ABSTRACT
Objective: Laboratory studies have demonstrated that impact protectors (IP) used in motorcycle clothing can reduce fracture severities. While crash studies have reported IP are associated with reduced likelihood of soft tissue injury, there is little evidence of their effectiveness in reducing fracture likelihood. This discrepancy might be related to IP quality. There are mandatory requirements for IP supplied with protective clothing in Europe, but not elsewhere. This study examines the energy attenuation performance of IP used by Australian riders.
Methods: IP were harvested from clothing worn by crashed riders admitted to hospital. The IP were examined and energy attenuation properties were determined using EN 1621-1 test procedures. Impact injury was identified from medical records and defined as fractures, dislocations, and avulsions that occurred following impact to the rider's shoulders, elbows, hips, and/or knees. Fisher's exact test was used to examine the relationship between meeting the EN 1621-1 energy attenuation requirements and impact injury. The association between the average and maximum transmitted force, and impact injury was examined using generalized estimating equations. Motorcycle riders were recruited as part of an in-depth crash study through three hospitals in New South Wales, Australia, between 2012 and 2014. Riders were interviewed, and engineers conducted site, vehicle, and clothing inspections. Clothing was collected, or identical garments were purchased.
Results: Clothing was inspected for 62 riders. Of these, 19 wore clothing incorporating 76 IP. Twenty-six of these were impacted in the crash event. Almost all impacted IP (96%) were CE marked, and most (83%) met Level 1 energy attenuation requirements of EN 1621-1 when tested. Of the 26 impacted IP, four were associated with impact injuries, including midshaft and distal clavicle fractures and a scapula and olecranon fracture. No associations between meeting EN 1621-1 requirements and impact injury were found (p = 0.5). There was no association between average force transmitted and impact injury (95% CI: 0.91–1.24); however, as maximum force transmitted increased, the odds of impact injury increased (95% CI: 1.01–1.2). These results indicate a high probability of impact injury at 50 kN, the limit of maximum transmitted force specified in EN 1621-1.
Conclusion: The allowable transmitted force of EN 1621-1 may be too high to effectively reduce the probability of impact injury. This is not surprising, given human tolerance levels that are reported in literature. Reducing the force limit below the reported fracture tolerance limits might be difficult with current technology. However, there is scope to reduce the EN 1621-1 maximum limit of 50 kN transmitted force. A reduction in the maximum force limit would improve rider protection and appears feasible, as 77% of tested IP recorded a maximum force <35 kN. This level of transmitted force is estimated to be associated with <20% probability of impact injury. While the performance of IP available to Australian riders is not regulated, most IP was CE marked. The results indicate a significant association between maximum transmitted force, tested according to EN 1621-1 procedures, and impact injury. Further investigation of the EN 1621-1 requirements may be warranted. This work will interest those targeting protective equipment for motorcyclists as a mechanism for reducing injury to these vulnerable road users.
Introduction
Motorcycle use in Australia continues to increase. Since 2010, motorcycle registrations within Australia have risen by more than 22%, with the largest increase occurring in New South Wales. Similarly, there has been an increase in police reported serious injuries (Centre for Road Safety [CRS] Citation2015).
Fractures of the extremities are one of the most common injuries sustained by crashed motorcycle riders, only preceded by soft tissue injuries such as skin abrasions, contusions and lacerations (Association of European Motorcycle Manufacturers Citation2004; Baldock et al. Citation2011; Cawich Citation2010; de Rome et al. Citation2011). Impact protectors (IP) reduce overall injury risk in motorcycle crashes, but there is little evidence that the IP commonly used in protective equipment for motorcyclists are effective in reducing the risk of fractures in the real world. In a previous Australian study, de Rome et al. (Citation2011) reported riders wearing IP had a significant reduction in the risk of soft tissue injury but the benefit of impact protection could not be detected specifically in relation to fractures. This is despite the fact that, dependent on the construction of IP, it has been demonstrated that IP are able to attenuate sufficient energy to reduce fracture severities in the laboratory (Nygren Citation1987; Otte Citation2002).
One reason for the apparent lack of effect in preventing fractures in real-world studies may be variations in the quality of impact protectors worn by riders. In laboratory studies, Otte et al (Citation2002) found differences in effect depending on the material components of the IP. For example, a foam layer with a hard shell had no benefit to load reductions, while a high-thickness foam impact protector reduced loads and the subsequent likelihood of fractures (Otte et al. Citation2002).
