ABSTRACT
Ferroptosis is a new non-apoptotic cell death caused by the accumulation of dysregulated metabolism of ferric iron, amino acids or lipid peroxidation. Increasing studies suggest that ferroptosis is involved in the acute lung injury (ALI). This article aims to review the role of ferroptosis in ALI. ALI is a common respiratory disease and presents a high mortality rate. Inhibiting cell ferroptosis of lung improves the ALI. In addition, several signaling pathways are related to ferroptosis in ALI, involving in iron homeostasis, lipid peroxidation, and amino acid metabolism. Moreover, there are various key factors to regulate the occurrence of ferroptosis in ALI, such as ACSL4, NRF2, and P53. The ACSL4 promotes the ferroptosis, while the NRF2 alleviates the ferroptosis in ALI. The main effect of P53 is to promote ferroptosis. Accordingly, ferroptosis is involved in ALI and may be an important therapeutic target for ALI.
1. Overview of ferroptosis and acute lung injury
Cell death is necessary for biological growth and development. Traditional cell death modes include accidental cell death (ACD) and regulatory cell death (ECD) [Citation1]. ACD is caused by serious injury, such as burn or trauma, which cannot be prevented or regulated and does not involve specific molecular mechanisms [Citation2]. ECD includes apoptosis, pyroptosis, autophagy and so on [Citation1]. As a new mode of cell death, ferroptosis is different from any of the previously known modes of cell death and is caused by a reduction in intracellular glutathione (GSH) synthesis and inactivation of glutathione peroxidase 4(GPX4) [Citation3,Citation4]. Iron, as an essential trace element, plays an important role in the growth and development of human body [Citation5]. For example, synthetic heme and other important cofactors and participate in the normal life activities of the body [Citation6]. The Fenton reaction between excess iron and hydrogen peroxide produces a large number of highly oxidizing hydroxyl radicals, which then leads to ferroptosis [Citation7,Citation8]. Different from apoptosis, necrosis, autophagy and other cell death modes [Citation9,Citation10], ferroptosis occurs without chromatin condensation and cytoplasm swelling and rupture, which are characteristic changes of conventional cell death patterns [Citation11]. The morphological characteristics of ferroptosis are that the cell membrane is not ruptured, the mitochondria atrophied, the membrane density increased, the outer membrane of mitochondria ruptured, and the intra luminal crest decreased significantly [Citation12]. Biochemically, reactive oxygen species (ROS) accumulation, GSH depletion, and decreased expression of the cystine/glutamate antiporter are the characteristics of ferroptosis [Citation13]. Increasing studies confirm that ferroptosis is involved in the occurrence of various diseases and is a therapeutic target of many diseases [Citation14–16]. It is reported that ferroptosis plays an important role in tumor suppression and immunity regulation. P53, a common tumor suppressor gene, can inhibit the expression of solute carrier family 7 member 11(SLC7A11) and promote ferroptosis [Citation17]. Selenium enhances the expression of GPX4, thereby inhibiting ferroptosis in T follicular helper (TFH) cells and promoting immune response [Citation18]. In addition, ferroptosis is also involved in red blood cell development and aging of various organs [Citation19]. All these studies indicate that ferroptosis plays an important physiological role in human body. Furthermore, ferroptosis is widely involved in kidney injury [Citation20], lung injury [Citation21], neurodegeneration [Citation22], autoimmune diseases [Citation23] and retinal diseases [Citation24].
ALI, the primary stage of acute respiratory distress syndrome (ARDS), is a common respiratory disease [Citation25], which is caused by a variety of causes, including radiotherapy [Citation26], ischemia-reperfusion injury [Citation27,Citation28], acute pancreatitis [Citation29], sepsis [Citation30], pneumonia [Citation31], COVID-19 infection [Citation32], etc. ALI is characterized by diffuse alveolar injury, interstitial edema of lung tissue, neutrophilic inflammation, and surfactant dysfunction [Citation33]. Clinically, ALI manifests as decreased lung compliance, severe hypoxemia, and bilateral lung infiltration [Citation34]. ALI and ARDS account for more than 10% of all intensive care unit (ICU) admissions and the overall mortality rate of ALI is highly 40% [Citation35]. Currently, many factors are involved in the pathogenesis of ALI. It is reported that long non-coding RNAs (lncRNAs) play an important role in ALI [Citation36]. For example, some lncRNAs participate in acute lung inflammation by regulating miRNA or downstream targets or by directly regulating pyroptosis [Citation37,Citation38]; LncRNA metastasis associated lung adenocarcinoma transcript 1 (MALAT1) involved in the pathogenesis of lung diseases by regulating the abnormal activation of macrophages [Citation39]; Inhibition of lncRNA nuclear paraspeckle assembly transcript 1 (NEAT1) reduces apoptosis and promotes cell proliferation during ALI [Citation39]. In addition, mast cells (MCs) and polymorphonuclear neutrophils (PMNs) are the major inflammatory cells and participants in ALI [Citation40,Citation41]. MC activation may be involved in the occurrence of ALI after orthotopic autologous liver transplantation (OALT) by regulating PMN apoptosis [Citation42]. Meanwhile, overexpressed Brahma-related gene-1 (Brg1) alleviated hepatic ischemia/reperfusion (HIR)-induced lung damage by increasing the expression of nuclear factor erythroid 2-related factor 2 (Nrf2) and nuclear translocation activated antioxidant enzymes [Citation43]. However, no significant progress has been made in the treatment of ALI so far.
Currently, increased studies suggest that the accumulation of lipid peroxides which is an important cause of ferroptosis plays an important role in ALI [Citation44]. It is reported that ferroptosis occurs in lung microvascular endothelial cell of ALI mouse induced by sepsis [Citation45]. Moreover, ALI is improved by inhibiting cell ferroptosis [Citation27,Citation28]. Consequently, ferroptosis may be involved in ALI and is an important therapeutic target for ALI [Citation46].
