ABSTRACT
The prostate epithelium is composed of two predominant cell populations: luminal and basal epithelial cells. Luminal cells have a secretory function that supports male fertility while basal cells function in regeneration and maintenance of epithelial tissue. Recent studies in humans and mice have expanded our knowledge of the role and regulation of luminal and basal cells in prostate organogenesis, development, and homeostasis. The insights from healthy prostate biology can inform studies focused on the origins of prostate cancer, progression of the disease, and development of resistance to targeted hormonal therapies. In this review, we discuss a critical role for basal cells in the development and maintenance of healthy prostate tissue. Additionally, we provide evidence supporting a role for basal cells in oncogenesis and therapeutic resistance mechanisms of prostate cancer. Finally, we describe basal cell regulators that may promote lineage plasticity and basal cell identity in prostate cancers that have developed therapeutic resistance. These regulators could serve as therapeutic targets to inhibit or delay resistance and thereby improve outcomes for prostate cancer patients.
Introduction
The human prostate is a glandular organ that sits immediately below the bladder and surrounds the urethra [Citation1]. The two main epithelial cell types that make up the bulk of prostate tissue are luminal and basal epithelial cells (hereafter referred to as luminal and basal cells). Luminal cells produce prostatic secretions to support male fertility, the main function of the prostate, while basal cells provide structural support between luminal layers and basement membrane as well as regenerative potential [Citation2]. The androgen receptor (AR) is a ligand-activated transcription factor that plays key roles in promoting development of the fetal prostate as well as homeostasis of prostate tissue in adults [Citation1,Citation3]. AR is required for survival of luminal cells, while basal cells express very little to no AR and can survive in the absence of AR transcriptional activity [Citation1,Citation2,Citation4]. Importantly, basal cells serve as multipotent progenitors in regeneration of the prostate epithelium [Citation3].
Prostate cancer is the second leading cause of male cancer deaths in the United States [Citation5]. The majority of prostate cancers have a luminal cell identity defined by gene expression programs regulated by active AR [Citation6]. Common transcriptional regulators of the healthy prostate and prostate cancer have been reviewed [Citation7]. While men with localized prostate cancer can typically be cured with surgery or radiation therapy, those with advanced forms of the disease are treated with hormonal therapies that inhibit AR transcriptional activity. Because AR supports proliferation and survival of the prostate luminal cell lineage, these therapies improve patient survival. Unfortunately, men will eventually become resistant to AR-targeted therapies and develop castration-resistant prostate cancer (CRPC, see for all abbreviations used in this review).
Table 1. Abbreviations.
The uncontrolled growth of CRPC resulting from resistance to AR-targeted therapies remains a significant challenge facing the prostate cancer field. Prostate cancer cells can develop resistance to AR-targeted therapies by restoring AR activity through diverse mechanisms including AR gene amplification, mutation, or structural rearrangement, as well as alternative splicing of AR mRNA [Citation8,Citation9]. In CRPC, approximately 87% of tumors display amplification, mutation, and/or structural rearrangement of the AR gene. In contrast, these alterations do not occur in primary prostate cancer [Citation10]. Prostate cancer can also undergo lineage plasticity, defined as the ability to de-differentiate to a stem-like state and/or re-differentiate to an alternative AR-null cell type, to bypass a reliance on AR for growth and survival [Citation11]. AR-independent cells of the prostate, including basal cells, have become cells of interest in enabling therapeutic resistance via lineage plasticity [Citation12]. Studying fetal prostate development, and the role of basal cells in this process, is particularly relevant when considering that prostate cancer progression and therapeutic resistance can occur via a “re-awakening” of developmental programs to promote sustained tumor growth [Citation13].
Here, we review the phenotypes and functions of basal cells in healthy prostate biology including prostate development and regeneration. Although the vast majority of primary prostate cancer presents with an AR-positive luminal identity, we describe evidence supporting that both luminal and basal cells are potential cells of origin for prostate cancer. We also describe cellular markers and gene signatures used to study basal cells, and how prostate cancers at various stages of development and progression display presence or absence of these markers and signatures. Finally, we highlight select regulators of basal cell identity that promote prostate cancer progression and therapeutic resistance. These regulators could represent therapeutic targets to slow or even stop deadly AR-independent forms of prostate cancer from appearing.
The prostate gland
The human prostate is located immediately below the bladder and surrounds the urethra. Unlike the mouse prostate, which has four distinct lobes (ventral, lateral, dorsal, anterior), the human prostate is sub-divided into zones that are contained within a thin fibrous capsule [Citation14]. The three main zones of the human prostate are the central, transition, and peripheral zones. The transition zone encircles the urethra, the central zone is located posterior to the urethra and surrounds the ejaculatory duct, and the peripheral zone is the most posterior part of the prostate, making up the majority (70%) of total prostate mass [Citation2,Citation15].
Prostate epithelial cell types
Prostate epithelial cells are supported by and function alongside several non-epithelial cell types, collectively known as the stroma. Stromal cells include fibroblasts, myofibroblasts, and smooth muscle cells [Citation16]. Signaling between stromal and epithelial cells occurs through several pathways including AR, WNTs, FGFs, BMPs, TGF-β, and others, and is required for normal prostate development, homeostasis, and regeneration [Citation17]. Historically, the human prostatic epithelium has been described as being composed of three main cell types. Luminal and basal epithelial cells make up the bulk of this tissue layer, with rare neuroendocrine cells located throughout [Citation1,Citation13]. These cell types each have distinct morphology, gene expression patterns, and functions. Luminal epithelial cells are terminally differentiated secretory cells that have a columnar shape and line the lumen of the prostate gland [Citation1,Citation2]. These cells express markers specific for the luminal epithelial cell lineage such as cytokeratin (CK) CK8 and CK18, AR, and AR regulated targets like KLK3. Their primary function is to produce proteins found in prostatic secretions required for male fertility. Basal epithelial cells are located between luminal epithelial cells and the surrounding basement membrane and stroma. The architecture of the interface between luminal and basal epithelial cells differs between humans and rodents. For instance, in tissue sections from human prostate, a row of basal cells rests behind a row of luminal cells in a “stratified columnar” organization with a luminal to basal cell ratio of 1:1 [Citation18]. In tissue sections from mice, basal cells have a less organized orientation along luminal cells, as well as a higher ratio of luminal to basal cells of 3:1 [Citation18]. Basal cells have a flattened morphology and express distinguishing cell markers such as CK5, CK14, and TP63 [Citation1,Citation4]. Notably, basal cells often express very low to no AR [Citation1]. Basal cells have high proliferative and regenerative capacity with the potential to differentiate into luminal cells [Citation12,Citation19–21]. An intermediate cell type, sometimes called a “transit amplifying” cell, has also been identified based on co-expression of luminal and basal cell markers in addition to CK19 [Citation6,Citation22].
Dispersed among the luminal and basal epithelial cells are rare neuroendocrine cells, which make up about 1% of the cells of the epithelium [Citation23,Citation24]. These elongated neuroendocrine cells lack AR expression and express neuroendocrine-lineage markers including chromogranin A (CHGA), synaptophysin (SYP), and neuron-specific enolase (NSE) [Citation24]. Neuroendocrine cells are negative for Ki67 staining, indicating they are non-proliferative [Citation24]. Neuroendocrine cells function in the healthy prostate to sense pH and chemical changes, as well as receive stimuli from nerve endings, blood vessels, and smooth muscle [Citation24].
Recent studies using single-cell RNA sequencing (scRNA-seq) have expanded our understanding of the repertoire of epithelial cell types in prostate tissue beyond classic luminal, basal, and neuroendocrine cell definitions. A study using scRNA-seq to characterize the healthy adult mouse prostate identified a single basal cell population, rare neuroendocrine cells, a cell population with mixed luminal and basal characteristics, and a heterogeneous mix of five subpopulations of luminal cells. There were four distinct distal luminal cell populations, each corresponding to lobes of the prostate (Anterior = LumA, Dorsal= LumD, Lateral = LumL, Ventral = LumV) and one proximally enriched luminal population (LumP). They noted the epithelial cell population with mixed luminal and basal marker expression was enriched proximally and within the adjacent rhabdosphincter and urethral junction, and named these cells “Periurethral” or PrU [Citation20]. Using isolated cell populations, they performed an organoid formation assay which showed PrU and basal cells have the highest organoid formation potential, followed by LumP, and finally LumA, LumD, LumL, and LumV epithelial cells which had low organoid forming potential.
