ABSTRACT
Background
Hypertensive retinopathy (HR) is a retinal disease that may lead to vision loss and blindness. Sex-determining region Y (SRY)-box (SOX) family transcription factors have been reported to be involved in HR development. In this study, the role and upstream mechanism of SRY-box transcription factor 17 (SOX17) in HR pathogenesis were investigated.
Methods
SOX17 and miR-194-5p levels in Angiotensin II (Ang II)-stimulated human retinal microvascular endothelial cells (HRMECs) and retinas of mice were detected by RT-qPCR. SOX17 protein level as well as levels of tight junction proteins and vascular endothelial growth factor (VEGF) signaling-associated proteins were quantified by western blotting. Tube formation assays were performed to evaluate angiogenesis in HRMECs. The structure of mouse retinal tissues was observed by H&E staining. The interaction between miR-194-5p and SOX17 was confirmed by a luciferase reporter assay.
Results
SOX17 was upregulated in HRMECs treated with Ang II. SOX17 knockdown inhibited angiogenesis in Ang II-stimulated HRMECs and increased tight junction protein levels. Mechanically, SOX17 was targeted by miR-194-5p. Moreover, miR-194-5p upregulation restrained angiogenesis and increased tight junction protein levels in Ang II-treated HRMECs, and the effect was reversed by SOX17 overexpression. MiR-194-5p elevation inactivated VEGF signaling via targeting SOX17. miR-194-5p alleviated pathological symptoms of HR in Ang II-treated mice, and its expression was negatively correlated with SOX17 expression in the retinas of model mice.
Conclusions
MiR-194-5p upregulation suppressed Ang II-stimulated HRMEC dysfunction and mitigates the symptoms of HR in mice by regulating the SOX17/VEGF signaling.
Introduction
Hypertensive retinopathy (HR) refers to retinopathy caused by arteriosclerosis and microcirculatory disturbance in hypertensive patients [Citation1]. The main manifestations of HR include retinal artery thinning, increased retinal reflectance, enhanced venous compression, the hemorrhage and exudation of retinas, and the existence of cotton wool spots in retinal surface [Citation2]. In severe cases, patients with HR even suffer from retinal vein thrombosis, exudative retinal detachment and loss of vision [Citation3]. For HR treatment, antihypertensive drugs, such as nifedipine sustained-release tablets and enalapril, are used to control blood pressure and then oral vitamin C, vitamin E, coxerutin are applied to alleviate ocular symptoms of patients with HR [Citation4]. However, these drugs cannot eradicate HR totally [Citation4]. Moreover, due to the strong association between HR signs and blood pressure, HR at the late stage is responsible for cardiovascular morbidity and increased risk of developing a uremic crisis [Citation5–7]. Therefore, exploring more effective therapeutic methods for patients with HR is necessary.
Vascular endothelium is a monolayer of flat cells lining the vascular wall [Citation8]. Vascular endothelium can sense changes in stimuli such as blood flow, hormones and inflammation and participate in the normal physiological function of the body by synthesizing and releasing a variety of cytokines [Citation9]. At the same time, it can also affect pathological processes such as hypertensive microangiopathy and tumor angiogenesis since extracellular matrix proteins such as fibronectin in blood vessels can control the stability and development of vasculature [Citation10,Citation11]. Human retinal microvascular endothelial cells (HRMECs) generate blood-retinal barrier (BRB) to maintain the stability of retinal microenvironment and limit access to inflammatory cells [Citation12]. In addition, HRMECs are the target cells for various physiological and pathological factors in hypertensive microangiopathy [Citation13]. Vascular endothelial function injury and neovascularization are the main pathological manifestations of retinopathy [Citation14]. Vascular endothelial growth factor (VEGF) is an indispensable vascular growth-inducing factor during angiogenesis, which can promote vascular endothelial cell division and accelerate angiogenesis [Citation15]. As an important part of the blood-eye barrier, BRB consists of outer retinal barrier and inner BRB [Citation16]. Inner BRB is mainly composed of RMECs and tight junction proteins (e.g. ZO-1, Occludin and Claudin-5) [Citation17]. Inner BRB stability depends on vascular homeostasis and is one of the bases of normal visual function [Citation18]. Under hypertensive environment, a decrease in protein levels of tight junctions elevates BRB permeability, subsequently causing HR progression [Citation19].
The sex-determining region Y (SRY)-box (SOX) gene family encodes transcription factors characterized by high mobility group DNA-binding domains [Citation20]. SOX family has been involved in regulating arterial-venous identity and the development of blood and lymphatic vasculature [Citation21]. SRY-box transcription factor 17 (SOX17) is a member of the SOX family and regulates embryonic development [Citation22]. The proteins encoded by SOX17 exert important functions in maintaining cell identity and determining cell fate [Citation23,Citation24]. Notably, SOX17 participates in angiogenesis in the progression of tumors [Citation25,Citation26]. Furthermore, SOX17 downregulation inhibits sprouting angiogenesis in endothelial cells during the embryonic and postnatal vascular development of mice and SOX17 expression is reduced by Notch activation in primary endothelial cells and in retinal angiogenesis [Citation27]. As a proangiogenic regulator, SOX17 is required for wound healing, and SOX17-deletion mice exhibit quite delayed wound healing [Citation28]. SOX17 is elevated during angiogenesis and remodeling of coronary arteries [Citation29]. Different combinations of SOX genes (SOX7, SOX17 and SOX18) contribute to retinal vascular defects of varying severity [Citation30]. Although a study has reported that SOX17 downregulation leads to non-hemorrhagic leakage in the retina during the progression of HR [Citation19], the specific upstream mechanism of SOX17 in HR pathogenesis is still uncharacterized.
In this study, the functions and molecular mechanism of SOX17 in HR development were explored. The study may provide a promising therapeutic method for patients with HR.
Material and methods
Bioinformatic analysis
The potential miRNAs binding to SOX17 and the binding site between miR-194-5p and SOX17 3”untranslated region (3”UTR) were predicted using Targetscan (http://www.targetscan.org/).
Cell culture and treatment
HRMECs were purchased from Haodi Huatuo Biotechnology Co. Ltd (Shenzhen, China). HRMECs were cultured in Dulbecco’s modified Eagle’s medium (DMEM; Gibco BRL, Grand Island, NY, USA) supplemented with 10% fetal bovine serum (Gibco) at 37°C with 5% CO2. Next, 150 μmol/L of Angiotensin II (Ang II) (Sigma, St. Louis, MO, USA) was used to treat HRMECs for 48 h [Citation31]. HRMECs without Ang II treatment served as a control (Con) group.