In Australia, as in a number of other countries, there is no mandatory performance standard for protective clothing for motorcyclists and/or impact protectors used in protective clothing, and therefore no regulation of the quality of impact protectors provided in motorcycle clothing. In Europe, all motorcycle gear that claims any protective benefit to a rider must carry CE certification. Impact protectors that are CE approved must provide minimum impact performance requirements, including minimum energy attenuation performance (National Standards Authority of Ireland [NSAI] 1998). Some European clothing approved to this standard, and some impact protectors approved to the standard, are available on the Australian market. However, there are also impact protectors and clothing on the Australian market that do not carry CE approval.
To date there has been no study that has examined injury outcomes among motorcyclists who wear protective clothing and/or impact protectors that meet CE requirements compared to those that do not. It is possible that good-quality impact protectors that meet the energy attenuation requirements of the European Standard might provide better protection against fractures.
The aim of this study was to examine the performance of impact protectors in the real world, in terms of the energy attenuation requirements of the European Standard.
Methods
Study design
Cases were a convenience sample of motorcyclists who had been seriously injured in a crash on New South Wales roads. To achieve the aims of this work, IP worn by riders involved in crashes were examined. The injury outcome of riders who used impact protection in a convenience sample of real-world crashes was then compared to the impact protector's performance in laboratory tests.
Setting and participants
Ninety motorcycle riders were recruited as part of an in-depth crash study (Brown et al. Citation2015). Recruitment occurred through three major trauma hospitals throughout the time period August 2012 to June 2014. Two were urban hospitals and one was a regional hospital. Cases were collected prospectively following e-mail notification from the trauma departments and on-site recruitment by research nurses. The motorcycle riders included in the study were aged 16 years or older and were admitted to these hospitals following involvement in a motorcycle crash (MBC). Additional inclusion criteria included a crash location within a 4-hour drive from Sydney, New South Wales, and at least one injury able to be coded to the Abbreviated Injury Severity (AIS) score and therefore a minimum Injury Severity Score (ISS) of 1 (Association for the Advancement of Automotive Medicine [AAAM] Citation2008).
All riders consenting to be part of the study underwent a detailed structured interview conducted by research nurses. Research engineers conducted site and vehicle inspections. Details of IP worn were collected during interview and clothing was inspected by research engineers. Where possible, clothing worn by riders was collected. Otherwise, if sufficient detail was obtained about the motorcycle specific clothing through inspection, identical garments were purchased. IP was then harvested from the clothing and photographed using a predefined standard series of photos and underwent detailed inspection, also using a predefined protocol (Supplementary ; see online supplement). Medical records were reviewed by research nurses and details of all injuries were collected, and coded using AIS (AAAM Citation2008).
Variables
For this analysis, rider details such as gender, age, height, and weight were extracted from rider interviews. An estimation of vehicle traveling speed prior to impact (impact speed) was made from information supplied in the rider interviews and confirmed from review of crash circumstances by the investigation team. To examine the reliability of these estimations, the actual impact speeds for a subset of cases (n = 6), were estimated using computer simulations of the two-dimensional trajectories of the vehicles involved. These simulations were obtained with a custom-built tool developed in the Matlab Simulink environment. This model was developed for the purpose of estimating changes in impact speed due to differences in rider reactions and is described in detail elsewhere (see Savino et al. Citation2014). In this case we have used this model to examine the reliability of speed estimates. Trajectories were modeled as straight and curved segments with transients at constant yaw acceleration in between. Rider and drivers' actions were modeled as constant-speed segments and/or constant-acceleration segments, with transients at constant acceleration rate in between. Swerve maneuvers were modeled as transients from straight segments to curved segments with high curvature. Accelerations were limited in accordance with road friction coefficients estimated from the crash reports. Fall events were modeled as points in which the trajectory became straight. The tool synchronized the trajectories in time and space at collision and allowed collision points to be spatially shifted. This tool was used to check consistency of all the information available in the crash report. Speed estimations from these two sources were compared by calculating the two-way, mixed intraclass correlation coefficient.
Impact injuries were defined as fractures, dislocations, and avulsions that occurred to regions of the rider's body potentially protected by IP (i.e., the rider's shoulders, elbows, hips, and/or knees). Impact injuries deemed likely to have resulted from a single impact to one of these regions were identified from medical records and the severity of these injuries was assigned using AIS. The presence of an impact to a region of the body potentially protected by IP (i.e., the shoulders, elbows, hips, and/or knees) was identified from clothing damage and/or presence of impact injury in these regions. Data from each case were presented to an expert multidisciplinary panel, where case details and analyses such as the identification of impact injuries, impact regions, and injury mechanisms were agreed to by panel members.
