Abstract
Objective: To improve vehicle safety in frontal collisions, the crash compatibility between the colliding vehicles is crucial. Compatibility aims to improve both the self and partner protection properties of vehicles. Although compatibility has received worldwide attention for many years, no final assessment approach has been defined.
Methods: Within the Frontal Impact and Compatibility Assessment Research (FIMCAR) project, different frontal impact test procedures (offset deformable barrier [ODB] test as currently used for Economic Commission for Europe [ECE] R94, progressive deformable barrier test as proposed by France for a new ECE regulation, moveable deformable barrier test as discussed worldwide, full-width rigid barrier test as used in Federal Motor Vehicle Safety Standard [FMVSS] 208, and full-width deformable barrier test) were analyzed regarding their potential for future frontal impact legislation. The research activities focused on car-to-car frontal impact accidents based on accident investigations involving newer cars. Test procedures were developed with both a crash test program and numerical simulations.
Results: The proposal from FIMCAR is to use a full-width test procedure with a deformable element and compatibility metrics in combination with the current offset test as a frontal impact assessment approach that also addresses compatibility.
Conclusions: By adding a full-width test to the current ODB test it is possible to better address the issues of structural misalignment and injuries resulting from high acceleration accidents as observed in the current fleet. The estimated benefit ranges from a 5 to 12 percent reduction of fatalities and serious injuries resulting from frontal impact accidents. By using a deformable element in the full-width test, the test conditions are more representative of real-world situations with respect to acceleration pulse, restraint system triggering time, and deformation pattern of the front structure. The test results are therefore expected to better represent real-world performance of the tested car. Furthermore, the assessment of the structural alignment is more robust than in the rigid wall test.
Supplemental materials are available for this article. Go to the publisher's online edition of Traffic Injury Prevention to view the supplemental file.
Introduction
Crash compatibility has long been promoted as a key component in improving vehicle safety. Although compatibility has received worldwide attention for many years, no final assessment approach has been defined. Frontal Impact and Compatibility Assessment Research (FIMCAR) was a research project completed in 2012 to address compatibility test procedures and was cofunded by the European Commission within the 7th Framework Programme. The objective of the project was to answer the remaining open questions identified in earlier projects (such as understanding the advantages and disadvantages of force-based metrics and barrier deformation–based metrics, confirmation of specific compatibility issues like structural interaction, investigation of force matching) and to finalize the test procedures required to assess compatibility. Within the project, the research activities focused on car-to-car frontal impact accidents. However, other configurations such as lateral impact, car-to-HGV (heavy goods vehicle) accidents, etc., were also considered to ensure that changes made to cars to improve their compatibility in frontal impacts are not detrimental for other impact types. The FIMCAR project harmonized its activities with the GRSP (working party on passive safety of UNECE [United Nations Economic Commission for Europe]) informal group on frontal impact and cooperated with the European Council for Automotive R&D (EUCAR) to involve relevant stakeholders.
Background
Improving road safety is one of the major goals of road authorities, vehicle manufacturers, rescue organizations, and research organizations, among others. Measures to improve safety are historically divided into the area of primary safety/ active safety (measures that help to avoid the occurrence of accidents) and secondary safety/passive safety (measures that help to reduce the consequences of accidents).
In the 27 European Union (EU27) member states, road fatalities are still a major cause of death, although important safety improvements have reduced the number of fatalities since 1990; see . It should be noted that almost 50 percent of the road fatalities in 2008 in the 27 European Union member states were car occupants ().
Fig. 1 Development of road accidents causing injuries and road facilities in EU27 (European Union Road Federation 2010) (color figure available online).
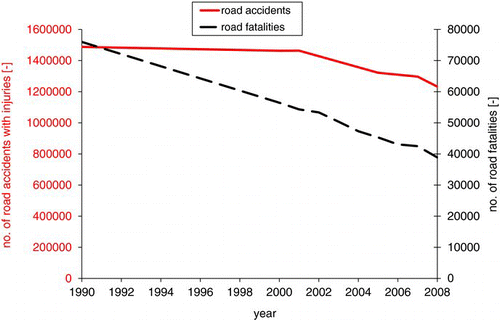
Fig. 2 Distribution of road fatalities among road user categories in 2008 in EU27 (European Union Road Federation 2010) (color figure available online).
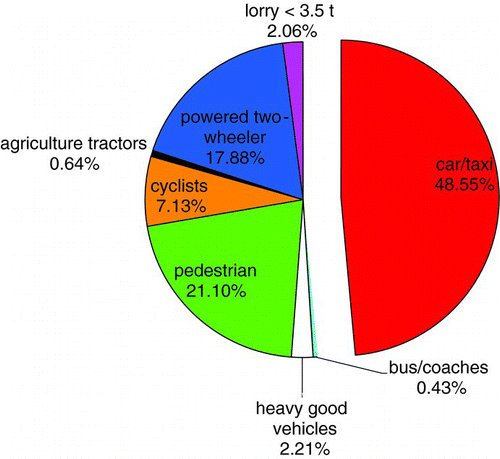
The passive safety capabilities of cars are mainly assessed by crash tests. Currently, different frontal test procedures are used in different regions of the world. The most important test procedures are as follows:
• | Offset test (40% of vehicle width) against a deformable element as currently used for homologation of cars in Europe (Economic Commission for Europe Regulation 94 [ECE R94]), the consumer information test program [European New Car Assessment Programme, Euro NCAP], the U.S. Insurance Institute for Highway Safety, and others. | ||||
• | Full-width test against a rigid wall as currently used for homologation of cars in the United States (Federal Motor Vehicle Safety Standard [FMVSS] 208), the consumer information program (U.S. New Car Assessment Program [US NCAP], homologation of cars in Japan and others. |
After introduction of these tests, in particular the offset test, the safety performance of cars in Europe has improved in terms of test results. However, it appears that cars rated good or excellent in the test programs do not always perform well in car-to-car accidents. This behavior was attributed as incompatibility between cars. It is this characteristic that was deemed important to assess and initiated different research activities.