Cell transfection
Short hairpin RNA (shRNA) targeting SOX17 (sh-SOX17) was used to knock down SOX17, and its negative control (NC) was named sh-NC as a negative control. MiR-194-5p mimics were used to overexpress miR-194-5p with NC mimics as a negative control. Coding region of SOX17 was subcloned into the pcDNA3.1 vector to elevate SOX17 functions with empty pcDNA3.1 vector as a negative control. Afterward, cell transfection was conducted using Lipofectamine 2000 (Invitrogen, USA). The concentration of shRNA is 40 nM and that for miRNA mimics is 50 nM. The transfection efficiency was examined using reverse transcription quantitative polymerase chain reaction (RT-qPCR) after 48 h.
Animal grouping
A total of 40 healthy C57BL/6 mice (male, 8–10 weeks old) were purchased from Cyagen Biosciences Inc. (Suzhou, China). One mouse was regarded as an experiment unit. All mice were allowed to access standard diet ad libitum and housed in a 12-h light/12-h dark light cycle. Mice were kept in the same environment and were separately treated to minimize potential confounders. To have statistical significance, more than 6 available data are required in each group. Considered other uncontrollable factors, the number of mice in each group was set as ten. All mice were randomly divided into four groups using random number table: SHAM+AAV-NC (n = 10), SHAM+AAV-miR-194-5p (n = 10), angiotensin II (Ang-II)+AAV-NC (n = 10), and Ang-II+AAV-miR-194-5p (n = 10). Group allocation, the conduct of the experiment, and outcome assessment as well as data analysis were independently performed by a designer, a conductor, and an evaluator.
Animal model establishment
As described previously [Citation32,Citation33], dorsum of all mice under anesthetization were implanted subcutaneously with osmotic minipumps. Mice in SHAM group were infused with saline (3000 ng/kg/min) and mice in Ang-II group received Ang-II (3000 ng/kg/min) using the minipumps at the rate of 0.11 μl/h. After 3 weeks of infusion, systolic and diastolic blood pressures were measured every 3 days using tail-cuff method (Kent Scientific, USA) in the morning and then mean value was calculated. Mice were considered as hypertensive only when systolic blood pressure level >140 mmHg and/or diastolic blood pressure >90 mmHg.
Adeno-Associated virus (AAV) injection
MiR-194-5p mimics (Genechem) and NC mimics (Genechem) were constructed onto adeno-associated virus (AAV) (Guyan Industrial Co., Ltd, Shanghai, China). After animal model was established successfully, 1 µl (2.13 × 1013 vg/mL) of AAV-NC and 1 µl (2.13 × 1013 vg/mL) of AAV-miR-194-5p were injected intravitreally into mice of the Ang-II group twice a week [Citation34]. After intravitreal injection with AAV-NC and AAV-miR-194-5p for 3–4 weeks, 100 mg/kg of pentobarbital (Sigma) was applied to anaesthetize all mice via intraperitoneal injection. Then, the blood of mice was collected, and the eyes were removed for further analysis. All the experiments involving mice were approved by the Ethics Committee of The Second Hospital of Anhui Medical University. No adverse events were reported in the study.
Tube formation assay
As previously described [Citation35], After culture on the different fiber meshes for 7 d, HRMECs were collected. According to the given protocol, 24-well tissue culture plate (TCP) was coated with Geltrexs Matrix solution (GMS) (Life Technologies, Germany) to assess tube formation ability of HRMECs. Subsequently, harvested HRMECs were seeded on the GMS-coated wells (7 × 104 cells/well) filled with cell culture medium. After 18 h of incubation, phase contrast microscope (AxioSkop, Carl Zeiss, Jena, Germany) was used to visualize and image the formed tubes. Random fields were selected to take images in each well. Image J software (National Institutes of Health, USA) and the software plugin “Angiogenesis Analyzer” were used to count the number of meshes and branch length. All experiments were repeated three times.
Hematoxylin & eosin (H&E) staining
Eyes separated from mice were fixed in 4% paraformaldehyde (Solarbio, Beijing, China), dehydrated, paraffin-embedded and then sliced up into sections (5 µm thickness) through the optic nerve head. Then, the eye specimen was subjected to hematoxylin staining for 5 min and eosin staining for 2 min. After that, the stained eye specimen was washed in distilled water for 1 min. Gradient alcohol and xylene were used for dehydration and cleanout of the specimen. Morphological structure of eye specimen collected from mice was observed using a light microscope (Olympus).
Western blotting
Radioimmunoprecipitation lysis buffer (Beyotime, Nanjing, China) was used to treat proteins extracted from HRMECs and retinal tissues of mice. BCA Protein Assay Kit (Thermo Fisher Scientific, USA) was applied to determine concentration of isolated proteins. Protein samples were loaded at 12% SDS-PAGE and then transferred onto PVDF membranes (Millipore, USA). Subsequently, the membranes were incubated with primary antibodies at 4°C overnight. Primary antibodies included anti-SOX17 (ab224637; 1:500; abcam), anti-zonula occludens 1 (ZO-1; ab221547; 1:500; abcam), anti-Occludin (ab216327; 1:500; abcam), anti-Claudin-5 (ab131259; 1:500; abcam), anti-VEGFA (ab52917; 1:500; abcam), anti-VEGFB (ab110649; 1:1000; abcam), anti-VEGFR (ab11939; 1:1000; abcam), and GAPDH (ab8245; 1:1000; abcam). GAPDH was used as a loading control. Then, the membranes were incubated with a secondary antibody horseradish peroxidase-labeled Immunoglobulin (IgG; ab6721; 1:1000; abcam) at room temperature for 2 h. The protein bands were visualized using enhanced chemiluminescence reagent (Bio-Rad, Hercules, CA, USA), and the intensity of bands was defined using ImageJ software (NIH, Bethesda, MA, USA). All experiments were performed in triplicate.
RT-qPCR
Total RNA was isolated from HRMECs and retinal tissues of mice using TRIzol reagent (Invitrogen) and then was reverse transcribed to complementary DNA (cDNA) using a First Strand cDNA synthesis kit (Roche, Switzerland). Next, a PrimeScript RT reagent Kit (Invitrogen) was used for RT-qPCR analysis through IQ5 real-time PCR system (Bio-Rad, USA). The levels of miR-194-5p and SOX17 were calculated using the 2−ΔΔCt method and were, respectively, normalized to U6 and GAPDH levels. Sequences of primers used in RT-qPCR are presented in . All experiments were conducted in thrice.
Table 1. Sequences of primers used for reverse transcription-quantitative PCR.
