ABSTRACT
Emetine is one of the most highly potent anti-SARS-CoV-2 agents ever identified. In addition to having strong anti-SARS-CoV-2 activities, emetine has other valuable therapeutic effects such as strong anti-inflammatory and anti-arterial pulmonary hypertension (APH) properties, which are suitable for the treatment of COVID-19. Its proper concomitant therapeutic effect has led researchers to test this compound in clinical trials to combat COVID-19. However, due to the risks of cardiac complications, very low doses of emetine have been used in different studies, which may not have significant therapeutic effects. The p38 MAPK signaling pathway is strongly highlighted as an important operator in cardiac cellular damages such as disruption of cardiac fibroblast function and myopathy/cardiomyopathy. Inhibition of this pathway by appropriate inhibitors has also been considered by scientists as a promising strategy for the treatment of fatal host-related hyper-inflammatory immune responses following SARS-CoV-2 infection. Although remarkable stimulatory effects of emetine on activation of the p38 MAPK pathway have been reported in recent studies and strong evidence suggests that this pathway plays an effective role in the emetine’s toxicities, it has not been discussed yet that emetine induced cellular cardiac complications may be due to the activation of this critical pathway. Considering these points could lead to the finding of strategies for applying the valuable potential of emetine in the treatment of COVID-19 at low risks.
1. Introduction
Emetine is one of the most potent anti-SARS-CoV-2 compounds identified to date. The high potency of this compound in inhibiting SARS-CoV-2 replication has been reported in numerous recent studies including several high-throughput screening efforts (HTS) [Citation1–6]. Some of these studies have shown that emetine is tens of times more potent in fighting SARS-CoV-2 than commonly clinically used antiviral drugs applied to treat COVID-19, such as remdesivir [Citation7,Citation8]. In addition to SARS-CoV-2, very strong effects of this compound in inhibiting replication of other famous coronaviruses such as HCoV-OC43, HCoV-NL63, SARS-CoV, and MERS-CoV have been reported [Citation9–12]. The broad-spectrum antiviral effects of this compound against a wide range of human and animal viruses with EC50 values in the nanomolar range have also been reported in previous studies (). [Citation13–19]
Figure 1. Structure of emetine as broad spectrum antiviral agent against coronaviruses and various types of human/animal viruses including Dengue viruses, Cytomegaloviruses, Immunodeficiency virus type 1 (HIV-1), Hantaan orthohantavirus (HTNV), Andes orthohantavirus (ANDV), Ebola (EBOV), Lassa, Rabies (RABV), Herpes simplex viruses (HSVs), Echovirus-1, Human metapneumovirus (HMPV), Rift Valley fever virus (RVFV), Buffalopoxvirus (BPXV), Bovine herpesvirus-1 (BHV-1), Peste des petits ruminants virus (PPRV), and Newcastle disease virus (NDV).
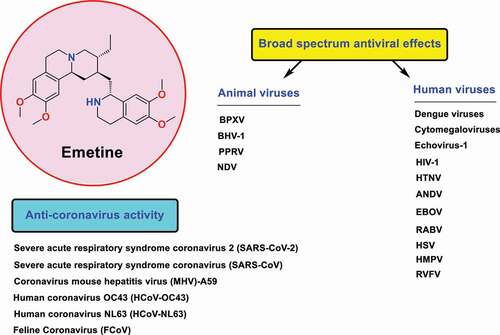
In addition to having direct anti-SARS-CoV-2 effects exerted by the inhibition of RNA-dependent RNA polymerase (RdRp) enzyme and nucleocapsid (N) protein [Citation4], this compound also has the potential to disrupt virus replication by regulating some host-based targets. Recent studies suggested that the potential of emetine to fight SARS-CoV-2 is due to its ability to suppress the host translation machinery [Citation5], as it has long been known as a protein synthesis inhibitor through the 40S ribosomal protein S14 inhibition mechanism. Another recent study also suggests that the inhibitory effect of emetine against SARS-CoV-2 replication is mediated by inhibiting the interaction of viral mRNA with eukaryotic translation initiation factor 4E (eIF4E) [Citation3].
