Abstract
Objective
Rear-impact electronic cases were reviewed for serious injury to rear-seated children and adults to identify mechanisms of injury and consider priorities, countermeasures and safety concepts.
Methods
1997-2015 NASS-CDS and 2017-2020 CISS electronic cases were analyzed for serious injury (MAIS 3+F) to rear-seated children 0-14 yo (years old) and adults 15+ yo in rear impacts.
Results
31 children and 43 adults were identified with serious injury. 86.0% of child injury was related to intrusion of rear structures with injury by direct force (55.3%), compression into front interior (27.5%) or acceleration into impact (3.2%). 14.0% of injury was not related to intrusion with front-seatback rotation (12.3%) or direct force (1.7%). Only 45.1 ± 17.6% of injured children were properly restrained. 68.0% of adult injury was related to intrusion of rear structures with injury by direct force (46.2%), compression into front interior (15.3%) and acceleration into impact (6.4%). 21.6% of injury was not related to intrusion with front seatback rotation (2.5%) or direct force (19.1%). 10.4% was from ejection and ground impact without seatbelt use. Only 17.8 ± 7.5% of injured adults were restrained. Priorities, countermeasure and concepts were considered to improve occupant protection in rear impacts: 1) new rear impact test with the IIHS high-hood barrier offset to the rear at 55 mph to reduce rear-seat intrusion, 2) stepped-up campaigns for proper child seat selection and use, particularly for infants and toddlers <2 yo and adult use of seatbelts in rear seats, 3) adoption of FMVSS 201L to reduce head injury risks of children impacting the rear interior below the beltline, 4) improved front seats by shifting restraining forces from the seatback to the cushion frame with an anti-ramping guide that retains the pelvis on the seat and reduces seatback rotation, 5) changes in front seatbelts to provide early restraint of occupant movement by a rear-impact pretensioner that moves the lap-belt anchors and gives a favorable angle for early lap-belt restraint or a rear wrap-around lap belt so the occupant moves into the belt in a rear impact, 6) implementation of kinematic controls on front-occupant movement that reduces ramping in severe rear impacts, 7) guarding the area around rear-seated child with an after-market ring structure that maintains space around the child and blocks rearward rotation of the front seatback and 8)-25) recommendations.
Conclusions
The primary mechanism for injury to rear-seated children and adults is intrusion, often without appropriate occupant restraint. There are priorities to improve protection by reducing intrusion, guarding the area around children, shifting front-occupant loads to the cushion frame and improving occupant restraints.
Introduction
Managing risk sensibly
In the 1980s, the federal government based policies on managing health risks sensibly (Morrall Citation1986). NHTSA developed HARM as a metric to manage crash injury risks and prioritized safety improvements in vehicles. Malliaris et al. (Citation1982) combined the incidence of injury with the cost for different injury severities to estimate the HARM caused by motor-vehicle crashes. They identified the sources of the injury by body region and contact source from the field accident data in the 1979-80 National Accident Sampling System (NASS) and listed priorities for safety improvements. Table A1 in the Appendix re-orders columns from Table 6 in Malliaris et al (Citation1982) to show priorities for HARM reduction to rear-seat passengers. Overall, 6.3% of HARM occurred to rear-seat passengers with 68.3% to drivers and 25.4% for right-front passengers. For rear occupants, 4.0% of HARM identified a contact source on the interior of the vehicle. 1.3% of HARM ranked in the top 25 sources of HARM for all occupants. The leading sources were the front seatback (0.44%, rank 22), window frames (0.29%, rank 21) and side interior (0.19%, rank 3) for rear-seat occupants. 50.5% of rear-seat HARM was to the head-face, 38.9% to the abdomen and 6.9% to the chest.
Table 1. Mechanisms of injury in rear-seated children and adults in rear impacts (modified from Parenteau and Viano Citation2022c).
Malliaris et al. (Citation1985) expanded the original analysis of HARM using 1980-83 NASS and other field data. They provided in-depth analysis of field accident injuries in the US and listed priorities for safety improvements. They further identified sources of the injury HARM by body region. Table A2 in the Appendix re-orders columns from Table 10 in Malliaris et al. (Citation1985) to show priorities for HARM reduction to other-seat passengers than the driver and right-front passenger. Overall, 12.0% of HARM occurred to other passengers with 9.0% prioritized by source and body region. The leading sources were the front seatback (2.91%) and lower side interior (2.88%). 39.3% of HARM was to the head, 26.6% to the abdomen and 20.0% to the chest-back of other passengers.
Table 2. Most seriously injured body regions for rear-seated children and adults in rear impacts (modified from Parenteau and Viano Citation2022c).
In the 1980s, the government and industry setup safety development based on HARM and the Haddon matrix, which provided frameworks for injury prevention (Haddon et al. Citation1964; Haddon Citation1972; Williams Citation1999). Automotive manufacturers started safety initiatives. For example, GM started VSIP (vehicle safety improvement program) to systematically address safety improvements in a sensible manner based on HARM reductions (Wilson Citation1983). One initiative involved improving fuel systems for vehicles in severe rear impacts to reduce the risk of fire. The FMVSS 301 barrier impact was increased to 35 mph from 30 mph in the standard by several manufacturers. The increased speed represented a 36% increase in kinetic energy of the crash.
Managing risk sensibly was the basis for industry support for federal programs for injury prevention (NAS Citation1985; Viano Citation1989a,Citationb, Citation1990). NHTSA continued to provide safety priorities based on the incidence and severity of injury to motor-vehicle occupants and other accident conditions as part of cost-effective regulation. Efforts to protect rear-seat occupants should be based on how they are injured in field accidents, including the sources of HARM.
2003 field data
Parenteau and Viano (Citation2003a,Citationb) studied injuries to rear-seat occupants using field accidents collected by NHTSA. The 1991-1999 NASS-CDS provided information on crashes, occupants and injuries. In Part I, Parenteau and Viano (Citation2003a) studied adults and teenagers in rear seats. The field injury data and the literature identified priorities for adults and teenagers in rear seats, including: 1) load-limiting belts with cinching and good restraint geometry, 2) EA material for the seatback, side interior and B-pillar, 3) reduced contact velocity with seatback, side interior and B-pillar, 4) improved containment with inflatable side curtains and laminated side glass and 5) technologies, such as inflatable belts. In Part II, Parenteau and Viano (Citation2003b) studied children 4-12 years old and toddlers-infants 0-3 years old in rear seats. The data was separated because of the use of infant, child and booster seats involved different injury mechanisms and tolerances.
Table A3 in the Appendix shows children 4-12 yo (years old) seriously injured in the rear seats (Parenteau and Viano Citation2003b). The children were most often injured in front impacts (48%), followed by side impacts (23%) and rollovers (21%). Only 5.5% of rear-seated children were seriously injured in rear impacts. The risk of serious injury to rear-seated children was highest in rollovers (3.7%) followed by front (1.4%) and side (1.2%) impacts. The risk for rear-seated children in rear impacts was 0.58%, the lowest among crash types. The priorities suggested by the data were to improve the proper use of belt restraints and child seats used in rear seats and to improve the energy absorption characteristics of the front and side interior around rear children. Injuries to rear-seated children in rear impacts did not emerge in the list of high-priority HARM to children.
Table 3. Crash characteristics with serious injury in rear-seated children and adults in rear impacts (based on data from Parenteau and Viano Citation2022c).
The distribution of serious injuries was assessed for belted and unbelted child occupants 4-12 in frontal impacts. Head and upper extremities were the body regions with most frequent serious injuries (AIS 3+). The seatback, head restraint, B-pillar and interior surfaces were common injury sources. Various mitigating actions were proposed to reduce injuries. Foremost was the application of FMVSS 201 to the seatback and interior surfaces below the beltline, which would address the contact areas engaged by belted and unbelted children. Other areas included using EA (energy absorbing) material on the back of front seats, B-pillar and interior surfaces below the beltline, improved cinching the occupant to the child seat, reducing contact velocity with side interior and B-pillar and using EA material for side structures above the beltline, such as side curtains.
Three-quarters (74%) of infants and children 0-3 years old exposed to towaway crashes were in rear seats as compared to 22% in the front passenger seat and 4% in other positions. The risk for serious injury was 1.1% in rear seats and 1.2% in front seats. The risk was statistically similar (p < 0.10). Unbelted 0-3 year old children had 5.8 times greater risk of serious injury than those restrained in a child seat. There were too few cases of vehicle crashes with airbags available to assess relative risk differences between front and rear seats for 0-3 year old children. Based on the most frequent injuries by restraint use and crash type, a series of safety areas were identified to improve protection for young children and others in the rear seats.
2008 field data
Viano and Parenteau (Citation2008a) studied the fatality risk of 0-7 yo children in rear seats. Tables A4 and A5 show that rear-seated children had the highest risk of fatality in rollovers (1.26%) followed by side impacts (0.39%). The risk varied by the principal direction of force (PDOF) of the collision. The paper showed that a left rear-seated child had the highest risk in 7 o’clock impacts at 2.74% followed by 4 o’clock impacts at 2.48%. The risk was 0.44% in 6 o’clock impacts. Overall, children 0-7 were safest in the center rear seat (0.27%) followed by the left rear (0.29%) and right rear (0.32%). There was a higher risk in the right-rear seat because of intersection crashes with the vehicle turning left in front of oncoming traffic.
Table 4. Rear impact tests and energy transfer.
Viano and Parenteau (Citation2008b) analyzed all cases of serious injury to children 0-7 yo in rear seats in rear impacts to identify priorities for safety improvements. Individual rear-impact cases were reviewed to better understand injury mechanisms of children in rear seats. The cases were obtained from the 1997-2005 NASS-CDS electronic database. Rear impact cases were chosen based on serious-to-fatal injury (MAIS 3 + F). There were 19 injured children involved in 16 rear impacts (see Table A4). Thirteen injuries (13 or 68.4%) were associated with ≥ 30.5 cm (≥12”) intrusion of their seating area, which pushed the child forward. Since intrusion occurred early during the collision forces and vehicle acceleration, it occurred before much rearward rotation of the front seats. This meant the child was pushed forward into an upright front seat. There was only one child (5.3%) who was injured by rearward rotation of the front seatback.
The study concluded that stiffening front seats was not a reasonable approach to improving safety of rear-seated children. Limiting head excursion and shielding the upper body from forces on the child seemed more appropriate. The idea of a ring structure integrated with the child seat was discussed for the most severe crashes where the rear-seated child was injured by intrusion pushing them forward into the front seatback, B-pillar or front component or directly injuring them. Viano and Parenteau (Citation2008b) included a discussion of protecting the space around the child with a ring structure that was compatible with child seats to improve the safety of rear-seated children in severe rear crashes. Other studies of field accidents explored the role of the principal direction of force (PDOF) and seating position on injury risks for rear and front occupants (Viano and Parenteau Citation2008c).
