ABSTRACT
Colorectal cancer (CRC) is the third most common cancer worldwide. In the United States alone, CRC was responsible for approximately 52,550 deaths in 2023, with an estimated 153,020 new cases. CRC presents with synchronous peritoneal spread in 5–10% of patients, and up to 20–50% of patients with recurrent disease will develop metachronous colorectal cancer peritoneal metastatic (CRC-PM) disease. Eradication of the tumor, tumor margins and microscopic residual disease is paramount, as microscopic residual disease is associated with local recurrences, with 5-year survival rates of less than 35%. The success of resection and reduction of residual disease depends on the accuracy with which cancer cells and normal tissue can be intra-operatively distinguished. Fluorescence Molecular Imaging (IFMI) and tumor-targeted contrast agents represent a promising approach for intraoperative detection and surgical intervention. Proper target selection, the development of scalable imaging agents and enhanced real-time tumor and tumor microenvironment imaging are critical to enabling enhanced surgical resection. LGR5 (leucine-rich repeat-containing G-protein-coupled receptor 5), a colonic crypt stem cell marker and the receptor for the R-spondins (RSPO) in the Wnt signaling pathway, is also expressed on colorectal cancer stem cells (CSC) and on CRC tumors and metastases, suggesting it could be a useful target for imaging of CRC. However, there are numerous diverging reports on the role of LGR5 in CRC therapy and outcomes. Herein, we report on the synthesis and validation of a 37 amino acid RSPO1-mimetic peptide, termed RC18, that was specifically designed to access the R-spondin binding site of LGR5 to potentially be used for interoperative imaging of CRC-PM. The receptor-binding capabilities of the RC18 indicate that direct interactions with LGR5 neither significantly increased LGR5 signaling nor blocked RSPO1 binding and signal transduction, suggesting that the RSPO1-mimetic is functionally inert, making it an attractive contrast agent for intraoperative CRC-PM imaging.
Introduction
Colorectal cancer (CRC) is a common and lethal disease accounting for ~9% of all cancer deaths in the USA. Unfortunately, despite advances in systemic therapy and oncologic surgical technique, recurrence of CRC is seen in approximately 30–40% of patients within the first 2 years following surgery. Most concerning, this includes approximately 25% of patients with theoretically curable node-negative, non-metastatic Stage I and II disease. Efforts to reduce this burden have been hindered by the lack of effective diagnostics, underpinned by gaps in our understanding of molecular drivers of cancer recurrence. While systemic therapy is important, eradication of microscopic residual disease at the time of surgery is paramount, as microscopic residual disease is associated with increased local recurrence, and a 5-year survival rate of less than 35% [Citation1]
The success of oncologic resection depends on the accuracy by which cancer cells and normal tissue can be intra-operatively distinguished. Unfortunately, neither current radiological imaging, such as MRI and CT, nor human vision, unaided or optically magnified, can effectively define surgical margins, micro metastases or residual disease. Modern surgery is therefore often confronted by these limitations, leading to surgical uncertainty, complicating decision-making and prolonging the surgical procedure. Complete removal of the tumor improves prognosis and decreases recurrence of disease for many cancer types (e.g. [Citation2–4]). Loco-regional tumor recurrence that results from dissemination of cancer cells into the peritoneal cavity, or as a result of a positive margin of resection, significantly and negatively impacts the prognosis of patients with CRC [Citation5–7]. In the absence of real-time feedback during surgery, only postoperative inspection of tissue specimens with histopathology can identify either complete resection or the presence of positive margins indicative of an incomplete excision. The uncertainty about resection margins directs excision of large volumes of healthy tissue around the tumor, leading to irreversible tissue damage and postoperative complications. Thus, real-time intraoperative feedback can improve decision-making, management, and prognosis. Unfortunately, preoperative and intraoperative characteristics defining and predicting microscopic or very low-volume peritoneal metastases at the time of primary surgery are still unclear, and as a result, the real-time morphological identification of high-risk groups and the application of effective management strategies in the early stage of the disease remain a challenge.
These challenges can be overcome through the use of Intraoperative Fluorescence Molecular Imaging (IFMI). IFMI makes use of fluorescently labeled moieties, including dyes, nanoparticles, peptides or antibodies, to enable real-time imaging of either the vasculature and/or tumor markers (e.g. [Citation8,Citation9]). Stratification based on imaging biomarkers can help determine which individuals are suited for preventive or therapeutic intervention, as well as to identify residual disease after resection. In this scenario, structural and molecular imaging can aid in treatment decisions based on the in vivo visualization of the location and extent of the disease process and can yield important information on the loco-regional, physiological, biochemical and biological processes involved.
Benefits of using peptides as IFMI contrast agents, compared to antibodies for example, include low toxicity, low or no immunogenicity, high specificity and increased tissue permeability due to their small size (<2 kDa), facilitating their delivery to cancer cells and their scalable synthesis. In 2015, the Food and Drug Administration (FDA) released its guidance for near-infrared (NIR) analytical procedures (FDA-2015-D-0868) and approved IRDye800CW as an NIR fluorophore for clinical use. Current examples of preclinical fluorescence imaging of tumors using peptides include EMI-137 targeting c-Met and QRHKPRE targeting EGFR, which provide the feasibility for real-time localization of subclinical disease localization in preclinical imaging of subclinical disease [Citation10–12]
Recently, de Valk et al. reported the first-in-human investigation of an NIR fluorescent peptide (cRGD-ZW800-1) for detection of colon carcinomas detection in 23 patients during laparoscopic surgery [Citation13], highlighting the translational prospect of peptide-based IFMI. We (e.g. [Citation14]) have investigated the feasibility of a novel multi-modal optical imaging approach using high-resolution optical coherence tomography (OCT) and high-sensitivity fluorescence laminar optical tomography (FLOT) for three-dimensional structural and molecular imaging. Quantitative structural (scattering coefficient) and molecular (relative enzyme activity) parameters were obtained from OCT and FLOT images for multi-parametric analysis. This multi-modal imaging method has demonstrated the feasibility for more accurate diagnosis, compared to either modality alone, with a sensitivity of 88.23% and a specificity of 82.35%.