As stated in recent studies, proper inhibition of hyper-inflammatory immune response following SARS-CoV-2 infection is a key clinical step in the treatment of COVID-19 patients [Citation20–22]. Emetine seems to have valuable capabilities in this context. In addition to having excellent anti-SARS-CoV-2 activities, this compound can exert strong anti-inflammatory effects through suppression of the NF-κB signaling pathway and also limiting the expression of pro-inflammatory cytokines such as TNFα, IL-1β, and IL-6 [Citation23,Citation24]. Interestingly, this compound has a bright history of treating patients in the horrible Spanish flu pandemic of 1918. Today, some researchers have hypothesized that the anti-inflammatory effect of emetine may have played a major role in the treatment of the Spanish flu patients [Citation25], although recent studies have also indicated strong anti-influenza effect for this compound [Citation18].
Another important therapeutic effect of emetine is its potential ability to treat pulmonary arterial hypertension (PAH) because COVID-19 patients with PAH are among the most vulnerable patients [Citation26]. The release of pro-inflammatory cytokines in the pulmonary arteries of patients with PAH leads to the production of reactive oxygen species (ROS) and ultimately induces oxidative stress conditions [Citation27]. This unfortunate condition damages the respiratory muscles, ultimately tends to hypoxemia and decreased cardiac output through reducing oxygen supply [Citation28,Citation29]. A recently comprehensive study in rats, reported that emetine has the ability to fight all of these complications, which led to the introduction of emetine as a promoting candidate for the treatment of PAH [Citation30].
This interesting therapeutic profile has led to the use of emetine for the treatment of COVID-19 in some clinical trials (Registration number: ChiCTR2000030022). However, since the use of this drug is associated with ECG abnormalities and myocardial cellular damages [Citation31–34], its use is limited over a long period of time due to the risk of accumulation [Citation35]. Therefore, accurate knowledge of its cardiac side effect mechanisms and finding a solution to overcome this restriction could be a sensible strategy for further utilizing the therapeutic capacity of this compound in the treatment of COVID-19.
2. Cardiac toxicity
Emetine has been used as a drug of choice for the treatment of acute intestinal and extraintestinal amoebiasis for five decades (From the discovery of its excellent anti-amoebiasis effect by Vedder in 1912 until the introduction of metronidazole in 1965). This long period of use in clinics has led to accurate identification of its side effects and toxicities. The dose of emetine hydrochloride used to treat patients with amoebiasis is 1 mg/kg/day for 10 days or less. Various studies have indicated that emetine causes a wide range of cardiac complications. The common side effects identified in patients taking emetine are ECG abnormalities, tachycardia, ventricular fibrillation, and hypotension, which sometimes persist only for a short time after treatment and then the patient recovers without any changes in cardiovascular function [Citation36–39]. In some cases, much more dangerous cardiac complications from cellular damage (such as cardiomyopathy) have also been reported with the use of emetine. Kini and coworkers evaluated the effect of emetine on serum transaminases in patients treated with doses of 60 mg/day, IM for 6–9 days. They reported that ECG abnormalities are a predominant cardiac complication, manifesting in the form of T-wave changes. Biochemical assessments in this study showed an increase in SGOT level coincided with ECG changes. Since no changes in other biochemical parameters (such as SGPT, serum bilirubin, thymol turbidity, and serum alkaline phosphatase) occurred, the researchers concluded that an increase in SGOT could indicate either mitochondrial myopathy/cardiomyopathy, not liver damage [Citation40]. This complication is the most serious concerns for the consumption of this drug (especially in the elderly patients and those with underlying cardiovascular diseases) [Citation31,Citation34,Citation41,Citation42]. Some studies suggested that it could be mediated by its effect on protein synthesis [Citation43]. Decreased protein synthesis of the myocardium can certainly provide the basis for cellular damage. Given these concerns, some studies warn of long-term or frequently repeated courses of this drug, due to its toxic effects on the heart especially fatal myocarditis [Citation44].
Creatine phosphokinase (CPK) is another important biochemical parameter that has been reported its serum levels increase during and after emetine treatment [Citation45,Citation46]. Some studies suggest that elevated CPK induced by emetine may be associated with its effects on skeletal muscle weakness (myopathy) and irritation [Citation47]. In support of these reports, other studies indicate that a significant increase in CPK levels is usually considered important clinical evidence for the presence of inflammatory disorders and myopathy/cardiomyopathy [Citation48,Citation49].