Another study performed scRNA-seq on healthy adult human prostate tissue [Citation15]. In addition to identifying luminal, basal, and rare neuroendocrine cells, they observed two additional epithelial populations which were initially labeled Other Epithelial 1 (OE1) and Other Epithelial 2 (OE2). The OE1 and OE2 cells were present proximal to the urethra, and eventually labeled as club and hillock cells based on their gene expression patterns being similar to those in club and hillock cells of the lungs [Citation15]. These prostate club and hillock epithelial cell populations have also been observed in large-scale scRNA-seq and single nucleus RNA-seq studies that analyzed prostate alongside other tissues in the human body [Citation25,Citation26]. The role and origin of club and hillock cells in prostate development and function are unknown, but clues can be obtained from studies of club and hillock cells in the lung [Citation27]. Club cells, marked by expression of SCGB1A1, have a variety of functions in the lung including regeneration of epithelium, secretion of proteins to maintain homeostasis, as well as anti-inflammatory and immunosuppressive functions. Hillock cells, which are marked by CK13 expression, have high turnover in the lung and function in cell adhesion and immunomodulation. Noteworthy, a hierarchy has been observed between basal, club, and hillock cell identities in the lung, with basal progenitor cells having the potential to give rise to club cells, sometimes through an intermediate hillock identity [Citation28]. Benign prostatic hyperplasia (BPH), a common condition in older men resulting from an expansion of the periurethral transition zone, can be treated with 5 alpha-reductase inhibitors (5ARIs). Most luminal cells undergo apoptosis in response to 5ARI treatment, which shrinks the prostate and relieves pressure on the urethra. However, some rare luminal cells have been shown to decrease AR expression and activity, and increase expression of club cell specific genes including LTF, PIGR, OLFM4, SCGB1A1, and SCGB3A1 to survive the treatment [Citation29]. This suggests a lineage plasticity mechanism by which luminal cells can transition to a club identity in response to an AR-targeted therapy. In mice, periurethral luminal cells that extend into the proximal prostate have been shown to be castration insensitive [Citation20]. These castration insensitive cells express markers of human prostate club and hillock cells and are enriched in atrophic regions following 5ARI treatment [Citation30].
Development of the human prostate
The foundation of our knowledge on human prostate development has been established in large part by studies performed in mice and rats. While the gross anatomy of human and rodent prostates differs significantly [Citation31], the general stepwise process of prostate development is very similar.
Fetal development of the human prostate can be divided into four stages: (1) pre-bud, (2) initial budding, (3) bud elongation and branching, and (4) ductal canalization and epithelial differentiation [Citation1] (). During the pre-bud phase, the AR plays a central role in sexual dimorphism and commitment to male-specific organogenesis. Male embryos have higher circulating testosterone levels than female embryos, which sets a cascade toward prostate development [Citation32]. During initial budding, paracrine signaling involving AR initiates buds to form from the urogenital sinus epithelium (UGE) into the urogenital sinus mesenchyme (UGM) [Citation2]. Two hypotheses, the andromedin model and the smooth muscle model, have been proposed for how androgens mediate prostate epithelial induction and budding [Citation2]. During bud elongation and branching, epithelial proliferation drives the newly emergent prostatic buds into the surrounding UGM. This is supported by studies showing that the tips of extending epithelial cords have significantly higher Ki67 staining and DNA synthesis than the canalized ducts closer to the prostatic urethra [Citation1,Citation33]. The buds extend and branch for several weeks, forming solid cords. Eventually, these solid cords will canalize into glandular structures via apoptosis and epithelial differentiation into luminal, basal, and neuroendocrine cell fates [Citation2,Citation34]. Expression of general epithelial, luminal-specific, and basal-specific cell markers are dynamic throughout prostate development and at the canalized-solid interface [Citation1]. Luminal markers CK7, CK8, and CK19 are present throughout prostate development from pre-bud through branching. These markers are present in central cells of solid cords, but lost in basal cells after epithelial differentiation. The basal cell marker TP63 is also highly expressed throughout development and is lost in luminal cells after canalization and differentiation. TP63 has been shown to be required for prostate formation, as TP63-null mice do not develop prostates [Citation35]. Interestingly, AR is absent in solid epithelial cords, only to emerge after canalization and differentiation [Citation1].
Figure 1. Fetal development of the prostate and the cellular composition of prostate epithelial tissue. (a) Development of the prostate can be divided into four stages. AR signaling causes buds to form from the urogenital sinus epithelium, which branch and extend into the urogenital sinus mesenchyme as solid cords. Eventually, the solid cords will canalize and the cells will differentiate into various epithelial cell types. (b) the epithelial cellular environment differs along the proximal-distal axis of the prostate. The proximal branch of the prostate is dominated by progenitor cell populations as well as club and hillock prostatic cells. Proximal multipotent basal progenitor cells give rise to bipotent basal cells which function to repopulate luminal and basal cell populations toward the distal ends of branches.
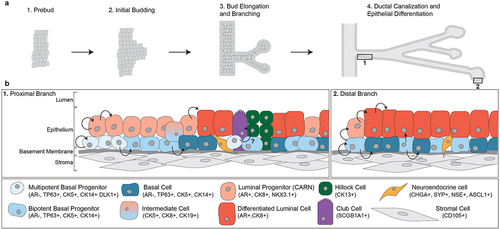
Lineage tracing studies in mice have enabled investigation of the extent to which basal cells can serve as progenitors for basal, luminal, and neuroendocrine cells [Citation3,Citation36]. Most lineage tracing experiments have used cell type-restricted promoters to drive expression of a fusion protein consisting of inducible Cre recombinase, along with a conditional allele to allow irreversible fluorescence labeling of cells with Cre activity. Cells descending from the original labeled cell retain the fluorescence label, which enables characterization of the various cellular phenotypes that can arise from that original cell. For instance, one study developed transgenic mice for doxycycline-inducible labeling of basal cells (CK14+) with yellow fluorescence protein (YFP). In these mice, YFP-labeled basal cells could differentiate into luminal progenitor (NKX3.1+) and terminally differentiated luminal cells (AR+) [Citation3]. Basal cells even differentiated into neuroendocrine cells as evidenced by 25% of neuroendocrine cells being labeled with YFP [Citation3]. To identify whether there were multipotent and unipotent progenitor subpopulations of basal epithelial cells, the dose of doxycycline was lowered to label fewer CK14-positive basal cells. This provided evidence that most basal epithelial cells (67%) completely differentiated into luminal cells, without any remaining basal cells nearby. Additionally, 10% of marked basal cells were classified as bipotent, with luminal and basal cells generated from the basal progenitor. About 23% of the cells were purely basal, suggesting a unipotent basal progenitor had been marked [Citation3]. This study also gave insight into the cell fate of intermediate cells (CK5+, CK8+). Intermediate cells were not found in unipotent basal cell populations, but were present in 25% of bipotent cell populations, suggesting they are biased toward a luminal differentiation rather than committed to basal cell differentiation. Importantly, labeling of luminal cells (CK8+) with YFP resulted in 0% of basal (CK5+) and neuroendocrine cells being labeled with YFP, suggesting differentiated luminal cells could not generate cells of basal or neuroendocrine lineage. A separate study performing lineage-tracing of basal cells expressing the ΔNp63 isoform of TP63 came to a similar conclusion, namely, that basal cells in the developing prostate can give rise to luminal, basal, and neuroendocrine cell lineages [Citation36].
