Abstract
Objective
In rear impacts, the seat and seatbelt are intended to provide occupant restraint and maintain the occupant on the seat with favorable kinematics and low biomechanical responses. This study analyzes the lack of restraint provided by lap-shoulder belts in rear impacts with and without pretensioning and offers thoughts on ways to provide early restraint by seatbelts.
Methods
Rear sled tests were conducted at 40.2 km/h (25 mph) delta V with a lap-shoulder belted, instrumented 50th Hybrid III. The dummy instrumentation included head, chest and pelvis triaxial acceleration and upper and lower neck triaxial loads and moments. Lap and shoulder belt loads were measured. High-speed video recorded different views of the occupant kinematics. In the first series, two sled tests were conducted with a Ford F-150 driver seat. One test was with the standard lap-shoulder belts only and a second with buckle pretensioner activation. In the second series, three matched tests were conducted with a Ford Escape driver seat. One test was with the lap-shoulder belts only, a second with retractor and anchor pretensioning and a third with only retractor pretensioning. The analysis included occupant kinematics, lap-belt movement and estimation of the load on the occupant’s torso. The load was the sum of force on the upper and lower torso. The upper torso mass was 30.8 kg (67.8 lb) based on GEBOD data for the 50th Hybrid III. It was multiplied by the resultant chest acceleration to calculate the upper torso force. The lower-torso mass was 30.9 kg (68.0 lb). It was multiplied by the resultant pelvic acceleration to calculate the lower torso force. The total load on the seatback was the sum of the upper and lower torso force. The change in angle (θ) of the lap belt was determined by video analysis. The angle θ was from the horizontal up to a line through the lap-belt webbing. Ways to provide early lap-belt restraint were considered.
Results
The rear sled testing at 40.2 km/h (25 mph) showed that the seatbelt provided essentially no restraint of the rearward movement of the occupant. The seat provided essentially all of the rearward restraint with and without pretensioning. There was minimal lap belt load in the series with the dual recliner Escape seat, except for a spike caused by pretensioning. There was more seat deformation in the tests with the single-side recliner F-150 seat. There were higher belt loads. The lap belt limited the lifting of the hips and thighs with essentially no rearward restraint of the occupant. Tension in the lap belt did not relate to restraint of rearward movement of the occupant. Seatbelts provided forward restraint of the occupant during rebound with the belts providing noticeable deceleration of the chest and pelvis. Concepts were considered to provide early lap-belt restraint. One involved a rear pretensioner that dynamically moves the lap-belt anchor forward and upward while tightening the belts in a rear impact. This provides a lap-belt angle greater than θ = 90 deg before occupant movement. With this geometry, the lap belt restrains rearward movement of the occupant and pulls the hip down early in a rear impact.
Conclusion
Seatbelts and pretensioners were designed for occupant restraint in frontal crashes, so it is not a surprise they do not provide much restraint of an occupant in rear impacts up to 40.2 km/h (25 mph). The lack of early lap-belt restraint is due to the unfavorable belt angle from the anchors over the hip. A concept is discussed that dynamically moves the anchors in rear impacts to provide early belt restraint.
Introduction
Field data on seatbelt effectiveness in rear impacts
Numerous field accident studies have found significant seatbelt effectiveness in preventing death and injury in rear crashes (Strother and James Citation1987; Evans 1991; Kahane Citation2000, Citation2015; Viano and Parenteau Citation2010, Citation2022). Evans (1991) used the double-pair comparison method and found that seatbelts were 49 ± 14% effective in preventing fatal injury in rear impacts and 22 ± 1% effective in preventing driver ejection in rear impacts. The FARS data involved driver seats in vehicles from the 1970s–1980s with single-side recliners and moment strength of 1,091 ± 333 Nm (9,652 ± 2,949 inlb) and peak force of 3,069 ± 952 N (690 ± 214 lb), n = 94 (Viano and White Citation2016).