There are many valid studies to suggest that inappropriate activation of the p38 MAPK signaling pathway is associated with cardiac problems. Because emetine is a p38 MAPK activator, analyzing the possible association between its cardiac side effects and activating this pathway could be useful for achieving a way to apply the valuable therapeutic potential of this drug in the treatment of COVID-19 at low risks.
3. Cardiac complications related to p38 MAPK activation
The p38 mitogen-activated protein kinases (p38 MAPKs) are stress-activated Ser/Thr kinases that are present in four subtypes α, β, γ, and δ [Citation50]. These kinases activated in the heart under various pathological stressors such as oxidative stress, viral infections, and cytokine release, and also have an important role in inflammatory responses and cell death [Citation51]. The function of p38 MAPK in the cardiovascular system includes the regulation of cardiomyocyte proliferation, apoptotic roles, and cardiac hypertrophy regulation [Citation52]. Previous studies have shown that chronic activation of p38 MAPK induces a hypertrophic response in cardiomyocytes, while inhibition of this pathway using pharmacological inhibitors can attenuate cardiomyocyte growth in response to hypertrophic triggers [Citation53–55]. Since activation of p38 MAPK arrests the cardiomyocyte cell cycle, so regulation of this pathway is thought to be a useful strategy for cardiac regeneration in response to cardiac cell damage [Citation56,Citation57].
The correlation between p38 and the incidence of apoptosis has also been characterized in several studies. The p38α and p38β subtypes have been shown to play opposite roles in apoptosis [Citation58]. While p38α MAPK has a pro-apoptotic role through p53 activation, p38β MAPK has an inhibitory role against apoptosis by inhibiting ROS formation [Citation59,Citation60]. B-cell lymphoma-extra large (Bcl-xL) is a transmembrane protein in the mitochondria that acts as an apoptosis suppressor, so overexpression of this protein inhibits apoptosis [Citation61,Citation62]. Importantly, the regulation of Bcl-xL plays an important role in both emetine-induced apoptosis and p38 MAPK activation. In a study by Boon-Unge et al., it was found that emetine downregulates the Bcl-xL mRNA levels in a dose- and time-dependent manner [Citation63]. The effect of emetine on the downregulation of Bcl-xL has been reported in some other independent studies [Citation64]. Interestingly, some in vitro studies have shown that overexpression of p38α MAPK (as a pro-apoptotic factor) reduces the expression of the Bcl-xL protein in cultured cardiomyocytes [Citation65,Citation66]. The combination of these findings strongly supports the hypothesis that emetine-induced myocardial damage could be mediated by activation of p38α MAPK and inhibitory effect on the expression of apoptosis suppressor protein Bcl-xL.
4. Management of emetine’s cardiac complications by p38 MAPK inhibition
Some recent studies reported that emetine has remarkable effect on the activation of the p38 MAPK, a pathway that is closely related to the cardiac problems and induction of apoptosis. A study by Kim et al. investigated the effect of emetine on the activation of MAPK signaling pathways (ERK, JNK, and p38 MAPK) using p38 siRNA. Results showed that emetine regulates cross-talk between p38 and ERK signaling pathway in A549 and H1299 human non-small-cell lung cancer cells (NSCLC). The mechanistic assessments showed that emetine (tested doses: 0, 0.3, 1, and 3 µM) effectively activates p38 MAPK by the increase in the level of phosphorylated-p38, and inhibits ERK, but has no significant effect on JNK [Citation67]. The results of this study were consistent with previous reports that showed inverse effect of ERK and p38 activation/inhibition on each other [Citation68,Citation69]. A similar study about the stimulatory effect of emetine on p38 MAPK and its inhibitory effect on ERK has been also reported by Son et al [Citation70].
This biological activity of emetine (p38 MAPK activation) seems to be a fundamental description in the justifying its cardiac side effects. Given the excellent multi-therapeutic effects of emetine to combat COVID-19, the question arises as to “whether p38 MAPK inhibitors can be used to protect against its adverse effects on the heart?” Although this seems logical in theory, the exact answer should be sought in experimental in vivo and in vitro studies. There is some promising evidence for a positive answer to this question. Interestingly, the effect of various standard p38 MAPK inhibitors (such as SB-203580, SB-731445, SB-202190, BIRB-796, and SB-731445) in attenuating cardiac complications and suppressing inflammatory cytokines has been confirmed in several in vitro and in vivo studies [Citation71–77]. In addition, because the destructive effects of p38 MAPK pathway activation are mediated by downstream MAPK-activated protein kinase 2 (MK2), in vivo experiments also showed that administration of MK2 inhibitors such as MMI-0100 have significant effects in inhibiting cardiac complications such as ischemia-induced systolic dysfunction and fibrosis induced by the activation of this pathway [Citation78–80].