2. The signaling pathway of ferroptosis in ALI
2.1. Iron homeostasis and ferroptosis in ALI
Normally, the absorption, output, utilization and storage of iron are relatively balanced. Once the iron homeostasis in the body is broken, the excess ferrous ions will undergo the Fenton reaction to produce a large amount of reactive oxygen and results in cell death, which is the iron metabolism mechanism of ferroptosis [Citation7]. The lung tissue of ALI mouse induced by oleic acid injection showed GSH and GPX4 expression were decreased, cell mitochondria atrophy, mitochondrial membrane rupture and other characteristics of ferroptosis as well as the intracellular iron content increased and transferrin (TF) expression decreased [Citation47]. TF is an important transporter to maintain iron homeostasis in human body [Citation48]. In the experiment, the reduction of transferrin level caused iron accumulation in mice, which led to ferroptosis and caused ALI. Accordingly, iron homeostasis in body is closely related to the occurrence of ferroptosis in ALI.
2.2. Lipid peroxidation and ferroptosis in ALI
Due to the abnormalities of lipid metabolism in body, a large number of lipids are oxidized under the action of free radicals or lipid peroxidase to produce cytotoxic lipid peroxides [Citation49,Citation50]. The accumulation of lipid peroxides is considered to be a hallmark of ferroptosis [Citation44] and is produced from a variety of substances, the most important of which are polyunsaturated fatty acids (PUFAs). PUFAs are esterified into membrane phospholipids and then oxidized by Arachidonate 15-Lipoxygenase (ALOX15) to lipid peroxides with cytotoxic to promote ferroptosis [Citation51]. Lipid peroxides damage cells in multiple ways [Citation52,Citation53]. One is the further decomposition of lipid peroxides into ROS, which further amplifies the lipid peroxidation process, and the formation and accumulation of lipid ROS is an important cause of ferroptosis [Citation54]. In addition, lipid peroxides disrupt cellular metabolism by changing the physical structure of cell membrane. Moreover, the derivative decomposition of lipid peroxidation products such as malondialdehyde (MDA) and 4-hydroxynonenal (4-HNE) cause cell damage [Citation52,Citation53] and these derivatives are important molecular markers of ferroptosis and lipid peroxidation [Citation55]. ALI mice induced by Lipopolysaccharide (LPS) showed that the Fe content, ROS accumulation, MDA, 4-HNE derivatives of lipid peroxidase decomposition were significantly increased, which indicates ferroptosis occurred in ALI mice [Citation56]. ALI induced by radiation showed the same characteristics of ferroptosis as the ALI mice induced by LPS [Citation57]. The mice induced by radiation developed pathological manifestations of ALI such as pulmonary interstitial congestion, edema, and abnormal intracellular mitochondrial morphology, reduction of GPX4, accumulation of a large number of ROS and other characteristics of ferroptosis [Citation57]. Importantly, ferroptosis inhibitor administration leads to the down-regulation of intracellular ROS and alleviation of the pathological manifestations of ALI. Accordingly, lipid peroxidation is an important cause of ferroptosis in ALI. However, the specific mechanism of lipid peroxidation involved in ferroptosis still needs to be further explored.
2.3. Amino acid metabolism and ferroptosis in ALI
It is reported that the decrease of intracellular GSH and GPX4 activity is the significant manifestations of ferroptosis [Citation46]. GSH is mainly present in two forms in body, reduced GSH and oxidized GSH. As an important antioxidant, GSH effectively scavenges free radicals in cells [Citation58]. GPX4, also known as Phospholipid Hydrogen Peroxide Glutathione Peroxidase (PHGPx), is synthesized using GSH as a substrate, whose function is to reduce lipid hydroperoxides to nontoxic lipid alcohols in cells, thereby protecting cells from damage [Citation59,Citation60]. It is suggested that GPX4 reduces the lipid peroxides on the cell membrane to inhibit the occurrence of ferroptosis [Citation61]. Accordingly, GPX4 activators may play a role in inhibiting the ferroptosis [Citation62]. In addition, GSH interacts with GPX4 to participate in anti-lipid peroxidation in cells [Citation61]. Depletion of GSH results in the loss of cellular antioxidant capacity and inhibition of GPX4 activity [Citation46,Citation63]. Meanwhile, the synthesis of GSH and GPX4 is inseparable from the metabolism of amino acids [Citation64]. Cystine/glutamic antiporter system (system xc-) is a transmembrane amino acid transporter on the cell surface and is composed of two amino acid chains, solute carrier family 3 member 2(SLC3A2) and SLC7A11 [Citation65]. Cells rely on the system xc- to mediate the exchange of extracellular cystine and intracellular glutamate to maintain the balance of internal and external amino acids [Citation66]. Intracellular cystine is reduced to cysteine and then co-synthesizes reduced glutathione with glutamate and glycine, which participates in intracellular antioxidant processes and protects cells from oxidative damage [Citation67]. Accordingly, inhibition of system xc- activity leads to dysfunction of GSH synthesis, decrease of GPX4 activity, reduction in cellular antioxidant capacity, accumulation of lipid ROS, which ultimately lead to oxidative damage and ferroptosis in cells [Citation67]. As the light-chain subunit of system xc-, SLC7A11 is an important regulator in the ferroptosis. It is suggested that Nrf2 regulates SLC7A1 through STAT3 and OH-1 to inhibit cell ferroptosis, and improves ALI induced by intestinal ischemia-reperfusion (IIR) [Citation27,Citation28]. Therefore, amino acid metabolism is closely related to the ferroptosis in ALI ().
Figure 1. Signaling pathways in ferroptosis. The figure shows the signaling pathways in ferroptosis, which can be roughly divided into three categories. The first one is iron metabolism pathways. The extracellular ferric iron is oxidized to ferrous iron and transported into the cell, and the excess iron ions undergo the Fenton reaction to generate a large amount of ROS. The second is lipid peroxidation. PUFA generate a large amount of lipid peroxides under the action of a series of enzymes. Accumulation of lipid ROS leads to ferroptosis. The third is amino acid metabolism. Cystine is transported into cells by system xc- and then reduced to cysteine, which together with glutamate and glycine synthesize GSH, promote the synthesis of GPX4, remove intracellular lipid ROS, and inhibit ferroptosis. The physiological function of ferroptosis is manifested in tumor suppression, immunological regulation, development and aging. In addition, ferroptosis is also widely involved in organ damage, neurodegeneration, autoimmune diseases and retinal diseases.
