Abstract
Objective
Rear-impact restraint guidelines have not developed to the same degree as for frontal crashes. This study provides criteria for favorable occupant kinematics in rear impacts.
Methods
Rear criteria were developed as an extension of Adomeit and Heger (Citation1975) and Adomeit (Citation1977) motion sequence criteria (MSC) for favorable occupant kinematics in frontal crashes. In this study, occupant kinematics in rear sled tests were studied to develop motion sequence criteria for favorable and unfavorable occupant kinematics in rear impacts with containment of the hip on the seat and no ramping up the seatback.
Results
Rear MSC limit the angle of the torso (α) rearward of vertical to less than the critical angle (αc) for ramping up the seatback and H-pt displacement rearward and downward, so zHpt < zHpt0, where zHpt0 is the initial height of the H-pt. The lateral displacement of the occupant is limited to less than the critical lateral displacement yT1c, where the head becomes unsupported by the head restraint or the chest by the seatback. The rear MSC contain the pelvis on the seat and provide uniform support of the torso, head and neck. Most front seats in production provide reasonably favorable occupant kinematics in rear impacts up to 40 km/h (25 mph) delta V with the 50th Hybrid III. Kinematics become unfavorable in testing at higher severities and with heavier occupants. The amount of energy that the seat needs to transfer to the occupant in a rear impact depends on the delta V (ΔV or change in velocity) of the vehicle and the mass of the occupant (m) among other variables. The seat provides an interface with the occupant and transfers energy (E), which can be approximated by E = ½*0.7*m(ΔV)2 using 70% of the occupant mass (m) and delta V. Rear MSC provide performance guidelines to advance seat designs with favorable occupant kinematics at higher energy transfer levels in rear impacts. Sled testing at 40 km/h (25 mph) involves an energy transfer of 3,421 J with the 50th Hybrid III generally gives favorable kinematics. A 56.3 km/h (35 mph) test involves 6,704 J, double the energy transfer and often unfavorable kinematics. A target energy needs to be set, and there are practical limits because the energy transfer is 12,858 J with a 150 kg (330 lb) occupant in a 56.3 km/h (35 mph) delta V rear crash.
Conclusion
Rear motion sequence criteria (MSC) define favorable kinematics in rear impacts. MSC complement the assessment of biomechanical responses in sled and crash testing to ensure an overall evaluation of occupant restraint in rear impacts.
Introduction
Principles of occupant restraint in frontal impacts with motion sequence criteria (MSC)
Adomeit and Heger (Citation1975) and Adomeit (Citation1977) defined motion sequence criteria for favorable and unfavorable occupant restraint by lap-shoulder belts and seat in frontal crashes. The work focused on the 48.3 km/h (30 mph) frontal crash test in FMVSS 208 (Adomeit Citation1979). (top) shows favorable restraint kinematics with the occupant leaning into the shoulder belt and the lap belt applying load on the bony structures of the pelvis. The hip moves along an arc forward and down following the arc of the lap belt around its anchor on the seat or floor.
Figure 1. (top) favorable motion sequence into the lap-shoulder belts, (bottom) unfavorable motion sequence with submarining the lap belt (modified from Adomeit and Heger Citation1975).
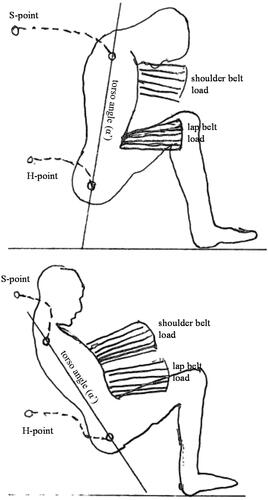
(bottom) shows unfavorable restraint with the occupant’s torso and hip rotated rearward (counterclockwise rotation), the lap belt loading soft tissues of the abdomen above the ASIS (anterior-superior iliac spine) and the shoulder belt loading the lower ribs of the thorax. The hip motion causes submarining of the lap belt, where submarining is defined as the lap belt moving above the ASIS into the abdomen with sufficient force to injure organs or the spine. Unfavorable kinematics involved a risk for injury to the 1) neck by sliding along the shoulder belt webbing, 2) thorax when the torso angle was greater than 90 degrees resulting in shoulder belt load in the area of ribs 6-12, 3) abdomen by compressing internal organs as the lap belt moves above the iliac crest and 4) knees moving forward into the instrument panel due to forward displacement of the H-point.