Losmapimod and dilmapimod are well-known p38 MAPK inhibitors whose satisfactory safety profiles have been reported in clinical studies [Citation81–83]. Losmapimod is currently undergoing clinical trials to increase respiratory support and prevent further clinical deterioration and mortality of COVID-19 patients (ClinicalTrials.gov Identifier: NCT04511819). These drugs or other proper p38 MAPK pathway inhibitors such as MMI-0100 appear to be appropriate candidates for their concomitant administration with emetine to utilize the capabilities of this highly potent anti-SARS-CoV-2 drug at higher doses ().
Figure 2. Activation of p38 MAPK signaling pathway by emetine leads to the pathogenic conditions such as disruption of cardiac fibroblast function, induction of pro-inflammation, vasoconstriction, pro-atrophy, and pro-fibrosis situations that eventually leads to cellular heart damages such as apoptosis and myopathy/cardiomyopathy. Theoretically, proper p38 MAPK inhibitors such as Losmapimod and Dilmapimod could protect emetine-induced cardiac complications through inhibition of the p38 MAPK pathway.
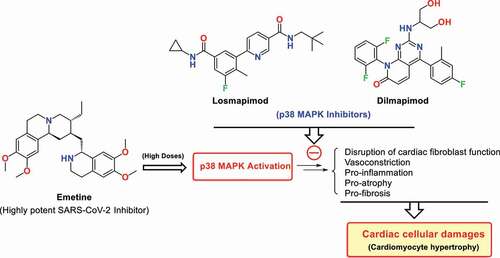
Overall, emetine’s cardiomyopathy (which may be triggered by activation of the p38 MAPK pathway) severely limits its use at higher doses in the treatment of COVID-19, especially in the elderly or for durations greater than 10 days. We here analyze the benefits of p38 MAPK inhibitors and recommend them for the treatment of emetine-induced cellular cardiac complications. A better understanding of the rationale for this clinical strategy and prescribing higher doses of emetine in combination with p38 MAPK inhibitor drugs for the more effective and less risky treatment of COVID-19 disease requires an understanding of the association between emetine-induced cellular complications with p38 MAPK activation and the incidence of apoptosis (discussed in Section 3).
5. Conclusion
Emetine has recently been tested in preclinical and clinical trials for the treatment of COVID-19 but has been used in low doses due to its cellular cardiac complications such as cardiomyopathy [Citation1,Citation35]. On the other hand, inhibiting the activation of the p38 MAPK pathway has recently been considered for the treatment of cellular damages and hyper-inflammatory complications of COVID-19. Accordingly, some clinical trials have also used p38 MAPK inhibitors, but it is important to note that these agents have no antiviral effect and can only reduce the inflammatory complications of the COVID-19 infection. Considering the strengths and weaknesses of emetine and p38 MAPK inhibitors in these independent clinical trials for fighting against COVID-19, the simultaneous use of these agents seems to be much more helpful. When co-administered, these agents can suppress the virus replication (by emetine), reduce the hyper-inflammatory situations and reduce the cardiac complications of emetine (by p38 MAPK inhibitors). This method, which is a targeted mixing of previous clinical trials, could be a rational strategy to use the valuable potential of emetine in the treatment of COVID-19 by reducing its cardiovascular risks.
Disclosure statement
No potential conflict of interest was reported by the author(s).
Data availability statement
Data sharing is not applicable to this article as no new data were created or analyzed in this study.
Additional information
Funding
References
- Wang A, Sun Y, Liu Q, et al. Low dose of emetine as potential anti-SARS-CoV-2 virus therapy: preclinical in vitro inhibition and in vivo pharmacokinetic evidences. Mol Biomed. 2020;1:1–9.
- Ellinger B, Bojkova D, Zaliani A, et al. A SARS-CoV-2 cytopathicity dataset generated by high-content screening of a large drug repurposing collection. Sci Data. 2021;8:1–10.