Different test methods have been investigated in various regions, but the global consensus in the IHRA (International Harmonized Research Activity) compatibility working group (O’Reilly 2003) is that both an offset and a full-width test are needed to fully assess compatibility and frontal protection performance. Each region has unique compatibility issues related to their respective traffic fleets, but similar strategies and approaches can be observed.
Previous research work on compatibility (e.g., EUCAR Compatibility Project [EUCAR 2001], Enhanced European Vehicle-Safety Committee [EEVC] Working Group 15 [Faerber 2007], VC-COMPAT [Edwards et al. Citation2007] and other international and national research projects and working groups) has shown that the main issues for improving compatibility are as follows:
• | Structural interaction | ||||
• | Global force-level matching | ||||
• | Compartment strength and stability |
The 2 most challenging compatibility issues were structural interaction and global force matching. Structural interaction describes how the contact forces are distributed across collision partners and the stability of the deforming structures. Good structural interaction is not commonly found in modern vehicles due the differences in vehicle sizes and crashworthiness designs. Poor structural interaction leads to phenomena such as over/underride or fork effects, which in turn lead to undesirable deformation and intrusion of the occupant
compartment. This can also lead to a back-loaded crash pulse and an imperfect restraint triggering. Frontal force-level matching is desirable to ensure that crash energy is appropriately shared between collision partners. Current international consumer and regulation test methods cause frontal crush forces to be mass dependent and require heavier vehicles to be stiffer than lighter vehicles. Earlier studies found that this disparity in vehicle force levels caused heavier vehicles to overcrush lighter vehicles and produce undesired occupant compartment deformations. The 2 compatibility characteristics described above require a strong and stable occupant compartment to support energy absorption in frontal structures.
FIMCAR Approach
One explanation for the lack of progress in compatibility can be the terminology and individual definitions used when discussing compatibility. An improved and more detailed description of compatibility characteristics is a key point on which to base any research project that addresses compatibility. For example, structural interaction can likely be divided into different subareas dealing with geometric placements of structures or the way structures internally distribute loads in the car. Until a terminology is commonly agreed upon, it will be difficul to design and evaluate a test approach with a general description like structural interaction.
The FIMCAR project worked with 2 main research activities. One was to develop an evaluation strategy for selecting some combination of suitable test configurations and the second was the technical development activities of specific test candidates. The first activity required terminology, priorities, and selection criteria. The second involved crash testing, computer simulation, and data processing to develop test procedures as well as assessment criteria and performance limits.
The FIMCAR project was designed to investigate the possibility of combining different configurations to assess compatibility. These tests are the full-width rigid barrier (FWRB), full-width deformable barrier (FWDB), offset deformable barrier (ODB), progressive deformable barrier (PDB), and mobile deformable barrier (MDB). A general description of the available test procedures is provided in the following subsections. The reader is referred to Adolph, Schwedhelm, et al. (2012), Adolph, Edwards, et al. (Citation2013), Lazaro et al. (Citation2013), Seyer et al. (Citation2003), Summers et al. (Citation2002), Edwards, Goodacre, et al. (2008), and Versmissen et al. (2006, 2013) for detailed descriptions of each of the candidate test procedures.
Full-Width Load Cell Barrier Tests
This test is effectively a modification of the U.S. FMVSS-208 full-width test used for the assessment of self-protection. The test is modified by the addition of a high-resolution load cell wall (LCW). The test should control both partner and self-protection. For partner protection, the car's structural interaction potential will be assessed using the measurements from the LCW. Configurations of the test, with and without a deformable honeycomb element, were examined by different research communities. The test assesses structural interaction as well as introduces a high deceleration pulse to assess occupant restraint systems.
As a general justification for the introduction of a full-width test in Europe, it should be stated that the introduction of UNECE Regulation 94 has eliminated the legislated requirement for a full-width test. Originally, UNECE Regulation 12 specified steering wheel intrusion requirements for European vehicles in a FWRB test configuration. However, vehicles complying with UNECE R94 will comply with UNECE R12, precluding the need for full-width testing of vehicles in Europe.
Offset Barrier Tests
The current offset test approaches, most common in vehicle testing, are used in the European frontal directive (96/79/EC) and in consumer tests like Euro NCAP. These consist of an impact into a honeycomb barrier (EEVC barrier) with a 40 percent overlap. There are no current activities investigating the use of this test configuration for measuring structural interaction, but frontal force levels have been measured using an LCW mounted behind the deformable element and was investigated previously (Edwards et al. Citation2007). Another offset test procedure—the PDB—has been investigated for structural interaction and frontal force-level assessment. This 50 percent offset test condition measures the deformation of the honeycomb barrier after the test. The PDB honeycomb is stiffer than the EEVC barrier and becomes progressively stiffer with increased deformation. The barrier deformation is used to analyze the structural interaction and force levels of the tested vehicle.
Moving Deformable Barrier Tests
A frontal impact test using a deformable barrier element mounted on a moving trolley has been investigated as a method to assess and control frontal force levels. In fixed barrier tests like the full-width and offset tests, the initial kinetic energy of the test vehicle must be absorbed in the deformation of the vehicle and the barrier. In a moving barrier test, the kinetic energy and momentum are distributed between the vehicles depending on the vehicle mass. This allows the test to evaluate vehicles for different conditions depending on their mass.