Adomeit and Heger (Citation1975) used the favorable kinematics to define motion sequence criteria for occupant restraint in frontal impacts. shows the motion sequence criteria (MSC) for favorable kinematics using the trajectory of the H-point (H-pt, center of the hip) and torso angle (α), which was defined as the angle between the H-point and S-point (S-pt, center of the shoulder) with α the initial position and α’ during occupant restraint. The MSC limit: 1) H-pt movement forward to 125-200 mm with downward motion of 30-40 mm and no rearward rotation of the pelvis (counterclockwise rotation) and 2) torso rotation forward from the initial design position of α = 105-120 deg torso angle to α’ = 70-90 deg at maximum shoulder belt load. MSC complement the control of biomechanical responses measured in the Hybrid III dummy in frontal crash tests. Adomeit and Heger (Citation1975) and Adomeit (Citation1977) did not develop MSC for favorable kinematics in the lateral direction in frontal crashes.
Figure 2. Motion sequence criteria for occupant restraint in frontal impacts (modified and updated from Adomeit and Heger Citation1975 and Adomeit 1977).
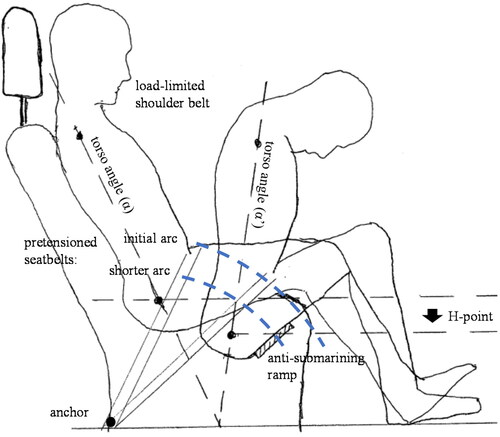
includes restraint features that were not used in the early 1970s. The restraint systems and seats in modern vehicles include a seatbelt retractor with load-limiter so that shoulder belt webbing unwinds above a threshold force allowing the upper torso to rotate forward into the shoulder belt and airbag. The seatbelt has one or more pretensioners that deploy at the beginning of the crash, tightening the belts and removing slack to increase ride-down by the occupant during deformation of front structures of the vehicle. This shortens the arc of the lap belt ensuring load on the anti-submarining ramp.
Modern seats include an anti-submarining ramp that supports the pelvis and minimizes rearward rotation of the hip that is associated with submarining. Anti-submarining ramps have various designs including cross-seat tubes, inclines, ramps and sheet metal pans, and they have many shapes and forms in seats. The top of the ramp is above the bottom of the pelvis. It restrains the forward movement and limits rearward rotation of the pelvis, which is pulled down by the arcing forward and downward motion of the lap belt about the anchor on the seat or floor. The use of an anti-submarining ramp in the seat, load-limiting shoulder belt and pretensioning the seatbelt promote favorable kinematics in frontal crashes.
Ramping
In a low-speed rear impact, the occupant moves rearward and is supported by the seatback and head restraint. The seatback rotates rearward depending on the severity of the crash, weight of the occupant and other factors. As the severity of the load increases, there is greater seatback rotation until the occupant starts to slide up the seatback. This is called ramping. Ramping is defined as the hip and lower back sliding up the seat more than 2” (5 cm), using a definition from analysis of 40 km/h (25 mph) rear sled tests (Viano et al. Citation2021a). The operative word is slide by overcoming the dynamic friction between the occupant and the seatback. It is whole body movement. Straightening of the kyphotic and lordotic curvature of the spine occurs in low-speed volunteer testing. This causes the head and shoulder to rise in a manner the dummies do not duplicate. The straightening of the human spine is not ramping, because it does not involve the whole body sliding of the hips and lower back up the seat.