- Kumar R, Afsar M, Khandelwal N, et al. Emetine suppresses SARS-CoV-2 replication by inhibiting interaction of viral mRNA with eIF4E. Antiviral Res. 2021;189:105056.
- Ren PX, Shang WJ, and Yin WC, et al. A multi-targeting drug design strategy for identifying potent anti-SARS-CoV-2 inhibitors. Acta Pharmacol Sin. 2022 Feb;43(2):483–493.
- Bojkova D, Klann K, Koch B, et al. Proteomics of SARS-CoV-2-infected host cells reveals therapy targets. Nature. 2020;583:469–472.
- Valipour M. Different aspects of emetine’s capabilities as a highly potent SARS-CoV-2 inhibitor against COVID-19. ACS Pharmacol Transl Sci. 2022;5:387–399.
- Choy K-T, Wong AY-L, Kaewpreedee P, et al. Remdesivir, lopinavir, emetine, and homoharringtonine inhibit SARS-CoV-2 replication in vitro. Antiviral Res. 2020;178:104786.
- Luo Y, Yu F, Zhou M, et al. Engineering a reliable and convenient SARS-CoV-2 replicon system for analysis of viral RNA synthesis and screening of antiviral inhibitors. Mbio. 2021;12:e02754–20.
- Dyall J, Coleman CM, Venkataraman T, et al. Repurposing of clinically developed drugs for treatment of Middle East respiratory syndrome coronavirus infection. Antimicrob Agents Chemother. 2014;58:4885–4893.
- Shen L, Niu J, Wang C, et al. High-throughput screening and identification of potent broad-spectrum inhibitors of coronaviruses. J Virol. 2019;93:e00023–19.
- Ko M, Chang SY, and Byun SY, et al. Screening of FDA-approved drugs using a MERS-CoV clinical isolate from South Korea identifies potential therapeutic options for COVID-19. BioRxiv. 2021;13(4):651.
- Yang C-W, Peng -T-T, Hsu H-Y, et al. Repurposing old drugs as antiviral agents for coronaviruses. Biomed J. 2020;43:368–374.
- Yin Low J, Chen K, Wu K, et al. Antiviral activity of emetine dihydrochloride against dengue virus infection. J Antivir Antiretrovir. 2009;1:062.
- Mukhopadhyay R, Roy S, Venkatadri R, et al. Efficacy and mechanism of action of low dose emetine against human cytomegalovirus. PLoS Pathog. 2016;12:e1005717.
- Valadão ALC, Abreu CM, Dias JZ, et al. Natural plant alkaloid (emetine) inhibits HIV-1 replication by interfering with reverse transcriptase activity. Molecules. 2015;20:11474–11489.
- Abate C, Niso M, and Abatematteo FS, et al. PB28, the sigma-1 and sigma-2 receptors modulator with potent anti–SARS-CoV-2 activity: a review about its pharmacological properties and structure affinity relationships. Front Pharmacol. 2020;11:589810.
- MacGibeny MA, Koyuncu OO, Wirblich C, et al. Retrograde axonal transport of rabies virus is unaffected by interferon treatment but blocked by emetine locally in axons. PLoS Pathog. 2018;14:e1007188.
- Andersen PI, Krpina K, Ianevski A, et al. Novel antiviral activities of obatoclax, emetine, niclosamide, brequinar, and homoharringtonine. Viruses. 2019;11:964.
- Khandelwal N, Chander Y, Rawat KD, et al. Emetine inhibits replication of RNA and DNA viruses without generating drug-resistant virus variants. Antiviral Res. 2017;144:196–204.
- Tay MZ, Poh CM, Rénia L, et al. The trinity of COVID-19: immunity, inflammation and intervention. Nat Rev Immunol. 2020;20:363–374.
- Lowery SA, Sariol A, Perlman S. Innate immune and inflammatory responses to SARS-CoV-2: implications for COVID-19. Cell Host & Microbe; 2021.
- Valipour M, Zarghi A, Ebrahimzadeh MA, et al. Therapeutic potential of chelerythrine as a multi-purpose adjuvant for the treatment of COVID-19. Cell Cycle. 2021;20:2321–2336.
- Miller SC, Huang R, Sakamuru S, et al. Identification of known drugs that act as inhibitors of NF-κB signaling and their mechanism of action. Biochem Pharmacol. 2010;79:1272–1280.