Adult prostate homeostasis
Once the human prostate has developed, it is a relatively quiescent organ with slow epithelial turnover. Lineage tracing studies in mice have shown that epithelial homeostasis is maintained by slow expansion of lineage restricted luminal (CK8+, CK18+) and basal (CK5+) cells [Citation3,Citation37]. The stem cell activities of basal cells become more restricted, but a small portion of basal cells retain bipotency and occasionally differentiate to luminal cells to support prostate homeostasis [Citation12,Citation38]. Importantly, tissue damage can dysregulate this balance of slow turnover and lineage restriction. In response to inflammation, the differentiation program of basal cells can be altered to induce luminal differentiation [Citation39]. Similarly, apoptosis of luminal cells due to anoikis can also trigger basal to luminal differentiation for tissue repair [Citation40].
One study used quantitative clonal mapping and three-dimensional reconstruction to track the origin of stem cells and their progeny in the adult human prostate. Using histocytochemical cytochrome c oxidase (CCO) deficiency as a reporter of mitochondrial DNA mutations, this study labeled and traced the lineage of stem cells in the prostate [Citation19]. Grouping glandular subunits into branches that were proximal or distal to the prostatic urethra revealed patterns of lineage potential, proliferation, apoptosis, and protein expression. The majority of basal cells in the most proximal ducts displayed multipotency and gave rise to bipotent basal progenitors. The multipotent basal progenitors in the proximal trunk never gave rise directly to luminal cells in distal end of branches. Rather, the basal bipotent progenitor cells gave rise to luminal cells that repopulated the luminal epithelium more commonly toward the distal end of branches. When tracking proliferative activity by staining with Ki67, proliferation was observed in the proximal branches and was restricted mostly to basal cells. Ki67 staining intensity of the basal cells weakened toward the distal regions of the branches. Conversely, staining for activated caspase 3, a marker of apoptotic cells, steadily increased along the proximal to distal axis and was largely restricted to luminal cells. The prostate stem-cell marker DLK1 was found to mark basal cells with potential to produce fully differentiated 3D spheroids in vitro. For instance, basal cells lacking DLK1 expression could not be grown as spheroids past 6–8 weeks, whereas basal cells expressing DLK1 could be grown as spheroids beyond 8 weeks, and the resulting spheroids had differentiated epithelial layers composed of basal (CK5+) and luminal (PSA+, AR+, CK8+) cell populations. Overall, this study established the idea of a uni-directional stream of cells that repopulate and maintain branches of the human prostate. DLK1+ multipotent basal cells give rise to bipotent basal cells, which extend through the branch to replenish luminal cells that are lost to apoptosis ().
Another study supported the concept that progenitor cells proximal to the prostatic urethra have increased potential for prostate regeneration. To analyze progenitor potential of various mouse prostate cell populations, in vivo tissue recombination assays were performed wherein dissociated epithelial cells were mixed with rat embryonic urogenital mesenchyme before renal grafting. This study found that grafts generated using distal luminal cells required significantly higher number of input cells to form (30,000), and lacked a full repertoire of differentiated prostate cell types. Conversely, grafts generated using proximal cells could be established from as few as 1,000 input cells, and contained multiple cell populations including basal and luminal cells [Citation20]. A similar study was performed wherein human luminal and basal prostate epithelial cells were isolated and tested for organoid forming potential from a single cell. Organoids formed from individual basal cells with much higher efficiency (70%) than individual luminal cells (1–2%) [Citation21]. While the majority of organoids derived from individual luminal cells were AR+ and CK8+, they did observe some cells that were CK5+ and CK8–. This supports the existence of rare multipotent luminal progenitor cells that can give rise to basal cells.
Regeneration of the prostate
Androgen deprivation via castration causes regression of the prostate, mostly due to apoptosis of luminal cells [Citation41]. Conversely, basal cells express very little to no AR and are largely unaffected by castration. Re-administration of androgens promotes prostate regeneration to normal size. This process of prostate regression/regeneration can be achieved over multiple cycles of androgen deprivation/replenishment [Citation42]. This indicates the presence of castration-resistant stem cells. Lineage tracing studies have shown that repopulation of luminal cells upon androgen re-administration after castration is primarily due to proliferation of luminal cells that survived castration [Citation43], with some basal stem cell populations contributing as well [Citation12]. One study that tracked basal to luminal differentiation during multiple rounds of regression and regeneration found that just 0.6% of basal cells differentiated to luminal cells after three rounds of regression and regeneration, which increased to 3.4% of cells after five rounds of regression and regeneration [Citation12]. This suggests a key role for basal to luminal differentiation to repopulate epithelial tissue lost due to castration. This study also tracked YFP+ basal cell differentiation to luminal cells during normal aging of the prostate, and found bipotent basal cells differentiate to luminal cells at similar rates in the context of healthy tissue turnover as observed when androgens are re-administered post-castration [Citation12].
Although TP63−/− mice do not develop prostates, a requirement for TP63+ basal cells can be studied by grafting TP63−/− urogenital sinus (UGS) tissue under the renal capsule of nude mice, which develop ductal structures resembling a prostate [Citation4]. These structures completely lacked basal cells, but were still able to form ducts in the presence of androgens. In response to castration, the sub-renal TP63−/− UGS grafts had severe reduction in ductal structures and luminal cell numbers. However, after one month of testosterone treatment, the sub-renal TP63−/− UGS grafts were able to regenerate, suggesting basal cells are not absolutely required for prostate regeneration. This can be explained by the presence of a luminal progenitor cell population that has been named CARNs by virtue of displaying a castration-resistant phenotype and being marked by expression of Nkx3.1 [Citation44]. The Nkx3.1 homeobox protein regulates epithelial differentiation and marks luminal stem cells with bipotent potential [Citation45]. In a lineage tracing study, about 3% of YFP-marked CARNs transformed into CK14+/CK5+/TP63+ basal cells during prostate regeneration, supporting bipotency of these luminal progenitors [Citation44]. In another study, luminal progenitor cells marked by Sca-1+, CD133+, CD44+, CD49F+, and CD117+ were shown to survive castration and assist in regeneration [Citation46]. Surprisingly, the luminal progenitor cells that display these markers maintain AR expression but are resistant to castration.
In a study that investigated the effects of AR deletion in basal cells, no significant change was found in apoptosis or proliferation rates between AR+ and AR- basal cells, suggesting AR is not needed for homeostatic maintenance of basal cells [Citation41]. However, AR was found to be required for basal cell biopotency, as evidenced by AR- basal cells having reduced ability to differentiate into luminal cells [Citation41]. In organoid forming assays, AR basal cells yielded structures that lacked hollow lumens and had minimal CK18+ staining, indicating lack of basal-to-luminal differentiation. Noteworthy, loss of the tumor suppressor Pten in basal cells caused excess basal-to-luminal differentiation regardless of AR status [Citation38,Citation41]. This suggests Pten loss overrides a requirement for AR expression in basal cells to differentiate to a luminal phenotype.
Prostate cancer
Prostate cancer is the most commonly diagnosed non-cutaneous cancer in males in the United States [Citation5]. The majority of prostate cancers originate in the peripheral zone of the prostate and present with an adenocarcinoma phenotype, marked by AR and CK8 staining. This would support a luminal cell type as a cell of origin, but studies over the past several years have implicated almost every epithelial cell type, including luminal, basal, intermediate, progenitor, and rarely neuroendocrine cells as potential sources of prostate cancer initiation. Understanding the cell of origin for prostate cancer development in individual patients has potential to increase accuracy of prognosis and improve prediction of treatment responses.
Cell of origin and primary prostate cancer
Luminal cells have historically been thought of as the cell of origin for prostate cancer since diagnosis of the disease by pathologists is facilitated by negative staining of basal cell markers in tissue specimens [Citation47]. Studies have used two main methods to determine whether luminal and/or basal cells have potential for oncogenic transformation to initiate prostate cancer. One method involves lineage tracing in transgenic mice to irreversibly label cell types of interest, and then track the phenotypes that emerge in the labeled cell populations. The other method involves isolating specific cell types of interest from benign prostate tissue, transforming these cells in vitro with oncogenes, and then studying the cancer phenotypes that emerge.
