SMAD4 cell line SNU-216 [Citation38] as experimental subjects. We used LDN-193189 and Noggin to block the canonical BMP-SMAD signaling pathway. Neither inhibitor restored the inhibitory phenotype of BMP2 in the HGC-27 and SNU-216 cell lines, but they intensified the inhibitory effects of BMP2. Therefore, we infer that the inhibitory effect of BMP2 on gastric cancer cells mainly worked through a novel non-SMAD pathway.
The polycomb group (PcG) proteins were first identified as regulatory factors in Drosophila [Citation39]. The PcG protein is mainly composed of two types of polymeric protein complexes, i.e. polycomb repressive complexes 1 (PRC1) and PRC2 [Citation40]. EZH2 is the core catalytic subunit of PRC2 and can trimethylated H3K27 through its C-terminal SET domain to generate H3K27me3 to promote transcription inhibition [Citation23]. In recent years, some studies have suggested that a relationship exists between BMP2 and EZH2. For example, the EZH2 inhibitor GSK126 plays a synergistic role with BMP2 [Citation41]. The use of GSK126 in bone healing may permit a reduction in BMP2 dosing to mitigate its side effects in vivo [Citation41]. Similarly, Dudakovic et al. reported that EZH2 inhibition facilitates the BMP2-mediated induction of osteogenic differentiation of progenitor cells and maturation of committed osteoblasts [Citation26]. Another study demonstrated that during Ras-induced senescence in mouse embryonic fibroblasts, BMP2 was the most activated secreted factor with H3K4me3 gain and H3K27me3 loss [Citation27]. These studies revealed the potential correlation between BMP2 and epigenomic modification of histones. How BMP2 mediates EZH2 in human tumors has never been explored. Our results showed that EZH2 and H3K27me3 were downregulated in gastric cancer cells after the treatment with rhBMP2. According to the above results, we speculate that BMP2 regulates EZH2 through a non-SMAD pathway. However, our conclusion differs from the results reported by Kaneda. et al., who showed that BMP2 suppresses EZH2 through classical BMP-SMAD signaling in mouse embryonic fibroblasts [Citation27]. We speculate that canonical BMP-SMAD signaling and noncanonical BMP signaling might exert effects with different intensities in different animals and tissues, thereby contributing to these contradictory results. Meanwhile, we found that BMP2 does not change the expression of BMI-1, a key component of PRC1 complexes. Hence, we focused on the effect of BMP2 on PRC2-dependent H3K27me3. We assumed that the inhibition of gastric cancer cells by rhBMP-2 seemed to be correlated with EZH2.
A high expression of EZH2 can promote proliferation, migration, and invasion in gastric cancer cells and is correlated with tumor progression and a poorer prognosis [Citation42,Citation43]. Therefore, EZH2 is generally considered an oncogene in gastric cancer. To verify the effect of EZH2 expression on gastric cancer, we found that the EZH2 knockdown could cause cell cycle arrest. Meanwhile, it could decrease the cell viability and colony formation ability. The western blot analysis showed that the knockdown of EZH2 increased the expression of P16 and P21 and reduced the expression of CDK2, CDK4, and CDK6. These results suggest that shEZH2 can inhibit cell proliferation by negatively regulating the cell cycle. To further verify the relationship between BMP2 and EZH2 and the role of EZH2, we overexpressed EZH2, which significantly increased the cell viability and colony formation ability and recovered the inhibitory effect of BMP2 on cells. Furthermore, following the decrease in the EZH2 levels induced by BMP2, P16, P21, and H3K27me3 were recovered by the overexpression of EZH2. In the xenograft tumors, rhBMP2 also showed significant inhibition of tumor growth.
The above results indicate that BMP2 inhibited cell proliferation by arresting the cell cycle in gastric cancer cells. The underlying molecular mechanisms were that BMP2 repressed the expression of EZH2 via a novel non-SMAD BMP pathway. Our findings might provide a new idea for the therapy of gastric cancer; however, more evidence is needed in the future.
Consent for publication
All authors consent to publication.
Author contributions
ZS X, ZL C, and LL Y performed the experiments, acquired the data, and drafted the article. All authors revised the article critically for important intellectual content. XP L, JH Y, and ZS X analysed and interpreted the data. ZS X and JH Y substantially contributed to the conception and design. All authors read and approved the final manuscript and agree to be accountable for all aspects of the research in ensuring that the accuracy or integrity of any part of the work is appropriately investigated and resolved.
Supplemental Material
Download Zip (4.6 MB)Acknowledgments
We are grateful for the help from Dr. Shan Li. We thank the Center for Translational Medicine of First Affiliated Hospital of Xi’an Jiaotong University for the support.
Disclosure statement
No potential conflict of interest was reported by the author(s).