- Shah V, Ferguson J, Hunsaker L, et al. Cardiac glycosides inhibit LPS-induced activation of pro-inflammatory cytokines in whole blood through an NF-κB-dependent mechanism. Int J Appl Res Nat Prod. 2011;4:11–19.
- Bleasel MD, Peterson GM. Emetine is not ipecac: considerations for its use as treatment for SARS-CoV2. Pharmaceuticals. 2020;13:428.
- Ryan JJ, Melendres-Groves L, Zamanian RT, et al. Care of patients with pulmonary arterial hypertension during the coronavirus (COVID-19) pandemic. Pulm Circ. 2020;10:2045894020920153.
- Forrester SJ, Kikuchi DS, Hernandes MS, et al. Reactive oxygen species in metabolic and inflammatory signaling. Circ Res. 2018;122:877–902.
- Honda T, Hirakawa Y, Nangaku M. The role of oxidative stress and hypoxia in renal disease. Kidney Res Clin Pract. 2019;38:414.
- Giussani DA, Niu Y, and Herrera EA, et al. Heart disease link to fetal hypoxia and oxidative stress. Adv Fetal Neonat Physiol. 2014;814:77–87.
- Siddique MAH, Satoh K, Kurosawa R, et al. Identification of emetine as a therapeutic agent for pulmonary arterial hypertension: novel effects of an old drug. Arterioscler Thromb Vasc Biol. 2019;39:2367–2385.
- Dresser LP, Massey EW, Johnson EE, et al. Ipecac myopathy and cardiomyopathy. J Neurol Neurosurg. 1993;56:560–562.
- Hopf NJ, Goebel HH. Experimental emetine myopathy: enzyme histochemical, electron microscopic, and immunomorphological studies. Acta Neuropathol. 1993;85:414–418.
- Halbig L, Gutmann L, Goebel H, et al. Ultrastructural pathology in emetine-induced myopathy. Acta Neuropathol. 1988;75:577–582.
- Thyagarajan D, Day BJ, Wodak J, et al. Emetine myopathy in a patient with an eating disorder. Med j Aust. 1993;159:757–760.
- Fan S, Zhen Q, Chen C, et al. Clinical efficacy of low-dose emetine for patients with COVID-19: a real-world study. J Bio-X Res. 2021;4:53–59.
- Dubick MA, Yang WC. Effects of chronic emetine treatment on mitochondrial function. J Pharm Sci. 1981;70:343–345.
- Yang WC, Dubick M. Mechanism of emetine cardiotoxicity. Pharmacol Ther. 1980;10:15–26.
- Dack S, Moloshok RE. Cardiac manifestations of toxic action of emetine hydrochloride in amebic dysentery. Arch Internal Med. 1947;79:228–238.
- Banerjea J. The effect of emetine therapy on the cardiovascular system. J Assoc Phys Ind. 1966;14:349–364.
- Kini P, Venugopal N, Santhamma K, et al. The effect of emetine on the electrocardiogram and the serum transaminases. J Assoc Phys Ind. 1969;17:457–461.
- Ho PC, Dweik R, Cohen MC. Rapidly reversible cardiomyopathy associated with chronic ipecac ingestion. Clin Cardiol. 1998;21:780–784.
- Khan MY, Haider B, Thind I. Emetine-induced cardiomyopathy in rabbits. J Submicro Cytol. 1983;15:495–507.
- Beller BM, Mongillo S. Observations on the mechanism of emetine poisoning of myocardial tissue. Circ Res. 1968;22:501–505.
- Brem TH, Konwaler BE. Fatal myocarditis due to emetine hydrochloride. Am Heart J. 1955;50:476–481.
- Mastrangelo MJ, Grage TB, Bellet RE, et al. A phase I study of emetine hydrochloride (NSC 33669) in solid tumors. Cancer. 1973;31:1170–1175.
- Kane R, Cohen M, Broder L, et al. Phase I-II evaluation of emetine (NSC-33669) in the treatment of epidermoid bronchogenic carcinoma. Cancer Chemother Rep. 1975;59:1171–1172.
- Siddiqui S, Firat D, Olshin S. Phase II study of emetine (NSC-33669) in the treatment of solid tumors. Cancer Chemother Rep. 1973;57:423–428.