Many lineage tracing experiments for prostate cancer have used cell type restricted promoters to drive expression of a fusion protein consisting of Cre recombinase and a mutant estrogen receptor (ER) ligand binding domain (CreERT2). CreERT2 is inactive in cells unless stimulated with the ER ligand tamoxifen. The timing of tamoxifen administration allows control over initiation of Cre recombinase activity, and the level/duration of tamoxifen dosing allows for control over the fraction of target cells in which CreERT2 activity is induced. Breeding CreERT2 mice with mice harboring conditional alleles for expression of a fluorescence marker such as YFP and deletion of a tumor suppressor such as Pten allows simultaneous transformation and fluorescence marking of individual cells for tracing them and their progeny. PTEN is one of the most commonly altered genes in prostate cancer. About 17% of primary prostate cancer displays mutation or homozygous deletion of PTEN, which increases to over 40% in metastatic CRPC [Citation48]. Commonly used Cre recombinase models have been CK5- or TP63-CreERT2 for studying basal cells, and CK8- or NKX3.1-CreERT2 for studying luminal cells. In the weeks to months following Cre recombination, fluorescence microscopy can be used to observe the extent of prostatic intraepithelial neoplasia (PIN, a premalignant precursor to prostate cancer) arising from single transformed cells, and eventual prostate cancer development.
One group deleted Pten in CARNs by using NKX3.1 to drive CreERT2 recombination in mice with floxed Pten. They observed rapid development of high-grade PIN and microinvasive carcinoma in mice with Pten deleted, whereas mice with wildtype Pten were phenotypically normal [Citation44]. Notably, the PIN and carcinoma that arose was negative for TP63 staining, indicating a lack of basal cells in those regions. Pten loss in CARNs has also been shown to override a requirement for AR to transform into luminal-like cancer [Citation41]. These results support CARNs as a cell of origin that can give rise to luminal-like carcinoma.
The CK5-CreERT2 driver has been used to produce labeled basal cells with Pten deleted as a platform for studying the potential of basal cells as a cell of origin for prostate cancer [Citation12]. Pten deletion in basal cells generated PIN lesions and culminated in the formation of carcinoma at six months. Interestingly, the PIN lesions and tumors derived from Pten-null basal cells presented as AR+/CK18+ luminal cells. This suggests basal cells can give rise to high grade PIN and prostate cancer, but first differentiate to a luminal cell identity. Tumors derived from Pten-null basal cells arose more slowly than tumors derived from Pten-null luminal cells, which was attributed to the time required for the transformed basal cells to differentiate to a luminal identity. Another group found that the tumors derived from Pten-null basal cells were more proliferative as measured by BrdU incorporation, and displayed greater invasion into surrounding stroma compared to tumors from Pten-null luminal cells with Cre driven by CK8 [Citation38]. Interestingly, basal cells with Pten and Ar co-deleted still readily differentiated into CK18+ luminal cells, suggesting Pten deletion overrides a need for AR in development of a luminal cell identity [Citation41]. Another study using lineage tracing in NP [Citation49], Hi-Myc [Citation50], and TRAMP [Citation51] mouse models of prostate cancer also found that luminal cells were favored as the cell of origin compared to basal cells based on CK8- vs CK5-YFP positivity ratios in carcinoma lesions [Citation52]. They did note that CK5+ basal cells isolated from the Hi-Myc model could form luminal-like tumors in sub-renal grafting assays, indicating basal to luminal differentiation had taken place [Citation52].
It is important to contrast these findings from lineage tracing studies in mice with findings from studies testing transformation potential of specific prostate cell types isolated from benign human prostate tissue. In one study, expression of AKT, ERG, and AR in basal cells produced tumors that resembled adenocarcinoma, while expression of these genes in luminal cells did not produce tumors as efficiently [Citation53]. The transformed basal cells did lose expression of some basal-specific markers, and also generated tumors with defined glands and tubules. This group concluded that histological classification of cancer does not consistently align with the cell type from which it originated. This study also supported the observation that basal cells with PTEN loss have higher frequency of luminal differentiation [Citation12].
Another study by this group found that both luminal and basal cells isolated from benign human prostate tissue can produce cancer given certain oncogenic stimuli, albeit with different degrees of aggressiveness [Citation54]. For instance, lentivirus transduction of human prostate luminal (CD26+) or basal (CD49fhi) cells with c-MYC and activated AKT1 produced cells that were able to form organoids in vitro. However, the organoid forming potential of transformed basal cells was 50-fold higher than transformed luminal cells. When these organoids derived from transformed basal cells were implanted in mice as xenografts, they formed tumors rapidly that displayed a poorly differentiated histology. These poorly differentiated tumors expressed CK8, lacked TP63 and CK5, and had low/absent AR and PSA. Conversely, luminal cell xenografts grew slower, had more defined glandular structure, and displayed strong staining of AR and PSA [Citation54]. This strong AR and PSA staining aligns more closely with what is observed in primary prostate cancer and the majority of metastatic CRPC. Conversely, low/absent AR and PSA staining in tumors derived from transformed basal cells mimics phenotypes observed in certain subtypes of metastatic CRPC that develop lineage plasticity. The presence of basal cell-like phenotypes that emerge in metastatic CRPC is expanded in more detail below.
Overall, the results from cell-of-origin studies can differ based on organism, assay, and oncogenic driver manipulations. Lineage tracing studies in mice support the concept that both luminal and basal cells can give rise to high-grade PIN and luminal-like adenocarcinoma after Pten deletion. In these studies, luminal cells give rise to carcinoma more quickly than basal cells, possibly due to a delay in the time it takes basal cells to transition to a luminal cell after Pten deletion. However, once transitioned, tumors derived from basal cells have high proliferation rates and invasiveness. Oncogenic transformation of luminal and basal cells isolated from benign human tissue support the concept that both cell types can give rise to tumors and organoids. However, the tumor- and organoid-forming potential of transformed basal cells is much higher than the tumor- and organoid-forming potential of transformed luminal cells. Further, the tumors and organoids derived from basal cells are less differentiated and more aggressive. The results from in vitro oncogenic transformation assays may be harder to interpret since basal cells inherently produce organoids more easily than luminal cells [Citation21]. Regardless, it is becoming more widely accepted that both luminal and basal cells have potential to serve as a cell of origin for prostate cancer, which could have important ramifications for clinical course of the disease. However, it is challenging to determine the cell of origin from which individual patients’ tumors arise because the epithelial cell identity of the prostate cancer does not correlate with epithelial cell type of origin.
To address these considerations, a study applied the PAM50 gene expression classifier, which is used to categorize breast cancers into luminal A, luminal B, HER2, and basal subtypes, to subtype primary prostate cancer [Citation55,Citation56]. After excluding the HER2 subtype given the lack of HER2/ERBB2 amplification across the samples, they were able to successfully separate 3782 primary prostate cancers from retrospective and prospective studies into either luminal A, luminal B, or basal subtypes. The results from the retrospective study support that a majority of localized prostate cancer had a luminal phenotype (34.3% luminal A, 25.8% luminal B), but a considerable fraction were classified as basal-like (37.1%) [Citation56]. It is tempting to speculate that these gene expression-based subtypes could be reflective of the cell of origin of these tumors.
While the majority of localized prostate cancers are adenocarcinoma, certain cases of localized prostate cancer present with basal cell histopathological features. Some groups have defined a subset of prostate cancer aberrantly expressing TP63. This subtype is rare, accounting for less that 1% of primary prostate cancer [Citation57]. These tumors have an intermediate phenotype including expression of AR, PSA, luminal marker CK8/18, and basal marker TP63 [Citation57]. However, they have weak expression of basal marker CK5 and also lack ERG gene rearrangements commonly found in luminal-like adenocarcinomas [Citation57]. Gene expression analysis found that ETS2 is more highly expressed in TP63+ prostate tumors compared to the more common adenocarcinoma tumors [Citation58]. While they often have higher Gleason score due to their poorly defined glandular structures, TP63+ primary tumors are relatively indolent [Citation58].