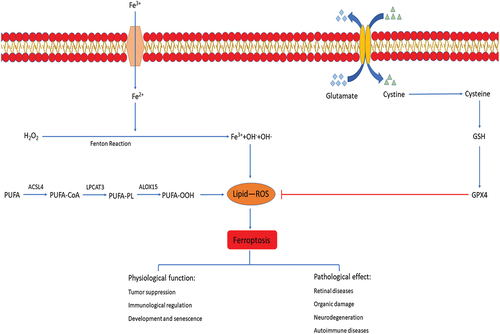
3. Key regulators of ferroptosis in ALI
3.1. ACSL4
Acyl-CoA synthase long-chain family member 4 (ACSL4) is a key enzyme in the esterification of fatty acids with 12–20 carbon chain length [Citation68,Citation69]. It is suggested that ACSL4 may play an important role in ferroptosis [Citation70]. With the action between ACSL4 and Recombinant Lysophosphatidylcholine Acyltransferase 3 (LPCAT3), PUFA generates PUFA-PL and is inserted into the cell membrane. In addition, PUFA-PL reacts with the lipid peroxidase ALOX15 to produce the lipid peroxide PUFA-OOH which damages the cell membrane and then the ferroptosis occurs [Citation71]. Several studies showed that ALI induced by intestinal ischemia is closely related to ferroptosis [Citation27,Citation28]. It is suggested that the expression of ACSL4 is elevated in ferroptosis induced by IIR [Citation70]. In addition, ROSI, an inhibitor of ACSL4, reduces lipid peroxidation significantly [Citation72], which suggests that inhibition of ACSL4 may alleviate ferroptosis [Citation73]. In addition, with the up-expression of CircEXOC5, the stability of ACSL4 mRNA is enhanced by binding to polypyrimidine tract-binding protein 1 (PTBP1), thereby promoting the occurrence of ferroptosis in lung microvascular endothelial cell of ALI mouse induced by sepsis [Citation45]. Therefore, ACSL4 is a key regulator of ferroptosis in ALI.
3.2. NRF2
Nrf2 is a key regulator for cells to maintain oxidation stability by regulating cellular antioxidant stress and eliminating ROS and is activated under conditions of high oxidative stress [Citation74,Citation75]. Nrf2 is considered to be an important regulator of ferroptosis and regulates cell ferroptosis by inducing transcription of GPX4 and other related genes [Citation76]. Nrf2 promotes STAT3 activation [Citation27]. As an oxidative reactive transcription factor, STAT3 plays an active role in oxidative stress of ferroptosis [Citation77]. The overexpression of STAT3 up-regulates SLC7A11 and inhibits ferroptosis, thereby alleviating ALI induced by intestinal ischemia [Citation27]. SLC7A11 is a gene target of Nrf2 [Citation78] and encodes the system xc- which is responsible for the input of cystine for glutathione biosynthesis and antioxidant defense [Citation13]. In addition, SLC7A11 regulates iron overloading in ferroptosis [Citation13]. Moreover, Nrf2 regulates ferroptosis in mouse lung epithelial cells by regulating toll-like receptor 4 (TLR4) and Akt signaling pathways [Citation79]. Loss of Nrf2 upregulates TLR4, increases IIR-induced Akt inactivation, and thereby causes ferroptosis in cells [Citation79]. In addition, the translocation of Nrf2 into the nucleus is promoted by Dimethyl Formamide (DMF), which inhibits the occurrence of ferroptosis in MLE-12 cells and then alleviates the ALI caused by seawater drowning [Citation80]. Nrf2 can also affect ferroptosis by regulating TERT and SLC7A11 [Citation81]. Meanwhile, NRF2 promotes the expression of telomerase reverse transcriptase TERT which alleviates ferroptosis by decreasing the intracellular ROS levels and promotes the expression of SLC7A11 [Citation81,Citation82]. Moreover, itaconate, a metabolite of inflammatory macrophages, inhibits ferroptosis through the Nrf2 pathway and alleviates sepsis-induced ALI [Citation83]. Accordingly, Nrf2 may play an important role in the ferroptosis of ALI [Citation84]. However, the regulation mechanism of Nrf2 on ferroptosis is unclear.
3.3. P53
P53 is a tumor suppressor gene that encodes the production of p53 protein [Citation85]. In normal cells, p53 protein is usually maintained at low levels [Citation86]. Under stress conditions, such as DNA damage, hypoxia and nutritive deficiency, p53 gene is activated, and a large amount of p53 protein is transcribed and accumulated [Citation87]. P53 protein plays a role in tumor inhibition through multiple cellular reactions such as apoptosis [Citation88] and ferroptosis [Citation17]. P53 enhances tumor suppressor function by regulating ferroptosis [Citation89], thereby ferroptosis may be a tumor suppressor [Citation12,Citation90]. It is reported that P53 promoting ferroptosis is closely related to SCL7A11, STA1, and glutaminase 2 (GSL2) [Citation91]. SLC7A11 is the target of p53 and the reduction of SLC7A11 caused by P53KR, an acetylation-deficient mutant form of p53 protein, promotes ferroptosis in cells under ROS-induced stress state [Citation17]. SAT1 is a transcriptional target of p53 [Citation92]. SAT1 activity is increased in various stress responses, and overexpression of SAT1 leads to rapid depletion of cellular spermidine and spermine, resulting in significant growth inhibition and mitochondrial apoptosis [Citation93]. GSL2, another transcriptional target of P53, increases the production of GSH in cells to enhance antioxidant function [Citation94] and is considered as a negative regulator of ferroptosis [Citation95]. In addition, inhibition of p53 leads to iASPP inducing the accumulation of Nrf2, thereby resulting in increase of HIF-1α and reduction of the TF and ferrous degradation-related proteins, and subsequently increases expression of GPX4 in cells, then the ferroptosis is inhibited in ALI [Citation96]. In addition, STAT6 inhibits the acetylation of p53 and restores the expression of SLC7A11, alleviates ferroptosis and attenuates ALI of mice [Citation97]. While SIRT1 reduces the deacetylation level of p53 and inhibit ferroptosis to alleviate ALI induced by heat stress [Citation98]. However, the role of P53 in the regulation of ferroptosis in ALI still needs more researches in future.