Malliaris (Citation1990) reported that seatbelts were 48% effective when cars were struck in the rear by another vehicle without additional impacts and 62% effective in rear impacts followed by a frontal impact. He also reported that contact with a frontal interior was the most common source for injury. Kahane (Citation2000, Citation2015) used the double-pair comparison method to determine seatbelt effectiveness in 1986–1999 FARS. Seatbelt use reduced fatalities by 56% in passenger cars and 81% in light trucks in rear impacts. Viano and Parenteau (Citation2010) found lap-shoulder belts were 92.7% effective in preventing serious injury (MAIS 3 + F) for non-ejected occupants in rear impacts. Viano and Parenteau (Citation2022) analyzed the risk for severe-to-fatal injury (MAIS 4 + F) by crash type, delta V and seatbelt use using 1994–2015 NASS-CDS. Seatbelt use was 84.5% ± 41.8% effective in reducing the risk for MAIS 4 + F in rear impacts. Clearly, seatbelts restrain forward movement of the occupant during rebound, which reduces injury risks.
Rear crash testing
Strother and James (Citation1987) compared belted and unbelted dummy biomechanics in FMVSS 301 NCAP tests involving 1979–1981 MY vehicles and did not find significant differences. The authors reviewed kinematics and found that head strikes were generally higher for unbelted occupants than belted occupants. The videos with the belted dummies showed the thighs and knees move upward and rearward in response to the forward vehicle acceleration. This motion prevented the belted dummies from slipping out of the lap belt. Seatbelts were beneficial in rear impacts by controlling or eliminating ramping up the seatback, reducing the velocity of the occupant relative to the vehicle interior, minimizing the potential for occupants to be out-of-position (OOP) at impact, and controlling forward rebound. However, the seatbelt had no effect until the seat had substantially deformed rearward and the occupant had displaced into the rear occupant compartment. This was very late. The findings are consistent with later studies (James et al. Citation1991; Digges et al. Citation1993).
Viano et al. (Citation2008) investigated full-width FMVSS 301 rigid barrier tests with belted occupants in 1995–2005 model year vehicles. There were 79 crash tests. The barrier weighed 1,818 kg (4,000 lb). The average delta V was 28.2 ± 2.7 km/h (17.5 ± 1.7 mph) and vehicle weight was 1,629 ± 1,091 kg (3,583 ± 678 lb). The head accelerations and HICs, neck forces and moments and chest accelerations were consistently below IARVs (injury assessment reference values). A review of the videos indicated that good occupant kinematics, and biomechanical responses were well below injury tolerances. Most of the occupant restraint was provided by yielding of the seat. The lap belt remained on the pelvis and thighs. The amount of restraint of rearward movement of the occupant by the lap belt was not determined.
Viano et al. (Citation2008) also analyzed 21 FMVSS 301 R offset deformable barrier (ODB) rear tests at 80.5 km/h (50 mph) with belted occupants in 1993–1998 model year vehicles. The average delta V was 43.5 ± 4.8 km/h (27.0 ± 2.3 mph) and the vehicle weight was 1,440 ± 811 kg (3,169 ± 504 lb). A review of the videos indicated that intrusion occurred before much rearward displacement of the occupant and that seatback rotation rearward was limited by contact with the rear seat as rear structures were pushed forward. The seat could not transfer sufficient energy before rotating into contact with the rear seat cushion. The amount of rearward restraint by the belts was not determined.
Tatem and Gabler (Citation2017) studied serious‐to‐fatal rear impact crashes and found that crush of the occupant compartment was an important factor in rear impact injuries. This is consistent with the higher risk for severe injury to the drivers in rear impacts due to intrusion into their seating area that supported or pushed the driver’s seat forward (Viano and Parenteau Citation2012). Parenteau and Viano (Citation2022) found that 64.3% of injury to rear-seat adults and 86.1% of injury to rear-seat children intrusion of the rear seat.
Pretensioning in rear impacts
Seatbelts were first pretensioned in rear crashes in the 1997 Saab 9-5 and 9-3 with the introduction of an active head restraint in a high retention seat (Viano and Olsen Citation2001; Viano Citation2002). The retractor pretensioner was activated in crashes above 24 km/h (15 mph) delta V to tighten the belts. The activation was based on the logic that pretensioning could “do no harm” and might offer safety benefits in severe rear impacts. Today, there is a mix of automotive manufacturers that do or do not fire pretensioners in rear impacts (Edwards et al. Citation2019). There are several types of pretensioners. Buckle pretensioners shorten the arc (reduces the radius) for the lap belt on the inboard side of the seat. Retractor pretensioners tighten the webbing of the shoulder belt by back-winding the retractor spool. Anchor pretensioners shorten the length of the lap belt on the outboard side at the floor anchor. Currently, there are no pretensioner designs specifically for rear impacts.