Most CRC cases result from dysregulated Wnt signaling, resulting in the induction of tumor-related genes, such as cyclin D1, via a canonical TCF/LEF binding site in the promoter [Citation15], and deregulation of Wnt signaling is an important modulator of colon cancer stem cells (CSC). LGR5 (leucine-rich repeat-containing G-protein-coupled receptor 5), the receptor-target of R-spondin (RSPO), is an agonist of canonical Wnt/β-catenin signaling.
We and others have shown that LGR5 expression, an adult stem cell marker of the intestinal tract, liver and ovaries, is increased in both primary CRC and colorectal cancer peritoneal (CRC-PM) tumors, with minimal expression in most normal tissue, making LGR5 an appealing target for IFMI. However, there are significant controversies regarding the functional role of LGR5 in CRC, in general, and CRC intervention, specifically, with the plasticity of LGR5 being a hallmark of the receptor [Citation16]. For example, the de Savage lab found that ablating LGR5-positive cells slowed tumor growth but did not lead to significant regression [Citation17]. Using mouse models of CRC, the Fumagalli group reported that LGR5-expressing CRC cells were absent in circulating tumor cells but were found in metastatic lesions [Citation18]. In addition, cell lineage tracking experiments in vivo indicated that LGR5 expressing CRC cells can adopt a dormancy phenotype in the context of chemotherapy [Citation19]. With respect to clinical CRC management, our studies and others have reported that LGR5 expression tracked with better outcomes (e.g. [Citation20,Citation21]). Conversely, other studies concluded that LGR5 expression predicated poorer outcomes (e.g. [Citation22]). These and many other studies emphasize the complexities associated with LGR5 expression and function in CRC.
We hypothesized that a small, fluorescently labeled peptide mimetic that spans the RSPO1-binding site of LGR5 may be a useful moiety for identifying LGR5 positive cells via interoperative imaging for CRC-PM. Herein, using known LGR5/RSPO1 ×-ray crystallographic-binding data, we designed, synthesized (manuscript in preparation) and validated a 37 amino acid RSPO1 mimetic, termed RC18. Our data indicate that the RC18 RSPO1-mimetic peptide binds to LGR5, both as an immobilized protein as well as an expressed membrane receptor on cells. Binding of RC18 to LGR5 produced minimal impacts on LGR5 function as assessed using a TOPFLASH reporter cell line. Given the controversies surrounding the role of LGR5 in patient outcomes, the relatively minimal impact of RC18 on LGR5 signaling suggests that RC18 may be a safe and effective intraoperative fluorescent contrast agent for imaging CRC and CRC-PM tumors [Citation20].
Methods
Peptide mimetic design
LGR5-binding interactions with RSPO1 occur through cysteine-rich Furin 1 (Fu1, residues 34–95) and Furin 2 domains (Fu2, residues 97–143) (), as adapted from PDB accession code 4KNG. The design of RSPO1 mimetics was predicated on the binding motifs described above. Two components were prepared, corresponding to the right Fu2 96K – K113 Fu2 cysteine knot and the left Fu1 77P – I95 loop (). These were synthesized by solid-phase peptide synthesis (SPPS) using a CEM Liberty PRIME automated microwave peptide synthesizer (CEM Corp.) on 0.19 mmol/g Rink Amide ProTide LL (Low Loading) resin. Deprotection was performed with 25% pyrrolidine in N,N-dimethylformamide (DMF). Coupling reactions were performed in DMF for 60 sec at 105°C. Cleavage was performed at room temperature and the peptides were purified using reverse phase HPLC (Waters 2545 binary pump, MeCN/H2O gradient) with a preparative Phenomenex Gemini-C18 column and the collected fractions lyophilized overnight. Peptide analysis was performed using HPLC-LCMS (manuscript in preparation).
Figure 1. Interactions with the LGR5 ectodomain that informed RSPO1 mimetic design. a) Interactions of the cysteine-rich domain of RSPO1 in the Furin-1 region (amino acids 77–113) of LGR5. Surface and ribbon depictions of residues P77-K113 adapted from X-ray crystal structure PDB accession code 4KNG. Important interacting residues R87, F106 and F110 are shown in space filling. b) the structure of the 37 amino acid contrast agent is shown.
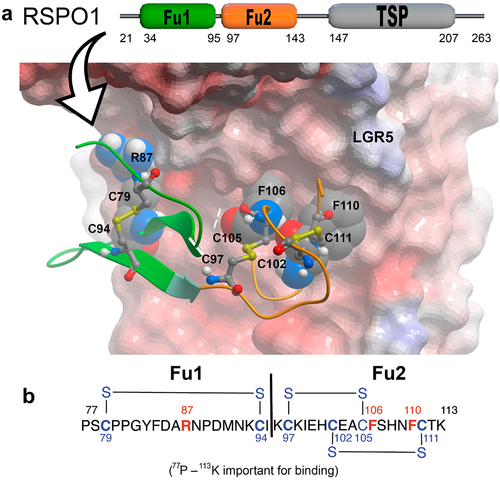
Figure 2. Synthesis of the RPSO1 mimetic constructs. a) Click chemistry was used to join the “left” (RC16) and “right” (RC06/07) side components to form the full-length mimetic peptide, RC18. b) The mutant peptide (RC21) contains the amino acid substitutions shown in red. c) Structure of the Cy5-based fluorescent dyes (sulCy5) used to label the mimetics.