Luciferase reporter assay
The wild type (Wt) or mutant (Mut) type of SOX17 3”UTR containing the binding site of miR-194-5p was subcloned into the pmirGLO vector (Promega, Madison, WI, USA) to generate SOX17 3”UTR-Wt/Mut reporter. Then, pmirGLO vector carrying SOX17 3’UTR and miR-194-5p mimics were cotransfected into HRMECs using Lipofectamine 2000 (Invitrogen). Luciferase Reporter Assay System (Promega) was applied to test luciferase activities. The assay was repeated three times.
Fluorescence in situ hybridization
After fixed with 4% paraformaldehyde at room temperature for 15 min, the samples were incubated with 0.2% Triton X-100 for 20 min. Next, SOX17 probe constructed by RiboBio (Guangzhou, China) was utilized to perform hybridization overnight in the darkness. The hybridization buffer was prepared utilizing the Fluorescence in situ hybridization Kit (RiboBio, China) according to the manufacturer’s protocols. After the samples were washed with sodium citrate solution, the nuclei were stained with DAPI. An Eclipse Ti inverted microscope (Nikon, Japan) was used to capture images.
Statistical analysis
Data in this study are exhibited as the mean ± standard deviation and statistically analyzed by GraphPad Prism 7 (La Jolla, CA, USA). Differences between two groups were compared using Mann–Whitney tests and those among multiple groups were evaluated using one-way analysis of variance followed by Tukey’s post hoc analysis. Correlation between miR-194-5p expression and SOX17 expression in retinal tissues of Ang-II-treated mice was analyzed by Pearson correlation analysis. For each experimental group, no animals or data points were not included in the analysis. For each analysis, n = 10 in each experimental group. Results were considered statistically significant when the p value was less than 0.05.
Results
SOX17 knockdown inhibits angiogenesis and upregulates levels of tight junction proteins in Ang-II-treated HRMECs
Since SOX17 was reported to be associated with hypertension-induced pathological leakage in the retina [Citation19], the precise function of SOX17 was explored in this study. First, Ang-II was used to stimulate HRMECs to mimic hypertensive environment, and HRMECs without Ang-II stimulation served as the control (Con) group. Compared with Con group, mRNA levels and protein levels of SOX17 in Ang-II-treated HRMECs were increased, as shown by RT-qPCR and western blot (). After transfection of sh-SOX17, mRNA and protein levels of SOX17 were significantly downregulated in HRMECs treated with Ang-II ( –). As indicated, the number of meshes and length of branches were increased in HRMECs after Ang-II treatment compared with Con group, and SOX17 knockdown suppressed the tube formation ability of Ang-II-stimulated HRMECs. In addition, Ang-II treatment markedly downregulated levels of tight junction proteins (ZO-1, Occludin and Claudin-5) in HRMECs, and SOX17 silencing elevated protein levels of tight junctions in Ang-II-stimulated HRMECs (). Overall, SOX17 knockdown inhibits angiogenesis and upregulates protein levels of tight junctions in Ang-II-treated HRMECs.
Figure 1. SOX17 knockdown inhibits angiogenesis and upregulates protein levels of tight junctions in Ang-II-treated HRMECs. (a-b) After transfection of sh-NC or sh-SOX17, SOX17 mRNA and protein levels in HRMECs with or without Ang-II treatment were detected by RT-qPCR and western blotting, respectively. (c) After transfection of sh-NC or sh-SOX17, angiogenesis of HRMECs with or without Ang-II treatment was examined by tube formation assays. (D) the protein levels of tight junctions (ZO-1, Occludin and Claudin-5) in HRMECs transfected with sh-NC/sh-SOX17 and treated with or without Ang-II were tested by western blotting. ** p <0.01, *** p <0.001.
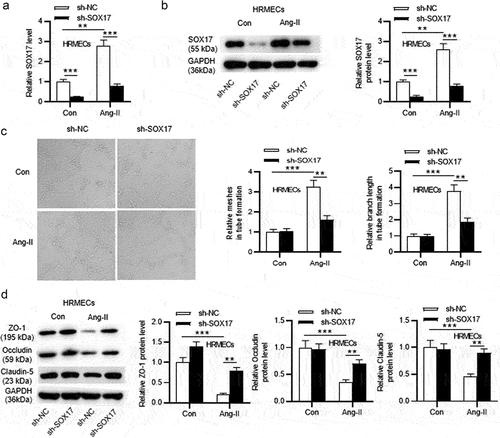
SOX17 is targeted by miR-194-5p
To investigate the mechanism of SOX17 in HRMECs, four upstream miRNAs (miR-141-3p, miR-200a-3p, miR-194-5p and miR-26-5p) of SOX17 were predicted using Targetscan (). In Ang-II-treated HRMECs, only miR-194-5p expression was significantly downregulated compared with the expression of other candidate miRNAs (). Thus, miR-194-5p was selected for the subsequent experiments. According to RT-qPCR, miR-194-5p mimics significantly increased miR-194-5p expression and decreased SOX17 mRNA expression in HRMECs (). A binding site between miR-194-5p and SOX17 was predicted using TargetScan. Additionally, the binding site is highly conserved among vertebrates (). Luciferase reporter assay exhibited that miR-194-5p upregulation markedly decreased the luciferase activity of SOX17 3”UTR-Wt rather than that of SOX17 3”UTR-Mut in HRMECs (), indicating the binding relationship between miR-194-5p and SOX17. In addition, SOX17 protein level is upregulated in Ang-II-treated HRMECs and miR-194-5p mimics downregulated the protein level of SOX17 in HRMECs treated with or without Ang-II (). In summary, SOX17 is targeted by miR-194-5p in HRMECs.
Figure 2. SOX17 is targeted by miR-194-5p. (a) Four upstream miRnas (miR-141-3p, miR-200a-3p, miR-194-5p and miR-26-5p) of SOX17 were predicted using Targetscan. (b) the expression of candidate miRnas in HRMECs treated with or without Ang-II was detected by RT-qPCR. (c) MiR-194-5p and SOX17 levels in HRMECs transfected with miR-194-5p mimics or NC mimics were tested by RT-qPCR. (d) a binding site between miR-194-5p and SOX17 was predicted using Targetscan. (e) the binding relationship between miR-194-5p and SOX17 was confirmed by luciferase reporter assay. (f) SOX17 protein level in HRMECs transfected with NC mimics/mir-194-5p mimics and treated with or without Ang-II was quantified by western blotting. ** p <0.01, *** p <0.001.