Terminology
From a review of previous research, such as EEVC WG15 (EEVC 2007), the VC-COMPAT project (Edwards et al. Citation2007), IHRA (Seyer et al. Citation2003), and additional accident analysis (Thompson et al. Citation2013), FIMCAR members defined a list of issues that describe the challenges in vehicle crashworthiness. The consortium agreed that
• | Compatibility consists of self- and partner protection. | ||||
• | Improved compatibility will decrease the injury risks for occupants in single- and multiple-vehicle accidents. | ||||
• | Compatible vehicles will deform in a stable manner, allowing the deformation zones to be exploited even when different vehicle sizes and masses are involved. |
Accident Analysis
Since the introduction of the European frontal impact legislation and consumer information activities by Euro NCAP, the frontal impact crashworthiness of cars has improved significantly. To identify the critical issues that need to be addressed, an accident analysis was performed with the following objectives:
• | To determine whether compatibility issues identified in previous studies (Edwards et al. Citation2007; Faerber 2007) and mentioned above are still relevant in the current vehicle fleet, including:
| ||||||||||||||||||||||
• | To determine the current nature of occupant injuries and injury mechanisms. | ||||||||||||||||||||||
• | To ensure that the results of the work were relevant to the current fleet, only cars that were compliant with UNECE R94 or had an equivalent safety level (i.e., new cars) were selected for the analysis. |
Data Sample
The accident data bases used for this study were as follows:
• | UK Cooperative Crash Injury Study (CCIS) | ||||
• | German In-Depth Accident Survey (GIDAS) |
The following criteria were used to select the data samples used in this study:
• | Cars involved in significant frontal impacts | ||||
• | Cars compliant with UNECE R94 or equivalent safety level | ||||
• | Front seat adult occupants (i.e., over 12 years old). | ||||
• | Occupant belted | ||||
• | Occupant sustained a Maximum Abbreviated Injury Scale (MAIS) 2+ injury |
Conclusions from Accident Analysis
Poor structural interaction between vehicles, in particular low overlap and over/underriding of car fronts, was identified as an issue in the current vehicle fleet. This was identified in 40 percent of fatal and 36 percent of MAIS 2+ injured cases. However, in only 25 percent of fatal and 12 percent of MAIS 2+ cases was intrusion present, and it is only in these cases that it can be said definitely that improved structural interaction would have improved the safety performance of the car.
Frontal force/compartment strength mismatch between cars in the current fleet appears to be less of an issue than poor structural interaction. It is important to note that structural interaction problems could be masking frontal force/compartment mismatch problems. Compartment strength of vehicles is still an issue in the current vehicle fleet, especially in accidents with heavy goods vehicles and objects. In particular,
• | the proportion of belted MAIS 2+ injured occupants with AIS 2+ injuries caused by contact with intrusion: CCIS 25 percent, GIDAS 12 percent. | ||||
• | when an occupant sustains an injury caused by contact with intrusion, in the majority of cases it is the most severe injury, often a leg or thorax injury but sometimes a head or arm injury. |
In the current fleet, a high proportion of MAIS 2+ injuries were related to the restraint system (i.e., those caused by loading of the occupant by the seat belt or air bag to decelerate the occupant and prevent greater injury by contact with other car interior structures). Over 40 percent of MAIS 2+ injuries were attributed to restraint loading in both CCIS and GIDAS data sets.
There was a high proportion of fatal and MAIS2+ injuries in accidents with high overlap (>75%) and the proportion of injuries related to the restraint increased with overlap, whereas the proportion of injuries caused by contact with intrusion decreased. It was also noted that there was a high proportion of fatal and MAIS 2+ survived injuries for low overlap impacts. Details of the FIMCAR accident analysis are available in (Johannsen et al. 2011; Thompson et al. Citation2013).
FIMCAR Priorities and Strategies
The main source for establishing the priorities was the accident analysis. Using this information and the results of previous studies, the experts present at FIMCAR meetings determined the FIMCAR strategies. Some of the relevant observations were as follows:
• | Poor structural interaction was observed to be a problem in the current vehicle fleet. The dominant structural interaction problems in car-to-car impacts are over/underriding of car fronts and low overlap. However, a fork effect is seen more often in car-to-object impacts because of impacts with narrow objects. | ||||
• | In a matched pair analysis of car-to-car impacts, a relationship was found between mass ratio and driver injury severity, namely, the higher the mass ratio, the higher the driver injury severity (a mass ratio above 1 means that the partner vehicle is heavier). However, no such relationship was found between mass ratio and compartment strength issues in the limited data available. | ||||
• | Compartment strength is a particular problem in collisions with HGVs and objects, with these collisions having a high proportion of fatal and MAIS 2+ injuries. | ||||
• | AIS 2+ injuries resulting from deceleration loading of the occupant by the restraint system were present in a significant proportion of frontal crashes, regardless of whether intrusion was present or not. | ||||
• | A high proportion of fatal and MAIS 2+ injuries occurred in cases with high overlap (>75%). |
Table 1 Main compatibility topics and associated priorities (color available online)
Table 2 List of compatibility characteristics
The last point reinforced the need for a test condition that requires a vehicle safety system (comprising the frontal structural and occupant restraint system) that is able to withstand a high deceleration, large overlap condition that is not addressed by the current UNECE R94 requirements.
Based on the information in previous work and the FIMCAR accident analysis, the consortium established a list of critical compatibility requirements. The first step was to prioritize these requirements; see .