2016 field data
At NHTSA’s request, Viano and Parenteau (Citation2016a) updated the earlier analysis of seriously injured rear-seated children in rear impacts and provided the information to NHTSA on 6-3-16. The 8-year update identified 24 children (5 plus the original 19) who were seriously injured in 21 rear impacts (5 plus the original 16), see Table A4. The new electronic cases were added to the original dataset. Seventy-one percent (71%, 17 of 24) of the electronic cases in the 1997-2013 NASS-CDS involved intrusion pushing the child forward into the back of the front seat, the B-pillar or other front structures. The conclusions of the original analysis remain unchanged and were strengthened by the additional cases. The five new cases were very severe rear impacts with delta V of 40.2-59.7 km/h (25-37 mph). They involved substantial intrusion of the rear seating area.
The safety improvement of adding a front shield around the child seats was restated. The approach would provide room protecting the child if intrusion pushed them forward into the front seatback or the front seatback rotated rearward preventing the front occupant or seatback contacting the child. The authors cautioned that stiffening front seats, such as with ABTS seats, may increase injury risks for rear-seated children because of the more frequent frontal crashes where children need a yielding front seatback even when belted in the rear seat.
Public attention to rear-seated children in rear impacts
On 3-9-16, Mr. Clarence M Ditlow from the Center for Auto Safety petitioned NHTSA to address the protection of children seated in the second row in rear crashes (Ditlow, Citation2016). The petition urged NHTSA to “take action to protect children riding in the rear seats of vehicles from the risk of being killed or severely injured when struck by a ‘collapsing’ front seatback in a rear-end crash.”
On 5-25-16, Senators EJ Markey and R Blumenthal sent letters of inquiry to automotive manufacturers on the same issue (Markey and Blumenthal Citation2016). The petition and inquiry refer to industry “efforts to protect passengers against the threat of front seatback failures.” It focused on second-row occupants, particularly children in rear impacts.
On 6-23-16, the Alliance of Automobile Manufacturers responded to the Senator’s letter (AAM Citation2016). They raised concerns for raising seatback strength beyond current standards. They noted that automakers design and engineer their vehicles to achieve the best overall occupant protection.
NHTSA’s reply
On 1-13-17, Administrator Rosekind from NHTSA replied to the Senator’s letter and petition (Rosekind Citation2017). He said that NHTSA is obligated to consider the potential benefits, as well as the drawbacks. For example, a standard that prescribes a stronger seatback structure to prevent large seatback deformation in high-speed rear impacts, without consideration of how this will contribute to the potential for whiplash injury in frequent low-speed rear crashes, may not optimize overall injury protection. Through multiple projects, including crash test dummy testing and research, NHTSA is working toward reducing the occurrence of rear-impact crashes, as well as mitigating their severity. NHTSA also continues to work toward reducing the occurrence of rear crashes as well as mitigating their severity. The Agency’s encouragement of industry to voluntarily adopt Automatic Emergency Braking (AEB) technology as standard equipment by 2022 will move us closer to that goal.
Neither NHTSA nor the Senators identified the underlying mechanisms that were the basis for child injury in rear seats in rear impacts. Both focused on a “solution” of further strengthening front seats or AEB without a clear description of the mechanisms of injury to children. The main point is what are the sensible means of improving safety that address the underlying mechanisms of injury to children in rear seats in rear impacts based on HARM. The Senators focused on front seatback strength. NHTSA replied that more research was being conducted and that automatic braking technology reduced rear impacts. They did not define what proportion of child injury was related to their “solutions” or how much benefit-disbenefit may occur with their “solutions.”
NHTSA and others raised the problem of tradeoffs. However, one tradeoff is not whiplash in front occupants for serious injury to rear children. The tradeoff is for serious injury to front-seat occupants for serious injury to rear-seat children. Viano and Parenteau (Citation2008d) studied serious injury in low-speed rear impacts and found up to 20% occurred in crashes with less than 24.1 km/h (15 mph) delta V. The injuries involved older occupant, many with spinal disorders that were injured by forces on their chest extending the thoracic spine in an upright seat (Viano Citation2011; Viano et al. Citation2019a). The tradeoff involved different risks for serious injury in front and rear seats.
2020 field data
Parenteau and Viano (Citation2020) updated earlier analyses of rear-seated children 0-7 years old in rear crashes using 1997-2015 NASS-CDS with 1994+ model year vehicles. Table A6 shows the distribution and risk of serious-to-fatally (MAIS 3 + F) injury to rear-seated children in the rear. The greatest number of rear-seated children were injured in frontal (39.6%) and side (20.5%) impacts. The highest risk for serious injury was in rollovers (1.72% ± 0.36%) followed by rear crashes (0.75% ± 0.56%). Individual rear and frontal impact cases were reviewed to better understand injury mechanisms of children in rear seats.
There were 15 injured children involved in 14 rear impacts with electronic cases in NASS-CDS (Tables A4 and A6). Seven of the cases were new and not previously included in the 2008 study. The analysis of the 15 children indicated that half were associated with significant intrusion >30.5 cm (>12”) into their seating area, pushing the child forward. The case review indicated that the front seat remained in its pre-crash position or was pushed forward in 7 out of 11 cases. The front seatback rotated rearward in 4 of 11 cases. Two involved a severe-to-fatal injury (AIS 4+) where the child was pushed forward due to significant intrusion.
Parenteau et al. (Citation2021a) determined occupant demographics and injury by body region and crash severity in rear crashes. Rear crashes accounted for 8.7% of exposed and 5.4% of serious-to-fatally injured rear-seat occupants. On average, rear-seat occupants were 14.3 ± 1.5 years old, weighed 44.7 ± 2.6 kg and were 130.3 ± 4.1 cm tall. They concluded that the height and weight of a 10 year old and 5th Hybrid III represented the average rear-seat occupant involved in rear crashes based on NASS-CDS. Crash tests with 30-37 km/h a delta V represented the typical collision causing serious-to-fatal injury. Parenteau and Viano (Citation2021) also analyzed interior impacts in rear crashes.
2022 FARS
Parenteau et al. (Citation2022) analyzed 1990-2019 FARS for 2nd row children 0-15 years old in front, side and rear impacts to study fatality trends by model year (MY) and calendar year (CY) with 1980-2020 MY vehicles. 1990-2015 POLK determined exposure of registered vehicles to estimate fatality rates. The data was analyzed by MY of the vehicle and CY of the crash. The results showed that nearly half of fatally injured 2nd row children in rear-crashes in the 2011-19 CY were in older vehicles with MY ≥ 10 years old. From 2010-19, 59% ± 7% of children fatally injured in rear impacts were in vehicles 10 or more years old.
Senate inquiry
On 11-15-22, Senators Markey et al. (Citation2022) sent a letter to Ann Carlson, Administrator of NHTSA, about the agencies progress in implementing the traffic safety initiatives laid out on the Infrastructure Investment and Jobs Act’s (IIJA). The act requires NHTSA action on the FMVSS 207 seatback standard, child safety and eight other initiatives including the new car assessment (NCAP) program of crash tests. It asked for a written update by 12-15-22.
Objective
This study further analyzed field data on serious injury (MAIS3 + F) to rear-seated children (0-14 yo, year old) and adults (15+ yo) in rear impacts. All individual electronic cases in NASS-CDS and CISS were evaluated for restraint use, body region injured, mechanism of injury and crash characteristics. The analysis provides a basis for proposing a series of countermeasures and “thought-starters” to improve occupant protection in rear seats in rear impacts based on managing risks sensibly.
Methods
Analysis of 1997-2015 NASS-CDS and 2017-2020 CISS field data
For complete details on the data analysis methods defining rear-seated occupants in rear impacts refer to Parenteau and Viano (Citation2022c) and Viano and Parenteau (Citation2008a,Citationb, Citation2016a). The data on children 0-14 yo and adults 15+ yo was analyzed separately. Only light vehicles with 1994+ MY were included. Rear impacts were defined as towaway vehicles with rear impact location (GAD1=’B’). Rear-seated occupants were identified by SEATPOS 21, 22, 23 for the 2nd row. Injury severity of each occupant was assessed using the Maximum Abbreviated Injury Scale score (MAIS) and the “TREATMNT” and “INJSEV” variable. Fatality was defined as TREATMNT = 1 since it means that the occupant was fatally injured and not transported to the hospital or the police injury severity INJSEV = 4, which represents a fatality according to police ratings. Exposed occupants were defined as MAIS 0-6 or a fatality using the notation MAIS 0 + F. Serious-to-fatally injured occupants were MAIS 3-6 or fatality with notation MAIS 3 + F. Weighted data provides estimates for the exposure and injury to rear-seated occupants.
Individual NASS-CDS and CISS cases were downloaded from NHTSA (www.nhtsa.gov) involving serious injury (MAIS 3 + F) to rear-seated children and adults in rear crashes. The crash circumstances, occupant age, height and weight, injury type, severity and injury source were summarized, and photographs of the vehicle and interior were reviewed. Intrusion at the occupant location (second row left, middle or right) and front seatback rotation was identified using the accident investigator’s coding (seatback prior and post inclination) and review of photographs.
Priorities, countermeasures and “thought-starter” concepts
Based on the mechanisms of injury to rear-seated children and adults in rear impacts, priorities, countermeasure and “thought-starter” concepts are proposed to improve the protection of occupants in rear impacts. The priorities, countermeasures and concepts include crash testing, public education campaigns, laws and regulations and new safety concepts to improve protection of children and adults in rear impacts with existing and future vehicles.
Results
2022 field data and electronic case reviews
summarizes the mechanisms of injury and restraint use with seriously injured (MAIS 3 + F), rear-seated children and adults in rear impacts from Parenteau and Viano (Citation2022a). The electronic cases identified 31 children (0-14 yo) and 43 adults (15+) seriously injured in rear impacts using 1994-2015 NASS-CDS and 2017-2020 CISS field data for 1994+ model year (MY) vehicles. The child injury cases are summarized in in the Appendix and the adult injury cases in Table B2. The mechanisms of injury were determined by review of the photographs of the vehicle and interior and analysis of crash characteristics. Restraint use was based on the field investigator’s determination of seatbelt use or misuse and child seat use or misuse in the crash. Overall, the risk for serious injury for 2nd row occupants was 0.93% ± 0.36% in rear crashes. It was 0.76% ± 0.39% for children and 1.22% ± 0.40% for adults.
On mechanisms of injury, shows that 86.0% of children (0-14 yo) and 67.9% of adult (15+ yo) serious injury was related to intrusion based on weighted counts. In most cases, the direct force of intrusion caused serious injury in 55.3% of children and 15.3% of adults. A tractor-trailer impacted the rear of the vehicle in 30.2 (1.1%) weighted count (2 cases) with children and 218 (8.0%) weighted count (3 cases) with adults. Tractor-trailer impacts caused extreme intrusion (Parenteau and Viano Citation2022b). The intrusion was sufficient to compress the occupant against the front seat or accelerate the occupant with impact on frontal components. Intrusion was not a factor in 14.0% of children and 21.6% of adult serious injury. The front seat or occupant moved rearward injuring 12.3% of children and 2.5% of adults.