- Wang DY, Salem J-E, Cohen JV, et al. Fatal toxic effects associated with immune checkpoint inhibitors: a systematic review and meta-analysis. JAMA Oncol. 2018;4:1721–1728.
- Ni J, Zhang L, Zhang X. Marked elevation of creatine phosphokinase alone caused by sintilimab-beware of hypothyroid myopathy. Eur J Cancer. 2020;128:57–59.
- Hale KK, Trollinger D, Rihanek M, et al. Differential expression and activation of p38 mitogen-activated protein kinase α, β, γ, and δ in inflammatory cell lineages. J Immunol. 1999;162:4246–4252.
- Kyriakis JM, Avruch J. Mammalian mitogen-activated protein kinase signal transduction pathways activated by stress and inflammation. Physiol Rev. 2001;81:807–869.
- Yokota T, Wang Y. p38 MAP kinases in the heart. Gene. 2016;575:369–376.
- Liang Q, Molkentin JD. Redefining the roles of p38 and JNK signaling in cardiac hypertrophy: dichotomy between cultured myocytes and animal models. J Mol Cell Cardiol. 2003;35:1385–1394.
- Nemoto S, Sheng Z, Lin A. Opposing effects of Jun kinase and p38 mitogen-activated protein kinases on cardiomyocyte hypertrophy. Mol Cell Biol. 1998;18:3518–3526.
- Zechner D, Thuerauf DJ, Hanford DS, et al. A role for the p38 mitogen-activated protein kinase pathway in myocardial cell growth, sarcomeric organization, and cardiac-specific gene expression. J Cell Biol. 1997;139:115–127.
- Engel FB, Hsieh PC, and Lee RT, et al. FGF1/p38 MAP kinase inhibitor therapy induces cardiomyocyte mitosis, reduces scarring, and rescues function after myocardial infarction. Proc Natl Acad Sci. USA. 2006;103:15546–15551.
- Engel FB, Schebesta M, Duong MT, et al. p38 MAP kinase inhibition enables proliferation of adult mammalian cardiomyocytes. Genes Dev. 2005;19:1175–1187.
- Wang Y, Huang S, Sah VP, et al. Cardiac muscle cell hypertrophy and apoptosis induced by distinct members of the p38 mitogen-activated protein kinase family. J Biol Chem. 1998;273:2161–2168.
- Kim JK, Pedram A, Razandi M, et al. Estrogen prevents cardiomyocyte apoptosis through inhibition of reactive oxygen species and differential regulation of p38 kinase isoforms. J Biol Chem. 2006;281:6760–6767.
- Liu H, Pedram A, Kim JK. Oestrogen prevents cardiomyocyte apoptosis by suppressing p38α-mediated activation of p53 and by down-regulating p53 inhibition on p38β. Cardiovasc Res. 2011;89:119–128.
- Kroemer G. The proto-oncogene Bcl-2 and its role in regulating apoptosis. Nat Med. 1997;3:614–620.
- Antonsson B, Martinou J-C. The Bcl-2 protein family. Exp Cell Res. 2000;256:50–57.
- Boon-Unge K, Yu Q, Zou T, et al. Emetine regulates the alternative splicing of Bcl-x through a protein phosphatase 1-dependent mechanism. Chem Biol. 2007;14:1386–1392.
- Sun Q, Yogosawa S, Iizumi Y, et al. The alkaloid emetine sensitizes ovarian carcinoma cells to cisplatin through downregulation of bcl-xL. Int J Oncol. 2015;46:389–394.
- Kaiser RA, Bueno OF, Lips DJ, et al. Targeted inhibition of p38 mitogen-activated protein kinase antagonizes cardiac injury and cell death following ischemia-reperfusion in vivo. J Biol Chem. 2004;279:15524–15530.
- Sharov VG, Todor A, Suzuki G, et al. Hypoxia, angiotensin‐II, and norepinephrine mediated apoptosis is stimulus specific in canine failed cardiomyocytes: a role for p38 MAPK, Fas‐L and cyclin D1. Eur J Heart Fail. 2003;5:121–129.
- Kim JH, Cho EB, Lee J, et al. Emetine inhibits migration and invasion of human non-small-cell lung cancer cells via regulation of ERK and p38 signaling pathways. Chem Biol Interact. 2015;242:25–33.