Priority 1 items were those that the consortium identified as important for FIMCAR to resolve within the project, and priority 2 items were important but deemed not critical to resolve during the project duration. The most interesting points to note were that the deformation forces of frontal structures and enhanced compartment strength for light vehicles in vehicle-to-vehicle accident issues were not a high priority for FIMCAR. This was because the matched pair analysis showed that smaller cars were not found to have a higher risk of intrusion than heavier vehicles. Although this requirement was a conclusion and a priority in earlier studies (Faerber 2007), evolution of vehicle safety is resulting in stronger vehicle compartments. Because lighter vehicles were not found to have a higher risk of compartment intrusions, even for heavier crash partners, frontal force differences between vehicles is not as critical today as perceived earlier. This was a conclusion from a limited data set and it should be noted that there is still a higher injury risk for small vehicle occupants in car-to-car crashes. Further work is needed to make definitive conclusions, but the injury risk for small vehicles seems to be related to the higher delta V experienced by a small car rather than its structural capacity.
After the top-level priorities were identified, a more detailed breakdown of requirements was used to create more specific targets for the technical development and evaluation activities in the project. Again, accident analysis and compatibility requirements and priorities led to the development and ranking of priority 1 and priority 2 issues that are presented in . These were used as the foundation for evaluating the different full-width and offset test procedures and to determine which combinations of test and assessment procedures could provide a complete assessment approach for frontal impact and compatibility.
The different load cases created in the full-width and offset test configurations helps to facilitate the evaluation of different compatibility characteristics. The potential for each test method is illustrated in , where the color coding (green = likely, yellow = maybe, red = not likely) indicates the potential of the test to assess a specific characteristic. The benefits and limitations of the different test procedures are apparent and, more important, the inability of a single test procedure to fulfill all 15 of the priority 1 requirements. The main weakness of the offset tests is the inability to assess structural alignment in the beginning of a crash (item 2), and the full-width tests do not suitably assess compartment strength (item 8).
Evaluation Process
The list of criteria and their prioritization provided a basis for an objective comparison of the test procedures. The technical development of each test and assessment procedure was documented and its capability to assess each of the requirements was reported. The methods for assessing each requirement varied and were essentially confirmation (yes/no), engineering documentation (data presentation), or assessment with reference vehicles with known properties. The latter case was critical because no single vehicle could be identified as fulfilling all compatibility requirements, but vehicles could be identified that fulfilled one or more compatibility requirement. The test procedures were among others validated against the performance of specific vehicles (actual vehicles and FIMCAR car models) with known compatibility performance. Experience in the VC-COMPAT project (Edwards et al. Citation2007) suggested that vehicles exhibit a combination of different compatibility requirements, but specific issues could be identified in car-to-car tests and simulations.
Data from each of the test development work packages in FIMCAR were summarized in a table format based on the items, but only the priority 1 issues were addressed in the evaluation. As expected, there was no single test method that could satisfy all of the issues and a combination of test procedures was necessary. As a result, the selection of an assessment approach could be separated into 2 independent evaluations—one for the full-width and one for the offset test configurations.
A key point to note in the following presentation of results is that the initial prioritization activities and evaluation activities occurred in the first 2 years of the project, before the full assessment metrics for any test procedure were finalized. The goal was to focus the final validation and documentation activities on the most viable test and assessment procedures.
Results
Full-Width Test Procedure
The selection of a full-width test procedure was difficult because the 2 candidates were similar but had unique advantages and disadvantages. The results of the initial evaluation of the full-width procedures are shown in Table A1, where an overview of the expected performance of the tests is compared for each test procedure using color coding. Although quite similar, the FWDB had the potential to address more compatibility requirements.
The FWRB and FWDB both provide a high deceleration pulse for the occupant and use similar test instrumentation. The main difference is the time window available for assessing vehicle structures. A rigid barrier may only allow a short assessment duration before the engine contacts the LCW and begins to mask the structural forces with high contact loads (Yonezawa et al. Citation2009). The deformable barrier face attenuates the engine contact and allows for a longer evaluation period before the engine contact. For both rigid and deformable barrier full width tests, Load Cell Wall (LCW) data was investigated as the method to assess the structural interaction characteristics of a vehicle by measuring the LCW force distribution. The current de facto standard for an LCW is one that consists of 125 mm square elements with the bottom row mounted with an 80 mm ground clearance (Figure 4). The load cell wall rows are counted from bottom to top.
Table 3 Advantages of different full-width tests
The influence of the barrier face on the measurement capabilities of the LCW was important in the decision to choose an FWRB or an FWDB. The FWRB is able to directly measure the structural loads from the vehicle because there is no honeycomb filtering the forces. However, the FWRB could not assess loads in rows 1 and 2 that come after the analysis window for structural alignment, sometimes as short as 6 ms (Adolph, Edwards, et al. 2013). There have been suggestions to supplement the FWRB with an ORB when assessing higher vehicle structures such as sport utility vehicles (SUVs; Patel et al. Citation2009), but FIMCAR data suggest that it may be possible to assess the secondary energy absorbing structures (SEAS) that are beneficial for car-to-car collisions by the FWDB, whereas the ORB at present does not seem to be able to distinguish sufficiently between beneficial and poor SEAS (Adolph, Edwards, et al. 2013; Stein et al. Citation2013).
Fig. 5 Comparison of front structure deformation pattern in different frontal impact tests: (a) FWDB test, (b) FWRB test, and (c) car-to-car test (color figure available online).
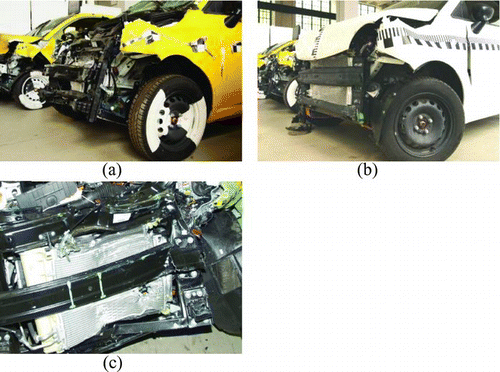
Fig. 6 (a) Late air bag deployment in 40 km/h FWDB test and its consequences for head acceleration. (b) At approximately 30 ms the air bag starts to deploy and (c) at approximately 50 ms the head contacts the deploying air bag (color figure available online).