Hip movement within <2” (<5 cm) is normal in 40 km/h (25 mph) rear sled tests and is the accommodation of the hips and lower back against the trim and foam as the seatback is loaded. There is always rotation and displacement of the hips as load increases on the seatback. Hip pocketing into the seatback can involve the H-pt displacing rearward up to 6” (15 cm) before much seatback rotation. When the seatback rotates the hip lifts off the seat cushion. This is the geometric effect of pocketing and seatback rotation that occurs without ramping or sliding movement of the occupant up the seatback. Ramping up the seatback is dynamic sliding and once the body starts sliding up the seatback, it is difficult to stop without sufficient plowing friction or restraining forces from the seatbelt.
Seat characteristics
The primary seat characteristics are strength and stiffness of the seat frame among other variables (Viano Citation2003b). Stiffness (k) is the rearward displacement of the occupant under occupant load in kN/m. Seat stiffness involves the amount of pocketing into the seatback and compliance of seat structures. Strength has been defined as (j), which is the inverse of strength with the angle change of the seatback frame divided by the occupant load in deg/kN. The stronger the seat frame in resisting seatback rotation, the smaller the j.
During the development of high retention seats, favorable occupant kinematics involved the H-pt dropping as the occupant moved rearward in the crash (Viano Citation2002). This required low stiffness (k) with an open or deformable components across the seatback, which are aspects of high retention seats (Viano Citation2002, Citation2003a, Citation2008). This kept the torso low on the seatback and the head low on the head restraint. Stiff components in the seatback promote ramping as the occupant displaces rearward.
New injury mechanisms in rear impacts
Seats designed in the 1970s-80s had a strength at the threshold for extension injury of the neck (Anderson Citation1961; Kihlberg Citation1969). The strength was consistent with the lower height of head restraints. In rear impacts with sufficient severity and occupant size, the seatback rotated rearward and the occupant ramped up the seatback with contact on the rear seating area. Once head restraints were raised and moved closer to the back of the head, seat strength was raised to improve occupant retention on the seat (Viano and White Citation2016). The strength of modern seats exceeds the tolerance of the thoracic and cervical spine in extension-tension, particularly in older occupants with spinal disorders. This has led to new injury mechanisms in rear impacts. The new mechanisms involve the seat remaining, relatively upright, typically <50 deg rearward of vertical (Viano and Parenteau Citation2008). One mechanism is hyper-extension around the upright seatback frame causing fracture-dislocation of the thoracic spine. Viano (Citation2011) identified 4 cases of the upper body hyper-extending rearward around the frame of the seatback. The fractures were very severe and often involved complete transection of the thoracic spine (T-spine). Figure A1 (see online supplement) (left) shows two radiology images of spinal transection with hyper-extension of the thoracic spine. The thoracic spine extended around the fulcrum from the frame of the seatback, which concentrated forces on the T-spine causing hyper-extension fracture-dislocation (Viano et al. Citation2021b).
Viano et al. (Citation2019) identified another mechanism of spinal fracture with the occupant in the seat and experiencing forces on the chest that extended the thoracic spine. Figure A1 (see online supplement) (right) shows four radiology images of extension fractures of the T-spine with the occupant remaining in the seat. As the occupant is accelerated forward, the shoulder, neck and head move rearward loading the upper seatback and head restraint. The forces on the thoracic spine are sufficiently high to try and extend the spine. In an occupant with DISH and other calcifications of the spine, the spine tries to extend and can fracture near the apex of the thoracic kyphosis. This mechanism of injury involves older occupants with spinal disorders and the spinal fractures can occur in low-to-moderate speed rear impacts because of the lower tolerances in the older occupants.
In this study, rear motion sequence criteria (MSC) were developed following the rationale of Adomeit and Heger (Citation1975) and Adomeit (Citation1977) MSC for favorable occupant kinematics in frontal crashes. Occupant kinematics in rear sled tests were studied to develop motion sequence criteria for favorable occupant kinematics in rear impacts with containment of the hip on the seat and no ramping up the seatback. The favorable kinematics contrast unfavorable kinematics with the occupant ramping up the seatback, the H-pt lifting up and a large torso angle rearward of vertical.