Rear impact kinematics
The occupant moves rearward relative to the interior and away from the seatbelts in a rear impact. The shoulder belt becomes unloaded. The lap belt moves in an arc from forward around the pelvis to rearward as the occupant pockets into the seatback and moves up the seatback (Viano et al. Citation2018). The thighs normally rise up with occupant loading into the seatback, trapping the lap belt on the pelvis (Strother and James Citation1987; James et al. Citation1991).
The amount of rearward movement of the occupant depends on the severity of the crash, size and posture of the occupant and strength and compliance of the seat. The seat transfers energy to the occupant in a rear crash with the goal of providing favorable occupant kinematics and low biomechanical responses (Viano Citation2022). The seat and seatbelt are intended to provide occupant restraint. It is desirable that the seatbelt provide early restraint of rearward movement of the occupant.
This study focuses on the restraint provided by the lap belt in rear impacts. While field data consistently shows effectiveness of seatbelts in rear impacts and rear crash testing shows rearward restraint by the lap belt very late in the crash, the issue is how to provide early lap-belt restraint. The issue is addressed by considering why the lap belt does not provide early restraint by analyzing the seatbelt in rear sled tests at 40.2 km/h (25 mph) delta V with and without pretensioning. Concepts were considered to increase lap-belt restraint early in rear impacts. The study is intended as a “thought-starter” on how to improve seatbelts in rear impacts.
Methods
Two series of matched rear sled tests at 40.2 km/h (25 mph) were analyzed to determine the amount of occupant restraint provided by the lap-shoulder belts with and without pretensioning. The tests have been reported on previously, but not analyzed with the purpose of this study.
Sled test series 1
Two rear sled tests were conducted at the Ford Safety Center in Dearborn, Michigan with a 2008 F-150 buck (Viano et al. Citation2012). The tests involved the same setup with a lap-shoulder belted 50th Hybrid III dummy on the driver seat. The buck included the 2nd row seat, rear window and other interior components. The tests were run with new 2008 F-150 bucket seats having a 21 deg seatback angle rear of vertical. The driver seat was in the mid-track position and the head restraint was full-up.
The first test (H31208) involved the standard lap-shoulder belts. The second test (H31209) involved buckle pretensioning at 20 ms. The belt systems were identical except for the buckle pretensioning. It was a 3-point continuous loop system with a sliding latch plate, webbing routed through a D-ring and single retractor mounted at the base of the B-pillar. The rear sled tests were at 40.2 km/h (25 mph) rear delta V and 15.7 g peak sled acceleration. High-speed video captured occupant kinematics and seat deformations from different perspectives. The Hybrid III dummy was instrumented with an array of transducers to measure biomechanical responses. Lap and shoulder belt loads were measured. For more details, go to Viano et al. (Citation2012).
The 2008 Ford F-150 driver seat is a yielding design that uses a single recliner on the outboard side and a pivot on the inboard side of the seat frame. A body block test was conducted on a 2004 Ford F-150 seat, which is the 2004–2008 model run. The test involved a rearward load on the seat through a wooden block with the shape of an occupant’s pelvis and lower back (Viano and White Citation2016). The test resulted in a 1,657 Nm (14,658 inlb) peak moment and 4,660 N (1,047 lb) peak load.
Sled test series 2
Three rear sled tests were conducted at the Ford Safety Center in Dearborn, Michigan with a 2016 Ford Escape buck (Viano et al. Citation2021a). The tests involved the same setup with a lap-shoulder belted 50th Hybrid III dummy on a 2013 Escape manual, 6-way adjustable seat. The tests were run with the seatback 21 deg rearward of vertical. The driver seat was in the mid-track position, cushion adjustments were full down and the head restraint was full-up. The center console was removed to have a clear view of the seatbelt buckle on the inboard side.