On restraint use, shows that only 17.8 ± 7.5% of adults were restrained by seatbelts when seriously injured. There were 9 cases weighted to 473 count that were ejected from the vehicle. This was 17.4% of the cases with adults and resulted in serious injury in 10.4% of the cases by ground contact. For children, restraint use was only 45.1 ± 17.6% and there were several cases of improper child seat or misuse of restraint in children <2 yo. While restraint use was at 81.8 ± 4.6% in adults and 82.7 ± 3.9% in children exposed to towaway crashes, the restraint use was significantly lower in serious-injury crashes.
shows the body regions seriously injured in rear-seated occupants in rear impacts. On average, there were 3.18 serious injuries (AIS 3+) when a child was seriously injured (MAIS 3 + F). The head (73.0%) was the most frequently injured body region in children. The thorax and abdomen (51.0%) were the most frequently injured body region in adults, followed by the head (26.5%).
shows the number of impacts in the collision sequence with serious injury to rear-seated occupants in rear impacts. For children (0-14 yo), 69.1% of the crashes involved two or more impacts in the accident based on the weighted data. For adults, 46.1% involved two or more impacts. The occupancy of the vehicle ranged up to 6 in the rear-impact collisions. For children, the majority (58.0%) involved three occupants in the vehicle with 28.6% having 4 or more occupants. There was one case with a sole rear child in a stopped vehicle, where the driver had exited the vehicle before it was struck in the rear. For adults, there were three (36.0%) or four (34.1%) occupants in the vehicle with 53.7% having 4 or more occupants.
Priorities, countermeasures and concepts
Crashworthiness test to reduce intrusion
shows the cumulative risk for severe injury (MAIS 4 + F) of adults (15+ yo) in rear impacts from Viano and Parenteau (Citation2022a). The analysis involved 22 years of data in the 1994-2015 NASS-CDS. The cumulative severe injury is separated for restrained and unrestrained occupants. The data is provided in Table A7 in the Appendix. The 50th percentile crash severity is about 40 km/h (25 mph) for restrained and about 24 km/h (15 mph) for unrestrained occupants. For restrained occupants, 24.7% of severe injury occurred in collisions with delta V greater than 72 km/h (45 mph). Equally important was that 19.9% of severely injured occupants were in collisions with < 24 km/h (15 mph). The occupants injured in low-speed rear impacts tend to be older and with spinal degeneration, so the management of intrusion needs to consider controlling force with sufficient space for older occupants (Viano and Parenteau Citation2008d; Viano Citation2011; Viano et al. Citation2019a; Parenteau et al. Citation2020a,Citationb).
Figure 1. Cumulative % of severe injury (MAIS 4 + F) in rear impacts with different rear-impact barrier crashes (based on data in Viano and Parenteau Citation2022a).
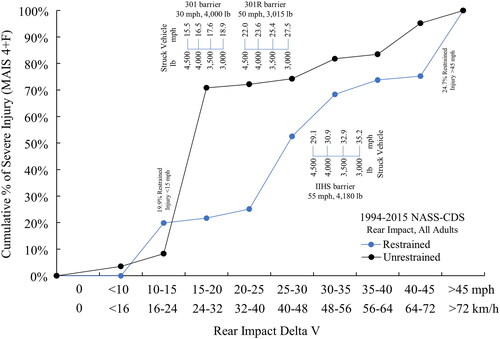
shows rear-impact crash tests and energy transfer with the delta V of the struck vehicle calculated by conservation of momentum based on the mass of the barrier striking and four different masses 1,364, 1,591, 1,818 and 2,045 kg (3,000, 3,500, 4,000 and 4,500 lb) of the struck-vehicle. The calculations included a 0.10 coefficient of restitution (Viano et al. Citation2008).
The original FMVSS 301 involved a 48 km/h (30 mph) rear impact by an 1,818 kg (4,000 lb) barrier. This results in 25.0-30.3 km/h (15.5-18.9 mph) delta V. The revision to the standard increased the energy of the collision. FMVSS 301 R involves an 80.5 km/h (50 mph) impact by a 1,368 kg (3,015 lb) deformable barrier offset to the rear of the struck vehicle. The delta V is 35.5-44.3 km/h (22.0-27.5 mph) for the range of vehicle weights. There was a significant improvement in safety with the introduction of FMVSS 301 R (Viano and Parenteau Citation2016b).
shows the effect of an NCAP or consumer information test using the IIHS high-hooded deformable barrier impacting offset to the rear of a vehicle at 88.5 km/h (55 mph). The IIHS barrier weighs 1,900 kg (4,180 lb) and would result in a delta V of 46.9-56.7 km/h (29.1-35.2 mph) in the struck vehicle. The test would increase the need for management of collision forces by rear structures at and above the frame rails and suspension of the vehicle. It would reduce intrusion of the rear seatback. The current FMVSS 301 R barrier has the profile of a passenger vehicle. It involves the side-impact barrier in FMVSS 214, which is lowered 50 mm (2”) for rear-impact tests of a vehicle. A large fraction of striking vehicles is SUVs, trucks and tractor-trailers with high front profiles that can over-ride rear structures and deform rear seatbacks.
The NCAP or consumer information test with the IIHS barrier and the current FMVSS 301 R should start measuring vehicle dynamics in the struck vehicle and determining rear-seat intrusion that should become a routine part of the test report. No dynamic data on cg (center of gravity) acceleration, yaw or pitch is collected on the struck vehicle in FMVSS 301 R, no post-test displacement of the rear seat is provided and no CDR readout of the crash is collected from the vehicle black box. This is a tremendous loss of information.
Tractor-trailer impacts
Viano and Parenteau (Citation2022b) determined the type of striking vehicle or object associated with serious injury to at least one occupant in the vehicle struck in the rear. The highest risk for serious injury was an impact by a tractor-trailer at 2.71%. The average risk was 0.33%, so the relative risk was 8.2 times for impacts by tractor-trailer. The study concluded that collision mitigation was necessary by automatic emergency braking of tractor-trailers, since the amount of energy in the collision was not manageable by deformation of the struck vehicle. shows a large fraction of rear-seat occupant injury related to intrusion. Tractor-trailer impacts were the cause in two cases (1.1%) with children and three cases (8.0%) with adults. In addition to automatic braking on tractor-trails and large vehicles, the technology should be standard equipment on all light vehicles.
Compatibility
The case review found serious injury by intrusion of the rear seat. In many cases, the striking vehicle over-rode rear structures loading above the frame rails, under-rode rear structures with floor deformation or obliquely loaded the rear at an angle. While engagement of the rails occurred in many cases, the structures above the rails deformed intruding the rear seatback forward. Analysis of vehicle-to-vehicle collisions shows that when intrusion occurs, it attains the speed of the front of the colliding vehicle while the struck vehicle center of gravity (cg) has a gradual increase in velocity up through the delta V (Parenteau et al. Citation2020a,Citationb). The intrusion occurs early without much movement of the vehicle or occupants. It involves direct, high-speed loading of the occupant adjacent to the intrusion and vehicle deformation. The compatibility of front and rear structures remains important to minimize rear-seat intrusion, because rear impacts often involve offset, over-ride or under-ride and PDOF that is not colinear.
Public education, proper use of seatbelts and child seats, warnings
Rear-seated adults used seatbelts only 17.8 ± 7.5% of the time when seriously injured (MAIS 3 + F) in rear impacts. The use rate was 81.8 ± 4.6% for adults exposed to tow-away crashes (). The very low use rate in serious injury crashes needs to be addressed. A multi-pronged approach should include public education about the importance of restraint use in all seating positions, warning alerts when rear-seated occupants are not buckled, improved access and convenience of belts in rear seats and state laws requiring adults to use seatbelts in rear seats.
Only 45.1 ± 17.6% of rear-seated children were restrained when seriously injured (MAIS 3 + F) in rear impacts. The use rate was 82.7 ± 3.9% for children exposed to tow-away crashes (). Seven out 14 children up to 2 yo (17.2% of the weighted sample) were either in the wrong child safety seat or improperly restrained. Proper restraint use is important in infants and toddlers. Public education needs to reinforce the correct choice of child seat and the proper installation of the seat in the vehicle and child in the seat. The campaigns should emphasize areas of low socioeconomic status (SES). Children 3-5 yo were in the proper child seat and were restrained. Older children were frequently unrestrained or the investigator could not determine restraint use. Improving proper restraint use by children is important. Public education, state laws and other efforts need to address the improper choice of child seat, improper or nonuse of restraints for young children in rear seats.
Older vehicles
shows the cumulative age of vehicles with serious injury (MAIS 3 + F) to rear-seated children and adults in rear impacts. It also includes the cumulative age of vehicles in the 2020 FARS. FARS shows that more than half of vehicles involved in fatal crashes are over 10 years old. In 2010, NASS-CDS and CISS stopped in-depth investigation of vehicles over 10 years old (NHTSA Citation2010a). The child injuries in rear impacts follows the age of vehicles in FARS until the vehicles are 8 years old and then it jumps to over 70% because there are only a few vehicles over 10 yo in the sample. NASS-CDS and CISS distort the understanding of field accidents by not investigating a sample of all vehicles in use on US roads. The adult rear crashes follow a similar trend in vehicle age. Older vehicles are an important part of crash injuries in the US. The older vehicles are often driven by those with lower SES. These occupants have lower restraint use and higher misuse of child safety seats.
Figure 2. Cumulative % of vehicles with serious injury (MAIS 3 + F) to rear-seated occupants and vehicle age in the 2020 FARS (MAIS 3 + F data based on Parenteau and Viano Citation2022c).
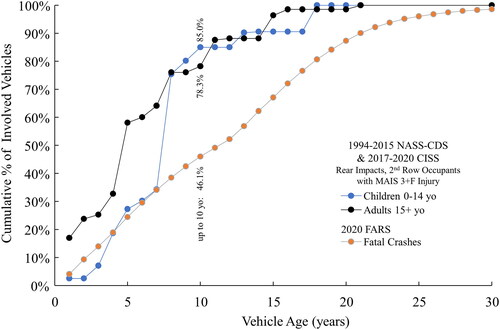
NASS-CDS stopped coding information on vehicles more than 10 years old in 2010. The current CISS includes only newer vehicles, excluding vehicles over 10 years old from in-depth investigation. This deprives researchers of an important stratum of traffic injuries. While FARS maintains information on all vehicle years, there is no in-depth analysis of the crash or injuries. NHTSA should rescind their decision and should again have investigators analyze all vehicle ages in CISS. Parenteau et al. (Citation2022) showed the importance of older vehicles in an analysis of child deaths in 1990-2019 FARS. The fatal crash data showed that 59 ± 7% of child deaths from 2010-2019 were in vehicles over 10 years old. Only 21 ± 9% of child deaths were in vehicles less 5 years old.