Data availability statement
The datasets generated and/or analysed during the current study are not publicly available but are available from the corresponding author upon reasonable request.
Supplementary material
Supplemental data for this article can be accessed online at https://doi.org/10.1080/15384101.2022.2092819
Additional information
Funding
References
- Siegel RL, Miller KD, Jemal A. Cancer statistics, 2019. CA Cancer J Clin. 2019 Jan;69(1):7–34.
- Rawla P, Barsouk A. Epidemiology of gastric cancer: global trends, risk factors and prevention. Prz Gastroenterol. 2019;14(1):26–38.
- Choi YY, Noh SH, Cheong JH. Evolution of gastric cancer treatment: from the golden age of surgery to an era of precision medicine. Yonsei Med J. 2015 Sep;56(5):1177–1185.
- Thrift AP, El-Serag HB. Burden of gastric cancer. Clin Gastroenterol Hepatol. 2020 Mar;18(3):534–542.
- Van Cutsem E, Sagaert X, Topal B, et al. Gastric cancer. Lancet. 2016 Nov 26;388(10060):2654–2664.
- Urist MR, Mikulski A, Lietze A. Solubilized and insolubilized bone morphogenetic protein. Proc Natl Acad Sci USA. 1979 Apr;76(4):1828–1832.
- Wozney JM, Rosen V, Celeste AJ, et al. Novel regulators of bone formation: molecular clones and activities. Science. 1988 Dec 16;242(4885):1528–1534.
- Skovrlj B, Koehler SM, Anderson PA, et al. Association between BMP-2 and carcinogenicity. Spine (Phila Pa 1976). 2015 Dec;40(23):1862–1871.
- Herberg S, McDermott AM, Dang PN, et al. Combinatorial morphogenetic and mechanical cues to mimic bone development for defect repair. Sci Adv. 2019 Aug;5(8):eaax2476.
- Thorey F, Menzel H, Lorenz C, et al. Enhancement of endoprosthesis anchoring using BMP-2. Technol Health Care. 2010;18(3):217–229.
- Ruan S, Deng J, Yan L, et al. Evaluation of the effects of the combination of BMP-2-modified BMSCs and PRP on cartilage defects. Exp Ther Med. 2018 Dec;16(6):4569–4577.
- Fukuda K, Sakamoto N, Narita T, et al. Application of efficient and specific gene transfer systems and organ culture techniques for the elucidation of mechanisms of epithelial-mesenchymal interaction in the developing gut. Dev Growth Differ. 2000 Jun;42(3):207–211.
- Tian H, Zhao J, Brochmann EJ, et al. Bone morphogenetic protein-2 and tumor growth: diverse effects and possibilities for therapy. Cytokine Growth Factor Rev. 2017;34:73–91.
- Park Y, Kim JW, Kim DS, et al. The bone morphogenesis protein-2 (BMP-2) is associated with progression to metastatic disease in gastric cancer. Cancer Res Treat. 2008 Sep;40(3):127–132.
- Lee KB, Jin H, Ye S, et al. Recombinant human bone morphogenetic protein-2 inhibits gastric cancer cell proliferation by inactivating Wnt signaling pathway via c-Myc with Aurora kinases. Oncotarget. 2016 Nov 8;7(45):73473–73485.
- Yang Y, Yang C, Zhang J. C23 protein meditates bone morphogenetic protein-2-mediated EMT via up-regulation of Erk1/2 and Akt in gastric cancer. Med Oncol. 2015 Mar;32(3):76.
- Liao A, Wang W, Sun D, et al. Bone morphogenetic protein 2 mediates epithelial-mesenchymal transition via AKT and ERK signaling pathways in gastric cancer. Tumour Biol. 2015 Apr;36(4):2773–2778.
- Zhang J, Ge Y, Sun L, et al. Effect of bone morphogenetic protein-2 on proliferation and apoptosis of gastric cancer cells. Int J Med Sci. 2012;9(2):184–192.
- Kang MH, Kim JS, Seo JE, et al. BMP2 accelerates the motility and invasiveness of gastric cancer cells via activation of the phosphatidylinositol 3-kinase (PI3K)/Akt pathway. Exp Cell Res. 2010 Jan 1;316(1):24–37.
- Yang YJ, Luo S, Wang LS. Effects of microRNA-378 on epithelial-mesenchymal transition, migration, invasion and prognosis in gastric carcinoma by targeting BMP2. Eur Rev Med Pharmacol Sci. 2019 Jun;23(12):5176–5186.
- Shirai YT, Ehata S, Yashiro M, et al. Bone morphogenetic protein-2 and −4 play tumor suppressive roles in human diffuse-type gastric carcinoma. Am J Pathol. 2011 Dec;179(6):2920–2930.