Other data collected from the inspection of IP included details of the IP construction, IP material hardness, whether the IP was marked as CE approved, how the position of IP was maintained within the clothing, and whether the IP met the minimum area requirements of EN 1621-1. Details of the clothing material, including material thickness, that surrounded the IP was also collected.
The harvested IP were categorized as CE certified or not based on CE marking, whether or not the IP contained a hard outer shell, IP construction (single-layer foam/plastic, multilayer foam/plastic, or foam only), and whether the IP met the minimum area requirements as defined by the European Standard (type A/B IP) (NSAI Citation1998; NSAI Citation2012).
Laboratory testing
The hardness and energy attenuation properties of each of the harvested IP were measured by laboratory testing. If the IP had been damaged during impact, the undamaged impact protector from the other shoulder, knee, and so on was used in the testing. Hardness was assessed using a Shore A durometer to determine the IP's resistance to indentation. For the measurement, the IP was placed on a hard, flat surface.
Energy attenuation characteristics were determined by measuring force transmitted through the impact protector using a wire-guided drop test rig in accordance with the energy attenuation requirements of EN 1621-1. A 5-kg impactor was dropped from a height of 1 m, impacting each IP in 3 separate locations: once in test area A (outer perimeter of the IP) and twice in test area B (inner central area of the IP) as defined by EN 1621.Testing of these areas ensures the energy attenuation readings are representative of the varying thickness locations across the IP.
Force data was captured using a PCB Piezotronic ICP model 205c quartz force ring and the PCB Piezotronics ICP sensor signal conditioner.
EN1621-1 contains energy attenuation performance requirements based on both the maximum and average transmitted force and specifies two levels of performance (NSAI Citation2012). Level 1 requires an average transmitted force ≤35 kN, and a maximum transmitted force ≤50 kN; Level 2 requires an average transmitted force ≤20 kN, and a maximum transmitted force ≤30 kN.
Analysis
Characteristics of the IP collected were described and relationships between IP construction, IP certification, whether or not IP met the EN 1621 energy requirements, and injury outcome for impacted IP were examined. Fisher's exact test was used to examine the significance of these relationships where possible. Generalized estimating equations (GEE) were used to conduct binary logistic regression analysis while accounting for clustering on the case ID to investigate the association between the IP's (i) hardness, (ii) average transmitted force, and (iii) maximum transmitted force and impact injury in the real world, controlling for potential confounders such as rider age, vehicle impact speed, and rider weight. Odds ratios (OR) and 95% confidence intervals (CI) were calculated. Odds ratios were used to construct probability of injury curves for any IP characteristics demonstrated to be significantly associated with injury outcome using GEE. Finally, the association between IP construction and energy attenuation was examined using one-way analysis of variance (ANOVA). All analyses were conducted using SPSS Statistics (IBM, Armonk, NY).
Results
In total, 90 riders were recruited in this study, and clothing was able to be inspected for 62 of these riders. Of the 62 riders, 19 wore clothing incorporating IP in the shoulder, elbow, knee, or hip regions. From these 19 riders, 76 impact protectors were collected.
The 19 riders wearing clothing incorporating IP were predominantly male (95%), and the age, height, and weight characteristics of this sample are shown in . As also shown in , the average estimated impact speed during the crash event was 53 km/h, with a range from 0 to 100 km/h. A two-way mixed intraclass correlation coefficient for absolute agreement was run to determine the reliability of the speed estimations made by the crash investigation and estimated speed output from computer crash reconstructions for a subset of crashes (n = 6) as described earlier. Due to the very high absolute agreement between the two methods of speed estimation (ICC = 0.993), speed estimations from the crash investigation were used in subsequent analyses.
Table 1. Characteristics of participants.
Across these 19 riders, the 76 impact protectors were worn over the shoulders (n = 36), elbows (n = 36), hips (n = 2), and knees (n = 2). As detailed in , a high proportion of the collected impact protectors were CE marked (97%). Inspection of the IP within their associated clothing found IP were most commonly restricted by means of a mesh pocket (79%), being stitched into the lining of the clothing (10%), by means of a flap closure (8%), or by Velcro (3%). All collected IP met the minimum area requirements of EN 1621-1 (n = 76). Most were constructed from foam only (49%) or a single layer of foam and plastic (43%), with a relatively small number constructed of multilayer foam and plastic (8%). The hardness of the IP ranged from 24 to 99 HA, with a mean of 63.16 (SD 25.34). The mean average force recorded across the IP was 30.7 kN (SD = 9.2 kN) and mean maximum transferred force was 35.8 kN (SD = 14.9 kN). See .