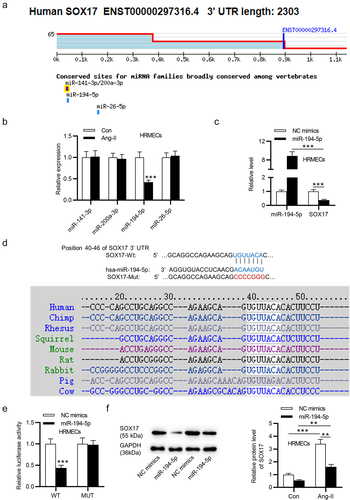
SOX17 overexpression reverses the suppressive effect of miR-194-5p upregulation on retinal endothelial cell dysfunction
Next, whether miR-194-5p inhibits HRMEC dysfunction by targeting SOX17 was explored. The mRNA and protein levels of SOX17 in Ang-stimulated HRMECs after transfection of pcDNA3.1/SOX17 were elevated (). The tube formation assay revealed that miR-194-5p upregulation decreased the number of meshes and the length of branches, and overexpressed SOX17 partially reversed the suppressive effect of miR-194-5p upregulation on the tube formation ability (). Protein levels of tight junctions (ZO-1, Occludin and Claudin-5) in Ang-II treated HRMECs was increased after miR-194-5p overexpression, and the increase was partially reversed by SOX17 upregulation (). Overall, SOX17 elevation reverses the inhibitory effect of miR-194-5p upregulation on retinal endothelial cell dysfunction under Ang-II treatment.
Figure 3. SOX17 overexpression reverses the effects of miR-194-5p upregulation on angiogenesis and protein levels of tight junctions in Ang-II-treated HRMECs. (a-b) the mRNA and protein levels of SOX17 in Ang-II-stimulated HRMECs after overexpressing SOX17 were detected by RT-qPCR and western blotting, respectively. (c) Angiogenesis of Ang-II-treated HRMECs after upregulating miR-194-5p or SOX17 was examined by tube formation assays. (b) the protein levels of tight junctions (ZO-1, Occludin and Claudin-5) in HRMECs upon Ang-II treatment after upregulating miR-194-5p or SOX17 were tested by western blotting. *p <0.05, ** p <0.01, *** p <0.001.
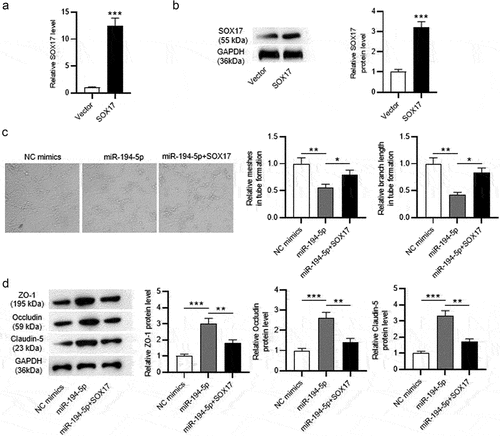
MiR-194-5p inactivates VEGF pathway by downregulating SOX17
It is demonstrated that SOX17 deficiency suppresses angiogenesis by regulating VEGF signaling pathway in HR development [Citation19]. Here, we investigated whether miR-194-5p can control the activation of VEGF signaling pathway by regulating SOX17 expression in Ang-II-treated HRMECs. As indicated, miR-194-5p overexpression decreased protein levels of VEGF signaling-associated genes (VEGFA, VEGFB and VEGFR) in Ang-II-stimulated HRMECs. Additionally, the decrease in VEGFA, VEGFB and VEGFR protein levels was partially reversed by SOX17 overexpression. Therefore, we concluded that miR-194-5p upregulation inactivates VEGF pathway by downregulating SOX17 in Ang-II-stimulated HRMECs.
Overexpressing miR-194-5p reduces retinal thickness and elevates protein levels of tight junctions in Ang-II-treated mice
To further investigate the role of miR-194-5p in HR pathogenesis, in vivo experiments were also carried out. First, electroretinograms (ERGs) were performed to detect whether Ang-II affected the visual function of mice. As shown by , the relative ratio of b-wave ratio was significantly decreased by Ang-II after the establishment of the animal model. H&E staining revealed that the whole retina was thickened in mice treated with Ang-II (). In addition, the thickness of central retinas, particularly the inner plexiform layer (IPL) and inner nuclear layer (INL) were higher in Ang-II-treated mice than that of the mice in the SHAM group ( –). Additionally, miR-194-5p overexpression significantly reversed these effects induced by Ang-II ( –). Moreover, Ang-II stimulation decreased protein levels of tight junctions (ZO-1, Occludin and Claudin-5) expression in mice, and the suppressive impact was counteracted by miR-194-5p elevation (). All these results suggested that miR-194-5p overexpression reduces retinal thickness and increases protein levels of tight junctions in Ang-II-treated mice.
Figure 5. Overexpressing miR-194-5p mitigates retinal thickness and elevates tight junction proteins of Ang-II-treated mice. (a) the effect of Ang-II on the b-wave ratio in electroretinograms (ERGs) was determined. (b-e) H&E staining was used to assess the central retinal thickness, IPL thickness and INL thickness in sham-operated mice and Ang-II-treated mice after injection with AAV-NC or AAV-miR-194-5p. (f) Protein levels of tight junctions in retinal tissues of sham-operated mice and Ang-II-treated mice after injection with AAV-NC or AAV-miR-194-5p were quantified by western blotting. *p <0.05, ** p <0.01, *** p <0.001.
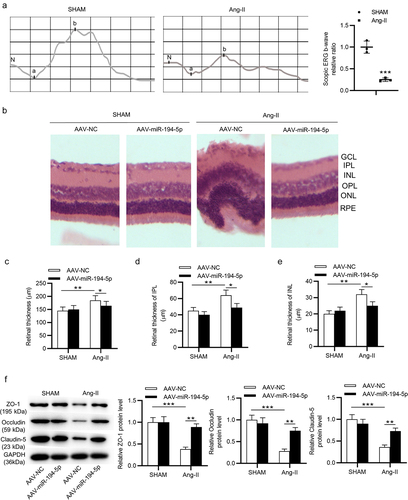
MiR-194-5p upregulation inhibits VEGF pathway in Ang-II-treated mice
Next, the influence of miR-194-5p on VEGF pathway in vivo was explored. As demonstrated, Ang-II-stimulated mice exhibited increased retinal VEGFA, VEGFB and VEGFR protein levels compared with the mice in the SHAM group. Nevertheless, miR-194-5p upregulation attenuated the activation of VEGF signaling induced by Ang-II in vivo (). In summary, miR-194-5p elevation inhibits VEGF pathway in Ang-II-treated mice.
Figure 6. MiR-194-5p upregulation inhibits VEGF pathway in Ang-II-treated mice. (a) Protein levels of genes (VEGFA, VEGFB and VEGFR) involved in the VEGF signaling pathway were quantified by western blotting in retinal tissues of sham-operated mice and Ang-II-treated mice after injection with AAV-NC or AAV-miR-194-5p was examined by western blotting. n = 10 mice/group. *p <0.05, ** p <0.01, *** p <0.001.