3.4. Other regulatory factors
It has been reported that GPX4 inhibits the occurrence of ferroptosis by reducing lipid peroxides on the cell membrane [Citation61] and is a key regulator of ferroptosis [Citation63]. Cyst(e)ine activates mechanistic/mammalian target of rapamycin complex 1 (mTORC1) and promotes GPX4 and inhibition of mTORC1 by drugs reduces GPX4 protein levels, which make cancer cells sensitive to ferroptosis [Citation99]. In addition, RSL3 promotes ferroptosis by reducing the expression of GPX4 through the NF-κB pathway [Citation100]. Meanwhile, the slight decrease of GPX4 in chondrocytes in the Osteoarthritis (OA) environment increases the sensitivity of cells to oxidative stress, leading to the occurrence of ferroptosis in OA [Citation101]. In addition, the amino acid antiporter system xc- plays an important role in maintaining amino acid balance and regulating ferroptosis. Studies suggest that inhibition of the system xc− results in a rapid decrease in intracellular GSH levels and ferroptosis [Citation67]. Naringenin (NAR) attenuates myocardial ischemia/reperfusion injury by inhibiting ferroptosis through activating Nrf2/system xc -/GPX4 axis [Citation102]. Since system xc- is composed of two subunits SLC7A11 and SLC3A2L [Citation65]regulation of SLC7A11 and SLC3A2L can affect system xc- and thereby regulate ferroptosis. It is suggested that ferroptosis-inducing small molecule erastin or activating transcription factor 3 (ATF3) inhibit the transmembrane protein SLC7A11, thereby inhibiting the amino acid antiporter system xc-, reducing the cellular uptake of cystine, and lowering GSH levels to promote ferroptosis [Citation103,Citation104]. Moreover, BECN1 directly blocks the activity of system xc- by binding to SLC7A11 to promote ferroptosis [Citation103]. Accordingly, system xc- is an important regulator of ferroptosis. In LPS-induced ALI mouse model, the expression of SLC7A11 and GPX4 in bronchial epithelial cells of ALI mice was significantly decreased [Citation56]. Based on the above studies, GPX4 and system xc- may be involved in the regulation of ferroptosis in ALI (, ).
Figure 2. Key regulators of ferroptosis in ALI. The figure shows the key regulators of ferroptosis in ALI. STIRT1 mediates P53 deacetylation, promotes SLC7A11 production, and inhibits ferroptosis. STAT6 inhibited the acetylation of P53, reduced the content of ACSL4, and restored the expression of SLC7A11 and GPX4. GPX4 further reduces the content of ROS and lipid peroxidation metabolites such as MDA, 4-HNE by reducing lipid peroxides, relieves the damage of lipid peroxides to cell membranes, and inhibits ferroptosis Nrf2 mainly regulates ferroptosis in ALI through three pathways. Nrf2 promotes the expression of STAT3 and TERT to upregulate SLC7A11, and inhibits ferroptosis. In addition, Nrf2 can also down-regulate TLR4 and inhibit ferroptosis by increasing the activity of AKT. Ferroptosis plays an important role in ALI, and inhibition of ferroptosis can effectively alleviate ALI.
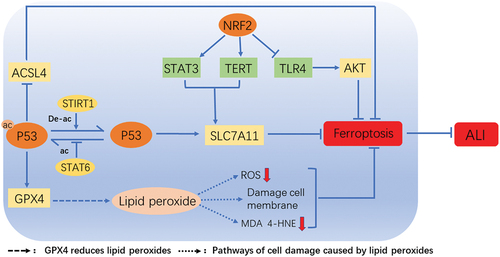
Table 1. The influence and regulation mechanism of several factors on ferroptosis.
4. Summary and prospect
As a newly discovered cell death mode, the ferroptosis occurs in ALI and the ALI is improved by inhibiting cell ferroptosis. Accordingly, ferroptosis plays an important role in ALI. Several signals are related to ferroptosis in ALI, involving in iron homeostasis, lipid peroxidation, and amino acid metabolism. Abnormalities of iron homeostasis and amino acid metabolism which mainly including glutamate, glycine and cysteine, and accumulation of lipid peroxidation are the main causes of ferroptosis. Moreover, there are various key factors to regulate the occurrence of ferroptosis in ALI, such as ACSL4, NRF2, and P53. The ACSL4 promotes the ferroptosis, while the NRF2 alleviates the ferroptosis in ALI. The main effect of P53 is to promote ferroptosis. In conclusion, ferroptosis is involved in ALI and may be an important therapeutic target for ALI. Several experiments have shown that iron metabolism inhibitors and lipid metabolism inhibitors have the effect of improving ALI. Iron chelators, especially deferoxamine (DFO), have been approved by the FDA for the treatment of ferroptosis, so it is speculated that iron chelators may also be effective for ALI. However, more clinical evidences are still needed for targeting ferroptosis to inhibit ALI.
Disclosure statement
No potential conflict of interest was reported by the author(s).
Data availability statement
The data of the present study are available from the corresponding author on reasonable request.
Additional information
Funding
References
- Tang D, Kang R, Berghe TV, et al. The molecular machinery of regulated cell death. Cell Res. 2019;29(5):347–364. doi: 10.1038/s41422-019-0164-5
- Galluzzi L, Bravo-San Pedro JM, Vitale I, et al. Essential versus accessory aspects of cell death: recommendations of the NCCD 2015. Cell Death Diff. 2015;22(1):58–73. doi: 10.1038/cdd.2014.137
- Dolma S, Lessnick SL, Hahn WC, et al. Identification of genotype-selective antitumor agents using synthetic lethal chemical screening in engineered human tumor cells. Cancer Cell. 2003;3(3):285–296. doi: 10.1016/S1535-6108(03)00050-3
- Dixon SJ, Lemberg KM, Lamprecht MR, et al. Ferroptosis: an iron-dependent form of nonapoptotic cell death. Cell. 2012;149(5):1060–1072. doi: 10.1016/j.cell.2012.03.042
- Abbaspour N, Hurrell R, Kelishadi R. Review on iron and its importance for human health. J Res Med Sci. 2014;19(2):164–174.