Table 2. Characteristics of the IP samples.
Eighty-three percent of the 76 IPs collected passed the Level 1 () and 1% passed the Level 2 energy attenuation requirements of EN 1621-1. Average transmitted force and maximum transmitted force measured during testing are presented in and , respectively. These figures also graphically represent the average and maximum transmitted force by IP of different construction, as well as the results with respect to the energy attenuation requirements of EN 1621-1.
Figure 2. Maximum transmitted force recorded from the IP collected from the crashed riders. Unfilled shapes denote the presence of an impact injury.
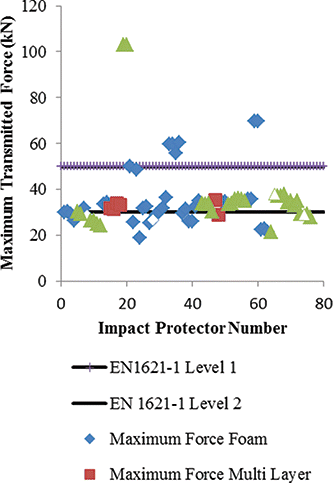
Impact protector characteristics and injury outcome
Twenty-six of the collected IP were identified as being worn in a location that had been impacted during the crash event. Characteristics of this subset of IP are also described in . Four impact injuries occurred among these 26 identified locations (see Appendix A, Table A1). This included midshaft and distal clavicle fractures and a scapula and olecranon fracture. All injuries were AIS2 severity.
Impact injury occurred to the single location covered by non-CE-marked IP (n = 1), and 12% of locations protected by CE-marked IP (3 of 25). As there was only one non-CE-marked IP among the impacted IP, statistical testing was not possible. Based on the energy attenuation testing, 85% (22/26) of the impacted IP passed the Level 1 EN1621-1 requirements. Injury occurred to 25% of the regions protected by IP that did not meet these requirements (1/4) and 14% of those that did meet the requirements (3/22); however, this difference did not reach statistical significance (p = 0.5, Fisher's exact test). Most of these IP contained a hard outer shell (n = 17/26), and injury appeared to be less likely to occur to areas covered by IP with a hard external shell, although this did not reach significance (OR = 0.637, 95% CI: 0.185–2.191). presents injury outcome by IP construction. No injury occurred to locations protected by IP constructed from multilayer foam and plastic, but this precluded statistical testing of any association between this characteristic and injury outcome.
No significant difference was found between the IP's material hardness and the presence of an impact injury (95% CI: 0.896–1.014) when controlling for rider age, weight, and vehicle impact speed via regression modeling. Additionally, there was no association between the average force transferred through an impact protector and the presence of an impact injury (95% CI: 0.91–1.24); however, as maximum force transmitted increased, impact injury was more likely (OR = 1.1, 95% CI: 1.01–1.2). As shown in , the probability of injury was high at the maximum transmitted force allowed by Level 1 EN 1621-1.
Figure 4. Probability of injury by maximum transmitted force. Dashed and solid lines donate Level 1 and Level 2 performance limits as specified in EN1621-1:Citation2012.
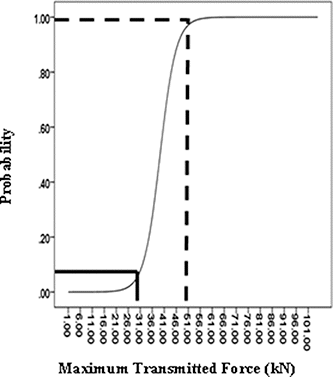
Impact protector construction and energy attenuation
A one-way ANOVA was conducted to compare the effect of IP composition on the average and maximum force transmitted through an IP. The effect of the IP composition on average transferred force was nonsignificant, (F(2, 23) = 0.645, p = 0.534), as was its effect on the maximum transmitted force, (F(2, 23) = 0.324, p = 0.726).