The first test (H41148) involved the standard lap-shoulder belts. The second test (H41149) involved retractor and anchor pretensioning at 60 ms. The third test (H41150) involved retractor pretensioning at 60 ms. The lap-shoulder belt was identical except for the pretensioning. It was a 3-point continuous loop system with a sliding latch plate, webbing routed through a D-ring and single retractor mounted at the base of the B-pillar. The rear sled tests were at 40.2 km/h (25 mph) delta V and 15.7 g peak sled acceleration following a protocol developed by Ford and described by Viano et al. (Citation2018). High speed video captured dummy kinematics, belt restraint and seat deformation from different perspectives. The Hybrid III dummy was instrumented with an array of transducers to measure biomechanical responses. For more details, go to Viano et al. (Citation2021a).
The 2013 Ford Escape driver seat uses dual recliners on the outboard and inboard sides seatback frame. A body block test was conducted on a 2016 Ford Escape seat in the 2013–2019 model run (Viano and White Citation2016). The body block test gave a peak moment of 2,520 Nm (22,299 inlb) and peak force of 7,089 N (1,593 lb).
Load on the torso and lap-belt angle θ
Chest and pelvic accelerations were analyzed to estimate the load on the torso by the seatback in the sled tests. The load was estimated as the sum of force from the upper and lower torso. The upper torso was defined using the GEBOD segments for the upper torso, center torso and right and left upper arms of the 50th Hybrid III (Cheng et al. Citation1994). The mass of these segments was 30.8 kg (67.8 lb) based on GEBOD weights of 24.5 kg for the upper torso, 2.4 kg for the center torso and 2.0 kg for the right and left upper arms of the 50th Hybrid III. The mass of the upper torso was multiplied by the resultant chest acceleration to calculate the force on the upper torso. The lower torso was defined using the GEBOD segments for the lower torso and right and left upper legs of the 50th Hybrid III. The mass of the lower torso was 30.9 kg (68.0 lb) based on 11.7 kg for the lower torso and 9.6 kg for the right and left upper legs. The mass of the lower torso was multiplied by the resultant pelvic acceleration to calculate the force on the lower torso. The total load on the torso from the seatback was the sum of the force on the upper and lower torso. The lap-belt angle θ is between the horizontal and line tangent to the lap-belt webbing up from the anchor in a side view.
Concepts for early lap-belt restraint in rear impacts
The reason for a lack of lap-belt restraint early in rear impacts is the unfavorable belt angle θ from the anchor over the hip. In normal use the angle θ < 90 deg, so load in the belt can only restrain forward movement of the occupant. Concepts were considered to get a favorable angle θ > 90 deg before the occupant moves on the seat. One concept is to dynamically move the anchors upward and forward early in rear impact by a specifically designed rear pretensioner. The rationale is discussed as a “thought starter.”
Results
Sled test series 1
shows an overlay of occupant kinematics at 0 and 200 ms from the onboard high-speed video with buckle pretensioning in test H31209. The lap belt extends upward and forward of the hip at an angle θ. The initial angle θ = 69 deg increased to θ = 109 deg at 200 ms, just at the start of rebound. The lap belt moves rearward in an arc around the anchor on the lower portion of the seat cushion. in the Appendix (see online supplement) shows side-by-side images of occupant kinematics at 0, 80, 120, 160 and 200 ms with and without buckle pretensioning in the 40.2 km/h (25 mph) rear sled tests. The kinematics without pretensioning are similar. The single-recliner seat had a moment strength of 1,657 Nm (14,658 inlb). The seat deflected as it transferred energy to the occupant.
shows the total load on the torso from the seatback in the matched tests. The load was essentially similar up to rebound at 200 ms with and without buckle pretensioning. There was minimal load in the lap belt until about 125 ms without pretensioning. The load was higher in the lap belt with pretensioning after 125 ms. There was an increase in lap-belt load with pretensioning at 25 ms and then another increase starting at 100 ms to a peak before rebound with pretensioning. The peak lap-belt load was 533 N without and 1,159 N with buckle pretensioning. shows occupant kinematics and the lap-belt angle from video images at 0 and 200 ms with buckle pretensioning.