Methods
Hyge rear sled test with favorable occupant kinematics
Ford Motor Company has conducted 40.2 km/h (25 mph) rear sled tests with belted 50th Hybrid III dummies since the mid-1990s. The methods have been described with analysis of 26 tests with conventional and ABTS seats (Viano et al. (Citation2018). The seat was mounted in a sled buck with a seatback angle of 20 deg rearward of vertical. The head restraint was full up. The Hybrid III dummy was used because of the velocity of the test, its overall biofidelity (Prasad et al. Citation1997) and its certification for testing to FMVSS 202a.
A test with a 2016 driver seat was analyzed because it is representative of tests with favorable occupant kinematics. Details of the testing can be found in Viano et al. (Citation2018). The seat was fixed in a sled buck with B-pillar and floor anchors for the lap-shoulder belts. Multiple high-speed videos captured occupant kinematics and seat deformation from different perspectives. The cameras were mounted to a framework attached to the sled buck. The dummy was instrumented with transducers measuring head, neck, spine, chest and pelvic responses.
Rear sled test with unfavorable occupant kinematics
SAFE LLC conducts rear sled tests using a vehicle body buck mounted to a frame with wheels that is rolled into a wall covered by crushable paper honeycomb. The delta V of the test is the sum of the impact and rebound velocity of the buck. The shape of the honeycomb controls the deceleration pulse and duration. The sled pulse and rotation of the upper seatback on the inboard and outboard side were measured.
A test with a 2017 passenger seat was analyzed because it is representative of tests with unfavorable occupant kinematics. The 50th Hybrid III was not instrumented. It was ballasted to 86.8 kg (191 lb) by adding weight to the thorax. The dummy had the pedestrian pelvis, which required leather thigh covers on the flesh seam between the thigh and pelvis to prevent the seatbelt webbing from catching in the offset caused by sitting the dummy with a standing pelvis.
The seatback was reclined 25 deg rearward of vertical. The head restraint was in the lowest locked position. The sled impacted the barrier at 25.3 mph with a 17.2 g peak deceleration and a change in velocity (delta V) of 30.4 mph with rebound off the barrier. The maximum seatback rotation was 43.8 deg with 849.5 deg/s on the outboard and 41.4 deg with 811.9 deg/s on the inboard side of the upper seatback.
Energy transfer by the seat
The amount of energy that is transferred to the occupant as the seatback deforms in a rear impact depends on the rear delta V of the vehicle (ΔV or change in velocity) and the mass of the occupant (m) among other variables. The seat provides an interface with the occupant and transfers energy (E) can be approximated by E = ½*0.7*m(ΔV)2 using 70% of the occupant mass (m) and delta V (Severy 1968; Viano Citation2002).
Anti-ramping guide
A concept is described to control hip motion in rear impacts by guiding the movement rearward and downward. The anti-ramping guide is a shell contoured to the shape of the back of the pelvis. It is attached to the seat cushion frame by deformable brackets. In a rear impact, the brackets deform by forces from the lower torso acting on the cushion frame, not on the seatback frame above the recliner. This lowers forces on the seatback and improves favorable kinematics.
Motion sequence criteria
Sled tests
shows occupant kinematics in the two sled tests at 0, 60, 100 and 140 ms. (left) shows favorable occupant kinematics in a 40.2 km/h (25 mph) Hyge sled test with a 50th Hybrid III in test H41148. The energy transfer to the occupant required 3,421 J (2,523 ftlb) with the dummy weighing 80 kg (176 lb). At 100 ms, the torso angle was 31 deg. At 140 ms, the torso angle was 38 deg rearward of vertical and the H-pt was relatively low with respect to the initial height. The occupant was contained by the seat through the delta V. All of the biomechanical responses were below tolerances for the Hybrid III dummy (Viano et al. Citation2018).
Figure 3. (left) favorable occupant kinematics in H41148, (right) unfavorable kinematics in SAFE test.