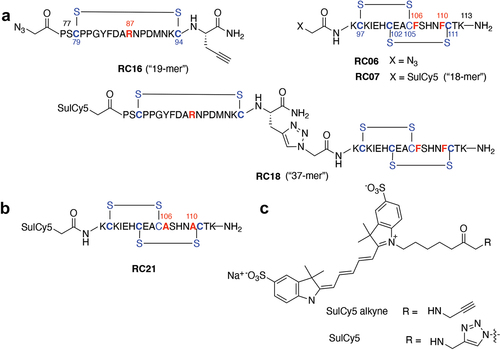
LGR5 receptor-binding enzyme linked immunosorbent assay (ELISA)
Ligand binding was assessed using an ELISA assay. Briefly, 96 well ELISA plates (R&D Systems # DY008) were coated with 100 μL of recombinant LGR5 (2ug/mL, R&D Systems, # 8078-GP) in PBS, covered, and incubated overnight at room temperature (RT). The wells were washed three times with Wash Buffer (R&D Systems, # DY464505) and briefly dried. The wells were then blocked using 300 μL/well of Reagent Diluent (R&D) for 1 h at RT, followed by washing three times with Wash Buffer. Recombinant R-spondin 1 (RSPO1, R&D Systems, #8078-GP) was reconstituted in PBS at a concentration of 2 ng/ml. The RC peptides were reconstituted in PBS at concentration of 1 mM. The reconstituted RSPO1 and the RC compounds were pipetted into 0.5 μL Eppendorf tubes and stored at −80°C.
For receptor-ligand binding assays, 100 μL/well of the recombinant RSPO1 at dilutions of 2 ng/mL, 1 ng/mL, 0.5 ng/mL, 0.25 ng/mL, 0.12 ng/mL, 0.06 ng/mL, 0.03 ng/mL were added and incubated for 2 h at RT (room temperature). The wells were washed three times with Wash Buffer and briefly dried. 100 μL of Detection Antibody (R&D Systems, # DY464505) in Reagent Diluent (R&D Systems, # DY464505) was added to each well, covered and incubated for 2 h at RT. Following three washes with Wash Buffer, 100 μL/well of Streptavidin-HRP (R&D Systems, # DY464505) at a 1:10 dilution was added and incubated for 20 min at RT. After aspiration, the wells were washed three times and briefly dried. 100 μL of the Substrate solution (R&D Systems, # DY464505) was added to each well, wrapped with foil and incubated for 20 min at RT. Following aspiration 50 μL of Stop solution (R&D Systems, # DY464505) was added and the plates gently tapped to ensure mixing. Finally, wells were aspirated and 50 μL of Stop solution (R&D Systems, # DY464505) added. Optical density was determined using a Victor 2 plate reader (Wallach) at 450 nm.
For the receptor-ligand competition assays, the RC compounds were added to the RSPO1 solution at the concentrations described in the Results and the binding assays were carried out as described above.
Cell lines and transfections
The CHO-K1 cells (CCL-61, ATCC), which lack LGR5 expression [Citation23] and the CRC HT29 cells and SW620 cells, which express LGR5, were acquired from the Georgetown-Lombardi Cell and Tissue Shared Resource and maintained in DMEM (10% FBS, 1% L-glutamine, 1% Pen/Strep) at 37°C in a CO2 incubator. The myc-tagged LGR5 expression vector was from Origene (#RC212825) and pEGFP-N1 was from Clontech.
Transfections of CHO-K1 cells were performed in 24 well plates (Fisher). Cells were seeded at 4 × 104 cells per well in 0.5 ml of complete growth medium and allowed to recover overnight to obtain a cell confluency of 50 ~ 80% on the day of transfection. For transfections, 1 μL of Lipofectamine LTX (Invitrogen, #15338100) was added to 500 ng of the myc-LGR5 and 15 ng of pEGFP-N1 DNA vectors in 100 μL of serum-free media, mixed gently and incubated for 25 min at RT. Immediately before transfection, fresh medium was added, and 100 μL of the Lipofectamine-DNA solution was added to the cells, mixed gently and cultured for 18–24 h.
β-catenin signaling reporter assays
HEK293 Super TOPFLASH (STF) cells, which stably express the SuperTOPFLASH reporter gene containing a 7× TCF/LEF motif, were used to estimate β-catenin transcriptional activity and were purchased from American Type Culture Collection (ATCC # CRL-3249). The cells were maintained in DMEM supplemented with 10% FBS at 37°C in a CO2 incubator. 1.0 × 104 cells were plated per well in a flat bottom 96-well plate. The next day, cells were treated with the indicated concentrations of the RSPO1 mimetics, Wnt3a (R&D Systems, # 5036-WN) or recombinant-human RSPO1 (rhRSPO1, R&D Systems, #4645-RS). Forty-eight hours after treatment, cells were lysed in 1× Passive Lysis Buffer (Promega) and luciferase activity was quantified with the Luciferase Assay System (Promega). Bradford assays were used to quantify protein concentration, and the luciferase signals were standardized to the absorbance values from the Bradford assay.
Western blotting
Protein extracts were separated on 4–20% Tris-glycine gels and electro-blotted onto PVDF membranes as previously described [Citation24,Citation25]. Antibodies against LGR5 (Origene #UM800104CF), myc-tag (Cell Signaling #2276) and β-actin (Cell Signaling Technology, #4967) were used according to the manufactures’ specifications. Densitometry was performed using an Amersham 600 imager (Amersham) and ImageJ (RRID:SCR003070) as previously described [Citation25].