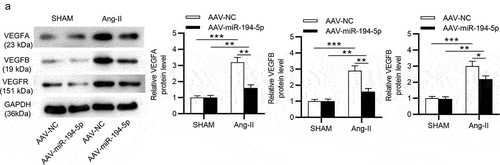
MiR-194-5p negatively regulates SOX17 in Ang-II-treated mice
Downregulated miR-194-5p () and upregulated SOX17 () were detected in retinal tissues of Ang-II-treated mice. After injection of AAV- miR-194-5p into Ang-II-stimulated mice, miR-194-5p expression was significantly upregulated () and SOX17 expression was decreased in retinal tissues (). Pearson correlation analysis revealed that miR-194-5p expression was negatively correlated with SOX17 expression in retinal tissues of mice (). All these findings suggested that miR-194-5p negatively regulates SOX17 in vivo. Moreover, fluorescence in situ hybridization (FISH) was performed to detect the in situ expression of SOX17 in retinal tissues of four groups. We found that SOX17 in situ expression was downregulated in sham+AAV-miR-194-5p group compared with that in sham group (). After Ang-II treatment, SOX17 in situ expression was markedly increased compared with that in sham group, and miR-194-5p overexpression attenuated the Ang-II-induced increase in SOX17 expression (). The findings further validated the in vivo results.
Figure 7. MiR-194-5p negatively regulates SOX17 in Ang-II-treated mice. (a-b) the expression of miR-194-5p and SOX17 in retinal tissues of sham-operated mice (n = 10 mice/group) and Ang-II-treated mice (n = 10 mice/group) after injection with AAV-NC or AAV-miR-194-5p was detected by RT-qPCR. (c) Correlation between miR-194-5p expression and SOX17 expression in retinal tissues of Ang-II-treated mice (n = 20) was analyzed by Pearson correlation analysis. (d) Fluorescence in situ hybridization (FISH) was performed to determine the in situ expression of SOX17 in retinal tissues of mice with or without Ang-II after injection of AAV-NC or AAV-miR-194-5p. *p <0.05, ** p <0.01, *** p <0.001.
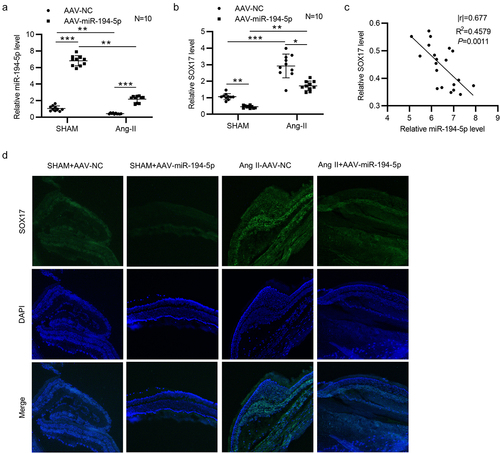
Discussion
HR is a retinal microvasculature disease caused by blood pressure elevation [Citation36]. Ang II can increase blood pressure by constricting systemic arterioles [Citation37]. In this study, Ang II was used to treat HRMECs and mice to simulate the hypertensive condition. After Ang II treatment, the mesh number and branch length were increased in HRMECs. In addition, Ang-II stimulation decreased protein levels of tight junctions (ZO-1, Occludin and Claudin-5) in HRMECs, indicating the successfully establishment of the in vitro cell model of HR. In in vivo studies, compared with sham-operated mice, Ang II-stimulated mice exhibited increased central retinal thickness, IPL thickness and INL thickness as well as decreased tight junction protein levels.
Accumulating studies have demonstrated that SOX family members are closely associated with retinal development [Citation38]. Downregulation of SOX8 and SOX9 decreases the number of Müller glial cells in mouse retina and increases rod photoreceptor proportion in retinas [Citation39]. High overlapping of SOX4 and SOX11 have been found in retinal development during embryonic stages and loss of SOX4 and SOX11 lead to RGC absence [Citation40]. As a subgroup of SOX family, SOX17 was reported to play a critical role in the pathogenesis of pulmonary arterial hypertension [Citation41,Citation42]. Norrin/Fz/Lrp signaling causes vascular growth defects in endothelial cells to decrease retinal neurons, and SOX17 is a major mediator in Norrin/Fz4/Lrp-controlled angiogenic program [Citation43]. Herein, SOX17 was detected to be overexpressed in HRMECs of Ang II group rather than in those of Con group. Notably, SOX17 was also upregulated in Ang-II-treated mice compared with that in sham-operated mice. Therefore, SOX17 may participate in HR progression. Then, the functions of SOX17 in Ang II-stimulated HRMECs were investigated. We observed that SOX17 knockdown inhibited Ang-II-induced angiogenesis and reversed the reduction in tight junction protein levels caused by Ang-II in HRMECs. Thus, SOX17 serves as a proangiogenic transcription factor and reduces protein levels of tight junctions in Ang-II-treated HRMECs to promote HR development.
MiRNA refers to the small noncoding RNA with 19–25 nucleotides in length that can bind with the 3’UTR of the target mRNA to degrade gene expression and suppress protein translation process [Citation44,Citation45]. Some studies have revealed the involvement of miRNAs in the development of retinopathy [Citation46,Citation47]. For example, miR-29a elevation decreases retinal vascular density and neovascularization by binding to angiotensinogen in the mice with oxygen-induced retinopathy [Citation48]. MiR-637 is downregulated in HR and targets STAT3 to restrict the proliferative and migratory abilities of retinal endothelial cells [Citation49]. In this study, miR-194-5p was identified as the downstream miRNA of SOX17, and miR-194-5p negatively regulated SOX17 in HRMECs and retinal tissues of mice. Downregulated miR-194-5p was detected in Ang II-stimulated HRMECs and mice, indicating that miR-194-5p was associated with HR development. In in vitro studies, miR-194-5p upregulation decreased the tube formation ability and increased protein levels of tight junctions in Ang II-stimulated HRMECs, and these effects were reserved by SOX17 elevation. All these suggested that miR-194-5p suppresses angiogenesis and upregulates protein levels of tight junctions in Ang II-stimulated HRMECs. More importantly, miR-194-5p overexpression decreased central retinal thickness, IPL thickness and INL thickness while upregulating protein levels of tight junctions in mice of Ang II group. The results demonstrated that overexpressing miR-194-5p inhibits pathological symptoms of HR in Ang II-treated mice. Therefore, miR-194-5p plays a protective role in HR pathogenesis.