A curve was fit to the percent involvement (I) in fatal crashes by vehicle age from 1-20 yo (years old) in the 2020 FARS (). There was a linear increase in vehicle age involved in fatal crashes with I = 4.48 ± 1.50%/yr, R2 = 0.9992 up to about 20 yo vehicles and then a tail-off with older vehicles. Less than half of fatal crashes involve vehicles up to 10 yo, similar to Parenteau et al. (Citation2022).
Interior head impacts
FMVSS 201 was modified in 1995 with a phase-in of new requirements in FMVSS 201 U from 1999-2003 (NHTSA Citation1995a,Citationb,Citationc). A “free motion” headform impact at 24 km/h (15 mph) was required on the upper interior above the beltline for front, side and rear interior locations. The Head Injury Criterion HIC(d) was defined using the triaxial acceleration of the head limiting it to 1000 for any 36 ms duration.
shows that 73.0% of serious injury to children involves a head impact. The sources are the front seatback, side interior and B-pillar area below the beltline. The addition of a FMVSS 201 L for areas below the beltline in the rear interior would lower injury risks for children. The test should involve a 6 yo Hybrid III headform with scaled requirements following the FMVSS 201 U procedures with specified impact locations and angles on the rear interior below the beltline. This recommendation was first made in 2003 (Parenteau and Viano Citation2003b).
Ejection
FMVSS 226 addresses ejection mitigation to reduce partial and complete ejection of vehicle occupants through side windows in crashes, particularly rollover crashes. The final rule phased-in requirements starting in September 1, 2013. The regulation involves a body-form impact. It limits the distance beyond the plane of a window for impacts at four locations and two time intervals following deployment of a side curtain.
The standard is particularly important for unbelted, rear-seat adults in rear impacts shown in . Only 17.8 ± 7.5% of rear-seated adults were restrained in the sample of serious injury (MAIS 3 + F). 17.4% of the weighted adult count were ejected from the vehicle with 10.4% experiencing injury on ground contact. It would be useful to analyze the 74 rear impacts with 31 children and 43 adults to determine if the ejection mitigation technology would have deployed and been effective in the accidents. If not, the triggering logic should include rear impacts above a threshold severity. With 73.0% head injuries in children and 26.5% head injuries in adults, the deployment of side curtains needs to occur in severe rear impacts; otherwise, the ejection mitigation technology is not deployed to contain rear occupants in rear impacts.
Front seat strength
Rotation of the front seatback rearward was the source of serious injury (MAIS 3 + F) in 12.3% of children and 2.5% of adults in 2nd row seats in rear impacts. There has been a steady increase in the strength of seats with the 2010 revision to FMVSS 202 that increased the height and reduced the gap behind the head of front occupants (NHTSA Citation2010b; Viano and White Citation2016). shows the peak moment and standard deviation of single recliner, dual recliner and ABTS seats in body block tests by decade of the vehicle MY. The data updates the chart in Viano and White (Citation2016). Seats in the 2010 MY decade are dual recliner designs with an average strength of 3,258 ± 827 Nm (28,830 ± 7,314 inlb). There has been a steady increase in strength since the 1970s along with the transition from single-side to dual recliners. ABTS seats are used in specialty vehicles without a B-pillar or vehicles with other design constraints. They are typically single recliner seats and their strength has declined since the 1990s.
Figure 3. Peak moment and standard deviation in body block tests of single recliner, dual recliner and ABTS seats by decade of vehicle MY (updated from Viano and White Citation2016).
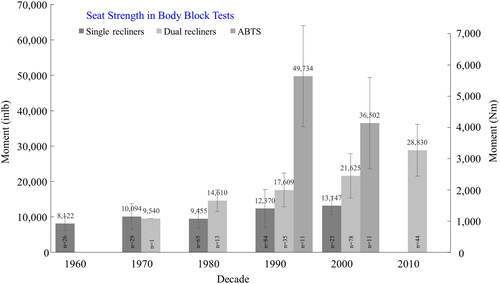
Viano et al. (Citation2021a) evaluated very strong seats in severe rear impacts with the 5th female, 50th male and 95th male Hybrid III dummy. The seats were dual recliner ABTS designs either production or modified designs. A production dual recliner ABTS had a strength of 7,213 Nm (63,827 inlb) in a body block test. A modified Sebring ABTS with dual recliners had a strength of 7,598 Nm (67,229 inlb) in a FRED III test. The body block and FRED II test methods have been described (Viano and White Citation2016; Viano et al. Citation2019b). Dual recliner ABTS seats remained relatively upright accelerating the 5th female forward with forces above tolerance in high-speed rear impacts. The lower neck Nij and extension moment, lower neck shear upper neck Nij, upper neck tension and the HIC36 were above tolerance with responses up to 245% of IARV. The testing shows the need for ride-down distance or yielding of the seatback to accelerate smaller occupants forward more gradually with upright seats.
It is possible to design very strong seats, but there is a need for ride-down distance by compliance of the seatback (Viano et al. Citation2021a). However, the seat needs to be low stiffness (Viano Citation2003d). This is difficult with limited space in the seatback that accommodates a side airbag on the outboard side of the seat frame, lumbar support mechanisms and suspension-support structures among other components. This points to a drawback in the design of very strong conventional and ABTS seats. All designs support the occupant by loads on the seatback in a rear impact with forces and moments acting across recliners attached to the cushion frame. All of the restraining loads act across the recliners.
Perimeter frame seats
Pocketing of the occupant into the seatback was a hallmark of the high retention seat that is widely used today (Viano Citation2002). The high retention seat replaced seatback rotation by pocketing to provide yielding or ride-down distance for the occupant. The seats were stronger than earlier models and included a higher and more forward head restraint to support the head and neck in rear impacts. The perimeter frame design used a strong frame structure with an open seatback to allow pocketing. Any component in the seatback had to be deformable so the occupant could pocket. The pocketing increased retention of the occupant by increase friction forces to include plowing restraint to reduce ramping.
Most modern seats use dual recliners with a x-tube between the inboard and outboard recliners. Over time structures have been added around the x-tube that are stiff and not deformable by the occupant in a rear impact. These structures limit pocketing and cause the occupant to ramp early in rear impacts. This is not a favorable kinematic because once ramping starts, it is difficult to stop the sliding up the seatback. It would be beneficial to use a Bowden cable or flexible connection between the recliners that does not impede the pelvis pocketing into the seatback.
Seats in use today often have multiple adjustments for the seat cushion, tracks and seatback. Whatever upgraded testing occurs, it would be useful to ensure seat performance over the range of adjustability. FMVSS 209 and 210 need to be understood as revisions to seatbelts are made to obtain early restraint in rear impacts. The current geometric requirements were developed for frontal crash restraint. There needs to be flexibility to modify seatbelt anchors and geometry for rear impacts.
Occupant kinematic
NHTSA (Citation2004a) terminated rulemaking on FMVSS 207 seating systems. They concluded that “improving seating system performance is more complex than simply increasing the strength of the seatback. A proper balance in seatback strength and compatible interaction with head restraints and seatbelts must be obtained to optimize injury mitigation. Comprehensive information needed to determine that proper balance is not available, although there has been work on pieces of the problem.”
The occupant can ramp up the seatback with increasing seatback rotation. Ramping is the sliding of the occupant’s hip, torso, head and neck up the seatback. This displaces the head above the head restraint increasing extension of the neck. With sufficient ramping, the occupant contacts the rear seat or an occupant in the 2nd row. Sled testing has shown that modern seats provide very low biomechanical responses in comparison to IARV for testing up to 40 km/h (25 mph) rear delta V (Viano et al. Citation2018). As the severity of the impact increases to higher delta V, the occupant starts to ramp. There are no kinematic controls to guide the preferred movement of the occupant in a severe rear impact.
Adomeit and Heger (Citation1975) and Adomeit (Citation1977, Citation1979) defined motion sequence criteria for favorable and unfavorable occupant kinematics by lap-shoulder belts and seat restraint in frontal crashes. The criteria guided the development of occupant restraints and led to the design of anti-submarining ramps in the front of the seat cushion, belt pretensioners and load limiters. The motion sequence criteria for favorable kinematics included the torso leaning forward of vertical to ensure belt loads on the shoulder and no rearward rotation of the hip as it displaced forward and downward. This ensured the lap belt did not migrate up onto the abdomen causing submarining.
Viano (Citation2022a) extended the motion sequence criteria (MSC) to control occupant kinematics in rear impacts. Figure A1 in the Appendix shows the criteria, which include 1) limiting the angle of the torso (α) rearward of vertical to less than the critical angle (αc) for ramping up the seatback and 2) H-pt displacement rearward and downward, so zHpt < zHpt0, where zHpt0 is the initial height of the H-pt. The lateral displacement of the occupant is 3) limited to less than the critical lateral displacement yT1c, where the head becomes unsupported by the head restraint or the chest by the seatback. The rear MSC aim to contain the pelvis on the seat and provide uniform support of the torso, head and neck.
Anti-ramping guide
Figure A1 shows favorable kinematics in a rear impact that may be achieved over a wider range of crash severities by an anti-ramping guide in the seat cushion frame that directs the hip rearward and downward. The guide provides a load path for the lower torso of the occupant to the seat cushion frame, diverting load from the seatback above the recliners. This lowers the moment supported by the recliners as loads on the guide and seat cushion frame restrain the hips, legs and lower torso. The anti-ramping guide is composed of a shell contoured to the back and bottom of the pelvis and deformable brackets attaching the shell to the cushion frame. The brackets deform allowing the anti-ramping guide to displace along a rearward and downward trajectory. The guide requires an open seatback frame or deformable components in the lower seatback that allow the occupant to pocket and displace with the guide. The displacement provides ride-down to gradually accelerate the occupant through the rear delta V.
Seat component strength
shows that seats in use today are stronger than earlier designs. In the 1990s, the average strength of a dual recliner seats was 1,990 ± 541 Nm (17,609 ± 4,788 inlb). By 2010s, the average strength was 3,258 ± 827 Nm (28,830 ± 7,314 inlb). There was a 63.7% increase in moment strength. With the increased strength, the seatback remains upright in higher severity crashes and transfers higher loads to the occupant. This means higher loads are applied to components of newer seats.
There are several standards for seat components that have not been revised from the early issuances of FMVSS standards. It is appropriate to increase the requirements in the standards to ensure that the head restraint remains on the seat in rear impacts and is strong enough to support higher loads from the occupant. This would require revisions to FMVSS 201 and 202. FMVSS 207 was originally promulgated as a seat anchor standard, but today it is viewed as a seat strength requirement. The 373 Nm (3,300 inlb) has been surpassed by seats since the 1960s. The standard should update the requirements for seat anchors to the vehicle floor, adjusters that change seat position on the tracks, frame structures and recliners. They need to be sturdy for the higher forces on the occupant in severe rear impacts.