Cell binding assays
Binding of the RC compounds was performed using CHO-K1 Control cells and LGR5-transfected-CHO-K1 cells on gelatin-coated glass cover slips. The cover slips (Fisher, #1254582) were first sterilized, placed in 24 well plates and incubated in 400 μL of a 0.1% gelatin (Sigma, #G9391) solution in PBS for 30 min at RT. The gelatin was removed and replaced with complete medium prior to seeding with cells and transfection as described above. The transfection solution was removed, and the cells were fixed using 4% PFA. For direct-binding assays, the RC compounds were added directly to the well at a final concentration of 5 uM and incubated for 90 min in the dark followed by three washes of PBS. For the competition assays, recombinant RSPO1 (40 pM) was added to the wells with or without the RC compounds (5uM) and incubated for 90 min in the dark, followed by three washes of PBS.
Fluorescence microscopy
The slides were imaged on an Olympus microscope in the Georgetown-Lombardi Microscopy and Imaging Shared resource. Fluorescence intensity quantification was performed using ImageJ analysis software (RRID:SCR003070).
Immunofluorescent staining
The cells were fixed with 4% paraformaldehyde (Sigma, # 100496) for 20 min at RT. The wells were washed three times with PBS for 5 min each. Blocking was performed with BSA 1% for 30 min and followed with three washes with PBS for 5 min each. Next, the cells were incubated with primary antibodies against LGR5 (Origene #UM800104CF) or myc-tag (Cell Signaling #2276) at the dilutions recommended by the manufacturer for 90 min at RT. The cells were washed three times with PBS for 5 min each. Alexa Fluor 594 was used as the secondary antibody for 1 h at RT. After three washes with PBS for 5 min each, the coverslips were mounted onto slides and images were acquired using a Zeiss confocal microscope in the Georgetown-Lombardi Microscopy and Imaging Shared Resource.
Results
RSPO1/LGR5 interaction modeling and peptide synthesis
Binding of RSPO1 to the ectodomain of LGR5 involves primarily the Furin 1 domain (Fu1, residues 34–95) and the Furin 2 domain (Fu2, residues 97–143) of RSPO1 [Citation26]. We hypothesized that a peptide that encompassed both RSPO1 binding sites on LGR5 would bind to the receptor. The design of RSPO1 peptide mimetics used in the present study was based on the crystal structure of the ectodomain of LGR5 in complex with a RSPO1 fragment spanning the Fu1 – Fu2 domains (residues 31–145) at 2.0 Å resolution (PDB accession code 4KNG) [Citation27]. The following peptides were synthesized as described below: RC16, which targets the left-side RSPO1 binding site on LGR5 and RC06/07 that targets the right side. The full-length 37-mer peptide, termed RC18, spans both regions of the receptor.
Important interactions occur in the77p – 113K sequence within two structurally distinct motifs. The first, “right side” motif is formed by the96K – K113 Fu2 segment, which contains a cysteine knot formed by two interlaced97C – C105 and102C – C111 disulfide bonds (). This region includes a key106FSHNF110 sequence that is situated within a highly conformationally constrained loop of the cysteine knot. The F106 and F110 residues of this segment function as a clamp on the LGR5 A190 residue (). The second, “left side” binding motif is comprised of the77P – I95 loop between the Fu1 domain79C – C94 disulfide bond. This loop contains the key R87 residue and proximal residues D85, N88 and N92 (). When bound to LGR5, the two motifs are aligned by means of a twist through a short two-residue strand between the C94 and C97 residues of the termini of disulfide bridges in the left Fu1 and right Fu2 segments.
The design of RSPO1 mimetics was predicated on the binding motifs described above. Two components were prepared corresponding to the right96K – K113 Fu2 cysteine knot and the left Fu1 77P – I95 loop. These were synthesized by solid-phase peptide synthesis (SPPS) (manuscript in preparation). The amino termini of both peptides were derivatized as their azidoacetyl amides. The C-terminus of the right Fu2 96K – K113 peptide was prepared in its amide form (peptide RC06) and the C-terminus of the left Fu1 peptide had the I95 residue replaced by (S)-2-aminopent-4-ynamide (RC16, ). The right side RC06 peptide was amino terminally labeled with Sulfo-Cy5 dye to yield RC07 by coupling the azido functionality with Sulfo-Cy5 alkyne using Huisgen alkyne-azide click cycloaddition chemistry () [Citation28]. Similarly, the amino-terminal azide of the left side RC16 peptide was converted to a Sulfo-Cy5 derivative and the C-terminal alkyne functionality was coupled with the amino-terminal azide of RC06 to yield the 37-mer peptide RC18. A variant of RC07 was prepared in which the key F106 and F110 residues were replaced with A106 and A110 residues, respectively (termed RC21) ().
Peptide binding using an LGR5 enzyme linked immunosorbent assay (ELISA)
In order to assess RC compound/receptor interactions, an ELISA assay was developed, using recombinant human LGR5 and R-spondin 1 (RSPO1). Briefly, human LGR5 was adhered to 96 well ELISA plates and human RSPO1 was added and quantified using an RSPO1 detection antibody as described in the Methods section. A range of concentrations were tested tested with 40 pM RSPO1, the approximate half-maximal binding dose to LGR5 (Supplementary Figure S1). Next, increasing concentrations of the fluorescently labeled RC compounds were added in the presence of 40 μM RSPO1 and the changes in RSPO1/LGR5 binding were assessed. As shown in , RC18 reduced RSPO1 binding over the course of the assay, with the “left side” (RC16) and “right side” (RC07) less able to consistently inhibit RSP01 binding across the range of concentrations. These data suggest a bivalency in RC18 binding, while both the left and right halves of the mimetic interact with LGR5, the combination of the two receptor-binding domains increased this interaction to levels that were significant at certain concentrations.
Figure 3. RSPO1 binding assays. a) ELISA data showing dose-response competition of recombinant human RSPO1 binding to human LGR5 coated plates by RC07, RC16 and RC18. b) brightfield (top) and fluorescent (bottom) images of direct binding of the fluorescent RC18 contrast agent to CHO-K1 (LGR5-negative) cells (left) vs. HT29 (LGR5-positive) human colon cancer cells (right). * p < 0.05 (Tukey’s multiple comparisons test).