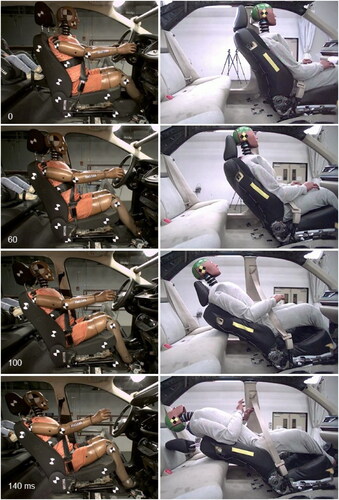
(right) shows unfavorable occupant kinematics in a 49.1 km/h (30.4 mph) sled test with a 50th Hybrid III weighing 86.8 kg (191 lb). The energy transfer to the occupant required 5,611 J (4,137 ftlb). At 100 ms, the torso angle was 52 deg as the occupant was up the seatback. At 140 ms, the torso angle was 65 deg and the H-pt was well above its initial position as the occupant continued to ramp up the seatback. The occupant was not contained by the seat. The dummy was not instrumented in the sled test.
Motion sequence criteria for favorable occupant kinematics in rear impacts
The sled testing shown in provides examples of favorable and unfavorable occupant kinematics. There is literature on sled and crash testing with kinematics that can be grouped in either category. That background provided a basis to propose motion sequence criteria (MSC) for favorable and unfavorable occupant kinematics in rear impacts.
(top) shows favorable occupant kinematics with a rearward and downward trajectory of the H-point as the seatback yielded rearward. The head, neck and torso were supported by the seatback and head restraint without ramping. The hip and shoulder move along an arc rearward and downward. Favorable kinematics prevent the mechanisms of injury with a portion of the occupant’s body losing support from the seatback and head restraint with ramping (Viano Citation2011). Ramping increases the position of the occupant up the seatback increasing the moment arm of the occupant loading about the recliners, which increases seat deformation.
Figure 4. (top) favorable motion sequence into the seatback and (bottom) unfavorable motion sequence with ramping up the seatback.
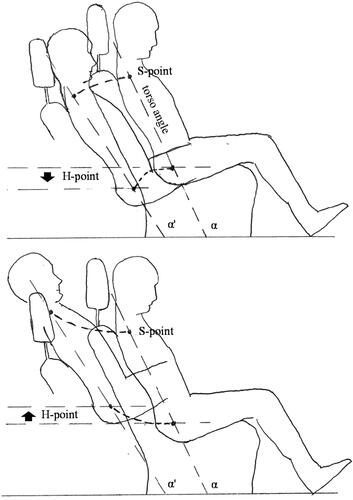
(bottom) shows unfavorable occupant kinematics with ramping up the seatback. This increases the height of the occupant on the seatback and head restraint, and it increases the tendency to lose support of the body by the seat. The S-pt can rise or fall depending on seatback rotation as the H-pt rises as it moves rearward. The ramping can cause the shoulders to load the bottom of the head restraint pushing it up against the stops at the highest position of adjustability.
Rear MSC
The rear MSC limit the angle of the torso (α) rearward of vertical to less than the critical angle (αc) for ramping up the seatback and direct H-pt displacement rearward and downward, so zHpt < zHpt0, where zHpt0 is the initial height of the H-pt. The lateral displacement is limited to less than the critical lateral displacement yT1c, where the head becomes unsupported by the head restraint or the chest by the seatback. The rear MSC contain the pelvis on the seat and provide uniform support of the torso, head and neck.
The rear MSC for favorable kinematics in rear impacts are based on the trajectory of the H-point (center of the hip) and torso angle (α), which is defined as the angle between the H-point and S-point, center of the shoulders. The proposed working criteria are: 1) the H-pt moves rearward 125-200 mm with a downward trajectory of 20-50 mm and 2) the torso angle rotates rearward from the initial design position with α = 105-120 deg to α’ = 140-155 deg at maximum restraint with the seatback reasonably upright. The criteria need refinement once test conditions and occupant sizes are specified. In any test, the point of maximum restraint or rearward movement of the occupant depends on the severity of the crash, size of the occupant and strength and design of the seat.
For rear impacts, control of lateral kinematics addresses the effects of oblique rear impacts and twist in the seatback. If there is sufficient lateral excursion of the occupant, the head can move off the head restraint and the chest and shoulders off the seatback, so MSC are needed to limit lateral excursions.