The FMVSS 207 standard involves loading an untrimmed seat on a rigid bedplate. It would be appropriate to upgrade the test using a fully trimmed seat with some type of body form that has contours and weight of the seated occupant. Several quasi-static test methods have been used that ensure the weight of the occupant on the seat and the shape of the pelvis and torso during the rear loading. Burnett et al. (Citation2022) reviewed the available quasistatic methods and provides background on the evaluation of seats with occupant loading. FRED was a reasonable test method (Viano et al. Citation2019b). Whatever test is required should involve loading the seatback to 70-75 deg rearward of vertical. Most of the current procedures provide strength data only from the design seatback angle to about 45-50 deg. Field accidents show crashes with large seatback rotation, so the energy management from 50-70 deg should be included in any required evaluation. Quasistatic testing is important during the development of a seat and provides the initial evaluation of a seat design and components for energy management and deformation modes. This sets the stage for sled and crash testing during the vehicle development.
The raising and moving forward of the head restraint was one of the requirements for increasing the strength of front seats (Viano Citation2002, Citation2008). The higher height and small gap of head restraints improved the support of the head and neck with the seatback more upright in rear impacts. The transition to higher and more forward head restraints was aided by IIHS safety ratings for static and dynamic performance of head restraints in a low-speed rear sled test with the BioRID dummy (IIHS Citation2019). NHTSA (Citation2010b) upgraded the requirements of head restraint on front seats, and rear seats if used. This required static positioning for the height and gap behind the head and dynamic performance in a rear sled with the Hybrid III dummy. The improved position has caused the front of the head restraint to protrude forward of the seatback with the reduced gap behind the head. A further revision is needed to ensure that the head restraint posts and guides in the seatback are strong enough to maintain the head restraint on the seat in a severe rear impact. With higher forces on the head restraint and ramping of front occupants, the head restraint is lifted by the occupant’s back and shoulders and guide components break. This can allow the head restraint to pull off the seat.
Seatbelts
Field accident studies have found significant effectiveness of seatbelts in preventing death and injury in rear crashes (Strother and James Citation1987; Evans Citation1991; Kahane Citation2000, Citation2015; Viano and Parenteau Citation2010, Citation2022a). Evans and Frick (Citation1988) used the double-pair comparison method and found seatbelts were 49 ± 14% effective in preventing fatal injury in rear impacts and 22 ± 1% effective in preventing driver ejection. In contrast, sled testing up to 40.2 km/h (25 mph) show that modern seats provide essentially all the occupant restraint with minimal loads in the lap and shoulder belts until later during rebound (Viano et al. Citation2012, Citation2021b). This indicates that the effectiveness of belts occurs later in the crash with movement of the occupant that is not seen in 40.2 km/h (25 mph) sled tests. Front seatbelts need to be redesigned to provide restraint of rearward movement of an occupant early in rear impacts by changes in the lap-belt angle or other webbing configurations.
Rear-impact pretensioner
Viano (Citation2022b) analyzed the lack of restraint provided by lap-shoulder belts in rear impacts with and without pretensioning. The geometry of the lap belt involves and angle (θ) up from the floor as it wraps forward and around the pelvis (Figure A2). The angle is less than 90 deg by design to pull the hips down into the anti-submarining ramp in a frontal impact. In a rear impact, the angle cannot provide restraint of the rearward movement of the occupant until the angle is greater than 90 deg and tension develops in the webbing to resist rearward movement of the occupant. This is very late in the crash and helps explain the lack of restraint in rear impacts with or without pretensioning of the seatbelt on 40.2 km/h (25 mph) tests (Viano et al. Citation2012, Citation2021b).
A different geometry for the lap belt is need to restrain the occupant early in a rear crash. Viano (Citation2022b) offered thoughts on ways to provide early restraint by the lap belt. Figure A2 shows a concept that involves a rear-impact pretensioner that dynamically moves the lap-belt anchor forward and upward while tightening the belts on the occupant in a rear impact. The anchor movement with a rear pretensioner provides a lap-belt angle greater than θ = 90 deg before the occupant starts moving rearward in a rear impact. A rear pretensioner also changes the arc of the lap belt by dynamically moving the anchor up and forward while tightening the belt early in a rear impact. With the change in belt geometry, the lap belt restrains rearward movement of the occupant and pulls the hip down early in a rear impact into an anti-ramping guide.
Wrap-around lap belt
Another concept for early lap-belt restraint involves a wrap-around lap belt that has webbing that wraps rearward around the hips, so the occupant moves into the webbing in a rear impact. Figure A3 shows extra webbing that wraps rearward around the back of the occupant’s hip. In a rear impact, the occupant moves rearward tightening the extra webbing, which is anchored to the seat frame and resists rearward movement of the occupant. The extra webbing can be integrated in the seatback trim and can be a net with larger area supporting the back and hips of the occupant in a rear impact. With the extra webbing anchored to the seat cushion frame, the restraining loads are not acting across the seatback recliners. This lowers the load on the seatback. In a rear impact, the hip moves into the wrap-around belt, which increases tension in the webbing and restrains the hip by forces in the webbing anchored to the cushion frame. This is a second approach to getting belt restraint early in rear impacts and lowering the forces on the seat.
Guarding the area around a rear-seated child
shows that 27.5% of serious injury (MAIS 3 + F) to rear-seated children is by intrusion compressing them against the front seat. The mechanism is the cause of 15.3% of adult injury. also shows that rotation of the front seatback rearward into a child caused 12.3% of serious injury. It was associated with only 2.5% of adult injury. Focusing on children, these mechanisms point to a need of guarding the area around the child, providing room around them and their child safety seat. The guarding would lower risk of being compressed into interior components and would block rotation of the front seat rearward into a child. Viano and Parenteau (Citation2008b) discussed protecting the space around the child with a ring structure that was compatible with child seats to improve the safety of rear-seated children in severe rear crashes. While there is pressure to increase the strength of front seats to address rearward rotation of the seatback, any design change in seats would involve a lengthy phase-in to future vehicles.
The notion that front seats have to be strengthened to protect rear-seated children is not supported by the field data in NASS-CDS and CISS. Stronger seats would require years to phase into production and would not address the millions of vehicles on the road today with children riding in rear seats. Stronger front seats would increase the forces compressing the child with intrusion pushing them forward.
An important factor is the high fraction of older vehicles involved in serious-to-fatal injury to rear-seated children. Any design change in seat strength would require decades to transition into vehicles on the road. Parenteau et al. (Citation2022) found 59% ± 7% of child fatalities in rear impacts was in vehicles 10 or more years old. There is a need to consider countermeasures that work in existing vehicles on the road, as well as improving future vehicles and risk-disbenefit if stiffness increases.
Wrap-around shield or ring structure
One safety approach is to guard the area around the child by a ring structure that prevents the child from being crushed by intrusion pushing them into front seats or the front seat or occupant moving rearward into the child. The ring structure would fit existing and future vehicles with front seats of any type or strength. It would be an after-market component. The ring structure can be used when there is a child in the rear seat and can be stowed if there isn’t a child in the vehicle. It can be moved from vehicle to vehicle.
One possible concept is a ring structure or wrap-around shield shown in Figure A4. It can be used in existing and future vehicles with the consent of parents. It would provide wrap-around protection for a rear-seated child in rear impacts and other crashes. The primary mechanism for serious injury to rear-seated children is intrusion in rear impacts that directly injure the child or compress them into front components. Another mechanism is the front seatback rotating rearward into the child and child seat. The ring structure would block rearward rotation of the front seatback. It would accommodate existing child seats and would be compatible with rear seats of vehicles on the road today. The ring structure attaches to the LATCH anchors in the rear seat and shares the anchor with the tethers for the child seat (NHTSA Citation2003, Citation2004b).
Figure A4 (side view) shows the wrap-around ring bracing against the front seat and providing protected space in front of the child and child seat. The ring structure provides space so the child is not crushed between the rear seat intrusion and front interior. It also provides a brace to limit the rearward rotation of the front seatback. This would prevent the front seatback from rotating rearward into the child by supporting the seatback and limiting its rearward rotation to an angle not sufficient to contact the child or allow the front occupant to ramp up the seatback. This would prevent the front-seatback or front-occupant contacting the child in the rear seat. Another purpose would be to prevent crushing loads on a rear-seated child when intrusion of the rear seatback occurs in a crash. The ring is strong enough to maintain a perimeter around the child as they are pushed forward into the front seat. The ring is strong enough to push the front seatback forward with severe rear intrusion maintaining survival space around the child. Both purposes are to reduce the risk of serious injury to rear-seated children by maintaining survival space around the child in rear impacts.
Figure A4 (top view) shows the wrap-around ring with protected space around the child and child seat. The ring structure fits around the child, rear-facing child seat, forward-facing child seat, convertible child seat or booster seat with a gap around the seated child. The ring structure has ample strength to resist collapse when the forces of a crash displace it into the front seatback or the front seat rotates rearward toward the child. The ring structure attaches to the LATCH anchors at the bight of the rear seat and is braced against the front seatback by telescoping forward to maintain a stable position with space around the child in a crash.
The front of the ring extends forward to meet the front seatback. The extension accommodates different rear-seat packaging and the track and comfort adjustments of the front seat. The ring structure can be connected to the front seat at the head restraint in the seatback or by another fixing to the front seat. The connection adds stability to the position of the ring or shield. The ring can have an arm that extends up the rear seatback to further stabilize it in use and during a crash. While many details of concept need to be evaluated, the approach is offered as a “thought-starter” for what is possible for use in existing vehicles to improve safety of rear-seat children with high usage of rear seats (Viano and Parenteau Citation2021).
Discussion
Crashworthiness test to reduce intrusion
FMVSS 301 assesses fuel system integrity. It originally consisted of a 48.3 km/h (30 mph) rear impact test with an 1,818 kg (4,000 lb) rigid barrier (). FMVSS 301 R involves a more severe offset impact using a lighter deformable barrier (ODB) weighing 1,368 kg (3,015 lb) and a higher test speed of 80.5 km/h (50 mph) with 70% offset (NHTSA Citation2003). FMVSS 301 R has 2.83-times more kinetic energy than the original standard and the offset loading of the rear structures caused more vehicle deformation on the struck side. The phased-in of FMVSS 301 R started September 1, 2006 with a required production percentage of 40%, 70%, and 100% by MY. Vehicles manufactured after September 1, 2008 (2009 MY) had to comply.