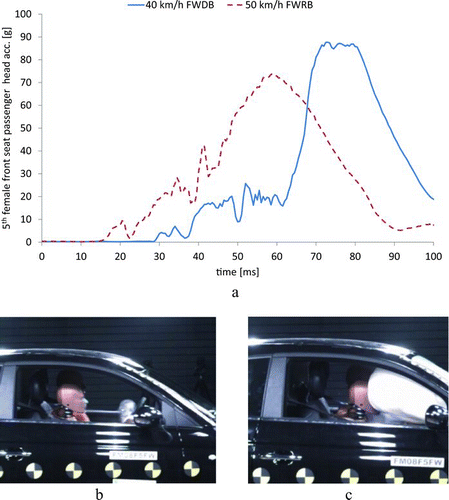
It is expected that the FWDB test results are more representative of real-world accident performance with respect to restraint system triggering and stability of energy-absorbing structures. shows the deformation pattern of the same car in different test configurations. There were similarities in the deformations in the car-to-car and FWDB test where the crash box was not used due bending of the main structures. The deformation pattern of the FWRB test, however, was evenly distributed vertically and laterally and the energy absorption structures like the crash box were well exploited. This shows that cars with good deformation behavior in FWRB test do not necessarily deform in a stable manner in car-to-car impacts. It is thus difficult to predict car-to-car crash performance from FWRB test results.
shows the front seat passenger air bag deployment in a 40 km/h FWDB test. The air bag deployment was delayed, resulting in a rather late contact between the passenger and the deploying air bag. This resulted in higher head accelerations in the 40 km/h FWDB test than in a 50 km/h FWRB test.
The FWDB had technical advantages for assessing structural alignment and for testing the cars in a more representative way, whereas the FWRB offers easier global harmonization and potentially less test variability due to a deformable face; see . After the initial evaluation of the test procedures, the consortium selected the FWDB test as the most promising candidate. There were different metrics available that had exhibited promising results. The outstanding issues that needed to be resolved were the selection and validation of the final assessment metric, criteria for occupant injury, and the test speed. Once this was established, integration with the offset test was required.
After selection of the FWDB in the FIMCAR assessment approach, further work was needed to finalize the structural alignment metric, confirm a test speed, report the repeatability and reproducibility results, and identify the occupant injury criteria. Due to the fact that none of the final FIMCAR test procedures were capable of assessing horizontal load spreading, some further research of the FWDB test was conducted to develop this capability.
Fig. 7 Incidence of injuries in high overlap accidents (overlap > 75%; Adolph, Edwards, et al. 2013).
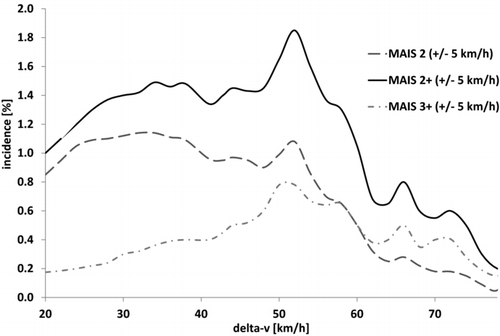
FIMCAR Deliverable 3.2 (Adolph, Edwards, et al. 2013) documents the final verification of the metric for evaluating the structural alignment of vehicles. The main results and recommendations of the FWDB investigations in the later stages were as follows:
• | FWDB test speed of 50 km/h: This meets the desired test severity of a 50 km/h delta V identified from accident analysis and also produces a high crash pulse. The test speed was verified by combining the risk of being involved in an accident within a specific delta V range and the injury risk for that delta V. The result is plotted in and indicates that the test delta V should be between 47 and 57 km/h. Taking into account the rebound velocity and to avoid too aggressive test requirements, the test speed was fixed at 50 km/h. | ||||
• | Structural alignment: The metric to assess structural alignment currently proposes that a vehicle must achieve minimum load requirements in rows 3 and 4 and can use loads in row 2 to help meet this requirement under certain conditions. The minimum load requirement promotes structural alignment and the credit of loads from row 2 encourages vertical load spreading (). The metric can be defined as follows: | ||||
• | Up to time of 40 ms:
where F T40 is the maximum of total LCW force up to time of 40 ms and
| ||||
• | (Note: values to be confirmed taking into account the new test velocity.) | ||||
• | Horizontal load spreading: The FWDB test approach is unable to assess the horizontal load spreading in a repeatable manner because of issues such as bottoming out of the barrier face. |
The FWDB metric was validated using the geometric data for the main structural members and the LCW data. There was a good correlation between the physical structures and the metric; see Table A3. Further validation using car-to-car test results in FIMCAR confirmed the metric suitability. The main car-to-car test approach in FIMCAR was to repeat test configurations with different structural alignments. Only one vehicle, the Super Mini (SM) 1, was tested in corresponding FWDB configurations. The FWDB results are shown in .
Table 4 FWDB results for car-to-car reference vehicles (color available online)
The first result to note is that the vehicles that pass the FWDB metric with both a good distribution between rows 3 and 4 (fulfilling structural alignment) and also qualifying for an LR had good car-to-car test results regardless of test conditions (Sandqvist et al. Citation2013). SM1 exhibited poor compatibility with a total misalignment of 76 mm and SM2 had good compatibility with a higher (100 mm) misalignment. The intrusions in the car tests are shown in for SM1 (test series 1a) and SM2 (test series 1b).