Energy transfer to the occupant
shows the energy transfer by the seat, which depends on the delta V and occupant mass among other variables. The four curves represent different occupant sizes, including the 5th female at 46.7 kg (102.7 lb), 50th male at 78.2 kg (172 lb) and 95th male at 102.5 kg (225.5 lb) Hybrid III and the 99th male in the US population at 150 kg (330 lb). The energy transfer increases with the square of the delta V. The 16.1 km/h (10 mph) sled test conducted by IIHS and required of FMVSS 202a involves 547 J (403 ftlb) energy transfer by the seat. The 40.2 km/h (25 mph) sled test with the 50th Hybrid III involved 3,421 J with favorable kinematics, as shown in the left image of and top image of . The 49.1 km/h (30.4 mph) sled test with the ballasted 50th Hybrid III involved 5,611 J with unfavorable kinematics, as shown in the right image of and bottom image of .
Anti-ramping guide
shows favorable kinematics that may be achieved over a wider range of situations by the design and development of an anti-ramping guide in the seat cushion frame that directs the hip rearward and downward. The guide provides a load path for the lower torso of the occupant to the seat cushion frame, avoiding load on the seatback above the recliners. The anti-ramping guide is composed of a shell contoured to the back and bottom of the pelvis and deformable brackets attaching the shell to the cushion frame. The brackets deform allowing the anti-ramping guide to displace along a rearward and downward trajectory. The guide requires an open seatback frame or deformable components in the lower seatback that allow the occupant to pocket and displace with the guide. The optimum trajectory for the anti-ramping guide depends on seat strength and design, and may vary from that shown in .
Discussion
Rear MSC provide performance guidelines to develop seats with favorable occupant kinematics in rear crashes. Sled testing at 40 km/h (25 mph) involves an energy transfer of 3,421 J with the 50th Hybrid III. A 56.3 km/h (35 mph) test involves 6,704 J, double the energy transfer, often with unfavorable kinematics. A target energy needs to be set. If it were set at 6,000 J, it would require significant changes in seats and would advance favorable performance to higher crash severities than current designs, assuming the biomechanical responses are below tolerances. However, there are practical limits in setting a target because the energy transfer is 12,858 J with a 150 kg (330 lb) occupant in a 56.3 km/h (35 mph) delta V rear crash.
The seats in use today yield rearward to gradually accelerate the occupant up through the delta V. Previous studies have found a low stiffness was one of the most important characteristics of a seat for occupant protection (Viano Citation2002, Citation2003a,Citationb). The stiffness defines the yielding behavior of the seat.
The higher energy test shown in (right) involved 5,611 J with unfavorable kinematics. The energy transfer requirement increases with crash severity and occupant mass. For example, a 99th US male in a 56.3 km/h (35 mph) delta V crash requires 12,858 J (9,481 ftlb) transfer by the seat. This amount of energy is beyond the capability of seats in use today and a test at that energy would result in unfavorable kinematics. Horsch (Citation1987) showed that stiffening structures to the extreme has safety downsides for occupants with lower tolerances, so balancing requirements is needed. Modern seats in use today provide reasonably favorable occupant kinematics in rear sled test up to 40.2 km/h (25 mph) with the 50th Hybrid III.
There are challenges to design seats with favorable kinematics up to 6,000 J, which would increase the range of crashes with favorable kinematics up to more than a 30 mph delta V with the 50th Hybrid III and just below that severity for the 95th Hybrid III. The seat would contain the 99th US male up to crashes less than 25 mph delta V.
The number of field accidents decreases as the delta V increases. Based on towaway crashes in NASS-CDS, 71.7% of rear impacts occur with less than 24.1 km/h (15 mph), 95.3% occur with less than 40.2 km/h (25 mph) and 99.4% occur with less than 56.3 km/h (35 mph) for belted occupants (Viano and Parenteau Citation2022). For occupants with severe to fatal injury, 19.9% occur with less than 24.1 km/h (15 mph), 25.1% occur with less than 40.2 km/h (25 mph) and 68.4% occur with less than 56.3 km/h (35 mph) for belted occupants. NASS-CDS indicates that 24.7% of severe injury and death occurs in crash with more than 72 km/h (45 mph) delta V.