- Venkataramani V. Iron homeostasis and metabolism: two sides of a coin. Adv Exp Med Biol. 2021;1301:25–40.
- Wang S, Luo J, Zhang Z, et al. Iron and magnetic: new research direction of the ferroptosis-based cancer therapy. Am J Cancer Res. 2018;8(10):1933–1946.
- Cao JY, Dixon SJ. Mechanisms of ferroptosis. Cellular and molecular life sciences: CMLS. Cell Mol Life Sci. 2016;73(11–12):2195–2209. doi: 10.1007/s00018-016-2194-1
- Gao M, Jiang X. To eat or not to eat-the metabolic flavor of ferroptosis. Curr Opinion Cell Biol. 2018;51:58–64. doi: 10.1016/j.ceb.2017.11.001
- DeHart DN, Fang D, Heslop K, et al. Opening of voltage dependent anion channels promotes reactive oxygen species generation, mitochondrial dysfunction and cell death in cancer cells. Biochem Pharmacol. 2018;148:155–162. doi: 10.1016/j.bcp.2017.12.022
- Yang WS, Stockwell BR. Synthetic lethal screening identifies compounds activating iron-dependent, nonapoptotic cell death in oncogenic-RAS-harboring cancer cells. Chem Biol. 2008;15(3):234–245. doi: 10.1016/j.chembiol.2008.02.010
- Li J, Cao F, Yin HL, et al. Ferroptosis: past, present and future. Cell Death Dis. 2020;11(2):88. doi: 10.1038/s41419-020-2298-2
- Xie Y, Hou W, Song X, et al. Ferroptosis: process and function. Cell Death Diff. 2016;23(3):369–379. doi: 10.1038/cdd.2015.158
- Skouta R, Dixon SJ, Wang J, et al. Ferrostatins inhibit oxidative lipid damage and cell death in diverse disease models. J Am Chem Soc. 2014;136(12):4551–4556. doi: 10.1021/ja411006a
- Martin-Sanchez D, Fontecha-Barriuso M, Martinez-Moreno JM, et al. Ferroptosis and kidney disease. Nefrologia. 2020;40(4):384–394. doi: 10.1016/j.nefro.2020.03.005
- Tian Y, Lu J, Hao X, et al. FTH1 inhibits ferroptosis through ferritinophagy in the 6-OHDA model of Parkinson’s disease. Neurotherapeutics. 2020;17(4):1796–1812. doi: 10.1007/s13311-020-00929-z
- Jiang L, Kon N, Li T, et al. Ferroptosis as a p53-mediated activity during tumour suppression. Nature. 2015;520(7545):57–62. doi: 10.1038/nature14344
- Yao Y, Chen Z, Zhang H, et al. Selenium–GPX4 axis protects follicular helper T cells from ferroptosis. Nat Immunol. 2021;22(9):1127–1139. doi: 10.1038/s41590-021-00996-0
- Stockwell BR. Ferroptosis turns 10: emerging mechanisms, physiological functions, and therapeutic applications. Cell. 2022;185(14):2401–2421. doi: 10.1016/j.cell.2022.06.003
- Ni L, Yuan C, Wu X. Targeting ferroptosis in acute kidney injury. Cell Death Dis. 2022;13(2):182. doi: 10.1038/s41419-022-04628-9
- Li Y, Yang Y, Yang Y. Multifaceted roles of ferroptosis in lung diseases. Front Mol Biosci. 2022;9:919187. doi: 10.3389/fmolb.2022.919187
- Masaldan S, Bush AI, Devos D, et al. Striking while the iron is hot: iron metabolism and ferroptosis in neurodegeneration. Free Radic Biol Med. 2019;133:221–233. doi: 10.1016/j.freeradbiomed.2018.09.033
- Lai B, Wu CH, Wu CY, et al. Ferroptosis and Autoimmune Diseases. Front Immunol. 2022;13:916664. doi: 10.3389/fimmu.2022.916664
- Tang J, Zhuo Y, Li Y. Effects of iron and zinc on mitochondria: potential mechanisms of glaucomatous injury. Front Cell Dev Biol. 2021;9:720288. doi: 10.3389/fcell.2021.720288
- Parekh D, Dancer RC, Thickett DR. Acute lung injury. Clin Med. 2011;11(6):615–618. (London, England). doi: 10.7861/clinmedicine.11-6-615
- Zhang F, Li ZL, Xu XM, et al. Protective effects of icariin-mediated SIRT1/FOXO3 signaling pathway on intestinal ischemia/reperfusion-induced acute lung injury. Mol Med Rep. 2015;11(1):269–276. doi: 10.3892/mmr.2014.2679
- Qiang Z, Dong H, Xia Y, et al. Nrf2 and STAT3 alleviates ferroptosis-mediated IIR-ALI by regulating SLC7A11. Oxid Med Cell Longevity. 2020;2020:5146982. doi: 10.1155/2020/5146982
- Dong H, Qiang Z, Chai D, et al. Nrf2 inhibits ferroptosis and protects against acute lung injury due to intestinal ischemia reperfusion via regulating SLC7A11 and HO-1. Aging. 2020;12(13):12943–12959. doi: 10.18632/aging.103378