Discussion
While there is no regulatory standard governing the quality of IP provided in motorcycle clothing sold in Australia, almost all (97%) of the IP collected from this sample of Australian riders admitted to hospital after crashing were CE marked. Furthermore, most (83%) when tested met the energy attenuation requirements of the European Standard. Comparing the energy attenuation performance of the IP worn by these riders and the real-world injury outcomes revealed a significant association between maximum force transmitted and the odds of injury. As the maximum force transmitted increased, the odds of injury increased. However, there was no relationship between the ability of the IP to meet the energy attenuation requirements of the standard and the odds of injury.
The work presented here relates energy attenuation as measured using the EN 1621-1 test method to real-world injury outcome. The results cannot be directly related to fracture tolerance levels reported in the literature from different loading conditions, and currently there has been no work linking energy attenuation measured in this way with the types of loading conditions leading to fracture in the real world. However, our findings suggest the energy attenuation requirements of the European Standard may be set too low to effectively reduce the probability of injury. A high percentage of the IP achieved maximum transmitted forces well below the 50-kN limit specified in the Standard for Level 1 IP. For example more than three -uarters (77%) had a maximum transmitted force below 35 kN, and just over one-third (35%) had a maximum transmitted force below 30 kN (Level 2 criteria). According to our analysis of the IP collected from this sample of crashed riders, these levels of transmitted force would be associated with a much lower probability of injury than the current limit of 50 kN. For example, from , a maximum transmitted force level of 35 kN is estimated to be associated with less than a 20% probability of impact injury. Ideally, future work should examine the link between the performance of IP in the EN 1621-1 test method and loading conditions that can be related to human fracture tolerance.
Despite the issues with the current energy attenuation limits possibly being set too high, it is important to note that only four riders, protected by IP, and identified to have sustained an impact to a region protected by IP, actually sustained an impact injury. In laboratory work, Otte et al. (2001) found differences in protective effect associated with the construction of the IP. We saw no injury in riders with IP constructed from multilayered foam and plastic shell, but were unable to statistically confirm this did not occur by chance. We also saw no association between energy attenuation and IP construction. These observations, coupled with the work of Otte et al. (2001), suggest IP construction might be the most important characteristic, or at least as important as energy attenuation, for impact injury protection.
While 97% of the IP examined from this sample of riders were CE marked, only 83% met the energy attenuation requirements of Level 1 EN1621-1. All non-CE-marked IP and 11 CE-marked IP did not pass the Level 1 energy attenuation performance requirements. It is a concern that products that should provide a minimum performance level as required by the EN1621-1 standard are failing this test. The implications of this may be that the lack of regulatory control in Australia allows for noncertified IP to be marked as certified, although this requires further investigation to confirm.
As with any study, there are a number of limitations to keep in mind. While this study delivers results from a convenience sample, the sample closely reflects the age and crash-type distributions of New South Wales crashed motorcyclists. According to Transport for Association for the Advancement of Automotive Medicine, 38% of serious injury crashes involved riders ages 17–29 years (our sample 43%); 39% involved riders ages 30–49 years (our sample 34%); and 23% involved riders ages 50+ years (our sample 21%). Association for the Advancement of Automotive Medicine statistics also indicate that 40% of motorcycle crashes are single-vehicle crashes, and 36% of our sample involved single-vehicle crashes (CRS Citation2013)
As noted earlier, this sample included only a small number of riders with impact injury, and the total number of riders was also small. The results presented here should be confirmed in a larger sample. Due to the small sample, IP from all regions were analyzed together. As the IP used in different regions of the body varies, so too does the mechanism of impact injury across these different regions, and it would be worthwhile examining the performance of specific types of IP (e.g., knee, hip, shoulder, etc.) in isolation. Additionally, the lack of effect in preventing fractures may be attributed to the inability to include all potential mechanisms of impact injury experienced by those with and without IP. This was partially controlled for by limiting the injury outcomes to specifically impact injury; however, unknown and unmeasured variation in impact mechanisms may still be present. There are also potential limitations in the methods used to identify regions of the body that were impacted during the crash and subsequent impact injuries, as well as limitations in estimating impact speed. We estimated impact speed using estimations of the vehicle traveling speed prior to impact. While we examined the reliability of our method of estimating impact speed using a small number of computer reconstructions, we have assumed, but not tested, that this level of reliability applies to all cases included in this analysis. Furthermore, the use of impact speed of the vehicle as an indicator of IP impact severity may not capture true impact severity. Due to the recruitment methods used in the larger in-depth investigation study, this sample of riders is also biased toward the more seriously injured riders involved in motorcycle crashes.