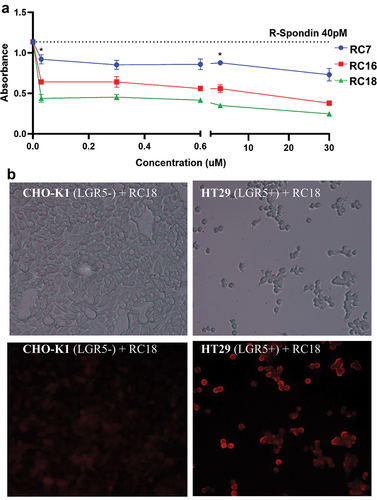
RC binding to LGR5 receptor in cells
We next interrogated RC binding to cells. CHO-K1 cells, which lack LGR5 expression [Citation23] and the LGR5 positive human CRC cell line, HT29, were incubated with RC18 (5uM) and imaged by fluorescence microscopy. As seen in , RC18 binding was significantly increased in the HT29 cells vs. CHO-K1.
In order to better define the binding of RC18 to LGR5, the CHO-K1 cells were co-transfected with a myc-tagged LGR5 expression vector and CMV-GFP (termed (CHO-K1-LGR5), or CMV-GFP alone as control (CHO-K1 Control). LGR5 expression was confirmed by immunoblotting (Supplementary Figures S2, 3) and by confocal microscopy of fixed cells, using either RC18 (), an anti-myc antibody () or an anti-LGR5 antibody (Supplementary Figure S4).
Figure 4. Cell Binding Assays. Confocal microscopy of fluorescent RC18 binding to a) CHO-K1 control vector-transfected cells or b) CHO-K1 cells transfected with LGR5 (CHO-K1-LGR5) and stained with RC18. c) Confocal imaging of CHO-K1-LGR5 stained with an anti-Myc-tag Alexafluor 594 antibody (Ab) d) Binding of RC18 (top) or the RC21 mutant fluorescent peptide containing phenylalanine to alanine mutations (F106A/F110A) (bottom) to CHO-K1 control cells and CHO-K1-LGR5 cells e) Quantification of fluorescence intensity the cells in (D).
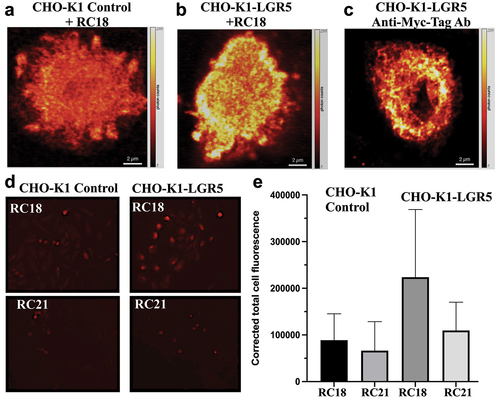
RSPO1 competition experiments were performed using LGR5-positive SW620 human CRC cells and the CHO-K1 control cells. The cells were incubated with RC18 (5uM) with and without recombinant RSPO1 (40 pM) for 90 min in the dark, washed three times with PBS and imaged by fluorescent microscopy (). RSPO1 significantly reduced the binding of RC18 to LGR5 on the SW620 cells, while no effects were seen in the CHO-K1 Control cells ().
Figure 5. RC18 cell binding in the absence and presence of human recombinant RSPO1. a) Fluorescence microscopy of SW620 CRC cells (top) vs. CHO-K1 (LGR5-) cells (bottom). b). RSPO1 reduced RC18 binding by over 50% in SW620 cells but by less that 2% in the LGR5-negative CHO cells by ImageJ. (*p < 0.05; N = 4).
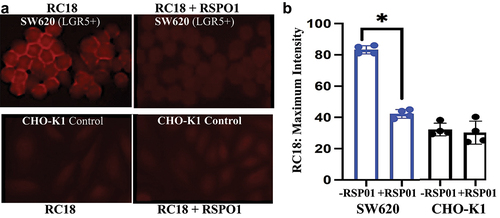
RC18-LGR5 intracellular signaling
We next investigated the effects of the RSPO1 mimetics on Wnt/β-catenin signaling. To do so, HEK293 Super TOPFLASH (STF) cells were treated with different doses of the RC18 RSPO1 mimetic, recombinant-human RSPO1 (rhRSPO1) or Wnt3a. Treatment of the HEK293 STF cells with Wnt3a and rhRSPO1 led to a statistically significant increase in Super TOPFLASH luciferase activity, an estimate of β-catenin transcriptional activity (). Conversely, treatment with varying doses of the RC18 RSPO1 mimetics did not lead to a statistically significant change in luciferase activity, suggesting these mimetics do not activate Wnt signaling (). We next assessed whether the RC18 RSPO1 mimetic would interfere with rhRSPO1-dependent signaling through LGR5. RC18 did not statistically significantly interfere with RSPO1-mediated β-catenin activity at any of the doses tested ().
Figure 6. β-catenin signal transduction in HEK293 super TOPFLASH cells. a) Induction of Wnt signaling using two different syntheses of RC18 (mimetic, blue and red bars) vs. recombinant human Wnt3a or recombinant human RSPO 1 (green bars) as positive controls, at the concentrations shown. b). Competition assays with RC18 added to the cells 10 minutes before addition of recombinant human RSPO1 at the concentrations shown. The data are percent maximum activity. Luciferase activity was corrected for protein abundance.