At time zero, the lap belt wraps forward and around the pelvis. The angle θ = 69 deg up from horizontal. As the occupant displaces rearward, the belt moves with the pelvis. By 80 ms, the lap belt angle is θ = 82 deg and by 100 ms the angle is beyond vertical with θ = 103 deg. shows the change in lap-belt angle in test H31209. It also shows the cosine of the angle, which is used to compute the horizontal component of the lap-belt load that resists rearward movement of the occupant. For θ < 90 deg, the horizontal load resists forward movement of the occupant and provides no restraint in the rearward direction. For θ > 90, the horizontal load is in the rearward direction and resists occupant movement rearward. The bottom graph in shows the component of load in the belt in the horizontal direction. With buckle pretensioning, θ was > 90 deg after 100 ms. The horizontal load reached −244 N at 180 ms, just before the start of rebound at 200 ms. The horizontal load of − 244 N involves 0.4 g restraint of the occupant’s torso. This is a negligible restraining force in a test of this severity; the lap belt provides essential no occupant restraint until rebound.
By 160 ms, the angle θ = 109 deg and the pelvis has maximum rearward displacement. The rearward movement of the lap belt is in an arc around the anchor for the lap belt. There is only a small vector of force restraining the rearward movement of the occupant after 80 ms. The force results in < 0.4 g deceleration of the torso in the x-direction, which has negligible influence on the rearward movement of the occupant. The lap belt restrains lifting of the pelvis and thighs. The higher force in the belt with buckle pretensioning is associated with more downward restraint of the pelvis with the shorter length of the lap belt with a smaller radius of the arc.
Sled test series 2
shows an overlay of occupant kinematics from the onboard high-speed video at 0 and 140 ms with retractor pretensioning in test H41150. The initial angle θ = 70 deg increased to θ = 107 deg at 140 ms, the start of rebound. The lap belt moved rearward in an arc around the anchor on the lower portion of the seat cushion. in the Appendix shows side-by-side images of occupant kinematics from the onboard high-speed video at 0, 80, 100, 120 and 140 ms without pretensioning, with retractor and anchor pretensioning and with only retractor pretensioning in 40.2 km/h (25 mph) rear sled tests. The kinematics are essentially the same in the three tests. The dual-recliner seat had a moment strength of 2,520 Nm (22,299 inlb). The seat deflected rearward less than in series 1 as it transferred energy to the occupant, because of the higher strength. Rebound started earlier.
Figure 4. Load on the torso and lap belt in matched tests with and without retractor and retractor and anchor pretensioning.
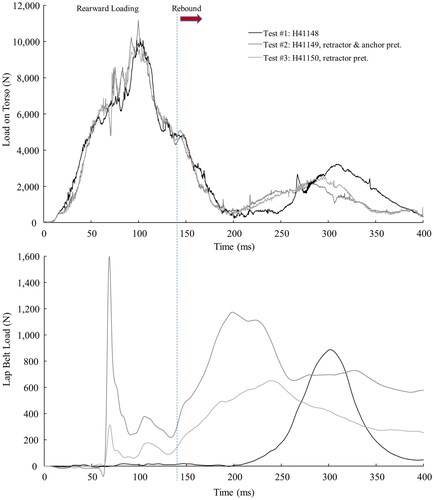
shows the calculated load on the torso and the force in the lap belt in the matched tests. The load on the torso was essentially similar up through rebound at 140 ms. There were higher loads in the lap belt with pretensioning, but the force was less than 200 N with retractor pretensioning and less than 400 N with retractor and anchor pretensioning after the spike in tension with pretensioner activation. The transient increase in lap-belt load was up to 1,600 N retractor and anchor pretensioning 60 ms and it was less than 300 N with retractor only pretensioning. The forces in the lap belt were very different during rebound with the pretensioned belts, which provided earlier restraint of forward movement of the occupant.
shows the occupant kinematics and lap-belt angle at 0 and 140 ms with retractor pretensioning in test H41150. shows the angle of the lap belt, and the horizontal load from the belt. At time zero, the lap belt wraps forward and around the pelvis. The angle is θ = 70 deg up from horizontal. By 80 ms, the lap belt angle is θ = 102 deg, which is past vertical. By 140 ms, the angle is θ = 104 deg at maximum rearward displacement of the pelvis. The lap belt has a small vector of the force in the forward direction after 80 ms, which restrains the rearward movement of the occupant. The force is inconsequential and has negligible influence on the rearward movement of the occupant. The lap belt restrains lifting of the pelvis and thighs. The higher force in the belt with retractor and retractor and anchor pretensioning is associated with more downward restraint of the pelvis with the tightened lap belt with retractor pretensioning and the tightened and shorter length of the lap belt with retractor and anchor pretensioning.