The SUV car-to-car tests demonstrated that structural alignment was preferable over the case when primary energy absorbing structures (PEAS) were misaligned but SEAS were able to provide vertical load spreading. The FWDB was able to detect the vertical load spreading of SUV 2 even with SEAS that positioned approximately 200 mm behind the bumper crossbeam (Sandqvist et al. Citation2013; Stein et al. Citation2013).
Reproducibility and Repeatability
The FWDB was investigated through a combination of component and full-scale tests. Component tests were conducted at TRL Limited, Bundesanstalt für Straßenwesen (BASt), and UTAC and reported in FIMCAR Deliverable 3.2 (Adolph, Edwards, et al. 2013). The component tests showed that the variation in load cell readings was consistent between the tests and below 10 percent. The component tests also showed no crosstalk or load spreading issues that were critical for the metric.
Full-scale tests with an FWDB were reviewed from previous European research projects such as VC-COMPAT and APROSYS (Advanced Protective Systems). From these projects, 7 test sets based on 3 car models tested in different crash labs were available. However, FIMCAR required an additional 3 tests at 2 crash labs with the same vehicle. The results from the earlier projects showed good repeatability and reproducibility, although some were only for 2 vehicles. The FIMCAR test results did not consistently show good repeatability and reproducibility. The total loads measured in the 3 tests were within expected test variation, but the 2 tests at the same research institute had slightly different results, which resulted in different evaluation outcomes, though one of the 2 tests demonstrated reproducibility with respect to the third test. The chosen test vehicle had demonstrated instability in car-to-car impacts (Sandqvist et al. Citation2013). The LCW where the tests were repeated did not meet the instrumentation requirements identified by FIMCAR (Adolph, Edwards, et al. 2013). Because of these issues, further validation is required to confirm whether or not the LCW with a deformable barrier has sufficient repeatability and reproducibility for the regulatory application. However, FIMCAR has concluded that the FWDB repeatability and reproducibility is acceptable—that is, in line with other crash tests—for cars with a stable front structure in this test mode. For further analysis of repeatability and reproducibility, the use of a car with a stable front structure and total forces above 500 kN is recommended. Furthermore, the LCW requirements as developed by FIMCAR should be met for the LCWs used.
Offset Test Procedure
Initial discussions in the FIMCAR project suggested that the existing ODB in UNECE R94 was not capable of evaluating the compatibility of a vehicle. The PDB and MPDB became the preferred offset test procedures for further development because it was anticipated that a metric for assessing the load spreading capabilities of a vehicle could be developed during the project. There have also been significant discussions on the ability of the PDB to provide a sufficiently severe test condition for all vehicle masses (GRSP Citation2010).
At the time of the evaluation of the different test candidates, details of the PDB and MPDB testing and simulation activities to assess compatibility characteristics were presented in FIMCAR Deliverables D2.1 (Lazaro et al. Citation2013) and D4.2 (Versmissen et al. Citation2013). The results of the offset test candidates are shown in Table A2. There were clear issues with the metrics being developed for the PDB and, at the time of evaluation, no robust metrics were available for the group. The test criteria proposed for assessing load spreading were based on complicated mathematical concepts and involved quantifying iso-curves for barrier deformations. There were discontinuities when the iso-curves crossed the assessment boundaries, and this introduced step effects that were not consistent when applied to different vehicles. An additional issue regarding the test severity for heavier vehicles arose for the PDB and, at the time of evaluation, the comparison of test severity for identical vehicles for PDB and ODB tests could not be presented.
Even though the ODB provides no potential for partner protection or load spreading compatibility issues, it was able to maintain the current level of self-protection for vehicles. The ODB complemented a full-width test in terms of fulfilling the compatibility characteristics that were identified in the project. Unfortunately, the lack of a horizontal load spreading criteria in the ODB and full-width test resulted in one priority 1 issue not being fulfilled. Given the time available and the uncertainty in producing a PDB metric, the ODB barrier was chosen as the test method to evaluate self-protection and maintain compartment strength in single-vehicle collisions. There was no perceived benefit in introducing a new offset test procedure without the guarantee of additionally developing an assessment criterion for compatibility within the FIMCAR project. The PDB and MPDB were thus not proposed as the offset test configuration. It should be noted that at the end of the FIMCAR project a draft (M)PDB ([Mobile] Progressive Deformable Barrier) metric was presented that analyzes slopes and hills of the barrier deformation (del Pozo et al. 2013).
The ODB test is proposed as is currently specified in UNECE R94. The current test speed is 56 km/h and no load cell or barrier assessments are required. Currently, an additional requirement on vehicle intrusions is proposed to ensure that all vehicles have a stable occupant compartment. A maximum deformation of 50 mm to the A-pillar is the recommended threshold for this requirement. This requirement will likely not change many of the cars produced for the European market today because Euro NCAP requirements are much more demanding. However, the FIMCAR consortium was reluctant to rely on Euro NCAP assessment for future car safety and proposed the additional requirement to ensure that cars that may not be designed to give good scores in Euro NCAP and may not be tested by Euro NCAP meet a minimum compartment strength requirement.
Conditions for Compliance
Two tests for frontal impact requirements were proposed by FIMCAR and each test configuration must be totally fulfilled, independent of the results of the separate tests.
Benefit Analysis
A cost–benefit analysis was performed to estimate the effect of the following potential changes to the frontal impact regulation:
• | Option 1: No change and allow current measures to propagate throughout the vehicle fleet. | ||||
• | Option 2: Add a full-width test to the current ODB test. | ||||
• | Option 3: Add a full-width test and replace the current ODB test with a PDB test. |
For the benefit analysis it was assumed that the introduction of a full-width test with appropriate compatibility and dummy metrics has the potential to address the frontal impact issues under/override related to structural alignment and restraint-related acceleration-type injuries. Limited potential of the full-width test was expected for addressing fork effect issues. It was also assumed that the replacement of the ODB by the PDB/MPDB test procedure with an appropriate homogeneity metric had the potential to address the frontal impact issues under/override related to vertical load spreading, fork effect, and low overlap as well as frontal force matching/
compartment strength.