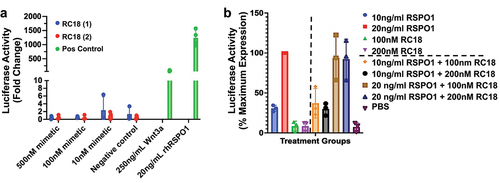
Discussion
Colorectal cancer (CRC) remains a leading cause of cancer-related morbidity and mortality worldwide, underscoring the urgent need for advancements in surgical techniques that can improve patient outcomes. Surgical resection is the cornerstone of curative treatment for CRC, but its success critically depends on the complete removal of malignant tissues while sparing healthy ones. This challenge is compounded by the limitations of conventional imaging modalities, which often struggle to delineate tumor margins accurately during surgery. As a result, there is a significant risk of either incomplete tumor resection or unnecessary removal of healthy tissue, each of which carries implications for recurrence rates, patient survival, and quality of life. To address these challenges, the field of intraoperative imaging has emerged as a promising avenue for enhancing surgical precision through the real-time visualization of tumor tissues.
IFMI is a rapidly developing area in recent years and highly translatable to the clinic in the form of wide-field imaging [Citation29], mesoscopic-scale imaging [Citation30], endoscopy [Citation31], laparoscopy [Citation13] and needle imaging devices [Citation32] and promises to enhance the clinician’s capability to diagnose diseases early. Progress in the design, synthesis, and testing of novel contrast agents with high brightness and targeting specificity will result in better performance for cancer detection and resection.
We capitalized on the fact that LGR5 is known to be dysregulated in a majority of CRC cases, including patients with CRC-PM. Targeting LGR5 with peptide mimetics specifically designed for intraoperative FMI represents a novel approach that leverages the molecular insights into CRC pathogenesis. By binding specifically to cells expressing LGR5, these probes may illuminate not only the primary tumor mass but also satellite lesions and micrometastases, thereby enhancing the surgeon’s ability to achieve a more complete and accurate resection.
Zhang et al. have reported on an RSPO-2 antibody conjugate that lacks LGR-binding capabilities and has been shown to improve in liver regeneration [Citation33] and Kwak et al. used phage display to identify a seven amino acid peptide, IPQILSI, that possessed LGR5 binding capabilities for gastric cancer, however the epitope to which the peptide bound was not stated [Citation34]. However, as discussed earlier, there are significant complexities associated with the role of LGR5 in CRC and it is not clear if either LGR5 expression or LGR-associated signaling should modify in the context of CRC.
The RC18 RSPO1-mimetic peptide presents certain advantages and could be a useful candidate for future clinical imaging of CRC. For example, unlike other potential targets, such as the widely expressed Epidermal Growth Factor Receptor, expression of LGR5 is more limited, is generally associated with the gastrointestinal tract [Citation35] and LGR5 expression is found in gastrointestinal, ovarian cancers, breast and some squamous cell carcinomas [Citation36–39]. Modifications to the length and/or amino acid composition of the RC18 peptide can be rapidly integrated and synthesis of the peptide is scalable for production and does not require cell culture. Notably, no significant impact on RSPO1 signaling was seen with the RC18 mimetic. This is an important and novel feature of the RC18 contrast agent, as the exact role of LGR5 activity in CRC progression and perhaps most notably, CRC treatment, remains highly controversial [Citation16,Citation20]. For example, analysis of publicly available data indicated that LGR5 expression tracked with decreased overall survival and disease-free survival (e.g. [Citation40,Citation41]); however, our studies and others found a positive correlation between LGR5 expression and survival [Citation20,Citation42] and therapeutic targeting of LGR5-expressing cells via gene silencing or antibody-drug conjugates was found to increase the chemoresistant profile of CRC cells [Citation43].
Further enhancement of RC18 contrast with NIR derivatives that match the detection capabilities of existing surgical instrumentation while providing improved in vivo imaging properties are planned. In this area, future directions include the in vitro and in vivo testing of RC18 against LGR5 and additional targets (e.g. LGR4 [Citation22]), as well as modifications with next-generation fluorescent probes, such as FNIR-tag derivatives, which exhibit improved in vivo properties and reduced off-target uptake [Citation44–46].
Competing interests
MP receives royalties and is a consultant of TheraLink Technologies, Inc. CA is a consultant for Palisades Therapeutics.
RC Paper Supplementary Figs Original Submission.1.pdf
Download PDF (2 MB)Acknowledgements
We would like to thank Dr. Qingyun Liu, University of Texas Health Science Center for help with LGR5 expression in CHO-K1 cells, the Lombardi Microscopy and Imaging Shared Resource, the Lombardi Tissue Culture and Biobanking Shared Resource and the Lombardi Genomics and Epigenomics Shared Resource. The Lombardi Microscopy and Imaging Shared Resource, the Lombardi Tissue Culture and Biobanking Shared Resource and the Lombardi Genomics and Epigenomics Shared Resource. This research was supported in part by the Intramural Research Programs of the National Cancer Institute, National Institutes of Health.
Disclosure statement
No potential conflict of interest was reported by the author(s).