The strength of this study is that it is the first attempt to examine the energy attenuation performance of IP using real-world injury outcomes. While the results demonstrate that increasing maximum force transmitted through IP is associated with increasing odds of impact injury, the results also indicate further investigation of the energy attenuation performance limits included in EN1621-1 may be warranted.
gcpi_a_1311014_sm4061.docx
Download MS Word (26.9 KB)Funding
This work was funded by Australian Research Council grant DP 140102866 and the NSW Centre for Road Safety. Julie Brown is supported by an NHMRC Career Development Fellowship. Lauren Meredith is supported by an Australian Post Graduate Award and the Laurie Cowled Scholarship.
References
- Australian Bureau of Statistics. Motor Vehicle Census, Australia, January 31, 2015. Available at: http://www.abs.gov.au/ausstats/[email protected]/mf/9309.0. Accessed June 27, 2016.
- Association for the Advancement of Automotive Medicine. 2008. Abbreviated Injury Scale 2005: Update 2008. Barrington, IL: Association for the Advancement of Automotive Medicine; 2008.
- Association of European Motorcycle Manufacturers. MAIDS In-Depth Investigation of Accidents Involving Powered Two Wheelers: Final Report 1.2. ACEM; 2004. Available at http://www.maids-study.eu. Accessed June 28, 2016.
- Baldock MRJ, Grigo JAL, Raftery SJ. Protective Clothing and Motorcyclists in South Australia (CASR088). Centre for Automotive Safety Research; 2011.
- Brown J, de Rome L, Baldock MRJ, Albanese B, Meredith L, Fitzharris M. The Austroads In-Depth Case Control Study of Motorcycle Crashes in NSW: Causal Relationship Findings. Australasian Road Safety Conference, Gold Coast, Queensland, 2015.
- Cawich SO, Harding HE, Evans NR, Crandon IW, Mitchell DIG, Martin AC, Rose REC. Strategies for secondary prevention of motorcycle road traffic accident injuries: Time for policy re-evaluation in Jamaica. Internet J Emerg Intens Care Med. 2010;12(1).
- Centre for Road Safety. Road Traffic Crashes in New South Wales: Statistical Statement for the Year Ended 31 December 2013. Author: 2013. Report 93.
- Centre for Road Safety. Injury Trends by Injury Severity, Reporting Years 2005 to 2013. 2015. Available at: http://roadsafety.transport.nsw.gov.au/statistics/interactivecrashstats/nsw.html?tabnsw=6,2015. Accessed November 15, 2016.
- Centre for Road Safety. Monthly Bulletin of Prelimnary Traffic Crash Data May 2016. Author: 2016.
- de Rome L, Ivers R, Fitzharris M, Du W, Haworth N, Heritier S, Richardson D. Motorcycle protective clothing: Protection from injury or just the weather? Accid Anal Prev. 2011;43(6):1893–1900.
- National Center for Statistics and Analysis. Motorcycles: 2013 Data. Traffic Safety Facts. Report No. DOT HS 812 148. Washington, DC: National Highway Traffic Safety Administration; 2015.
- National Standards Authority of Ireland. EN 1621-1:1998 Motorcyclists' Protective Clothing Against Mechanical Impact - Part 1: Requirements and Test Methods for Impact Protectors- Requirements and Test Methods. Author; 1998.
- National Standards Authority of Ireland. EN 1621-1:2012 Motorcyclists' Protective Clothing Against Mechanical Impact—Part 1: Motorcyclists' Limb Joint Impact Protectors—Requirements and Test Methods. Author; 2012.
- Nygren A. Protective Effect of a Specially Designed Suit for Motorcyclists. Passive Safety for Motorcyclists. Bochum, Germany: Institut fur Zweiradsicherheit (IFZ); 1987.
- Otte D Possibilities and limitation for protective measures for injury reduction of vulnerable road users. Int J Crashworthiness. 2002;7(4):441–462.
- Otte D, Schroeder G, Richter M. Possibilities for load reductions using garment leg protectors for motorcyclists—A technical, medical and biomechanical approach. Annu Proc Assoc Adv Automot Med. 2002;46:367–385.
- Savino G, Rizzi M, Brown J, Piantini S, Meredith L, Albanese B, Pierini M, Fitzharris M. Further development of motorcycle autonomous emergency braking (MAEB): What can in-depth studies tell us? A multinational study. Traffic Inj Prev. 2014;15:165–172.