- Ge P, Luo Y, Okoye CS, et al. Intestinal barrier damage, systemic inflammatory response syndrome, and acute lung injury: A troublesome trio for acute pancreatitis. Biomedicine & Pharmacotherapy = Biomedecine & Pharmacotherapie. 2020;132:110770. doi: 10.1016/j.biopha.2020.110770
- Wang YM, Qi X, Gong FC, et al. Protective and predictive role of Mucin1 in sepsis-induced ALI/ARDS. Int Immunopharmacol. 2020;83:106438. doi: 10.1016/j.intimp.2020.106438
- Kumar V. Pulmonary innate immune response determines the outcome of inflammation during pneumonia and sepsis-associated acute lung injury. Front Immunol. 2020;11:1722. doi: 10.3389/fimmu.2020.01722
- Anka AU, Tahir MI, Abubakar SD, et al. Coronavirus disease 2019 (COVID-19): an overview of the immunopathology, serological diagnosis and management. Scand J Immunol. 2021;93(4):e12998. doi: 10.1111/sji.12998
- Sahetya SK, Goligher EC, Brower RG. Fifty years of research in ARDS. Setting positive end-expiratory pressure in acute respiratory distress syndrome. Am J Respir Crit Care Med. 2017;195(11):1429–1438. doi: 10.1164/rccm.201610-2035CI
- Mokra D, Kosutova P. Biomarkers in acute lung injury. Respir Physiol Neurobiol. 2015;209:52–58. doi: 10.1016/j.resp.2014.10.006
- Bellani G, Laffey JG, Pham T, et al. Epidemiology, patterns of Care, and mortality for patients with acute respiratory distress syndrome in intensive Care units in 50 countries. JAMA. 2016;315(8):788–800. doi: 10.1001/jama.2016.0291
- Cui H, Banerjee S, Guo S, et al. Long noncoding RNA Malat1 regulates differential activation of macrophages and response to lung injury. JCI Insight. 2019;4(4): doi: 10.1172/jci.insight.124522
- Li J, Tian H, Yang J, et al. Long Noncoding RNAs Regulate Cell Growth, Proliferation, and Apoptosis. DNA Cell Biol. 2016;35(9):459–470. doi: 10.1089/dna.2015.3187
- Bär C, Chatterjee S, Thum T. Long noncoding RNAs in cardiovascular pathology, diagnosis, and therapy. Circulation. 2016;134(19):1484–1499. doi: 10.1161/CIRCULATIONAHA.116.023686
- Chen C, He Y, Feng Y, et al. Long non-coding RNA review and implications in acute lung inflammation. Life Sci. 2021;269:119044. doi: 10.1016/j.lfs.2021.119044
- Grommes J, Soehnlein O. Contribution of neutrophils to acute lung injury. Mol Med. 2011;17(3–4):293–307. (Cambridge, Mass). doi: 10.2119/molmed.2010.00138
- Zhang A, Chi X, Luo G, et al. Mast cell stabilization alleviates acute lung injury after orthotopic autologous liver transplantation in rats by downregulating inflammation. PLoS One. 2013;8(10):e75262. doi: 10.1371/journal.pone.0075262
- Chen C, Zhang Z, Tan F, et al. Stabilizing mast cells improves acute lung injury after orthotopic liver transplantation via promotion of apoptosis in polymorphonuclear neutrophils. Am J Physiol Lung Cell Mol Physiol. 2021;320(2):L266–l75. doi: 10.1152/ajplung.00046.2020
- Ge M, Chen C, Yao W, et al. Overexpression of Brg1 alleviates hepatic ischemia/Reperfusion-induced acute lung injury through antioxidative stress Effects. Oxid Med Cell Longevity. 2017;2017:8787392. doi: 10.1155/2017/8787392
- D’Herde K, Krysko DV. Ferroptosis: Oxidized PEs trigger death. Nat Chem Biol. 2017;13(1):4–5. doi: 10.1038/nchembio.2261
- Wang W, Xu R, Zhao H, et al. CircEXOC5 promotes ferroptosis by enhancing ACSL4 mRNA stability via binding to PTBP1 in sepsis-induced acute lung injury. Immunobiology. 2022;227(4):152219. doi: 10.1016/j.imbio.2022.152219
- Yang WS, SriRamaratnam R, Welsch ME, et al. Regulation of ferroptotic cancer cell death by GPX4. Cell. 2014;156(1–2):317–331. doi: 10.1016/j.cell.2013.12.010
- Zhou H, Li F, Niu JY, et al. Ferroptosis was involved in the oleic acid-induced acute lung injury in mice Sheng Li Xue Bao. 2019;71(5):689–697.