Concepts for early lap-belt restraint in rear impacts
Seatbelts have the anchor point rearward of the belt that wraps around the occupant. The anchors need to be where they are for occupant restraint in frontal crashes. It is not possible to provide occupant restraint in the rearward direction unless the anchor points are forward of the lap belt that wraps over the pelvis. A concept is presented that dynamically moves the anchor points for the lap belt early in rear impacts to develop restraining loads from the seatbelt.
shows the conventional lap belt wrapping forward and around the pelvis with a lap-belt angle θ = 71 deg. The lap belt moves in the initial arc around the anchor on the cushion frame of the seat. The arc is consistent with the sled tests shown in and . The occupant kinematics can be changed if the anchor is dynamically moved forward and upward, as shown in the moved anchor position. The moved anchor is by activation of a rear pretension that shifts the anchor locations and tightens the belts. With the new anchor positions, the lap belt has a different arc that is shorter with the anchor moved up and forward.
The rear pretensioner moves the lap-belt anchors causing a lap-belt angle is increased to θ = 128 deg early in a rear impact. This gives a 62% component of the lap load in the horizontal direction to restrain rearward movement of the occupant. The lap-belt angle increases with rearward movement of the pelvis. Force in the lap belt restrains rearward movement of the occupant and there is a larger downward component of force on the pelvis. With conventional lap belt geometry, the hip and lower torso gain speed with respect to the seat increasing the tendency to slide out of the lap belt because the seatbelt provides essentially no early restraint of rearward movement of the occupant in tests up to 40.2 km/h (25 mph). With the moved anchor position, the arc length of the belt is shorter and the belt engaged the pelvis earlier minimizing the tendency to slip out of the lap belt in a rear impact.
The lap belt anchor is initially low on the track or floor. Rear pretensioning dynamically moves the anchors to a new position on the seat frame for the remainder of the crash. By moving the anchor up and forward, the radius of the lap belt webbing passing over the occupant is reduced. The anchors can have load-limiting components in the new position to improve control of occupant motion. The concept has not been evaluated in sled testing. Only static evaluations have been performed. The concept is present as a “thought-starter” to stimulate consideration of other concepts that would provide early restraint of an occupant in rear impacts.
Discussion
There are five reasons why there is a lack of restraint with pretensioning current seatbelts in 40.2 km/h rear impacts. First, the rearward movement of the dummy is away from the lap and shoulder belts causing some slack in the shoulder belt that normally routes forward around the torso and shoulder. The slack does not influence occupant kinematics. Second, the belts wrap around and forward of the dummy’s pelvis and chest. The belts are normally tight with spring tension from the retractor. The lap belt is angled forward as it wraps around the pelvis. The lap belt moves rearward shortening the arc but the webbing remains “in place” on the pelvis. Third, retractor and anchor or retractor pretensioning caused a spike in lap belt load, but it was a short duration increase without sustained tension in the belt to influence occupant responses. Tightening of the belts and pulling the latch down limits the upward movement of the hip and thighs. Fourth, the transient increase in lap-belt load with pretensioning has no effect on restraining the rearward movement of the occupant and does not influence the load on the torso from the seat. There is no significant change in the acceleration of the chest or pelvis. The dummy responses were essentially similar with or without pretensioning. Fifth, the strength of the seats was sufficient to contain the occupant restraint in these sled tests.
Seatbelts do not have a primary role in restraining the occupant in 40.2 km/h rear impacts with modern seats. The yielding seat provides the necessary acceleration forward through the rear delta V and it is not unexpected that pretensioning the seatbelt did not have an effect on occupant kinematics or biomechanical responses until rebound. Tensioning the seatbelts is not helpful for several reasons: (1) the angle of the lap belt to the anchor is less than 90 deg and the belt cannot provide restraint of rearward movement until the angle is greater the 90 deg. The component of belt load in the forward direction is related to the cosine of the angle θ shown in , (2) the occupant pockets into the seatback without ramping and yielding of the seatback provides gradual acceleration through the delta V and (3) the occupant is moving rearward away from the belts. Conventional seatbelts have the lap-belt anchors rearward of the wrap-around of the belts on the pelvis and torso. Current pretensioners tighten the belts but do not alter the rearward position of the anchors. The lap belt anchors need to be forward of the lap belt wrapping around the pelvis to provide occupant restraint early in a rear impact. It can be achieved dynamically with a specifically designed rear pretensioner that moves the belt anchors while tightening the belts.