Unfavorable kinematics in rear impact shown in the right images in and bottom image in involve a risk for injury to the cervical or thoracic spine (C-spine or T-spine) by the head or upper body hyper-extending rearward around the frame of the seatback causing fracture-dislocation of the cervical or thoracic spine (Viano Citation2011). With sufficiently severe crash forces and/or occupant weight, the seatback rotation and ramping may involve occupant impact in the rear interior with possible injury to the cervical or thoracic spine by a diving mechanism.
Seats with favorable MSC can still involve occupant ramping, particularly by induced ramping. Induced ramping is not caused by the occupant sliding up the seatback, but by other dynamics in rear impacts. It can be caused by over-ride of the rear of the struck vehicle, where the seat is pushed down away from the seated occupant held in space by inertia (Parenteau et al. Citation2020). There is another means to induce ramping if the rear of the seat cushion squats down during occupant loading. The squatting is related to the downward force on the rear attachments of the seat to the floor. With sufficient force, components in the seat deform downward. The speed of over-ride and squatting induce ramping with inertia holding the position of the hip suspended in space as the seat drops and the occupant shifts upward on the seatback.
The MSC for rear impact complement the control of biomechanical responses compared to tolerances to provide an overall evaluation of restraint performance. The occupant kinematics shown in the left images of and top image of involve favorable motion of the H-point and S-point. Sled tests show a tendency for ramping up the seatback with the H-pt moving horizontal or slightly higher in some modern seats at 40.2 km/h (25 mph) delta V. These tests also show essentially no restraint from the seatbelt with and without buckle or retractor pretensioning (Viano et al. Citation2012, Citation2021a; Viano Citation2022). If the sled tests were run at higher severity, more of the unfavorable kinematics emerge. The sled testing shows that occupant pocketing and seatback rotation occur simultaneously and the amount of either is a factor that depends on the seat design. New and existing seats should be evaluated with motion sequence criteria and biomechanical responses in test conditions with enough energy transfer to advance occupant safety in rear impacts.
There is no guarantee of safety performance if only motion sequence criteria or biomechanical responses are considered. This was a basis for the motion sequence criteria in front restraint development. Adomeit (Citation1977) and others were aware that submarining lowered head and chest accelerations in frontal tests. Submarining increased the distance that the occupant was decelerated reducing head and chest accelerations in FMVSS 208, which was an issue during the development of seatbelts for rear occupants in frontal crashes (Viano and Arepally Citation1990). During the research leading to the development of high retention seats, tests with occupants in 1980s-90s seats often had low biomechanical response with ramping into the rear seat (Viano Citation2002). This was a basis for the motion sequence criteria in rear impacts.
Supplemental Material
Download Zip (108.6 KB)Acknowledgments
The author has no direct or indirect financial interest in any seat design concept discussed in this study. The anti-ramping guide has been discussed in a US patent application 17693900 owned by a non-profit devoted to supporting research and education on automotive safety and occupant protection. The tests analyzed in this study were chosen from a large library. They were selected as representative of favorable and unfavorable kinematics. The difference between favorable and unfavorable kinematics depends on the weight of the occupant, strength of the seat and delta V among other factors. A seat can have favorable kinematics at one severity that becomes unfavorable at higher severities and favorable kinematics can involve high biomechanical responses if there is insufficient ride-down (Viano et al. Citation2021b). The author appreciates the use of the Ford and SAFE sled tests. The Ford Safety Center has a test laboratory in Dearborn, MI and SAFE LLC has a test laboratory in Goleta, CA.
References
- Adomeit D. 1977. Evaluation methods for the biomechanical quality of restraint systems during frontal impact. SAE 770936, 21st Stapp Car Crash Conference, SAE International, Warrendale, PA. doi:10.4271/770936
- Adomeit D. 1979. Seat design-A significant factor for safety belt effectiveness. Warrendale (PA): SAE International. SAE 791004.
- Adomeit D, Heger A. 1975. Motion Sequence criteria and design proposals for restraint devices in order to avoid unfavourable biomechanic conditions and submarining. SAE 751146. 19th Stapp Car Crash Conference, Society of Automotive Engineers, Warrendale, PA.
- Anderson JO. 1961. Dynamics of occupants in automotive accidents involving rear impacts. Warren (MI): Research Laboratories General Motors Corporation. Report #R-34–1295.