FMVSS 301 R reduced the risk of fatal injury. Viano and Parenteau (Citation2016b) analyzed FARS and NASS-CDS data for light vehicles involving non-rollover, rear impacts. The data was analyzed by model year (MY) groups: 1996-2001, 2002-2007 and 2008+ to represent the years prior to, during the phase-in and post-revision phase-in of FMVSS 301. The fatality risk was 20.6% in the 1996-2001, 17.3% in the 2002-2007 and 15.0% in the 2008+ MY vehicles using FARS with the initial crash direction variable (IMPACT1) to the rear. There was a 27.1% reduction in risk with 2008+ MY vehicles manufactured after the revision to FMVSS 301. The NASS-CDS analysis showed that the risk for severe-fatal injury (MAIS 4 + F) was 0.27 ± 0.05% for 1996-2001, 0.30% ± 0.13% for 2002-2007 and 0.08 ± 0.04% for 2008+ MY year vehicles. There was a 70.2% reduction in the risk for severe injury with 2008+ MY vehicles. The revision to FMVSS 301 has reduced the risk of fatal and severe injury in 2008+ MY vehicles. Rear structures have improved in comparison to the earlier models tested by NHTSA (Parenteau et al. Citation2020a,Citationb). It is not possible to parse the effects of other design changes in seats and restraint systems that also increased safety over the study years.
NHTSA improved occupant protection with FMVSS 301 R. The rear structures of vehicles were strengthened for FMVSS 301 R reducing intrusion of the rear occupant compartment in severe rear impacts. This has preserved space in rear seats increasing the severity of rear crashes where intrusion pushes the rear-seat forward. Parenteau and Viano (Citation2020, Citation2022c) found intrusion remained the primary source of serious injury in rear impacts, even as rear structures have improved, so further improvements are needed.
A rear NCAP or Consumer test is proposed that would have higher impact energy and a higher front profile of the deformable barrier than in FMVSS 301 R. It could use the IIHS high-hood barrier that weighs 1,900 kg (4,180 lb) impacting the rear of a vehicle at 88.5 km/h (55 mph). The front profile of the barrier is higher than FMVSS 301 R and better represents a truck or SUV (IIHS Citation2020). Many field accidents also involve over-ride, which engage different structures on the struck vehicle than engaged with FMVSS 301 R. The test would involve 2.80-times more energy than with FMVSS 301 R test and would have 12.3-times the energy as the original FMVSS 301 (). The combination of high front profile and energy would load rear structures and lead to a reduction in intrusion in vehicles that comply. The test should involve four, instrumented crash test dummies, such as two adults in the front seats and two children in 2nd row seats, a 3 yo Hybrid III in a forward-facing child seat behind the driver and a 6 yo either in the vehicle lap-shoulder belts or on a booster seat behind the right-front passenger.
Next generation high retention seats
High retention seats were developed in the 1990s to a target energy transfer of 2,000 J (1,475 ftlb) in a quasistatic seat test (QST), which represented the 50th Hybrid III in a 31.4 km/h (19.5 mph) rear delta V. That crash severity was the average of 10 GM passenger cars in a due-care 35 mph rear FMVSS 301 impact (Viano Citation2002). The final design was tested to 15.4 km/h (22 mph) with an unbelted occupant, which represented 2,708 J (1,996 ftlb) energy transfer to a 50th Hybrid III dummy. The energy transfer capability of 19 high retention seat was 3,229 ± 651 J (2,374 ± 480 ftlb) with seatback rotation up to 60 deg in Appendix QST from Viano (Citation2002, Citation2003a,Citationc). One goal of high retention seats was to retain an unbelted Hybrid III on the seat in crashes up to 30.6 km/h (19 mph). Pocketing the pelvis into the seatback improved retention. The high retention seat retained the belted Hybrid III on the seat with seatback angles of 65-70 deg (Viano Citation2002). The seat also included a higher and more forward head restraint to ensure support of the head and neck.
High retention seats were first introduced in the 1997 Pontiac Grand Prix and 1997 Chevrolet Malibu, among others. They have been found effective in preventing fatal injury in rear crashes. GM converted its fleet to high retention seats from 1997-2002. Starting in 1999, there was a migration by the industry to high retention seat designs. Most manufacturers have adopted them today. Viano (Citation2003b) analyzed 1991-2000 FARS data to assess the safety performance trends in the first four years of high retention seats in preventing fatalities. The 1991-2000 FARS analysis showed that high-retention seats reduced the odds of fatal injury by 30.4% (0.9%-51.1%, p < 0.05) when compared to baseline seats.
Viano and Parenteau (Citation2015a) updated the analysis by adding 8 years of FARS data. The fatality risk was 16.6% in high retention seats and 27.1% in baseline seats. The reduction in odds for fatal injury was 46.3% (39.3–52.4 CI) with high retention seats. The reduction was statistically significant with z = 9.982, p < 0.0001. Viano and Parenteau (Citation2015b) repeated the 2014 study using 2001-2008 NASS-CDS and found that the odds for fatality were 46.8% (39.8%-53.0%, 95% confidence interval, z = 10.001, p < 0.0001) lower with high retention seats. They also found a trend for lower serious injury.
Anti-ramping guide
A next generation high retention seat should include load paths to the seat cushion frame during restraint of an occupant in rear impacts. This would lower forces and moments acting on the recliners and lower seatback rotation. One concept is to include an anti-ramping guide that supports the pelvis and is attached the seat cushion frame by deformable brackets. The anti-ramping guide provides a load path the to the seat cushion diverting forces that normally act across the recliners. The guide would direct the motion of the pelvis rearward and downward to better support the head and neck by the head restraint and reduce ramping. The idea of further strengthening recliners in the seat does not provide an approach to control motion of the hip.
Motion sequence criteria
The benefits of anti-submarining ramp in the front of the seat are well known in frontal crashes. A similar concept such as the anti-ramping guide is needed to control hip motion in rear impacts. The use of motion sequence criteria (MSC) would help during the development and validation of seats in rear impact testing. It will lead to seat designs with more favorable occupant kinematics and less ramping in high-speed rear impacts.
Changes to front seats need to consider front-occupant protection
Any changes to front seats need to consider two new injury mechanisms that have appeared with the introduction of stronger front seats over the past 20 years (Viano Citation2011; Viano and White Citation2016; Viano et al. Citation2019a). New injury mechanisms have emerged in field accidents with seats that remain upright in rear impacts. One mechanism is hyper-extension around the upright seatback frame causing fracture-dislocation of the thoracic spine. Viano (Citation2011) identified four cases of the upper body hyper-extending rearward around the frame of the seatback. The fractures were very severe and often involved complete transection of the thoracic spine. The thoracic spine (T-spine) extended around the frame of the seatback, which concentrated forces on the spine, acted as a fulcrum and caused hyper-extension fracture-dislocations.
Another mechanism is spinal fracture of occupants who remain in the seat and experiences forces on the chest that extend the spine and exceed their tolerance. Viano et al. (Citation2019a) identified extension fractures of the thoracic spine with the occupant remaining in the seat during a rear impact. The head, neck and shoulder moved rearward loading the upper seatback and head restraint as the occupant was accelerated forward. The forces on the spine were sufficient to try and extend the thoracic spine. In an occupant with DISH and other calcifications of the spine, the spine tried to extend but fractured near the apex of the thoracic kyphosis. The two mechanisms of injury involved older occupants with spinal disorders. The spinal fractures occurred in low-to-moderate speed rear impacts and were related to the lower tolerance of older occupants. Increasing the strength of front seats needs to consider the downside risks by stiffening front seats. It would increase the stiffness of the seat and increase forces on the occupant accelerating them up through the delta V, when lowering the stiffness is needed.
The T-spine injuries occur in low-speed rear impacts. In contrast, the intrusion mechanism injuries occur in high-speed impacts. The exposures are very different. Table A7 shows the exposure and severe injury in rear impacts by delta V. For belted occupants, 88.8% of exposure to towaway crashes occurs for delta V < 32 km/h (20 mph). In contrast, only 0.36% of exposure occurs for crashes with > 64 km/h (40 mph). For severe injury (MAIS 4 + F) to belted occupants, 21.7% of exposure involves crashes with delta V < 32 km/h (20 mph) and 26.2% occurs for crashes with > 64 km/h (40 mph). The mechanisms of injury are different in very low- and very high-speed rear impacts (Viano and Parenteau Citation2008d). There are other downsides with ABTS (White et al. Citation2019).
Seatbelt restraint early in rear impacts
Sled testing up to 40.3 km/h (25 mph) delta V showed no early lap-belt restraint of the rearward movement of the occupant with or without pretensioning. Viano et al. (Citation2012) reported on two rear sled tests with a 2008 F-150 buck and driver seat. The first test (H31208) involved the production lap-shoulder belts and the second test (H31209) involved buckle pretensioning at 20 ms. Except for a spike in lap-belt load with pretensioning, the belts provided essentially no restraint of the rearward movement of the dummy. Viano et al. (Citation2021b) next reported on three rear sled tests with a Citation2016 Ford Escape buck and driver seat. The first test (H41148) involved the production lap-shoulder belts, the second test (H41149) involved retractor and anchor pretensioning at 60 ms and the third test (H41150) involved retractor pretensioning at 60 ms. Again, except for spikes in seatbelt load with pretensioning, the belts provided essentially no restraint of the rearward movement of the dummy.
Rear-impact pretensioner
Viano (Citation2022b) analyzed the lack of restraint by seatbelts with and without pretensioning and determined the lack of restraint was due to an unfavorable angle of the lap belt. Figure A2 shows that with the lap-belt angle less than 90 deg, there is no direction of force to restrain rearward movement of the occupant. The angle must to be >90 deg for a component of load in the belt to resist rearward movement. The rearward restraint increases with angles >90 deg and lap belt load. A rear impact pretensioner was designed to dynamically move the lap-belt anchors forward and upward to give a lap belt angle >90 deg before the occupant starts moving rearward. With this orientation, tension in the lap belt resists rearward movement of the occupant.
Wrap-around lap belt
Figure A3 shows extra webbing that wraps rearward around the occupant’s hip. In a rear impact, the occupant moves rearward tightening the wrap-around webbing, which is anchored to the seat frame and resists rearward movement of the occupant. With the webbing anchored to the seat cushion frame, the restraining loads are not acting across the seatback recliners lowering loads on the seatback and providing early restraining forces on the occupant.
Interior head impacts below the beltline
FMVSS 201 originally addressed head impacts with the instrument panel, seatback, glove box, sunvisor and armrest (NHTSA Citation1992; Kahane and Tarbet Citation2006). FMVSS 201 was upgraded in 1995 (NHTSA final rule Citation1995a,Citationb,Citationc) to include locations for head contact with the vehicle’s front, side, rear and upper interior above the beltline. FMVSS 201 U involved more impact locations on the front, side and rear of the interior above the beltline. The standard involved a 24.1 km/h (15 mph) impact with an instrumented headform based on the Hybrid III that was called FMH (free motion headform). The head response requirements were also modified. The original requirement was an 80 g limit for 3 ms head acceleration. In the revised requirement, the Head Injury Criterion HIC(d) was limited to 1000 for any 36 ms period. FMVSS 201 U phased-in from 1999 to 2003 model year with 10% for 1999 MY, 25% for 2000 MY, 40% for 2001 MY, 70% for 2002 MY, 100% for 2003 MY. The revision was modified to include a pole impact test option for vehicles equipped with head airbags, generally curtain airbags. The test consisted of a 29.0 km/h (18 mph) right-angled impact with a 25.4 cm (10”) diameter pole. If chosen, the vehicle must be tested with an undeployed airbag. In return, the vehicle could meet the interior impact requirements with the free motion headform at a lower impact speed of 19.3 km/h (12 mph) for areas within 2” (50 mm) of the curtain airbag coverage.
shows that 73.0% of serious injury to children involves a head impact. The sources are the front seatback, side interior and B-pillar area below the beltline. An extension of FMVSS 201 U is proposed to address area of rear seats below the beltline. A FMVSS 201 L would involve a 6 yo Hybrid III headform with scaled requirements following the FMVSS 201 U procedures with specified impact locations and angles on the rear interior below the beltline. This recommendation was first made in 2003 (Parenteau and Viano Citation2003b). The standard would lower head injury risks for children in areas of the rear interior not covered by a standard. FMVSS 201 L would improve padding for interior surfaces struck by rear-seated children. This would include areas below the beltline in rear seat areas. Arbogast et al. (Citation2012) tested the back of front seats because they were a likely contact for rear-seated children in frontal impacts.