VEGF is a heparin-bound homodimer glycoprotein and a potent mitotic promoter [Citation50]. VEGF activates phospholipase C-γ (PlcY) that can hydrolyze phosphatidylinositol 4,5-bisphosphate (PIP2) to produce inositol 1,4,5-trisphosphate (IP3) and diacylglycerol (DAG), and IP3 induces intracellular Ca2+ release to promote prostaglandin production and improve vascular permeability [Citation51]. VEGF family members include VEGFA, VEGFB, VEGFC and VEGFD, among which the bioregulatory activity of VEGFA is the most important [Citation52]. VEGF receptors refer to VEGFR1 (FLT1), VEGFR2 (KDR/FLK1) and VEGFR3 (FLT4) [Citation53]. The signaling pathway mediated by VEGF/VEGFR can control the angiogenesis by regulating the proliferation, migration, and survival of vascular endothelial cells, leading to vascular tumors, vascular penetration, edema, and inflammation [Citation54]. VEGF signaling was reported to participate in the pathogenesis of retinopathy [Citation55]. For example, miR-142-5p knockdown activates IGF/IGF1 R-mediated signaling pathways (p-PI3K, p-ERK, p-AKT, and VEGF) to enhance high glucose-induced human retinal endothelial cell proliferation [Citation56]. MiRNA-21 repression suppresses the growth and angiogenesis of vascular endothelial cells via PTEN dependent-PI3K/Akt/VEGF signaling pathway in diabetic retinopathy [Citation57]. Moreover, SOX17 was reported to participate in the regulation VEGF signaling in HR [Citation19]. Endothelial SOX17 silencing restrains Dll4 in retinal arteries to drive vascular leakage, which is attenuated by inhibition of SREBP1 or VEGF-VEGFR2 signaling [Citation58]. Herein, miR-194-5p overexpression reduced protein levels of VEGF signaling-associated genes (VEGFA, VEGFB and VEGFR) in HRMECs stimulated with Ang-II, and these effects mediated by miR-194-5p overexpression were offset by SOX17 elevation. The results suggested that miR-194-5p inactivated the VEGF signaling by SOX17. Furthermore, the activation of VEGF signaling mediated by miR-194-5p was verified in vivo.
Furthermore, according to the previous study, Ang II reduces SOX17 expression and upregulates downstream molecules (SREBP1 and VEGFR2) via reactive oxygen species (ROS) [Citation19]. Specifically, Ang II generates ROS and decreases SOX17, and the treatment of N-acetyl cysteine (an ROS inhibitor) restores SOX17 expression in human retinal endothelial cells [Citation19]. The imbalance between the production and breakdown of ROS is defined as oxidative stress which is a major cause of endothelial dysfunction [Citation59,Citation60]. Whether Ang II can induce oxidative stress in HRMECs has not been reported, and reports focusing on the oxidative stress in HRMECs is limited. However, clinical evidence demonstrates that hypertensive patients with retinopathy exhibit high gamma-glutamyl transferase activity, an indicator of oxidative stress [Citation61]. Thus, oxidative stress might be involved in the process of HR. Moreover, SOX17 was reported to increase ROS level in cholangiocarcinoma cells [Citation62]. miR-194-5p is downregulated in hypoxia/reoxygenation H9c2 in vitro cell model and inhibits oxidative stress by targeting MAPK1 through PTEN/AKT pathway [Citation63]. In response to high dose of hydrogen peroxide, miR-194-5p expression is increased in retinal pigment epithelium cells (ARPE-19) [Citation64]. Hence, the role of miR-194-5p/SOX17 in oxidative stress is complex and more experiments will be conducted in the future to verify the relationship of oxidative stress, miR-194-5p/SOX17 axis, and HR.
In conclusion, miR-194-5p upregulation suppressed the dysfunction of Ang II-treated HRMECs and mitigates the symptoms of HR in mice by regulating the SOX17/VEGF signaling. This study may provide a promising approach for future HR treatment. However, the limitations existed in the study cannot be ignored. For example, the upstream genes of miR-194-5p were not further explored in the study. In addition, the roles of other signaling pathways, such as Notch signaling and Wnt signaling pathway were not investigated here. According to previous reports, Notch signaling and Wnt signaling are also associated with retinal vasculature development [Citation65]. Hence, other potential genes or signaling pathways implicated with the miR-194-5p/SOX17 axis in HR development will be explored in future studies.
Data availability
All relevant data are available from the corresponding author upon request.
Disclosure statement
No potential conflict of interest was reported by the author(s).
Additional information
Funding
References
- Modi P, Arsiwalla T. Hypertensive retinopathy. Stat pearls treasure Island (FL): stat pearls publishing copyright © 2021,Stat Pearls Publishing LLC; 2021.
- Harjasouliha A, Raiji V, Garcia Gonzalez JM. Review of hypertensive retinopathy. Dis Mon. 2017 March;63(3):63–69.
- Wong TY, Mitchell P. Hypertensive retinopathy. N Engl J Med. 2004 November 25;351(22):2310–2317. DOI:10.1056/NEJMra032865
- Tsukikawa M, Stacey AW. A review of hypertensive retinopathy and chorioretinopathy. Clin Optom (Auckl). 2020;12:67–73.
- Wong TY, McIntosh R. Hypertensive retinopathy signs as risk indicators of cardiovascular morbidity and mortality. Br Med Bull. 2005;73-74:57–70.
- Jacob F, Polzin DJ, Osborne CA, et al. Association between initial systolic blood pressure and risk of developing a uremic crisis or of dying in dogs with chronic renal failure. J Am Vet Med Assoc. 2003 February 1 222;(3)322–329. 10.2460/javma.2003.222.322
- Wong W, Gopal L, Yip CC. Hypertensive retinopathy and choroidopathy. Cmaj. 2020 April 6;192(14):E371. DOI:10.1503/cmaj.191275
- Ali HJB. SCUBE2, vascular endothelium, and vascular complications: a systematic review. Biomed Pharmacother. 2020;127:110129.
- Kumar G, Dey S, Sjls K. Functional implications of vascular endothelium in regulation of endothelial nitric oxide synthesis to control blood pressure and cardiac functions. Life Sci. 2020;259:118377.
- Tang X, Wang J, Wang J, et al. Endothelium-Specific deletion of Nox4 delays retinal vascular development and mitigates pathological angiogenesis. Angiogenesis. 2021;24(2):363–377. DOI: 10.1007/s10456-020-09757-3
- Turner CJ, Badu-Nkansah K, Hynes RO. Endothelium-Derived fibronectin regulates neonatal vascular morphogenesis in an autocrine fashion. Angiogenesis. 2017 November;20(4):519–531.