- Wen XZ, Miyake S, Akiyama Y, et al. BMP-2 modulates the proliferation and differentiation of normal and cancerous gastric cells. Biochem Biophys Res Commun. 2004 Mar 26;316(1):100–106.
- Gan L, Yang Y, Li Q, et al. Epigenetic regulation of cancer progression by EZH2: from biological insights to therapeutic potential. Biomark Res. 2018;6(1):10.
- Duan R, Du W, Guo W. EZH2: a novel target for cancer treatment. J Hematol Oncol. 2020 Jul 28;13(1):104.
- Yin X, Yang S, Zhang M, et al. The role and prospect of JMJD3 in stem cells and cancer. Biomed Pharmacother. 2019 Oct;118:109384.
- Dudakovic A, Samsonraj RM, Paradise CR, et al. Inhibition of the epigenetic suppressor EZH2 primes osteogenic differentiation mediated by BMP2. J Biol Chem. 2020 Jun 5;295(23):7877–7893.
- Kaneda A, Fujita T, Anai M, et al. Activation of Bmp2-Smad1 signal and its regulation by coordinated alteration of H3K27 trimethylation in Ras-induced senescence. PLoS Genet. 2011 Nov;7(11):e1002359.
- Yu JH, Zheng JB, Qi J, et al. Bile acids promote gastric intestinal metaplasia by upregulating CDX2 and MUC2 expression via the FXR/NF-κB signalling pathway. Int J Oncol. 2019 Mar;54(3):879–892.
- Kendal JK, Singla A, Affan A, et al. Is use of BMP-2 associated with tumor growth and osteoblastic differentiation in murine models of osteosarcoma? Clin Orthop Relat Res. 2020 Dec;478(12):2921–2933.
- Carragee EJ, Chu G, Rohatgi R, et al. Cancer risk after use of recombinant bone morphogenetic protein-2 for spinal arthrodesis. J Bone Joint Surg Am. 2013 Sep 4;95(17):1537–1545.
- Kang MH, Oh SC, Lee HJ, et al. Metastatic function of BMP-2 in gastric cancer cells: the role of PI3K/AKT, MAPK, the NF-κB pathway, and MMP-9 expression. Exp Cell Res. 2011 Jul 15;317(12):1746–1762.
- Wen XZ, Akiyama Y, Baylin SB, et al. Frequent epigenetic silencing of the bone morphogenetic protein 2 gene through methylation in gastric carcinomas. Oncogene. 2006 Apr 27;25(18):2666–2673.
- Hardwick JC, Van Den Brink GR, Bleuming SA, et al. Bone morphogenetic protein 2 is expressed by, and acts upon, mature epithelial cells in the colon. Gastroenterology. 2004 Jan;126(1):111–121.
- Zhang Y, Que J. BMP signaling in development, stem cells, and diseases of the gastrointestinal tract. Annu Rev Physiol. 2020 Feb 10;82(1):251–273.
- Al-Shabrawey M, Hussein K, Wang F, et al. Bone morphogenetic protein-2 induces non-canonical inflammatory and oxidative pathways in human retinal endothelial cells. Front Immunol. 2020;11:568795.
- Saxena M, Agnihotri N, Sen J. Perturbation of canonical and non-canonical BMP signaling affects migration, polarity and dendritogenesis of mouse cortical neurons. Development. 2018 Jan 9;1451. 10.1242/dev.147157
- Ohta S, Wang B, Mansour SL, et al. BMP regulates regional gene expression in the dorsal otocyst through canonical and non-canonical intracellular pathways. Development. 2016 Jun 15;143(12):2228–2237.
- Ju HR, Jung U, Sonn CH, et al. Aberrant signaling of TGF-beta1 by the mutant Smad4 in gastric cancer cells. Cancer Lett. 2003 Jul 10;196(2):197–206.
- Morey L, Helin K. Polycomb group protein-mediated repression of transcription. Trends Biochem Sci. 2010 Jun;35(6):323–332.
- Sauvageau M, Sauvageau G. Polycomb group proteins: multi-faceted regulators of somatic stem cells and cancer. Cell Stem Cell. 2010 Sep 3;7(3):299–313.
- Lui H, Samsonraj RM, Vaquette C, et al. Combination of BMP2 and EZH2 inhibition to stimulate osteogenesis in a 3D bone reconstruction model. Tissue Eng Part A. 2021 Jan 12;27(15–16):1084–1098.
- Gan L, Xu M, Hua R, et al. The polycomb group protein EZH2 induces epithelial-mesenchymal transition and pluripotent phenotype of gastric cancer cells by binding to PTEN promoter. J Hematol Oncol. 2018 Jan 15;11(1):9.
- Lu Y, Hou K, Li M, et al. Exosome-delivered LncHEIH promotes gastric cancer progression by upregulating EZH2 and stimulating methylation of the GSDME promoter. Front Cell Dev Biol. 2020;8:571297.