Guarding the space around the child
Universal attachments for child seats, ISOFix and LATCH
In 1972, the Federal standard for child seat tethering involved a static pull test specified in FMVSS 213. In 1981, FMVSS 213-80 was revised and included a sled test that specified an 81.3 cm (32”) limit on forward excursion of the child dummy with or without a tether when the forward-facing child seat was subjected to a 48.3 km/h (30 mph) test. In 1985, the standard was revised so child seats had to pass the sled test without a tether. As a result, top tethers became less prevalent until the mid-1990s when field data on the benefits of child seats with tethers prompted renewed interest.
In the 1990s, ISO (International Standards Organization) developed a universal child restraint anchorage system that allowed child seats to install in vehicles without seatbelts. It was called ISOFix and involved two bars in the seat bight and rigid lower anchors on the child seat. ISOFix continued to evolve with flexible lower anchors on the child seat. NHTSA mandated that top tether anchors must be in place in new cars and top tether straps must be on all front-facing child seats starting with 80% after 9-1-99 and 100% after 9-1-00. A top tether prevented the child seat from tipping forward in a frontal crash and reduced the movement of a child’s head.
NHTSA issued FMVSS 225 (the LATCH standard) that required lower anchors be phased-in on 20% of vehicles after 9-1-00, 50% after 9-1-01 and 100% after 9-1-02 (NHTSA Citation2003, Citation2004b). LATCH required hooks for the attachment of lower tethers on child seats. LATCH was a variation of ISOFix in the U.S. It made the installation of child seats easier by standardizing the attachments to the vehicle and eliminating incompatibilities with vehicle seatbelt systems. The LATCH anchors became a structural point on the vehicle.
Wrap-around ring
The NASS-CDS and CISS cases show there is benefit in guarding the space around the rear-seated child if there is intrusion-related or non-intrusion-related injury. A ring of protection would prevent the front seat from rotating rearward allowing the front occupant or seat to impact a rear-seated child. The concept of a ring structure around the child is an after-market device that fits the LATCH system and braces against the back of the seatback in front. Most vehicles have two or three rear seating positions and the right-front passenger seat with LATCH attachments. The child sits in the rear of the vehicle and is protected by the ring structure, which minimizes the collapse of space around the child.
The ring structure works with existing and future vehicles irrespective of the strength or design of the front seat. Since the field data shows that injured rear-seated children are pushed into relatively upright front seats, further strengthening of the front seat would make a stiffer barrier that the child is pushed into and would increase injury risks, rather than decrease them for this mechanism of injury.
The ring structure is an after-market product that is voluntarily used by parents if there is concern for injury to children in rear seats in rear impacts. The ring structure limits front seatback rotation and stiffens the front seat. The stiffening increases the risk for injury to the front occupant, who uses the system to protect their children riding in rear seats. Parents who use the ring structure or ring do so with the understanding that there is an increase in risk for their injury as the safety of their rear child is improved. The parent makes the decision to protect their children over their own safety.
The parent would accept a higher risk of intrusion forces on the ring deforming the front seat forward for the benefit of their child’s safety riding in a rear seat. The ring structure attaches to the vehicle LATCH system and wraps around the child. The ring can be detached and stored in the trunk of the vehicle when there is not a rear-seated child in the vehicle. The ring is used only when it benefits the safety of a rear-seated child and is not installed in the rear seat when there is no child in the seat.
Phase-in of countermeasures
An after-market safety countermeasure, such as the wrap around ring, can be phased into use much quicker than a vehicle design change. Foremost, the ring can be used in existing vehicles because it fits vehicles in use today. The older vehicles have seats with lower strength, so need the protection of a ring around the child more than modern seats that are stronger. An aftermarket product has a different phase-in than a technology in new vehicles. For example, the age of vehicles in use can be roughly considered new to 20 years old. There is about an equal number of vehicles up to 6 years old and then a linear decrease as the vehicle age increases based on exposure data, which has a newer vehicle age profile than serious-injury and fatal crashes (). This was approximated in Figure A5 with 7.69% of the fleet per year up to 6 years old and then a decrease with 5.13% of the fleet 10 years old, 2.56% of the fleet 15 years old to 0.00% 20 years old.
If the countermeasure involves a change in the vehicle, the phase-in occurs with the production and sale of new vehicles. Figure A6 shows the penetration of the countermeasure in the fleet assuming the phase-in to new vehicles is 25% in 2025, 50% in 2026 and 100% in 2027. By 2027, only 13.5% of the fleet has the change. By 2030, only 36.5% has the change. If the countermeasure is an after-market product, the phase-in can be assumed 25% in 2025 for all existing vehicles with rear-seated children, 50% in 2026 and 100% in 2027. The penetration of the countermeasure in relevant vehicles is complete within three years, assuming a motivated public buying the safety feature for their rear-seated children or incentives to make the component available.
Figure A6 shows the gap in penetration with the slower penetration of a vehicle change compared to an after-market component. By 2027, 86.5% of vehicles in use do not have a countermeasure. By 2030, 63.5% of vehicles in the fleet still do not have the change. The fleet is 90% converted only after 16 years of phase-in of a vehicle change. In contrast, the after-market component is available in all relevant vehicles, if the parent or caregiver is motivated to use the countermeasure.
Priorities, countermeasures and concepts
Approaches are proposed to improve the safety of rear seated children and adults and front-seated occupants in rear impacts:
NCAP or consumer rear impact test with the 1,900 kg (4,180 lb) IIHS high-hood, offset deformable barrier (ODB) at 88.5 km/h (55 mph) to reduce rear-seat intrusion and assess occupant kinematics,
stepped-up public campaigns for proper child seat selection and use, particularly for infants and toddlers <2 yo and for adult use of seatbelts in rear seats with focus on different SES,
adoption of FMVSS 201L to reduce child head injury risks with impact on the rear- and side-interior below the beltline,
improved design of front seats by shifting restraining forces from the seatback to the cushion frame with an anti-ramping guide that retains the pelvis on the seat and reduces seatback rotation,
changes in front seatbelts to provide early restraint of front-occupant movement by a rear-impact pretensioner that moves the lap-belt anchors and gives a favorable angle for early lap-belt restraint of rearward movement or a wrap-around the rear of the hip lap belt so the occupant’s pelvis moves into a belt in rear impacts,
use of kinematic controls in Motion Sequence Criteria (MSC) to ensure movement of front occupants that reduces ramping in severe, rear-impacts,
guarding the area around rear-seated child with an after-market ring structure that maintains space around the child and blocks rearward rotation of the front seatback, if the parent or guardian approves,
automatic emergency braking on tractor-trailers, heavy trucks and light vehicles to reduce the severity of rear impacts,
improved compatibility of the rear- and front-structures of vehicles to control crush and intrusion in rear impacts that are offset, oblique PDOF and involve over-ride or under-ride,
upgrading the requirements in FMVSS 202, 201 and 207 to recognize higher forces on the seat in modern vehicles with integrity of adjusters in a range of positions, anchors to the floor and head restraints,
FMVSS 202 (NHTSA Citation2010b) led to the front of the head restraint protruding forward of the seatback to reduce the gap behind the occupant’s head. A revision is needed to ensure attachments are strong enough to maintain the head restraint on the seat and minimize component breakage,
FMVSS 207 needs revision to ensure modern seats maintain the adjusted position as they deform rearward and there is no separation of components in the load-path supporting the seatback and head restraint. A device like FRED is reasonable for assessing energy management and deformation modes before sled and crash testing,
upgrade FMVSS 209, 210 and 207 to allow changes in lap-belt systems that provide earlier occupant restraint in rear impacts,
upgrading FMVSS 213 to address the performance of rear-facing, front-facing and booster child seats in rear impacts,
ensure that seat anchors, tracks, adjusters and frames withstand the forces of occupant load in an NCAP or consumer test at 88.5 km/h (55 mph) with a 1,900 kg (4,180 lb) offset deformable barrier (ODB),
better understanding of older vehicle crashes, occupant restraint misuse and improper transport of children, since >50% of fatal crashes involve vehicles >10 yo,
reinstating the investigation of vehicles over 10 years old in CISS, so the safety community has information on this stratum of accidents, other than FARS,
NHTSA collecting dynamic data on cg (center of gravity) acceleration, yaw and pitch of the struck vehicle in FMVSS 301R tests and the NCAP or Consumer crash test to include vehicle dynamics, post-test displacement of rear seats and structures and the CDR readout from the vehicle black box,
requirement that vehicle manufacturers trigger side curtains and airbags in severe rear impacts for ejection mitigation and to prevent head injuries because of the high fraction of unrestrained occupants in rear seats,
NHTSA implementing a translator to convert injury data in earlier years of NASS-CDS from AIS 90, AIS 98, AIS 05, AIS 08 to AIS 15 and any future version of injury coding used by the Agency. It is inappropriate to direct the safety community to stop using older data. The full years of data are important to tracking safety progress and quantifying changes. There is an attitude to ignore the past, just because the injury coding is not identical and only newer vehicles are investigated,
add warnings and reminders of unlatched seatbelts with rear adults and older children to increase seatbelt use,
by using motion sequence criteria that ensure the occupant pockets in the seatback, the seatback needs to remove stiff components that cause early ramping. Once ramping starts, it is hard to stop the sliding. Replace the x-tube with another connection between the recliners, such as a Bowden cable, or ensure the x-tube can deform rearward without causing ramping,
NHTSA and IIHS make rear testing available to researchers with complete data including vehicle dynamics, dummy responses, interior deformation and high-speed video with timing, so product performance can be analyzed,
NHTSA should reinstate HARM as a metric they use to measure progress and update priorities for sensible means to improve crash safety. They should tie the annual crash statistics to changes in HARM so there is an objective view of progress and needs. They should develop a complimentary metric for crash avoidance,
countering the notion that strengthening front seats is the “solution” to protect rear-seat children. NHTSA is aware that safety is more than seat strength (NHTSA Citation2004a). They should not let loud voices interfere with their conducting the needed steps to understand how children are injured in rear impacts and identifying sensible ways to improve safety.