- Ragelle H, Dernick K, Khemais S, et al. Human retinal microvasculature-on-a-chip for drug discovery. Adv Healthc Mater. 2020 November;9(21):e2001531. DOI:10.1002/adhm.202001531
- Wang Z, Zhang X, Wang Y, et al. Dysregulation of miR-374a is involved in the progression of diabetic retinopathy and regulates the proliferation and migration of retinal microvascular endothelial cells. Clin Exp Optom. 2021;1–6.
- Ozdemir O, Arman A, Cjgsafc T, et al. Intraocular pressure effect of anti-vascular endothelial growth factor injection for aggressive posterior retinopathy of prematurity. Graefe’s Archive for Clinical and Experimental Ophthalmology. 2021:3469–3476. DOI:10.1007/s00417-021-05278-x
- Geindreau M, Ghiringhelli F, Mjijoms B. Vascular endothelial growth factor, a key modulator of the anti-tumor immune response. Int J Mol Sci. 2021;22(9). DOI:10.3390/ijms22094871
- Tawfik A, Samra YA, Elsherbiny NM, et al. Implication of hyperhomocysteinemia in blood retinal barrier (BRB) dysfunction. Biomolecules. 2020 July 29; 10(8). 10.3390/biom10081119
- Napoli D, Biagioni M, Billeri F, et al. Retinal pigment epithelium remodeling in mouse models of retinitis pigmentosa. Int J Mol Sci. 2021 May 20 22;(10)5381. 10.3390/ijms22105381
- Ţălu Ş, Nicoara SD. Malfunction of outer retinal barrier and choroid in the occurrence and progression of diabetic macular edema. World J Diabetes. 2021 Apr 15;12(4):437–452. DOI:10.4239/wjd.v12.i4.437
- Yang JM, Park CS, Kim SH, et al. Dll4 suppresses transcytosis for arterial blood-retinal barrier homeostasis. Circ Res. 2020 March 13 126;(6)767–783. 10.1161/CIRCRESAHA.119.316476
- Gubbay J, Collignon J, Koopman P, et al. A gene mapping to the sex-determining region of the mouse Y chromosome is a member of a novel family of embryonically expressed genes. Nature. 1990 July 19 346;(6281)245–250. 10.1038/346245a0
- Francois M, Koopman P, Beltrame M. SoxF genes: key players in the development of the cardio-vascular system. Int J Biochem Cell Biol. 2010 March;42(3):445–448.
- Nakajima-Takagi Y, Osawa M, Oshima M, et al. Role of SOX17 in hematopoietic development from human embryonic stem cells. Blood. 2013 January 17 121;(3)447–458. 10.1182/blood-2012-05-431403
- Irie N, Weinberger L, Tang WW, et al. SOX17 is a critical specifier of human primordial germ cell fate. Cell. 2015 January 15 160;(1–2)253–268. 10.1016/j.cell.2014.12.013
- Tan DS, Holzner M, Weng M, et al. SOX17 in cellular reprogramming and cancer. Semin Cancer Biol. 2020 December;67(Pt 1):65–73. DOI:10.1016/j.semcancer.2019.08.008
- Whipple C, Young A, Korc MJO. A KrasG12D-driven genetic mouse model of pancreatic cancer requires glypican-1 for efficient proliferation and angiogenesis. Oncogene. 2012;31(20):2535–2544. DOI:10.1038/onc.2011.430.
- Yang H, Lee S, Lee S, et al. Sox17 promotes tumor angiogenesis and destabilizes tumor vessels in mice. J Clin Invest. 2013;123(1):418–431. DOI: 10.1172/JCI64547
- Lee S, Lee S, Yang H, et al. Notch pathway targets proangiogenic regulator Sox17 to restrict angiogenesis. Circ Res. 2014;115(2):215–226. DOI: 10.1161/CIRCRESAHA.115.303142
- Yang J, Ryu J, Kim I, et al. Dll4 blockade promotes angiogenesis in nonhealing wounds of Sox7-deficient mice. Adv Wound Care (New Rochelle). 2020;9(11):591–601. DOI: 10.1089/wound.2019.1015
- González-Hernández S, Gómez M, Sánchez-Cabo F, et al. Sox17 controls emergence and remodeling of nestin-expressing coronary vessels. Circ Res. 2020;127(11):e252–e270. DOI: 10.1161/CIRCRESAHA.120.317121
- Zhou Y, Williams J, Smallwood P, et al. Sox7, Sox17, and Sox18 cooperatively regulate vascular development in the mouse retina. PLoS One. 2015;10(12):e0143650.
- Kim JH, Kim JH, Yu YS, et al. Blockade of angiotensin II attenuates VEGF-mediated blood-retinal barrier breakdown in diabetic retinopathy. J Cereb Blood Flow Metab. 2009 March;29(3):621–628. DOI:10.1038/jcbfm.2008.154
- Guan Z, Fuller BS, Yamamoto T, et al. Pentosan polysulfate treatment preserves renal autoregulation in ANG II-infused hypertensive rats via normalization of P2X1 receptor activation. Am J Physiol Renal Physiol. 2010 May;298(5):F1276–84. DOI:10.1152/ajprenal.00743.2009
- Yue J, Zhao X. GPR174 suppression attenuates retinopathy in angiotensin II (Ang II)-treated mice by reducing inflammation via PI3K/AKT signaling. Biomed Pharmacother. 2020 Feb;122:109701.
- Mak HK, Yung JSY, Weinreb RN, et al. MicroRNA-19a-PTEN axis is involved in the developmental decline of axon regenerative capacity in retinal ganglion cells. Mol Ther Nucleic Acids. 2020 September 4;21:251–263. DOI:10.1016/j.omtn.2020.05.031
- Sun X, Tung W, Zou J, et al. Elasticity of fiber meshes from multiblock copolymers influences endothelial cell behavior. Clin Hemorheol Microcirc. 2020;74(4):405–415. DOI:10.3233/CH-190696
- Fraser-Bell S, Symes R, Vaze A. Hypertensive eye disease: a review. Clin Exp Ophthalmol. 2017 January;45(1):45–53.