- Horsch JD. 1987. Evaluation of occupant protection from responses measured in laboratory tests. Warrendale (PA): Society of Automotive Engineers. SAE 870222.
- Kihlberg KK. 1969. Flexion-torsion neck injury in rear impact. Technical report. CAL report No. VJ-2721-R2.
- Parenteau C, Croteau J, Zolock J. 2020. The effect of crash severity and structural intrusion on ATD responses in rear-end crashes. SAE 2020-01-1224. Warrendale (PA): Society of Automotive Engineers,
- Prasad P, Kim AS, Weerappuli D, Roberts VL. 1997. Relationships between passenger-car seat back strength and occupant injury severity in rear-end collisions: field and laboratory studies. Warrendale (PA): Society of Automotive Engineers. SAE 973343.
- Viano DC. 2002. Role of the seat in rear crash safety. Warrendale (PA): Society of Automotive Engineers. SAE Book SAE R-317: p. 1–491.
- Viano DC (Ed.). 2003a.The debate between stiff and yielding seats: A new generation of yielding seats with high retention in rear crashes. Warrendale (PA): Society of Automotive Engineers. SAE Book PT-106.
- Viano DC. 2003b. Influence of seat properties on occupant dynamics in severe rear crashes. Traffic Inj Prev. 4(4):324–336. doi:10.1080/714040490
- Viano DC. 2008. Seat design principles to reduce neck injuries in rear impacts. Traffic Inj Prev. 9(6):552–560. doi:10.1080/15389580802381939
- Viano DC. 2011. Fracture-dislocation of the thoracic spine in extension with upright seats in severe rear crashes. Warrendale (PA): Society of Automotive Engineers. SAE 2011-01-0274.
- Viano DC. 2022. Analysis of the lack of restraint with and without belt pretensioning in 40.2 km/h rear impacts. Traffic Inj Prev. 1–7. doi:10.1080/15389588.2022.2121143
- Viano DC, Arepally S. 1990. Assessing the safety performance of occupant restraint systems. 34th Stapp Car Crash Conference, SAE 902328, Society of Automotive Engineers, Warrendale, PA, doi:10.4271/902328
- Viano DC, Burnett R, Miller GA, Parenteau CS. 2021a. Influence of retractor and anchor pretensioning on dummy responses in 40 km/h rear sled tests. Traffic Inj Prev. 22(5):396–400. doi:10.1080/15389588.2021.1910243
- Viano DC, Burnett R, White S, Stephens G, Parenteau CS. 2021b. Dual-recliner ABTS seats in severe rear sled tests with the 5th, 50th and 95th Hybrid III. Warrendale (PA): Society of Automotive Engineers. SAE 2021-01-0917.
- Viano DC, Parenteau CS, Burnett R. 2012. Influence of belt pretensioning on dummy responses in 40 km/h rear impact sled tests. Traffic Inj Prev. 13(1):65–71. doi:10.1080/15389588.2011.629558
- Viano DC, Parenteau CS, Burnett R, Prasad P. 2018. Occupant responses in conventional and ABTS seats in high-speed rear sled tests with a normally seated dummy. Traffic Inj Prev. 219(1):54–59. doi:10.1080/15389588.2017.1347782
- Viano DC, Parenteau CS. 2008. Serious injury in very-low and very-high speed rear impacts. Warrendale (PA): Society of Automotive Engineers. SAE 2008-01-1485.
- Viano DC, Parenteau CS, White S. 2019. Influence of DISH, ankylosis, spondylosis and osteophytes on serious-to-fatal fracture-dislocation of the spine and spinal cord injury in rear impacts. Warrendale (PA): Society of Automotive Engineers. SAE 2019-01-1028.
- Viano DC, White S. 2016. Seat Strength in Rear Body Block Tests. Traffic Inj Prev. 17(5):502–507. doi:10.1080/15389588.2015.1111513
- Viano DC, Parenteau CS. 2022. Injury by delta V in front, near-side, far-side and rear impacts: Analysis of 1994-2015 NASS-CDS. Warrendale (PA): Society of Automotive Engineers. SAE 2022-01-0835.