The benefits of 3 potential changes to the frontal impact regulation were calculated for Great Britain and Germany and scaled to give an indicative estimate for Europe.
• | For option 1, no change, a small benefit of about 2.0 percent or less of all car occupant killed and seriously injured (KSI) casualties was estimated. | ||||||||||||||||
• | For option 2, add full-width test, a benefit of 5–12 percent of all car occupant KSI casualties was estimated. It was shown that this benefit consisted of
| ||||||||||||||||
• | For, option 3, add full-width test and replace ODB test with PDB test, 9–14 percent of all car occupant KSI casualties. | ||||||||||||||||
• | Benefit percentages for options 2 and 3 do not include the benefit of option 1, no change. |
Breakeven costs for options 2 and 3 were calculated. A comparison of these costs with costs estimated by previous projects indicated that the monetary value of the benefits of implementing option 2 should be greater than the costs to modify the cars for restraint system changes. However, further work is needed to determine precisely what changes would be needed to deliver the injury reduction assumed for the benefit analysis and precisely what test configuration (in particular dummies) and performance limits would be needed to enforce these changes.
When considering the results of the benefit analysis, the following points should be noted:
• | The benefit was calculated assuming the implementation of complete assessment procedures. However, appropriate dummy assessment values and dummy selection have not been addressed by FIMCAR and appropriate PDB/MPDB metrics are not yet established. | ||||
• | Possible further potential benefits from the definition of a common interaction zone related to truck underrun protection and roadside guard rails were not considered in the study. |
Further information on the benefit analysis is available in Edwards, Cuerden, et al. (2012) and FIMCAR Deliverable D1.2 (Edwards, Wisch, et al. 2013).
Summary
Within the European-funded FIMCAR project, different frontal impact test procedures were analyzed regarding their potential for future frontal impact legislation. The research activities focused on car-to-car frontal impact accidents based on accident investigations involving newer cars.
The FIMCAR consortium has identified a suite of tests and assessment procedures that can evaluate a vehicle's frontal impact protection capability, namely, the offset test with the ODB barrier at 56 km/h and a full-width test with a deformable barrier and a load cell wall (FWDB) at 50 km/h. A metric based on the LCW readings was developed to establish a common interaction zone.
These recommendations are submitted to rule-making officials in UNECE committees for final evaluation and potential adoption. The current test procedures in the FIMCAR project will potentially introduce new requirements for European vehicles.
Technical advantages for the FWDB (more representative of real-world accident situations and more reliable structure assessment) have been identified and are documented in FIMCAR Deliverable D3.2 (Adolph, Edwards, et al. 2013).
Nevertheless, the FWDB is not a globally harmonized test procedure. A great deal of attention is being turned to the detection of lower load paths and SEAS as defined in the U.S. voluntary commitment (Barbat Citation2005; Patel 2009). Initial evaluations within FIMCAR using simulation, car-to-car testing, and barrier tests indicate that the FWDB may be able to detect the structures relevant for structural alignment and structure interaction without relying on additional tests like the ORB (Stein et al. Citation2013).
The selected offset test procedure, ODB, does not satisfy the load spreading issues identified by the consortium. Subsequent to the initial test candidate selections, work with a horizontal load spreading metric using the FWDB has not succeeded.
Because the ODB barrier requires no significant development work, a modest effort was directed toward the PDB metrics even after it was eliminated from the FIMCAR final assessment approach. The PDB and MPDB tests are currently the only configurations that can potentially assess horizontal load spreading. Candidates for metrics to assess load spreading have been identified, but validation and repeatability issues still must be resolved before the candidates can be forwarded to rule makers. This eliminates them from the final FIMCAR test protocol but not for evaluation after the completion of the project.
Appendices
Download Zip (159.6 KB)Acknowledgments
This article was written on behalf of the members of the FIMCAR project. The FIMCAR project was cofunded by the European Commission under the 7th Framework Programme (Grant Agreement No. 234216). The members of the FIMCAR consortium are Technische Universität Berlin, Bundesanstalt für Straßenwesen, Chalmers tekniska hoegskola AB, Centro Recerche Fiat S.C.p.A., Daimler AG, FIAT Group Automobiles Spa, Humanetics GmbH, IAT Ingenieurgesellschaft für Automobiltechnik mbH, IDIADA Automotive Technology SA, Adam Opel GmbH, Peugeot Citroën Automobiles SA, Renault s.a.s, TNO, TRL Limited, UTAC, Volvo Car Corporation, Volkswagen AG, and TÜV Rheinland TNO Automotive International BV.
This article used accident data from the UK CCIS collected during the period 2000–2009. CCIS was managed by TRL Limited, on behalf of the DfT (Transport Technology and Standards Division), who funded the project along with Autoliv, Ford Motor Company, Nissan Motor Company, and Toyota Motor Europe. Previous sponsors of CCIS have included Daimler Chrysler, LAB, Rover Group Ltd., Visteon, Volvo Car Corporation, Daewoo Motor Company Ltd., and Honda R&D Europe (UK) Ltd. Data were collected by teams from the Birmingham Automotive Safety Centre of the University of Birmingham, the Transport Safety Research Centre at Loughborough University, TRL Limited, and the Vehicle & Operator Services Agency of the DfT.