Occupant kinematics in high-speed rear impacts differ from low-speed kinematics, seen in the 16.1 km/h (10 mph) IIHS rear sled test. At high speeds, the energy transfer from the seat to the occupant typically exceeds the strength of the seat causing plastic deformation of the recliners and seat frame with a seatback angle sufficient large that the occupant ramps up the seatback (Viano et al. Citation2021b). The amount of energy transfer depends on the crash severity (delta V) and occupant weight among other factors (Viano Citation2022). The lap belt only provides restraint when the occupant has displaced into the rear occupant compartment and the belt angle is sufficient for lap belt loads to restraint the movement. In low-speed rear impacts, the seatback and head restraint displace into the occupant. The foam trim compresses and the seatback rotates rearward depending on the strength of the seat. The energy transfer levels are usually low enough that the lap belt angle moves to 90 deg or slightly more. The lap belt provides no horizontal restraint of the occupant. The belt can provide restraint of vertical motion, but this is typically negligible in the low-speed testing. Clearly, lap-shoulder belts provide restraint once the occupant rebounds in low- and high-speed impacts.
There is a need to modify current lap-shoulder belts and pretensioners to achieve early restraint of the rearward movement of an occupant in rear impacts. A purpose of a rear pretensioner concept is to provide restraint early by dynamically moving the anchors upward and forward in rear impacts. With the anchors moved, the lap-belt geometry has θ > 90 deg, so load in the belt restrains rearward movement of the occupant and pulls the hip down early in a rear impact. This reduces forces on the seatback and reduce loads supported by the recliners. It would lower deformation of the seat. Moreover, the moved anchors with a rear pretensioner would increase the horizontal and vertical restraint of the occupant early in a rear impact, lowering the tendency for ramping up the seatback. While the concept considered involves dynamically moving the lap-belt anchors, adding a second set of static anchors that are forward and up from the existing anchors is an option. Whatever is done for rear impacts should not interfere with restraint function in other crashes.
Limitation
The restraining loads in the lap belt hold the occupant on the seat reducing the potential for ejection. Ejection is defined as the occupant moving outside the occupant compartment of the vehicle. Evans (1991) found that the lap belt was the primary restraint preventing ejection from the vehicle in rear impacts. He reported the lap belt provided 23% of the overall 49% effectiveness of seatbelts in preventing fatal injury in rear crashes. The rear pretensioner is intended to increase the seatbelt effectiveness in rear impacts. The seats and seatbelts in the FARS sample were from 1970s–1980s vehicles in Evans’ study. The fact that the lap belt reduced the risk for ejection indicates the belt was eventually providing restraint beyond the severities of crash evaluated here and with occupant kinematics not seen in these tests.
Supplemental Material
Download Zip (557.9 KB)Acknowledgments
The sled test data and videos may be made available upon request. The author has no direct or indirect financial interest in any pretensioner concept discussed in this study. The rear pretensioner has been discussed in a US patent application 17693977 owned by a non-profit devoted to supporting research and education on automotive safety and occupant protection. Another concept is a wrap around seatbelt, which is discussed in US 10,946,829 B2, issued 3-16-21.
Funding
The author(s) reported there is no funding associated with the work featured in this article.
References
- Cheng H, Obergefell, Rizer A. 1994. Generator of body (GEBOD) manual. AL/CF-TR-1994-0051. Armed Forces Materiel Command, Wright-Patterson Air Force Base, Ohio, March.
- Digges KH, Morris JH, Malliaris AC. 1993. Safety performance of motor vehicle seats. SAE 930348. Society of Automotive Engineers, Warrendale, PA.
- Edwards MA, Brumbelow ML, Trempel RE, Gorjanc TC. 2019. Seat design characteristics affecting occupant safety in low- and high-severity rear-impact collisions. IRCOBI Conference. IRC-19-11.