Supplementary material
Supplemental data for this article can be accessed online at https://doi.org/10.1080/15384101.2024.2364578
Additional information
Funding
References
- Landmann RG, Weiser MR. Surgical management of locally advanced and locally recurrent colon cancer. Clin Colon Rectal Surg. 2005 Aug;18(3):182–189. doi: 10.1055/s-2005-916279
- Moran MS, Truong PT. Intraoperative accelerated partial breast irradiation: caution still warranted. Int J Radiat Oncol Biol Phys. 2014 Jul 1;89(3):496–498. doi: 10.1016/j.ijrobp.2014.01.034
- Camp ER, McAuliffe PF, Gilroy JS, et al. Minimizing local recurrence after breast conserving therapy using intraoperative shaved margins to determine pathologic tumor clearance. J Am Coll Surg. 2005 Dec;201(6):855–861. doi: 10.1016/j.jamcollsurg.2005.06.274
- Mendenhall WM, Morris CG, Amdur RJ, et al. Radiotherapy alone or combined with surgery for salivary gland carcinoma. Cancer. 2005 Jun 15;103(12):2544–2550. doi: 10.1002/cncr.21083
- Jayne DG, Fook S, Loi C, et al. Peritoneal carcinomatosis from colorectal cancer. Br J Surg. 2002 Dec;89(12):1545–1550. doi: 10.1046/j.1365-2168.2002.02274.x
- Lemmens VE, Klaver YL, Verwaal VJ, et al. Predictors and survival of synchronous peritoneal carcinomatosis of colorectal origin: a population-based study. Int J Cancer. 2011 Jun 1;128(11):2717–2725. doi: 10.1002/ijc.25596
- Segelman J, Granath F, Holm T, et al. Incidence, prevalence and risk factors for peritoneal carcinomatosis from colorectal cancer. Br J Surg. 2012 May;99(5):699–705. doi: 10.1002/bjs.8679
- Mieog JSD, Achterberg FB, Zlitni A, et al. Fundamentals and developments in fluorescence-guided cancer surgery. Nat Rev Clin Oncol. 2022 Jan;19(1):9–22. doi: 10.1038/s41571-021-00548-3
- Lauwerends LJ, van Driel P, Baatenburg de Jong RJ, et al. Real-time fluorescence imaging in intraoperative decision making for cancer surgery. Lancet Oncol. 2021 May;22(5):e186–e195. doi: 10.1016/S1470-2045(20)30600-8
- de Jongh SJ, Voskuil FJ, Schmidt I, et al. C-Met targeted fluorescence molecular endoscopy in Barrett’s esophagus patients and identification of outcome parameters for phase-I studies. Theranostics. 2020;10(12):5357–5367. doi: 10.7150/thno.42224
- Vries HM, Bekers E, van Oosterom MN, et al. c-MET receptor-targeted fluorescence on the road to image-guided surgery in penile squamous cell carcinoma patients. J Nucl Med. 2022 Jan;63(1):51–56. doi: 10.2967/jnumed.120.261864
- Zhou J, Joshi BP, Duan X, et al. EGFR overexpressed in colonic neoplasia can be detected on wide-field endoscopic imaging. Clin Transl Gastroenterol. 2015 Jul 16;6(7):e101. doi: 10.1038/ctg.2015.28
- de Valk KS, Deken MM, Handgraaf HJM, et al. First-in-human assessment of cRGD-ZW800-1, a zwitterionic, integrin-targeted, near-infrared fluorescent peptide in colon carcinoma. Clin Cancer Res. 2020 Aug 1;26(15):3990–3998. doi: 10.1158/1078-0432.CCR-19-4156
- Tang Q, Wang J, Frank A, et al. Depth-resolved imaging of colon tumor using optical coherence tomography and fluorescence laminar optical tomography. Biomed Opt Express. 2016 Dec 1;7(12):5218–5232. doi: 10.1364/BOE.7.005218
- Shtutman M, Zhurinsky J, Simcha I, et al. The cyclin D1 gene is a target of the beta-catenin/LEF-1 pathway. Proc Natl Acad Sci USA. 1999 May 11;96(10):5522–5527. doi: 10.1073/pnas.96.10.5522
- Morgan RG, Mortensson E, Williams AC. Targeting LGR5 in colorectal cancer: therapeutic gold or too plastic? Br J Cancer. 2018 May;118(11):1410–1418. doi: 10.1038/s41416-018-0118-6
- de Sousa e Melo F, Kurtova AV, Harnoss JM, et al. A distinct role for Lgr5(+) stem cells in primary and metastatic colon cancer. Nature. 2017 Mar 29;543(7647):676–680. doi: 10.1038/nature21713
- Fumagalli A, Oost KC, Kester L, et al. Plasticity of Lgr5-negative cancer cells drives metastasis in colorectal cancer. Cell Stem Cell. 2020 Apr 2;26(4):569–578 e7. doi: 10.1016/j.stem.2020.02.008
- Ohta Y, Fujii M, Takahashi S, et al. Cell-matrix interface regulates dormancy in human colon cancer stem cells. Nature. 2022 Aug;608(7924):784–794. doi: 10.1038/s41586-022-05043-y
- Ihemelandu C, Naeem A, Parasido E, et al. Clinicopathologic and prognostic significance of LGR5, a cancer stem cell marker in patients with colorectal cancer. Colorectal Cancer. 2019 Nov 12;8(4):CRC11. doi: 10.2217/crc-2019-0009
- Jang BG, Kim HS, Chang WY, et al. Expression profile of LGR5 and its prognostic significance in colorectal cancer progression. Am J Pathol. 2018 Oct;188(10):2236–2250. doi: 10.1016/j.ajpath.2018.06.012
- AbdelMageed M, Ismail HTH, Olsson L, et al. Clinical significance of stem cell biomarkers EpCAM, LGR5 and LGR4 mRNA levels in lymph nodes of colon cancer patients. Int J Mol Sci. 2021 Dec 30;23(1):403. doi: 10.3390/ijms23010403
- Carmon KS, Gong X, Yi J, et al. LGR5 receptor promotes cell-cell adhesion in stem cells and colon cancer cells via the IQGAP1-Rac1 pathway. J Biol Chem. 2017 Sep 8;292(36):14989–15001. doi: 10.1074/jbc.M117.786798