© Heiko Johannsen, Thorsten Adolph, Mervyn Edwards, Ignacio Lazaro, Ton Versmissen, and Robert Thomson
References
- Adolph , T , Edwards , M , Thomson , R and Vie , N . Updated Full Width Test Protocol Universitätsverlagder TU Berlin, 2013
- Adolph , T , Schwedhelm , H Lazaro , I . Compatibility assessment in FIMCAR for frontal impact test procedures . Paper presented at: ICRASH Conference; July 18–20 , Milan , , Italy
- Barbat , S . Status of enhanced front-to-front vehicle compatibility technical working group research and commitments. Paper Number 05–463 . Paper presented at: 19th International Technical Conference on the Enhanced Safety of Vehicles; June 6–9 , Washington DC
- del Pozo de Dios , E , Lázaro , I , Delannoy , P and Thomson , R . Development of a structural interaction assessment criteria using progressive deformable barrier data . Paper presented at: 23rd International Technical Conference on the Enhanced Safety of Vehicles; May 27–30 , Seoul , Korea
- Edwards , M J , Cuerden , R W , Price , J , Broughton , J , Wisch , M and Adolph , T . Estimation of the benefits for potential options to modify UNECE Regulation No. 94 to improve a car's compatibility . Paper presented at: ESAR Conference , Hannover , , Germany ; September 7–8,
- Edwards , M J , de Coo , P van der Zweep , C . 2007 . Improvement of Vehicle Crash Compatibility through the Development of Crash Test Procedures VC-COMPAT Final Report GRD2/2001/50083. Available at http://vc-compat.rtdproject.net/cgi/getdoc.cgi?id=453; Accessed December 10, 2012
- Edwards , M J , Goodacre , O Versmissen , T . 2008 . Evaluation of Advanced European Full Width (AE-FW) Test APROSYS Deliverable 1.2.2
- Edwards , M J , Wisch , M , Pastor , C , Price , J , Broughton , J and Adolph , T . Report Detailing an Estimation of the Costs and Benefits of Improved Car-to-Car Compatibility on a National and European Scale Universitätsverlagder TU Berlin, 2013
- Enhanced European Vehicle-Safety Committee . 2007 . Car crash compatibility and frontal impact . Available at: http://www.eevc.org. Accessed December 10, 2012
- European Council for Automotive R&D . 2001 . Development of Criteria and Standards for Vehicle Compatibility Project BE4049
- European Union Road Federation . 2010 . European road statistics 2010 . Available at: http://www.irfnet.eu/images/stories/Statistics/2010/ERF_European_Road_Statistics_2010.pdf. Accessed April 4, 2013
- Faerber , E . June 18–21 . “ EEVC approach to develop test procedure(s) for the improvement of crash compatibility between passenger cars ” . Lyon , , France. : Paper presented at 20th International Technical Book on the Enhanced Safety of Vehicles .
- GRSP . Draft minutes of the 7th meeting of the UN-ECE/WP29/GRSP/ informal working group on frontal impact , Available at: http://www.unece.org/fileadmin/DAM/trans/doc/2010/wp29grsp/FI-07–07e.pdf. Accessed April 4, 2013
- Johannsen , H , Adolph , T , Thomson , R , Edwards , M , Lázaro , I and Versmissen , T . June 13–16, 2011 . “ FIMCAR – Frontal impact and compatibility assessment research: strategy and first results for future frontal impact assessment ” . In Paper presented at: 22nd International Technical Book on the Enhanced Safety of Vehicles Washington D.C. , , USA
- Lazaro , I , Vie , N , Thomson , R and Schwedhelm , H . Final Development of Off-Set Test Universitätsverlagder TU Berlin, 2013
- O’Reilly , P . Status report of IHRA Compatibility and Frontal Impact Working Group . Paper presented at: 18th International Technical Conference on the Enhanced Safety of Vehicles; May 19–22 , Nagoya , , Japan
- Patel , S , Mohan , P and Prasad , A . NHTSA's recent test program on vehicle compatibility . Paper presented at: 21st Technical Conference on the Enhanced Safety of Vehicles; June 15–18 , Stuttgart , , Germany
- Sandqvist , P , Thomson , R Kling , A . Report on Car-to-Car Test Results Universitätsverlagder TU Berlin, 2013
- Seyer , K , Newland , C and Terrell , M . Australian research to support the IHRA Vehicle Compatibility Working Group . Paper presented at: 18th International Technical Conference on the Enhanced Safety of Vehicles; May 19–22 , Nagoya , , Japan
- Stein , M , Johannsen , H and Thomson , R . FIMCAR—influence of SEAS on frontal impact compatibility . Paper presented at: 23rd International Technical Conference on the Enhanced Safety of Vehicles; May 27–30 , Seoul , Korea
- Summers , S , Hollowell , W T and Prasad , A . Design considerations for a compatibility test procedure . Paper presented at: Society of Automotive Engineers World Congress; March 4–7 , Detroit , MI
- Thompson , A , Edwards , M , Wisch , M , Adolph , T , Krusper , A and Thomson , R . Report Detailing the Analysis of National Accident Databases Universitätsverlagder TU Berlin, 2013
- Versmissen , T , van der Zweep , C , Mooi , H , McEvoy , S and Bosch-Rekveldt , M . The development of a load sensing trolley for frontal off-set testing . Paper presented at: ICRASH Conference; July 4–7 , Athens , , Greece
- Versmissen , T , Welten , J and Rodarius , C . MPDB Test and Simulation Results Universitätsverlagder TU Berlin, 2013
- Yonezawa , H , Mizuno , K Hirasawa , T . Summary of activities of the Compatibility Working Group in Japan . Paper presented at: 21st Technical Conference on the Enhanced Safety of Vehicles; June 15–18 , Stuttgart , , Germany