A second basal-like subset of primary carcinoma has also been described, commonly referred to as “basal cell carcinoma of the prostate” [Citation59,Citation60]. Also extremely rare, these tumors can be more aggressive and have unique features distinguishing them from traditional prostate adenocarcinoma. Basal cell carcinoma of the prostate lacks TMPRSS2-ERG fusions, has low to no AR staining, and is uniformly negative for NEPC markers like SYP and CHGA [Citation59]. The most consistent abnormalities present are loss of PTEN (56%) and overexpression of EGFR (67%).
Castration-resistant prostate cancer
Given the majority of prostate cancer presents as AR+ adenocarcinoma, AR-targeted therapies are an effective method to slow progression of locally advanced or metastatic disease. Accordingly, men whose cancer cannot be cured with surgery or radiation will often be started on a treatment regimen of androgen deprivation therapy (ADT) with or without second-generation AR antagonists like enzalutamide [Citation61], apalutamide [Citation62], and darolutamide [Citation63]. Because prostate cancer relies on AR transcriptional activity for survival, these therapies are initially effective. However, men will become resistant to these therapies and progress to castration resistant prostate cancer (CRPC).
Progression to CRPC marks a shift in disease presentation and outcomes, with advanced CRPC becoming remarkably heterogenous (). About 70–75% of CRPC tumors retain adenocarcinoma histology, remain positive for AR expression, and restore AR transcriptional activity during treatment with AR-targeted therapies through various mechanisms including amplification, mutation, or structural rearrangement of the AR gene, or alternative splicing of AR mRNA [Citation8,Citation9]. Interestingly, about 25–30% of CRPC tumors can bypass a reliance on AR, sometimes losing expression of AR completely. These CRPC tumors that lack AR likely arise because AR targeted therapies can promote lineage plasticity, which is a biological process that occurs during development and also in survival responses to stress [Citation64,Citation65]. Many cancer types display lineage plasticity in response to targeted therapies, wherein they de-differentiate to a stem cell-like state and then re-differentiate to another cell lineage that is not reliant on the molecule being targeted [Citation11]. Sometimes prostate cancer cells will adopt a neuroendocrine cell lineage, defined as neuroendocrine prostate cancer (NEPC). Additionally, these lineage plastic phenotypes brought about by targeted therapy can mimic signatures of basal cells.
Figure 2. Prostate cancer initiation and progression. Several epithelial cell types, including luminal, basal, and neuroendocrine cells, have been suggested as potential cells of origin for prostate cancer. However, the majority of primary prostate cancer has a luminal-like adenocarcinoma phenotype, defined by CK8 and AR expression. Treatment with AR-targeted therapies like ADT and enzalutamide can be initially effective, but invariably bring about heterogenous CRPC subtypes. These include luminal-like CRPC with restored AR signaling, lineage plastic CRPC with reduced or lost AR activity and increases in basal cell phenotypes, and NEPC with complete loss of AR and gain of NEPC markers CHGA, SYP, and NSE.
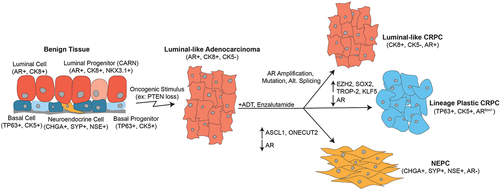
Application of the PAM50 gene expression classifier to 634 metastatic CRPC samples demonstrated that 45% of CRPC could be classified as luminal and 55% could be classified as basal. This indicates that a non-luminal gene expression program may be more frequent in CRPC than would otherwise be apparent from reduced/lost AR expression and activity, or presence of defined phenotypes such as NEPC. Interestingly, 90% of CRPC samples originally defined as NEPC fell into the PAM50 basal subtype. As expected, the PAM50 approach revealed that the luminal and basal classifications of CRPC tumors occur along a spectrum. Interestingly, the NEPC samples represented the most basal-like across this spectrum. This study also found that men with basal-like CRPC had poorer outcomes and were less likely to benefit from AR-targeted therapy [Citation66].
Combined loss of tumor suppressors TP53 and RB1 has been shown to exacerbate the occurrence of lineage plasticity in CRPC [Citation67–69]. TP53 mutations or homozygous deletions occur in about 8% of localized prostate cancer, which increases to approximately 50% in metastatic CRPC [Citation48]. About 1% of localized prostate cancers have an RB1 mutation or homozygous deletion, which increases to about 9% in metastatic CRPC [Citation48]. Co-occurrence of TP53/RB1 alterations has been noted to occur more frequently in treatment-resistant CRPC, and commonly presents with a neuroendocrine phenotype [Citation68]. Additionally, knock down of both TP53 and RB1 downregulated luminal markers (AR, CK8, CK18), and increased basal (CK5, CK14, TP63) and neuroendocrine (SYP, CHGA) markers [Citation68]. These cell identity changes were concurrent with increased resistance to enzalutamide.
Several groups have evaluated gene expression in CRPC specimens, which has shown that expression of genes defining basal cell identity is enriched in tumors that have developed resistance to AR-targeted therapies. For instance, one group developed a 91-gene signature from a CD49fhi basal cell population and found this signature was enriched in metastatic CRPC compared to localized prostate cancer [Citation70]. The group also found this signature was enriched in small cell NEPC compared to localized prostate cancer, which supports the concept that a basal phenotype develops during prostate cancer progression to NEPC. The expression of TP63, in particular the ΔNp63α isoform, has been shown to promote adhesion and stem cell-like growth of prostate cancer bone metastases [Citation71], although more recent studies have shown these ΔNp63α+ cells are rarely observed in clinical prostate cancer specimens [Citation72]. Another group found that a signature derived from healthy human basal cells was enriched in high Gleason score samples compared to those with lower Gleason scores. Conversely, a luminal cell signature displayed the opposite pattern of enrichment in samples with lower versus higher Gleason scores [Citation73]. Further, using matched patient samples obtained before and after ADT, they found that luminal signatures were enriched in samples prior to ADT, but basal cell signatures were enriched in samples post-ADT. Consistent with this finding, a basal cell identity was found to emerge transiently during castration-induced progression of a luminal adenocarcinoma patient-derived xenograft (PDX) to a neuroendocrine CRPC phenotype [Citation74]. Collectively, these findings support the hypothesis that AR-targeted therapies promote basal like phenotypes in CRPC, which may precede lineage transition to overt NEPC.
By integrating chromatin accessibility (Assay for Transposase Accessible Chromatin, ATAC-seq) and gene expression (RNA-seq) data from patient-derived organoids, CRPC cell lines, and CRPC PDX models, one group identified a stem-cell-like subtype of CRPC [Citation75]. This subtype displayed significant enrichment for basal cell and prostate basal stem-cell signatures [Citation68,Citation70] and was associated with short durations of response to treatment with abiraterone or enzalutamide. AP-1 transcription factors including FOSL1, FOSL2, FOS, and JUNB were shown to have a significant role in regulating this stem-cell-like subtype of CRPC. Functionally, overexpression of FOSL1 was sufficient to drive this stem-cell-like phenotype in the adenocarcinoma-like LNCaP cell line. This study further supports the notion that prostate cancer cells can adopt stem-cell-like and basal-cell properties in response to AR-targeted therapies, which allows them to progress to AR-independent CRPC. Collectively, these observations indicate that signaling pathways and transcriptional programs regulating basal cell function or identity may play a role in CRPC cell survival in response to AR-targeted therapy.