- Huggenvik JI, Craven CM, Idzerda RL, et al. A splicing defect in the mouse transferrin gene leads to congenital atransferrinemia. Blood. 1989;74(1):482–486. doi: 10.1182/blood.V74.1.482.482
- Yang WS, Stockwell BR. Ferroptosis: death by lipid peroxidation. Trends Cell Biol. 2016;26(3):165–176. doi: 10.1016/j.tcb.2015.10.014
- Ayala A, Muñoz MF, Argüelles S. Lipid peroxidation: production, metabolism, and signaling mechanisms of malondialdehyde and 4-hydroxy-2-nonenal. Oxidative medicine and cellular longevity. Oxid Med Cell Longevity. 2014;2014:1–31. doi: 10.1155/2014/360438
- Kagan VE, Mao G, Qu F, et al. Oxidized arachidonic and adrenic PEs navigate cells to ferroptosis. Nat Chem Biol. 2017;13(1):81–90. doi: 10.1038/nchembio.2238
- Gaschler MM, Stockwell BR. Lipid peroxidation in cell death. Biochem Biophys Res Commun. 2017;482(3):419–425. doi: 10.1016/j.bbrc.2016.10.086
- Feng H, Stockwell BR. Unsolved mysteries: how does lipid peroxidation cause ferroptosis? PLoS Biol. 2018;16(5):e2006203. doi: 10.1371/journal.pbio.2006203
- Yang WS, Kim KJ, Gaschler MM, et al. Peroxidation of polyunsaturated fatty acids by lipoxygenases drives ferroptosis. Proc Natl Acad Sci, USA. 2016;113(34):E4966–75. doi: 10.1073/pnas.1603244113
- Doll S, Proneth B, Tyurina YY, et al. ACSL4 dictates ferroptosis sensitivity by shaping cellular lipid composition. Nat Chem Biol. 2017;13(1):91–98. doi: 10.1038/nchembio.2239
- Liu P, Feng Y, Li H, et al. Ferrostatin-1 alleviates lipopolysaccharide-induced acute lung injury via inhibiting ferroptosis. Cell Mol Biol Lett. 2020;25(1):10. doi: 10.1186/s11658-020-00205-0
- Li X, Zhuang X, Qiao T. Role of ferroptosis in the process of acute radiation-induced lung injury in mice. Biochem Biophys Res Commun. 2019;519(2):240–245. doi: 10.1016/j.bbrc.2019.08.165
- Lu SC. Glutathione synthesis. Biochim Biophys Acta Gen Subj. 2013;1830(5):3143–3153. doi: 10.1016/j.bbagen.2012.09.008
- Brigelius-Flohé R. Glutathione peroxidases and redox-regulated transcription factors. Biol Chem. 2006;387(10–11):1329–1335. doi: 10.1515/BC.2006.166
- Gong Y, Wang N, Liu N, et al. Lipid peroxidation and GPX4 inhibition are common causes for myofibroblast differentiation and ferroptosis. DNA Cell Biol. 2019;38(7):725–733. doi: 10.1089/dna.2018.4541
- Maiorino M, Conrad M, Ursini F. GPx4, lipid peroxidation, and cell death: discoveries, rediscoveries, and open issues. Antioxid Redox Signaling. 2018;29(1):61–74. doi: 10.1089/ars.2017.7115
- Proneth B, Conrad M. Ferroptosis and necroinflammation, a yet poorly explored link. Cell Death Diff. 2019;26(1):14–24. doi: 10.1038/s41418-018-0173-9
- Brigelius-Flohé R, Maiorino M. Glutathione peroxidases. Biochim Biophys Acta Gen Subj. 2013;1830(5):3289–3303. doi: 10.1016/j.bbagen.2012.11.020
- Stockwell BR, Friedmann Angeli JP, Bayir H, et al. Ferroptosis: a regulated cell death nexus linking metabolism, Redox biology, and disease. Cell. 2017;171(2):273–285. doi: 10.1016/j.cell.2017.09.021
- Bridges RJ, Natale NR, Patel SA. System xc− cystine/glutamate antiporter: an update on molecular pharmacology and roles within the CNS. Br J Pharmacol. 2012;165(1):20–34. doi: 10.1111/j.1476-5381.2011.01480.x
- Ji X, Qian J, Rahman SMJ, et al. xCT (SLC7A11)-mediated metabolic reprogramming promotes non-small cell lung cancer progression. Oncogene. 2018;37(36):5007–5019. doi: 10.1038/s41388-018-0307-z
- Sun Y, Chen P, Zhai B, et al. The emerging role of ferroptosis in inflammation. Biomed Pharmacother. 2020;127:110108. doi: 10.1016/j.biopha.2020.110108
- Soupene E, Kuypers FA. Mammalian long-chain acyl-CoA synthetases. Exp Biol Med (Maywood). 2008;233(5):507–521. (Maywood, NJ). doi: 10.3181/0710-MR-287
- Ellis JM, Frahm JL, Li LO, et al. Acyl-coenzyme a synthetases in metabolic control. Curr Opin Lipidol. 2010;21(3):212–217. doi: 10.1097/MOL.0b013e32833884bb
- Li Y, Feng D, Wang Z, et al. Ischemia-induced ACSL4 activation contributes to ferroptosis-mediated tissue injury in intestinal ischemia/reperfusion. Cell Death Diff. 2019;26(11):2284–2299. doi: 10.1038/s41418-019-0299-4
- Lei G, Zhuang L, Gan B. Targeting ferroptosis as a vulnerability in cancer. Nat Rev Cancer. 2022;22(7):381–396. doi: 10.1038/s41568-022-00459-0
- Kung YA, Chiang HJ, Li ML, et al. Acyl-coenzyme a synthetase Long-chain family member 4 is involved in viral replication organelle formation and facilitates virus replication via ferroptosis. MBio. 2022;13(1):e0271721. doi: 10.1128/mbio.02717-21
- Xu Y, Li X, Cheng Y, et al. Inhibition of ACSL4 attenuates ferroptotic damage after pulmonary ischemia-reperfusion. FASEB J. 2020;34(12):16262–16275. doi: 10.1096/fj.202001758R
- Dinkova-Kostova AT, Kostov RV, Kazantsev AG. The role of Nrf2 signaling in counteracting neurodegenerative diseases. FEBS J. 2018;285(19):3576–3590. doi: 10.1111/febs.14379
- Nguyen T, Nioi P, Pickett CB. The Nrf2-antioxidant response element signaling pathway and its activation by oxidative stress. J Biol Chem. 2009;284(20):13291–13295. doi: 10.1074/jbc.R900010200
- Kerins MJ, Ooi A. The roles of NRF2 in modulating cellular iron homeostasis. Antioxid Redox Signaling. 2018;29(17):1756–1773. doi: 10.1089/ars.2017.7176
- Linher-Melville K, Singh G. The complex roles of STAT3 and STAT5 in maintaining redox balance: lessons from STAT-mediated xCT expression in cancer cells. Mol Cell Endocrinol. 2017;451:40–52. doi: 10.1016/j.mce.2017.02.014