- Wang S, Li J, Wang T, et al. Ablation of immunoproteasome β5i subunit suppresses hypertensive retinopathy by blocking ATRAP degradation in mice. Mol Ther. 2020 January 8 28;(1)279–292. 10.1016/j.ymthe.2019.09.025
- Ishii Y, Weinberg K, Oda-Ishii I, et al. Morphogenesis and cytodifferentiation of the avian retinal pigmented epithelium require downregulation of group B1 Sox genes. Development. 2009 August;136(15):2579–2589. DOI:10.1242/dev.031344
- Muto A, Iida A, Satoh S, et al. The group E Sox genes Sox8 and Sox9 are regulated by Notch signaling and are required for Müller glial cell development in mouse retina. Exp Eye Res. 2009;89(4):549–558. DOI: 10.1016/j.exer.2009.05.006
- Jiang Y, Ding Q, Xie X, et al. Transcription factors SOX4 and SOX11 function redundantly to regulate the development of mouse retinal ganglion cells. J Biol Chem. 2013;288(25):18429–18438. DOI: 10.1074/jbc.M113.478503
- Zhu N, Welch C, Wang J, et al. Rare variants in SOX17 are associated with pulmonary arterial hypertension with congenital heart disease. Genome Med. 2018;10(1):56.
- Wang T, Wang S, Xu Y, et al. SOX17 loss-of-function mutation underlying familial pulmonary arterial hypertension. Int Heart J. 2021;62(3):566–574. DOI: 10.1536/ihj.20-711
- Ye X, Wang Y, Cahill H, et al. Norrin, frizzled-4, and Lrp5 signaling in endothelial cells controls a genetic program for retinal vascularization. Cell. 2009;139(2):285–298. DOI: 10.1016/j.cell.2009.07.047
- Han J, Li S, Feng Y, et al. A novel circular RNA (hsa_circ_0059930)-mediated miRNA-mRNA axis in the lipopolysaccharide-induced acute lung injury model of MRC-5 cells. Bioengineered. 2021 December;12(1):1739–1751. DOI:10.1080/21655979.2021.1916276
- Yan F, Wufuer D, Wang J, et al. MicroRNA miR-146a-5p inhibits the inflammatory response and injury of airway epithelial cells via targeting TNF receptor-associated factor 6. Bioengineered. 2021 December;12(1):1916–1926. DOI:10.1080/21655979.2021.1927545
- Martinez B, Peplow PV. MicroRnas as biomarkers of diabetic retinopathy and disease progression. Neural Regen Res. 2019 November;14(11):1858–1869.
- Vishnoi A, Rani S. MiRNA biogenesis and regulation of diseases: an overview. Methods Mol Biol. 2017;1509:1–10.
- Chen X, Ouyang L, Yin Z, et al. Effects of microRNA-29a on retinopathy of prematurity by targeting AGT in a mouse model. Am J Transl Res. 2017;9(2):791–801.
- Yang W, Su M, Yu Y, et al. Dysregulation of miR-637 is involved in the development of retinopathy in hypertension patients and serves a regulatory role in retinol endothelial cell proliferation. Ophthalmic Res. 2021; 10.1159/000514915
- Xiao Y, Duan Y, Wang Y, et al. Resveratrol suppresses malignant progression of oral squamous cell carcinoma cells by inducing the ZNF750/RAC1 signaling pathway. Bioengineered. 2021 December;12(1):2863–2873. DOI:10.1080/21655979.2021.1940616
- Dai YP, Gao XQ. Effects of silencing epididymal vascular endothelial growth factor (VEGF) expression on hyaluronidase (HYD) activity in arsenic poisoning rats through downregulating VEGF receptor 2 (VEGFR2). Bioengineered. 2021 December;12(1):1351–1359.
- Masgutov R, Zeinalova A, Bogov A, et al. Angiogenesis and nerve regeneration induced by local administration of plasmid pBud-coVEGF165-coFGF2 into the intact rat sciatic nerve. Neural Regen Res. 2021 September;16(9):1882–1889. DOI:10.4103/1673-5374.306090
- Asghar MY, Lassila T, Paatero I, et al.Stromal interaction molecule 1 (STIM1) knock down attenuates invasion and proliferation and enhances the expression of thyroid-specific proteins in human follicular thyroid cancer cellsCell Mol Life Sci2021 June 215827–584610.1007/s00018-021-03880-0
- Fu P, Zhu R, Jia J, et al. Aerobic exercise promotes the functions of brown adipose tissue in obese mice via a mechanism involving COX2 in the VEGF signaling pathway. Nutr Metab (Lond). 2021 June 3 18;(1)56. 10.1186/s12986-021-00581-0
- Janani R, Anitha RE, Perumal MK, et al. Astaxanthin mediated regulation of VEGF through HIF1α and XBP1 signaling pathway: an insight from ARPE-19 cell and streptozotocin mediated diabetic rat model. Exp Eye Res. 2021 May;206:108555.
- Liu X, Li J, Xjijoi L, et al. miR-142-5p regulates the progression of diabetic retinopathy by targeting IGF1. Int J Immunopathol Pharmacol. 2020;34:2058738420909041.
- Lu J, Zhang Z, Ma X, et al. Repression of microRNA-21 inhibits retinal vascular endothelial cell growth and angiogenesis via PTEN dependent-PI3K/Akt/VEGF signaling pathway in diabetic retinopathy. Exp Eye Res. 2020;190:107886.
- Feng J, Wen T, Li Z, et al.Cross-Talk between the ER pathway and the lncRNA MAFG-AS1/miR-339-5p/cdk2 axis promotes progression of ER+ breast cancer and confers tamoxifen resistance.Aging (Albany NY). 2020 October 24;12:20658–20683.
- Dinh QN, Drummond GR, Sobey CG, et al. Roles of inflammation, oxidative stress, and vascular dysfunction in hypertension. Biomed Res Int. 2014;2014:406960.
- Touyz RM. Reactive oxygen species and angiotensin II signaling in vascular cells – implications in cardiovascular disease. Braz J Med Biol Res. 2004 August;37(8):1263–1273.
- Karaca M, Coban E, Felek R, et al. The association of oxidative stress with hypertensive retinopathy. Clin Exp Hypertens. 2013;35(1):16–19. DOI:10.3109/10641963.2012.685535
- Merino-Azpitarte M, Lozano E, Perugorria MJ, et al. SOX17 regulates cholangiocyte differentiation and acts as a tumor suppressor in cholangiocarcinoma. J Hepatol. 2017 July;67(1):72–83. DOI:10.1016/j.jhep.2017.02.017
- Zhang Q, Wu X, Yang J. miR-194-5p protects against myocardial ischemia/reperfusion injury via MAPK1/PTEN/AKT pathway. Ann Transl Med. 2021 Apr;9(8):654.
- Ayaz L, Dinç E. Evaluation of microRNA responses in ARPE-19 cells against the oxidative stress. Cutan Ocul Toxicol. 2018 June;37(2):121–126.
- Lou C, Zhao J, Gu Y, et al. LINC01559 accelerates pancreatic cancer ceEvaluation of microRNA responses in ARPE-1ll proliferation and migration through YAP-mediated pathway. J Cell Physiol. 2020 April;235(4):3928–3938. DOI:10.1002/jcp.29288