- Westermarck J, Li S-P, Kallunki T, et al. p38 mitogen-activated protein kinase-dependent activation of protein phosphatases 1 and 2A inhibits MEK1 and MEK2 activity and collagenase 1 (MMP-1) gene expression. Mol Cell Biol. 2001;21:2373–2383.
- Munshi HG, Wu YI, Mukhopadhyay S, et al. Differential regulation of membrane type 1-matrix metalloproteinase activity by ERK 1/2-and p38 MAPK-modulated tissue inhibitor of metalloproteinases 2 expression controls transforming growth factor-β1-induced pericellular collagenolysis. J Biol Chem. 2004;279:39042–39050.
- Son J, Lee SY. Emetine exerts anticancer effects in U2OS human osteosarcoma cells via activation of p38 and inhibition of ERK, JNK, and β‐catenin signaling pathways. J Biochem Mol Toxicol. 2021;35:e22868.
- Ma XL, Kumar S, Gao F, et al. Inhibition of p38 mitogen-activated protein kinase decreases cardiomyocyte apoptosis and improves cardiac function after myocardial ischemia and reperfusion. Circulation. 1999;99:1685–1691.
- Auger-Messier M, Accornero F, Goonasekera SA, et al. Unrestrained p38 MAPK activation in Dusp1/4 double-null mice induces cardiomyopathy. Circ Res. 2013;112:48–56.
- Croons V, Martinet W, Herman AG, et al. The protein synthesis inhibitor anisomycin induces macrophage apoptosis in rabbit atherosclerotic plaques through p38 mitogen-activated protein kinase. J Pharmacol Exp Ther. 2009;329:856–864.
- Marber MS, Rose B, Wang Y. The p38 mitogen-activated protein kinase pathway—a potential target for intervention in infarction, hypertrophy, and heart failure. J Mol Cell Cardiol. 2011;51:485–490.
- Barros LF, Young M, Saklatvala J, et al. Evidence of two mechanisms for the activation of the glucose transporter GLUT1 by anisomycin: p38 (MAP kinase) activation and protein synthesis inhibition in mammalian cells. J Physiol. 1997;504:517–525.
- Li H, Mittal A, Paul PK, et al. Tumor necrosis factor-related weak inducer of apoptosis augments matrix metalloproteinase 9 (MMP-9) production in skeletal muscle through the activation of nuclear factor-κB-inducing kinase and p38 mitogen-activated protein kinase: a potential role of MMP-9 in myopathy. J Biol Chem. 2009;284:4439–4450.
- Page TH, Brown A, Timms EM, et al. Inhibitors of p38 suppress cytokine production in rheumatoid arthritis synovial membranes: does variable inhibition of interleukin‐6 production limit effectiveness in vivo? Arthritis Rheumatism. 2010;62:3221–3231.
- Meng Q, Bhandary B, Osinska H, et al. MMI‐0100 inhibits cardiac fibrosis in a mouse model overexpressing cardiac myosin binding protein C. J Am Heart Assoc. 2017;6:e006590.
- Xu L, Yates CC, Lockyer P, et al. MMI-0100 inhibits cardiac fibrosis in myocardial infarction by direct actions on cardiomyocytes and fibroblasts via MK2 inhibition. J Mol Cell Cardiol. 2014;77:86–101.
- Brown DI, Cooley BC, Quintana MT, et al. Nebulized delivery of the MAPKAP kinase 2 peptide inhibitor MMI-0100 protects against ischemia-induced systolic dysfunction. Int J Pept Res Ther. 2016;22:317–324.
- Barbour AM, Sarov-Blat L, Cai G, et al. Safety, tolerability, pharmacokinetics and pharmacodynamics of losmapimod following a single intravenous or oral dose in healthy volunteers. Br J Clin Pharmacol. 2013;76(1):99–106.
- Cheriyan J, Webb AJ, Sarov-Blat L, et al. Inhibition of p38 mitogen-activated protein kinase improves nitric oxide–mediated vasodilatation and reduces inflammation in hypercholesterolemia. Circulation. 2011;123:515–523.
- Christie JD, Vaslef S, Chang PK, et al. A randomized dose-escalation study of the safety and anti-inflammatory activity of the p38 mitogen-activated protein kinase inhibitor dilmapimod in severe trauma subjects at risk for acute respiratory distress syndrome. Crit Care Med. 2015;43:1859–1869.