- Naeem A, Harish V, Coste S, et al. Regulation of chemosensitivity in human medulloblastoma cells by p53 and the PI3 kinase signaling pathway. Mol Cancer Res. 2022 Jan;20(1):114–126. doi: 10.1158/1541-7786.MCR-21-0277
- Parasido E, Avetian GS, Naeem A, et al. The sustained induction of c-MYC drives nab-paclitaxel resistance in primary pancreatic ductal carcinoma cells. Mol Cancer Res. 2019 Sep;17(9):1815–1827. doi: 10.1158/1541-7786.MCR-19-0191
- Kim KA, Wagle M, Tran K, et al. R-Spondin family members regulate the Wnt pathway by a common mechanism. Mol Biol Cell. 2008 Jun;19(6):2588–2596. doi: 10.1091/mbc.e08-02-0187
- Peng WC, de Lau W, Forneris F, et al. Structure of stem cell growth factor R-spondin 1 in complex with the ectodomain of its receptor LGR5. Cell Rep. 2013 Jun 27;3(6):1885–1892. doi: 10.1016/j.celrep.2013.06.009
- Huisgen R. 1,3-dipolar cycloadditions. past and future. Angew Chem Int Ed Engl. 1963;2(10):565–598. doi: 10.1002/anie.196305651
- van Dam GM, Themelis G, Crane LM, et al. Intraoperative tumor-specific fluorescence imaging in ovarian cancer by folate receptor-alpha targeting: first in-human results. Nat Med. 2011 Sep 18;17(10):1315–1319. doi: 10.1038/nm.2472
- Tang Q, Nagaya T, Liu Y, et al. 3D mesoscopic fluorescence tomography for imaging micro-distribution of antibody-photon absorber conjugates during near infrared photoimmunotherapy in vivo. J Control Release. 2018 Jun 10;279:171–180. doi: 10.1016/j.jconrel.2018.04.027
- Nagengast WB, Hartmans E, Garcia-Allende PB, et al. Near-infrared fluorescence molecular endoscopy detects dysplastic oesophageal lesions using topical and systemic tracer of vascular endothelial growth factor a. Gut. 2019 Jan;68(1):7–10. doi: 10.1136/gutjnl-2017-314953
- Lorenser D, Quirk BC, Auger M, et al. Dual-modality needle probe for combined fluorescence imaging and three-dimensional optical coherence tomography. Opt Lett. 2013 Feb 1;38(3):266–268. doi: 10.1364/OL.38.000266
- Zhang Z, Broderick C, Nishimoto M, et al. Tissue-targeted R-spondin mimetics for liver regeneration. Sci Rep. 2020 Aug 18;10(1):13951. doi: 10.1038/s41598-020-70912-3
- Kwak MH, Yang SM, Yun SK, et al. Identification and validation of LGR5-binding peptide for molecular imaging of gastric cancer. Biochem Biophys Res Commun. 2021 Nov 26;580:93–99. doi: 10.1016/j.bbrc.2021.09.073
- Haegebarth A, Clevers H. Wnt signaling, lgr5, and stem cells in the intestine and skin. Am J Pathol. 2009 Mar;174(3):715–721. doi: 10.2353/ajpath.2009.080758
- Zhao Y, Feng F, Zhou YN. Stem cells in gastric cancer. World J Gastroenterol. 2015 Jan 7;21(1):112–123. doi: 10.3748/wjg.v21.i1.112
- Katoh M. Canonical and non-canonical WNT signaling in cancer stem cells and their niches: cellular heterogeneity, omics reprogramming, targeted therapy and tumor plasticity (Review). Int J Oncol. 2017 Nov;51(5):1357–1369. doi: 10.3892/ijo.2017.4129
- Lee S, Jun J, Kim WJ, et al. WNT signaling driven by R-spondin 1 and LGR6 in high-grade serous ovarian cancer. Anticancer Res. 2020 Nov;40(11):6017–6028. doi: 10.21873/anticanres.14623
- Kim H, Lee DH, Park E, et al. Differential epithelial and stromal LGR5 expression in ovarian carcinogenesis. Sci Rep. 2022 Jul 1;12(1):11200. doi: 10.1038/s41598-022-15234-2
- Chen Q, Zhang X, Li WM, et al. Prognostic value of LGR5 in colorectal cancer: a meta-analysis. PLOS ONE. 2014;9(9):e107013. doi: 10.1371/journal.pone.0107013
- Jiang Y, Li W, He X, et al. Lgr5 expression is a valuable prognostic factor for colorectal cancer: evidence from a meta-analysis. BMC Cancer. 2016 Jan 12;16(1):12. doi: 10.1186/s12885-015-1986-2
- Sato K, Uehara T, Iwaya M, et al. Correlation of clinicopathological features and LGR5 expression in colon adenocarcinoma. Ann Diagn Pathol. 2020 Oct;48:151587. doi: 10.1016/j.anndiagpath.2020.151587
- Posey TA, Jacob J, Parkhurst A, et al. Loss of LGR5 through therapy-induced downregulation or gene ablation is associated with resistance and enhanced MET-STAT3 signaling in colorectal cancer cells. Mol Cancer Ther. 2023 May 4;22(5):667–678. doi: 10.1158/1535-7163.MCT-22-0415
- Usama SM, Thapaliya ER, Luciano MP, et al. Not so innocent: Impact of fluorophore chemistry on the in vivo properties of bioconjugates. Curr Opin Chem Biol. 2021 Aug;63:38–45. doi: 10.1016/j.cbpa.2021.01.009
- Hernandez Vargas S, AghaAmiri S, Ghosh SC, et al. High-contrast detection of somatostatin receptor subtype-2 for fluorescence-guided surgery. Mol Pharm. 2022 Nov 7;19(11):4241–4253. doi: 10.1021/acs.molpharmaceut.2c00583
- Usama SM, Marker SC, Li DH, et al. Method to diversify cyanine chromophore functionality enables improved biomolecule tracking and intracellular imaging. J Am Chem Soc. 2023 Jul 12;145(27):14647–14659. doi: 10.1021/jacs.3c01765