- Fan Z, Wirth AK, Chen D, et al. Nrf2-Keap1 pathway promotes cell proliferation and diminishes ferroptosis. Oncogenesis. 2017;6(8):e371. doi: 10.1038/oncsis.2017.65
- Yan J, Li J, Zhang L, et al. Nrf2 protects against acute lung injury and inflammation by modulating TLR4 and Akt signaling. Free Radic Biol Med. 2018;121:78–85. doi: 10.1016/j.freeradbiomed.2018.04.557
- Qiu YB, Wan BB, Liu G, et al. Nrf2 protects against seawater drowning-induced acute lung injury via inhibiting ferroptosis. Respir Res. 2020;21(1):232. doi: 10.1186/s12931-020-01500-2
- Dong H, Xia Y, Jin S, et al. Nrf2 attenuates ferroptosis-mediated IIR-ALI by modulating TERT and SLC7A11. Cell Death Dis. 2021;12(11):1027. doi: 10.1038/s41419-021-04307-1
- Indran IR, Hande MP, Pervaiz S. hTERT overexpression alleviates intracellular ROS production, improves mitochondrial function, and inhibits ROS-mediated apoptosis in cancer cells. Cancer Res. 2011;71(1):266–276. doi: 10.1158/0008-5472.CAN-10-1588
- He R, Liu B, Xiong R, et al. Itaconate inhibits ferroptosis of macrophage via Nrf2 pathways against sepsis-induced acute lung injury. Cell Death Discovery. 2022;8(1):43. doi: 10.1038/s41420-021-00807-3
- Wang Y, Chen D, Xie H, et al. AUF1 protects against ferroptosis to alleviate sepsis-induced acute lung injury by regulating NRF2 and ATF3. Cellular and molecular life sciences: CMLS. Cell Mol Life Sci. 2022;79(5):228. doi: 10.1007/s00018-022-04248-8
- Levine AJ. p53, the cellular gatekeeper for growth and division. Cell. 1997;88(3):323–331. doi: 10.1016/S0092-8674(00)81871-1
- Liebl MC, Hofmann TG. The role of p53 signaling in colorectal cancer. Cancers. 2021;13(9):2125. doi: 10.3390/cancers13092125
- Liu J, Zhang C, Wang J, et al. The regulation of ferroptosis by tumor suppressor p53 and its pathway. Int J Mol Sci. 2020;21(21):8387. doi: 10.3390/ijms21218387
- Gottlieb TM, Oren M. p53 and apoptosis. Semin Cancer Biol. 1998;8(5):359–368. doi: 10.1006/scbi.1998.0098
- Wang SJ, Ou Y, Jiang L, et al. Ferroptosis: A missing puzzle piece in the p53 blueprint? Mol Cell Oncol. 2016;3(3):e1046581. doi: 10.1080/23723556.2015.1046581
- Liu J, Kuang F, Kroemer G, et al. Autophagy-dependent ferroptosis: machinery and regulation. Cell Chem Biol. 2020;27(4):420–435. doi: 10.1016/j.chembiol.2020.02.005
- Kang R, Kroemer G, Tang D. The tumor suppressor protein p53 and the ferroptosis network. Free Radic Biol Med. 2019;133:162–168. doi: 10.1016/j.freeradbiomed.2018.05.074
- Ou Y, Wang SJ, Li D, et al. Activation of SAT1 engages polyamine metabolism with p53-mediated ferroptotic responses. Proc Natl Acad Sci, USA. 2016;113(44):E6806–e12. doi: 10.1073/pnas.1607152113
- Mandal S, Mandal A, Park MH. Depletion of the polyamines spermidine and spermine by overexpression of spermidine/spermine N1 -acetyltransferase 1 (SAT1) leads to mitochondria-mediated apoptosis in mammalian cells. Biochem J. 2015;468(3):435–447. doi: 10.1042/BJ20150168
- Hu W, Zhang C, Wu R, et al. Glutaminase 2, a novel p53 target gene regulating energy metabolism and antioxidant function. Proc Natl Acad Sci, USA. 2010;107(16):7455–7460. doi: 10.1073/pnas.1001006107
- Gao M, Monian P, Quadri N, et al. Glutaminolysis and Transferrin Regulate Ferroptosis. Molecular Cell. 2015;59(2):298–308. doi: 10.1016/j.molcel.2015.06.011
- Li Y, Cao Y, Xiao J, et al. Inhibitor of apoptosis-stimulating protein of p53 inhibits ferroptosis and alleviates intestinal ischemia/reperfusion-induced acute lung injury. Cell Death Diff. 2020;27(9):2635–2650. doi: 10.1038/s41418-020-0528-x
- Yang Y, Ma Y, Li Q, et al. STAT6 inhibits ferroptosis and alleviates acute lung injury via regulating P53/SLC7A11 pathway. Cell Death Dis. 2022;13(6):530. doi: 10.1038/s41419-022-04971-x
- Chen H, Lin X, Yi X, et al. SIRT1-mediated p53 deacetylation inhibits ferroptosis and alleviates heat stress-induced lung epithelial cells injury. Int J Hyperthermia. 2022;39(1):977–986. doi: 10.1080/02656736.2022.2094476
- Zhang Y, Swanda RV, Nie L, et al. mTORC1 couples cyst(e)ine availability with GPX4 protein synthesis and ferroptosis regulation. Nat Commun. 2021;12(1):1589. doi: 10.1038/s41467-021-21841-w
- Li S, He Y, Chen K, et al. RSL3 Drives ferroptosis through NF-κB pathway activation and GPX4 depletion in glioblastoma. Oxid Med Cell Longevity. 2021;2021:2915019. doi: 10.1155/2021/2915019
- Miao Y, Chen Y, Xue F, et al. Contribution of ferroptosis and GPX4‘s dual functions to osteoarthritis progression. EBioMedicine. 2022;76:103847. doi: 10.1016/j.ebiom.2022.103847
- Xu S, Wu B, Zhong B, et al. Naringenin alleviates myocardial ischemia/reperfusion injury by regulating the nuclear factor-erythroid factor 2-related factor 2 (Nrf2)/System xc-/glutathione peroxidase 4 (GPX4) axis to inhibit ferroptosis. Bioengineered. 2021;12(2):10924–10934. doi: 10.1080/21655979.2021.1995994
- Song X, Zhu S, Chen P, et al. AMPK-Mediated BECN1 phosphorylation promotes ferroptosis by directly blocking system X(c)(-) activity. Curr Biol. 2018;28(15):2388–99.e5. doi: 10.1016/j.cub.2018.05.094
- Wang L, Liu Y, Du T, et al. ATF3 promotes erastin-induced ferroptosis by suppressing system Xc(). Cell Death Diff. 2020;27(2):662–675. doi: 10.1038/s41418-019-0380-z