- Evans L. 1991. Traffic safety and the driver. New York (NY): Van Nostrand Reinhold.
- James MB, Strother CE, Warner CY, Decker RL, Perl TR. 1991. Occupant protection in rear-end collisions: I. Safety priorities and seat belt effectiveness. 35th Stapp Car Crash Conference, SAE 912913, Society of Automotive Engineers, Warrendale, PA. doi:10.4271/912913
- Kahane CJ. 2000. Fatality reduction by safety belts for front-seat occupants of cars and light truck. NHTSA Technical Report HS 809 199, National Highway Traffic Safety Administration, Washington, DC, December.
- Kahane CJ. 2015. Lives saved by vehicle safety technologies and associated federal motor vehicle safety standards, 1960 to 2012—passenger cars and LTVs—with reviews of 26 FMVSS and the effectiveness of their associated safety technologies in reducing fatalities, injuries, and crashes. DOT HS 812 069, National Highway Traffic Safety Administration, Washington, DC, January.
- Malliaris AC. 1990. Current issues of occupant protection in car rear impacts. Data Link, Docket 89-20-NO1.
- Parenteau CS, Viano DC. 2022. Serious injury to 2nd row occupants in rear impacts using 1994–2020 field data. Traff Inj Prev.
- Strother CE, James MB. 1987. Evaluation of seat back strength and seat belt effectiveness in rear end impacts. 31st Stapp Car Crash Conference, SAE 872214, Society of Automotive Engineers, Warrendale, PA. doi:10.4271/872214
- Tatem WM, Gabler HC. 2017. Preliminary analysis of serious‐to‐fatal injury in rear impact crashes in the United States. # IRC-17-15, IRCOBI Conference.
- Viano DC. 2002. Role of the seat in rear crash safety. Warrendale (PA): Society of Automotive Engineers. SAE R-317:1–491.
- Viano DC. 2022. Motion sequence criteria for favorable kinematics in rear impacts. Traff Inj Prev.
- Viano DC, Burnett R, Miller GA, Parenteau CS. 2021a. Influence of retractor and anchor pretensioning on dummy responses in 40 km/h rear sled tests. Traffic Inj Prev. 22(5):396–400. doi:10.1080/15389588.2021.1910243
- Viano DC, Burnett R, White S, Stephens G, Parenteau CS. 2021b. Dual-recliner ABTS seats in severe rear sled tests with the 5th, 50th and 95th hybrid III. SAE 2021-01-0917, Society of Automotive Engineers, Warrendale, PA.
- Viano DC, Olsen S. 2001. The effectiveness of active heads restraint in preventing whiplash. J Trauma. 51(5):959–969. doi:10.1097/00005373-200111000-00022
- Viano DC, Parenteau CS. 2010. Ejection and severe injury risks by crash type and belt use with focus on rear impacts. Traffic Inj Prev. 11(1):79–86. doi:10.1080/15389580903479186
- Viano DC, Parenteau CS. 2012. Front-to-rear crashes involving two-vehicles with severe driver injury. Traffic Inj Prev. 13(1):55–60. doi:10.1080/15389588.2011.625735
- Viano DC, Parenteau CS. 2022. Injury by delta V in front, near-side, far-side and rear impacts: analysis of 1994–2015 NASS-CDS. SAE 2022-01-0835, Society of Automotive Engineers, Warrendale, PA.
- Viano DC, Parenteau CS, Burnett R. 2012. Influence of belt pretensioning on dummy responses in 40 km/h rear impact sled tests. Traffic Inj Prev. 13(1):65–71. doi:10.1080/15389588.2011.629558
- Viano DC, Parenteau CS, Burnett R, Prasad P. 2018. Occupant responses in conventional and ABTS seats in high-speed rear sled tests with a normally seated dummy. Traffic Inj Prev. 19(1):54–59. doi:10.1080/15389588.2017.1347782
- Viano DC, Parenteau CS, Prasad P, Burnett R. 2008. Occupant responses in high-speed rear crashes: analysis of government-sponsored tests. SAE 2008-01-0188, Society of Automotive Engineers, Warrendale PA.
- Viano DC, White S. 2016. Seat strength in rear body block tests. Traffic Inj Prev. 17(5):502–507. doi:10.1080/15389588.2015.1111513