Therapeutic potential of targeting mediators of basal cell phenotypes
The increased incidence of cells with a basal identity in CRPC tumors supports a need for development of alternative therapies targeted to basal cell regulators. One of these targets is SOX2, supported by the finding that SOX2 levels are higher in lineage plastic CRPC tumors with TP53/RB1 loss [Citation68]. Functionally, knock-down of SOX2 in models harboring TP53/RB1 loss reduced expression of basal cell markers and sensitized cells to treatment with enzalutamide [Citation68]. These findings support a key role for SOX2 in supporting these phenotypes. SOX2 expression is repressed by androgens, supporting the hypothesis that AR-targeted therapies can induce AR-repressed genes to bring about cell identity changes [Citation76]. When SOX2 is induced, it has transcriptional repression functions that require the histone demethylase LSD1 to downregulate genes that support a luminal adenocarcinoma cell identity [Citation77]. These multiple functions of SOX2 combine to produce oncogenic phenotypes like increased cell growth and invasion in models of CRPC [Citation78]. The utility of targeting SOX2 is evident in vivo as well, as SOX2 silencing has been shown to restore enzalutamide sensitivity in mouse xenografts of TP53/RB1-null prostate cancer cells [Citation68]. Several drugs targeting LSD1, including INCB059872, have been developed and are currently in clinical trials for advanced and metastatic cancers (NCT02959437) [Citation79]. SP2509, another drug targeting LSD1, has shown efficacy in slowing growth of prostate cancer cell lines grown as xenograft tumors [Citation80]. Inhibiting SOX2-mediated lineage plasticity by targeting LSD1 remains a promising therapeutic avenue to prevent AR-independent CRPC from emerging.
The histone methyltransferase EZH2 has also been implicated in driving prostate cancer lineage plasticity in tumors harboring TP53/RB1 loss [Citation67]. EZH2 is known to repress genes that determine lineage, thereby promoting a stem-cell-like state [Citation81]. EZH2 interacts with N-Myc to achieve these repressive functions [Citation82]. This repressive action of EZH2 on genes regulating differentiation likely plays an important role in the ability of prostate cancer cells to develop resistance to targeted therapies, supported by the fact that EZH2 is upregulated as prostate cancer progresses on therapy [Citation83]. Two EZH2 inhibitors, GSK126 and EPZ6438/tazemetostat, showed efficacy in re-sensitizing CPRC cell lines with loss of PTEN, TP53, and RB1 to enzalutamide [Citation67]. These promising results have led to a clinical trial of EPZ6438/tazemetostat in CRPC, which is currently underway (NCT04179864) [Citation84].
TROP-2, a cell membrane spanning glycoprotein, has been shown to correlate with SOX2 and EZH2 expression [Citation85]. The levels of TROP-2 are higher in prostate cancer compared to healthy prostate tissue, and increase further in metastatic CRPC, making it an intriguing biomarker and therapeutic target [Citation85]. Roles for TROP-2 in regulating a variety of oncogenic phenotypes have been demonstrated, including cancer proliferation, metastasis, as well as cell identity. For instance, overexpression of TROP-2 promotes resistance to AR-targeted therapy, which is associated with reduced levels of luminal cell markers (AR and AR target genes) and increased levels of basal-cell markers like TP63. An antibody drug conjugate treatment, Sacituzumab Govitecan, has been developed to target TROP-2 expressing cells [Citation86]. Sacituzumab Govitecan has been approved for use in triple-negative breast cancer and advanced bladder cancer, with clinical trials currently underway in CRPC (NCT03725761) [Citation87].
Our lab identified a role for the stem-cell transcription factor KLF5 in regulating the balance between luminal and basal identity in CRPC [Citation74]. In normal prostate, AR is restricted to luminal cells and KLF5 is restricted to basal cells. In a subset of CRPC, both transcription factors can be co-expressed. In this context, KLF5 and AR physically interact on chromatin and regulate opposing transcriptional programs with KLF5 promoting a basal cell identity and AR promoting a luminal cell identity [Citation74]. There are currently no FDA approved drugs targeting KLF5. However, ERBB2 was identified as a target of AR/KLF5 transcriptional opposition that was activated by KLF5 but repressed by AR. Importantly, the ERBB2-specific inhibitor mubritinib, as well as the dual EGFR/ERBB2 inhibitor lapatinib, were both effective in reducing colony formation and migration driven by KLF5 [Citation74]. Additional studies have shown that lapatinib synergizes with enzalutamide in inhibiting growth of prostate cancer cell lines [Citation88]. Another dual EGFR/ERBB2 inhibitor, neratinib, is effective in slowing in vitro cell proliferation and in vivo tumor growth in models classified as mesenchymal and stem like [Citation89].
Conclusion
Basal cells play a critical role in the development and maintenance of the human prostate. Lineage tracing studies have shown that basal cells can serve as progenitors for development of all other epithelial cell types in the prostate. This function is especially important in replenishing luminal cells lost due to apoptosis in homeostatic turnover as well as in response to inflammation and androgen depletion. While AR is not necessary for survival of basal cells, it is required for basal cells to properly differentiate to a luminal identity. However, PTEN loss overrides this requirement for AR in luminal differentiation. Using lineage tracing and in vitro oncogenic transformation assays, luminal and basal cells have both been shown to serve as a cell of origin for prostate cancer, which more commonly presents with an AR+ CK8+ luminal identity. Whether cancer derived from luminal or basal cells is more aggressive can vary depending on the assay and oncogenic stimulus used. Prostate cancer cells can exploit lineage plasticity as a mechanism of resistance to AR-targeted therapies, by de-differentiating to cell identities that display basal cell markers and gene expression signatures. Several factors including SOX2, EZH2, TROP-2, and KLF5 have been shown to mediate this transition. Future studies should continue to elucidate these mechanisms by which basal cell identities mediate therapeutic resistance, with the goal of preventing or delaying cell identity changes. This could provide a way to enhance the efficacy and durability of the current arsenal of AR-targeted therapies for prostate cancer.
Disclosure statement
No potential conflict of interest was reported by the author(s).
Data availability statement
Data sharing is not applicable to this article as no new data were created or analyzed in this study.
Additional information
Funding
References
- Cunha GR, Vezina CM, Isaacson D, et al. Development of the human prostate. Differentiation. 2018 SepOct;103:24–45.
- Toivanen R, Shen MM. Prostate organogenesis: tissue induction, hormonal regulation and cell type specification. Development. 2017 Apr 15;144(8):1382–1398.
- Ousset M, Van Keymeulen A, Bouvencourt G, et al. Multipotent and unipotent progenitors contribute to prostate postnatal development. Nat Cell Biol. 2012 Nov;14(11):1131–1138.
- Kurita T, Medina RT, Mills AA, et al. Role of p63 and basal cells in the prostate. Development. 2004 Oct;131(20):4955–4964.
- Siegel RL, Miller KD, Fuchs HE, et al. Cancer statistics, 2022. CA Cancer J Clin. 2022 Jan;72(1):7–33.
- Strand DW, Goldstein AS. The many ways to make a luminal cell and a prostate cancer cell. Endocr Relat Cancer. 2015 Dec;22(6):T187–97.
- Labbe DP, Brown M. Transcriptional regulation in prostate cancer. Cold Spring Harb Perspect Med. 2018 Nov 1;8(11):a030437.
- Westaby D, Fenor de La Maza MLD, Paschalis A, et al. A new old target: androgen receptor signaling and advanced prostate cancer. Annu Rev Pharmacol Toxicol. 2022 Jan 6;62:131–153.
- Desai K, McManus JM, Sharifi N. Hormonal therapy for prostate cancer. Endocr Rev. 2021 May 25;42(3):354–373.
- Li Y, Yang R, Henzler CM, et al. Diverse AR gene rearrangements mediate resistance to androgen receptor inhibitors in metastatic prostate cancer. Clin Cancer Res. 2020 Apr 15;26(8):1965–1976.
- Boumahdi S, de Sauvage FJ. The great escape: tumour cell plasticity in resistance to targeted therapy. Nat Rev Drug Discov. 2020 Jan;19(1):39–56.
- Wang ZA, Mitrofanova A, Bergren SK, et al. Lineage analysis of basal epithelial cells reveals their unexpected plasticity and supports a cell-of-origin model for prostate cancer heterogeneity. Nat Cell Biol. 2013 Mar;15(3):274–283.
- Joseph DB, Turco AE, Vezina CM, et al. Progenitors in prostate development and disease. Dev Biol. 2021 May;473:50–58.
- McNeal JE. Normal histology of the prostate. Am J Surg Pathol. 1988 Aug;12(8):619–633.
- Henry GH, Malewska A, Joseph DB, et al. A cellular anatomy of the normal adult human prostate and prostatic urethra. Cell Rep. 2018 Dec 18;25(12):3530–3542 e5.
- Farnsworth WE. Prostate stroma: physiology. Prostate. 1999 Jan 1;38(1):60–72.
- Prins GS, Putz O. Molecular signaling pathways that regulate prostate gland development. Differentiation. 2008 Jul;76(6):641–659.
- El-Alfy M, Pelletier G, Hermo LS, et al. Unique features of the basal cells of human prostate epithelium. Microsc Res Tech. 2000 Dec 1;51(5):436–446.
- Moad M, Hannezo E, Buczacki SJ, et al. Multipotent basal stem cells, maintained in localized proximal niches, support directed long-ranging epithelial flows in human prostates. Cell Rep. 2017 Aug 15;20(7):1609–1622.
- Crowley L, Cambuli F, Aparicio L, et al.A single-cell atlas of the mouse and human prostate reveals heterogeneity and conservation of epithelial progenitors. Elife. 2020 Sep 11;9:e59465.
- Karthaus WR, Iaquinta PJ, Drost J, et al. Identification of multipotent luminal progenitor cells in human prostate organoid cultures. Cell. 2014 Sep 25;159(1):163–175.
- van Leenders G, Dijkman H, Hulsbergen-van de Kaa C, et al. Demonstration of intermediate cells during human prostate epithelial differentiation in situ and in vitro using triple-staining confocal scanning microscopy. Lab Invest. 2000 Aug;80(8):1251–1258.
- di Sant’agnese PA. Neuroendocrine cells of the prostate and neuroendocrine differentiation in prostatic carcinoma: a review of morphologic aspects. Urology. 1998 May;51(5A Suppl):121–124.
- Butler W, Huang J. Neuroendocrine cells of the prostate: histology, biological functions, and molecular mechanisms. Precis Clin Med. 2021 Mar;4(1):25–34.
- Tabula Sapiens C, Jones RC, Karkanias J, et al. The Tabula Sapiens: a multiple-organ, single-cell transcriptomic atlas of humans. Science. 2022 May 13;376(6594):eabl4896.
- Eraslan G, Drokhlyansky E, Anand S, et al. Single-nucleus cross-tissue molecular reference maps toward understanding disease gene function. Science. 2022 May 13;376(6594):eabl4290.
- Davis JD, Wypych TP. Cellular and functional heterogeneity of the airway epithelium. Mucosal Immunol. 2021 Sep;14(5):978–990.
- Montoro DT, Haber AL, Biton M, et al. A revised airway epithelial hierarchy includes CFTR-expressing ionocytes. Nature. 2018 Aug;560(7718):319–324.
- Joseph DB, Henry GH, Malewska A, et al. 5-alpha reductase inhibitors induce a prostate luminal to club cell transition in human benign prostatic hyperplasia. J Pathol. 2022 Apr;256(4):427–441.
- Joseph DB, Henry GH, Malewska A, et al. Urethral luminal epithelia are castration-insensitive cells of the proximal prostate. Prostate. 2020 Aug;80(11):872–884.
- Oliveira DS, Dzinic S, Bonfil AI, et al. The mouse prostate: a basic anatomical and histological guideline. Bosn J Basic Med Sci. 2016 Feb 10;16(1):8–13.
- Pointis G, Latreille MT, Mignot TM, et al. Regulation of testosterone synthesis in the fetal mouse testis. J Steroid Biochem. 1979 Nov-Dec;11(5–6):1609–1612.
- Sugimura Y, Cunha GR, Donjacour AA, et al. Whole-mount autoradiography study of DNA synthetic activity during postnatal development and androgen-induced regeneration in the mouse prostate. Biol Reprod. 1986 Jun;34(5):985–995.
- Bruni-Cardoso A, Carvalho HF. Dynamics of the epithelium during canalization of the rat ventral prostate. Anat Rec. 2007 Oct;290(10):1223–1232.
- Signoretti S, Waltregny D, Dilks J, et al. P63 is a prostate basal cell marker and is required for prostate development. Am J Pathol. 2000 Dec;157(6):1769–1775.
- Pignon JC, Grisanzio C, Geng Y, et al. P63-expressing cells are the stem cells of developing prostate, bladder, and colorectal epithelia. Proc Natl Acad Sci U S A. 2013 May 14;110(20):8105–8110.
- Choi N, Zhang B, Zhang L, et al. Adult murine prostate basal and luminal cells are self-sustained lineages that can both serve as targets for prostate cancer initiation. Cancer Cell. 2012 Feb 14;21(2):253–265.
- Lu TL, Huang YF, You LR, et al. Conditionally ablated Pten in prostate basal cells promotes basal-to-luminal differentiation and causes invasive prostate cancer in mice. Am J Pathol. 2013 Mar;182(3):975–991.
- Kwon OJ, Zhang L, Ittmann MM, et al. Prostatic inflammation enhances basal-to-luminal differentiation and accelerates initiation of prostate cancer with a basal cell origin. Proc Natl Acad Sci U S A. 2014 Feb 4;111(5):E592–600.
- Toivanen R, Mohan A, Shen MM. Basal progenitors contribute to repair of the prostate epithelium following induced Luminal Anoikis. Stem Cell Rep. 2016 May 10;6(5):660–667.
- Xie Q, Liu Y, Cai T, et al. Dissecting cell-type-specific roles of androgen receptor in prostate homeostasis and regeneration through lineage tracing. Nat Commun. 2017 Jan 23;8:14284.
- Tsujimura A, Koikawa Y, Salm S, et al. Proximal location of mouse prostate epithelial stem cells: a model of prostatic homeostasis. J Cell Bio. 2002 Jun 24;157(7):1257–1265.
- Liu J, Pascal LE, Isharwal S, et al. Regenerated luminal epithelial cells are derived from preexisting luminal epithelial cells in adult mouse prostate. Mol Endocrinol. 2011 Nov;25(11):1849–1857.
- Wang X, Kruithof-de Julio M, Economides KD, et al. A luminal epithelial stem cell that is a cell of origin for prostate cancer. Nature. 2009 Sep 24;461(7263):495–500.
- Abate-Shen C, Shen MM, Gelmann E. Integrating differentiation and cancer: the Nkx3.1 homeobox gene in prostate organogenesis and carcinogenesis. Differentiation. 2008 Jul;76(6):717–727.
- Shi X, Gipp J, Dries M, et al. Prostate progenitor cells proliferate in response to castration. Stem Cell Res. 2014 Jul;13(1):154–163.
- Wojno KJ, Epstein JI. The utility of basal cell-specific anti-cytokeratin antibody (34 beta E12) in the diagnosis of prostate cancer. A review of 228 cases. Am J Surg Pathol. 1995 Mar;19(3):251–260.
- Cancer Genome Atlas Research N. The molecular taxonomy of primary prostate cancer. Cell. 2015 Nov 5;163(4):1011–1025.
- Kim MJ, Cardiff RD, Desai N, et al. Cooperativity of Nkx3.1 and Pten loss of function in a mouse model of prostate carcinogenesis. Proc Natl Acad Sci U S A. 2002 Mar 5;99(5):2884–2889.
- Ellwood-Yen K, Graeber TG, Wongvipat J, et al. Myc-driven murine prostate cancer shares molecular features with human prostate tumors. Cancer Cell. 2003 Sep;4(3):223–238.
- Greenberg NM, DeMayo F, Finegold MJ, et al. Prostate cancer in a transgenic mouse. Proc Natl Acad Sci U S A. 1995 Apr 11;92(8):3439–3443.
- Wang ZA, Toivanen R, Bergren SK, et al. Luminal cells are favored as the cell of origin for prostate cancer. Cell Rep. 2014 Sep 11;8(5):1339–1346.
- Goldstein AS, Huang J, Guo C, et al. Identification of a cell of origin for human prostate cancer. Science. 2010 Jul 30;329(5991):568–571.
- Park JW, Lee JK, Phillips JW, et al. Prostate epithelial cell of origin determines cancer differentiation state in an organoid transformation assay. Proc Natl Acad Sci U S A. 2016 Apr 19;113(16):4482–4487.
- Perou CM, Sorlie T, Eisen MB, et al. Molecular portraits of human breast tumours. Nature. 2000 Aug 17;406(6797):747–752.
- Zhao SG, Chang SL, Erho N, et al. Associations of luminal and basal subtyping of prostate cancer with prognosis and response to androgen deprivation therapy. JAMA Oncol. 2017 Dec 1;3(12):1663–1672.
- Tan HL, Haffner MC, Esopi DM, et al. Prostate adenocarcinomas aberrantly expressing p63 are molecularly distinct from usual-type prostatic adenocarcinomas. Mod Pathol. 2015 Mar;28(3):446–456.
- Torres A, Alshalalfa M, Davicioni E, et al. ETS2 is a prostate basal cell marker and is highly expressed in prostate cancers aberrantly expressing p63. Prostate. 2018 Sep;78(12):896–904.
- Simper NB, Jones CL, MacLennan GT, et al. Basal cell carcinoma of the prostate is an aggressive tumor with frequent loss of PTEN expression and overexpression of EGFR. Hum Pathol. 2015 Jun;46(6):805–812.
- Ali TZ, Epstein JI. Basal cell carcinoma of the prostate: a clinicopathologic study of 29 cases. Am J Surg Pathol. 2007 May;31(5):697–705.
- Davis ID, Martin AJ, Stockler MR, et al. Enzalutamide with standard first-line therapy in metastatic prostate cancer. N Engl J Med. 2019 Jul 11;381(2):121–131.
- Smith MR, Saad F, Chowdhury S, et al. Apalutamide and overall survival in prostate cancer. Eur Urol. 2021 Jan;79(1):150–158.
- Fizazi K, Blue I, Nowak JT. Darolutamide and survival in nonmetastatic, castration-resistant prostate cancer: a patient perspective of the ARAMIS trial. Future Oncol. 2021 May;17(14):1699–1707.
- Beltran H, Hruszkewycz A, Scher HI, et al. The role of lineage plasticity in prostate cancer therapy resistance. Clin Cancer Res. 2019 Dec 1;25(23):6916–6924.
- Graf T, Enver T. Forcing cells to change lineages. Nature. 2009 Dec 3;462(7273):587–594.
- Aggarwal R, Rydzewski NR, Zhang L, et al. Prognosis associated with luminal and basal subtypes of metastatic prostate cancer. JAMA Oncol. 2021 Nov 1;7(11):1644–1652.
- Ku SY, Rosario S, Wang Y, et al. Rb1 and Trp53 cooperate to suppress prostate cancer lineage plasticity, metastasis, and antiandrogen resistance. Science. 2017 Jan 6;355(6320):78–83.
- Mu P, Zhang Z, Benelli M, et al. SOX2 promotes lineage plasticity and antiandrogen resistance in TP53- and RB1-deficient prostate cancer. Science. 2017 Jan 6;355(6320):84–88.
- Nyquist MD, Corella A, Coleman I, et al. Combined TP53 and RB1 loss promotes prostate cancer resistance to a spectrum of therapeutics and confers vulnerability to replication stress. Cell Rep. 2020 May 26;31(8):107669.
- Smith BA, Sokolov A, Uzunangelov V, et al. A basal stem cell signature identifies aggressive prostate cancer phenotypes. Proc Natl Acad Sci U S A. 2015 Nov 24;112(47):E6544–52.
- Di Giacomo V, Tian TV, Mas A, et al. DeltaNp63alpha promotes adhesion of metastatic prostate cancer cells to the bone through regulation of CD82. Oncogene. 2017 Aug;36(31):4381–4392.
- Galoczova M, Nenutil R, Pokorna Z, et al. Tap63 and DeltaNp63 (p40) in prostate adenocarcinomas: DeltaNp63 associates with a basal-like cancer stem cell population but not with metastasis. Virchows Arch. 2021 Apr;478(4):627–636.
- Zhang D, Park D, Zhong Y, et al. Stem cell and neurogenic gene-expression profiles link prostate basal cells to aggressive prostate cancer. Nat Commun. 2016 Feb 29;7:10798.
- Che M, Chaturvedi A, Munro SA, et al. Opposing transcriptional programs of KLF5 and AR emerge during therapy for advanced prostate cancer. Nat Commun. 2021 Nov 4;12(1):6377.
- Tang F, Xu D, Wang S, et al. Chromatin profiles classify castration-resistant prostate cancers suggesting therapeutic targets. Science. 2022 May 27;376(6596):eabe1505.
- Kregel S, Kiriluk KJ, Rosen AM, et al. Sox2 is an androgen receptor-repressed gene that promotes castration-resistant prostate cancer. PLoS ONE. 2013;8(1):e53701.
- Li H, Wang L, Li Z, et al. SOX2 has dual functions as a regulator in the progression of neuroendocrine prostate cancer. Lab Invest. 2020 Apr;100(4):570–582.
- de Wet L, Williams A, Gillard M, et al. SOX2 mediates metabolic reprogramming of prostate cancer cells. Oncogene. 2022 Feb;41(8):1190–1202.
- Fang Y, Liao G, Yu B. LSD1/KDM1A inhibitors in clinical trials: advances and prospects. J Hematol Oncol. 2019 Dec 4;12(1):129.
- Sehrawat A, Gao L, Wang Y, et al. LSD1 activates a lethal prostate cancer gene network independently of its demethylase function. Proc Natl Acad Sci U S A. 2018 May 1;115(18):E4179–4188.
- Lee TI, Jenner RG, Boyer LA, et al. Control of developmental regulators by Polycomb in human embryonic stem cells. Cell. 2006 Apr 21;125(2):301–313.
- Dardenne E, Beltran H, Benelli M, et al. N-Myc induces an EZH2-mediated transcriptional program driving neuroendocrine prostate cancer. Cancer Cell. 2016 Oct 10;30(4):563–577.
- Zhang Y, Zheng D, Zhou T, et al. Androgen deprivation promotes neuroendocrine differentiation and angiogenesis through CREB-EZH2-TSP1 pathway in prostate cancers. Nat Commun. 2018 Oct 4;9(1):4080.
- Li C, Wang Y, Gong Y, et al. Finding an easy way to harmonize: a review of advances in clinical research and combination strategies of EZH2 inhibitors. Clin Epigenetics. 2021 Mar 24;13(1):62.
- Hsu EC, Rice MA, Bermudez A, et al. Trop2 is a driver of metastatic prostate cancer with neuroendocrine phenotype via PARP1. Proc Natl Acad Sci U S A. 2020 Jan 28;117(4):2032–2042.
- Cardillo TM, Govindan SV, Sharkey RM, et al. Sacituzumab Govitecan (IMMU-132), an anti-trop-2/SN-38 antibody-drug conjugate: characterization and efficacy in pancreatic, gastric, and other cancers. Bioconjug Chem. 2015 May 20;26(5):919–931.
- Bardia A, Mayer IA, Vahdat LT, et al. Sacituzumab Govitecan-hziy in refractory metastatic triple-negative breast cancer. N Engl J Med. 2019 Feb 21;380(8):741–751.
- Shiota M, Bishop JL, Takeuchi A, et al. Inhibition of the HER2-YB1-AR axis with Lapatinib synergistically enhances Enzalutamide anti-tumor efficacy in castration resistant prostate cancer. Oncotarget. 2015 Apr 20;6(11):9086–9098.
- Han H, Wang Y, Curto J, et al. Mesenchymal and stem-like prostate cancer linked to therapy-induced lineage plasticity and metastasis. Cell Rep. 2022 Apr 5;39(1):110595.