Supplemental Material
Download Zip (316.6 KB)Acknowledgments
The sled test data and videos in the study may be made available upon request. The author has no direct or indirect financial interest in any of the countermeasures or concepts presented in this study. The anti-ramping guide, rear-impact pretensioner, wrap-around ring and other concepts have been discussed in US patents and applications owned by a non-profit devoted to supporting research and education on automotive safety and occupant protection. More information on the concepts can be found in the pelvic restraining strap (US10,723,251 B2, July 28, 2020), wrap around seatbelt (US10,946,829 B2, March 16, 2021), wrap around shield (US11,427,113 B1, August 30, 2022), system and method for protecting an occupant in a rear impact of a vehicle: anti-ramping guide (EFS 45220162, application o#: 17/693900, 3-14-22) and system and method for protecting an occupant in a rear impact of a vehicle: rear pretensioner (EFS 45220927, application o#: 17/693977, 3-14-22).
Additional information
Funding
References
- AAM. 2016. Global Automakers and Alliance of Automobile Manufacturers, letter to Senator Markey and Blumenthal, June 23.
- Adomeit D, Heger A. 1975. Motion Sequence criteria and design proposals for restraint devices in order to avoid unfavourable biomechanic conditions and submarining. SAE 751146, 19th Stapp Car Crash Conference, Society of Automotive Engineers, Warrendale, PA.
- Adomeit D. 1977. Evaluation methods for the biomechanical quality of restraint systems during frontal impact. SAE Paper #770936, 21st Stapp Car Crash Conference, SAE International, Warrendale, PA.
- Adomeit D. 1979. Seat design-A significant factor for safety belt effectiveness. SAE 791004. Warrendale, PA: SAE International.
- Arbogast K, Maltese M, Locey C, Bohman K. 2012. Headform impact tests to assess energy management of seat back contact points associated with head injury for pediatric occupants. SAE Int J Passeng Cars – Mech Syst. 5(1):454–467. doi:10.4271/2012-01-0561
- Burnett RA, Viano DC, Parenteau CS. 2022. Quasi-static methods to evaluate seat strength in rear impacts. Traff Inj Prev. 1–6. doi:10.1080/15389588.2022.2140280
- Ditlow CM. Petition for warning placed on rear seats. Center for Auto Safety; [accessed 2016 March 9]. https://www.autosafety.org/nhtsa-urged-to-warn-parents-of-seat-back-failure-dangers-to-children-in-rear-seats.
- Evans L, Frick M. 1988. Seating position in cars and fatality risk. Am J Public Health. 78(11):1456–1458. doi:10.2105/ajph.78.11.1456
- Evans L. 1991. Traffic safety and the driver. New York: Van Nostrand Reinhold. ISBN 0-442-00163-0.
- Haddon W, Jr Suchman EA, Klein D, editors. 1964. Accident research: methods and approaches. New York, NY: Harper and Row.
- Haddon W. Jr 1972. Reducing highway losses: a logical framework for categorizing highway safety phenomena and activity. J Trauma. 12(3):193–207.
- IIHS. 2020 October. Side impact crashworthiness evaluation moving deformable barrier 2.0 specification (Version I). Ruckersville, VA: Insurance Institute for Highway Safety. www.iihs.org.
- Kahane CJ, Tarbet MJ. 2006 November. HIC Test Results before and after the 1999-2003 Head Impact Upgrade of FMVSS 201, NHTSA Technical Report, DOT HS 810 739.
- Kahane CJ. 2000 December. Fatality reduction by safety belts for front-seat occupants of cars and light trucks. NHTSA, DOT HS 809 199.
- Kahane CJ. 2015 January. Lives saved by vehicle safety technologies and associated Federal Motor Vehicle Safety Standards, 1960 to 2012 – Passenger cars and LTVs – With reviews of 26 FMVSS and the effectiveness of their associated safety technologies in reducing fatalities, injuries, and crashes. Report No. DOT HS 812 069. Available from National Highway Traffic Safety Administration, Washington, DC.
- Malliaris A, Hitchcock R, Hansen M. 1985. Harm causation and ranking in car crashes. SAE 850090. Warrendale, PA: Society of Automotive Engineers.
- Malliaris A, Hitchcock R, Hedlund J. 1982. A search for priorities in crash protection. SAE 820242. Warrendale, PA: Society of Automotive Engineers.
- Markey EJ, Blumenthal R. 2016 May 25. Letters to CEOs of automotive manufacturers on the company’s efforts to protect passenger against the threat of front seatback failures. US Senate, Washington DC.
- Markey EJ, Blumenthal R, Brown S, Durbin D, Klobuchar A, Lujan B, Reed J, Hollen C, Warren E, Wyden R. 2022 Nov 15. Letter to Ann Carlson, Administrator, NHTSA requestion an update on NHTSA’s efforts to implement the Infrastructure Investment and Jobs Act’s (IIJA) requirements. US Senate, Washington DC .
- Morrall JF. 1986 November/December. A review of the record. Regulation 25-34.
- NAS. 1985. Injury in America: A continuing public health problem. Washington, DC: Committee on Trauma Research, Commission on Life Sciences, National Research Council and the Institute of Medicine, National Academy Press.
- NHTSA. 2004a. Federal Motor Vehicle Safety Standards; Seating Systems. Termination of Rulemaking. Federal Register. 69(220):67068–67070. Tuesday, November 20,
- NHTSA. 2003 Dec 1. Federal Motor Vehicle Safety Standards – Fuel Systems, 49 CFR Parts 571 and 586, Docket No. NHTSA–03–16523.
- NHTSA. 2003 Jun 27. Federal Motor Vehicle Safety Standards; Child Restraint Systems, Child Restraint Anchorage Systems 225. Federal Register. 68(124):38208–38231. Friday.
- NHTSA. 2004b Aug 11. Federal Motor Vehicle Safety Standards; Federal Motor Vehicle Safety Standards; Child Restraint Anchorage Systems 225. Fed Regist. 69(154):48818–48825. Wednesday.
- NHTSA. 2010b Nov 2. Federal Motor Vehicle Safety Standards; Head Restraints. 49 CFR Part 571, Docket No. NHTSA–2010–0148, Federal Register. 75(211):67233–67233.
- NHTSA. 1995a. Final economic assessment, FMVSS 201, upper interior head protection Washington: NHTSA Plans and Policy.
- NHTSA. 1995b. Federal Motor Vehicle Safety Standards – Head Impact Protection, 49 CFR Parts 571, 572, and 589, Docket No. 92-28; Notice 7 [RIN No. 2127-AB85].
- NHTSA. 1995c Aug 18. Head impact protection. Final Rule.
- NHTSA. 2010a. National Automotive Sampling System (NASS) Crashworthiness Data System, Analytical User’s Manual 2010 Files. DOT HS 811 532, National Center for Statistics and Analysis, National Highway Traffic Safety Administration, U.S. Department of Transportation, Washington, D.C.
- NHTSA, 1992 Dec. Preliminary regulatory impact analysis: upper interior head protection FMVSS 201. National Highway Traffic Safety Administration, Plans and Policy, Office of Regulatory Analysis.
- NHTSA. 1992 Sep. Summary of Safety Issues Related to FMVSS No. 207, Seating Systems.
- NHTSA. 2002 May 1. Federal Motor Vehicle Safety Standards Child Restraint Systems, Proposed Rule. Federal Register. 67(84):21806–21836. Wednesday.
- IIHS. 2019 Dec. Vehicle Seat/Head Restraint Evaluation Protocol Dynamic Criteria (Version V). IIHS, 988 Dairy Rd, Ruckersville, VA.
- Parenteau C, Croteau J, Zolock J. 2020a. The effect of crash severity and structural intrusion on ATD responses in rear-end crashes. SAE 2020-01-1224. Warrendale, PA: Society of Automotive Engineers. doi:10.4271/2020-01-1224.
- Parenteau C, Stephens G, Yaek J, Gregory S. 2020b. The effect of FMVSS 301R on vehicle structure in rear impact. SAE 2020-01-1226. Warrendale, PA: Society of Automotive Engineers. doi:10.4271/2020-01-1226.
- Parenteau C, Viano DC. 2003a. Field data analysis of rear occupant injuries part I: adults and teenagers. SAE 2003-01-0153. Warrendale, PA: Society of Automotive Engineers.
- Parenteau C, Viano DC. 2003b. Field data analysis of rear occupant injuries Part II: children, toddlers and infants. Warrendale, PA: Society of Automotive Engineers.
- Parenteau CS, Burnett RA, Viano DC, Lau E. 2021a. Effect of ABTS and conventional seats on occupant injury in rear impacts: analysis of field and test data. Traffic Inj Prev. 22(2):147–152. doi:10.1080/15389588.2020.1849644
- Parenteau CS, Viano DC, Lau E. 2022. Fatalities of second-row children in front, side and rear impacts by calendar year (CY) and model year (MY). SAE 2022-01-0860. Warrendale, PA: Society of Automotive Engineers.
- Parenteau CS, Viano DC, Lau EC. 2021b. Rear-seat occupant demographics in rear impacts: analysis of NASS-CDS. Traffic Inj Prev. 22(3):218–223. doi:10.1080/15389588.2020.1871474.
- Parenteau CS, Viano DC. 2020. An update on 2nd row children responses in rear and frontal crashes. SAE 2020-01-1215. Warrendale, PA: Society of Automotive Engineers.
- Parenteau CS, Viano DC. 2022a. Head and face injury to 2nd row occupants by crash type with focus on basilar and facial fractures in side and rear impacts. Traffic Inj Prev. 23(5):238–243. doi:10.1080/15389588.2022.2067330
- Parenteau CS, Viano DC. 2022b. Size and age of fatal drivers by crash type, vehicle type and gender. Traffic Inj Prev. 1–5. doi:10.1080/15389588.2022.2143235
- Parenteau CS, Viano DC. 2021. Serious head, neck and spine injuries in rear impacts: Frequency and sources. IRCOBI Conference, IRC-21-10:1-12.
- Parenteau CS, Viano DC. 2022c. Serious-to-fatal injury to second-row occupants in rear impacts using 1994-2020 field data. Traffic Inj Prev. 1–5. doi:10.1080/15389588.2022.2140279
- Rosekind MR. 2017. Letters to senators EJ Markey and M Blumenthal on Petition for rulemaking on seatback strength. NHTSA, US Department of Transportation. Washington DC. 1-13-17.
- Strother CE, James MB. 1987. Evaluation of seat back strength and seat belt effectiveness in rear end impacts. 31st Stapp Car Crash Conference, SAE 872214, Society of Automotive Engineers, Warrendale, PA.
- Viano DC, Burnett R, Miller GA, Parenteau CS. 2021b. Influence of retractor and anchor pretensioning on dummy responses in 40 km/h rear sled tests. Traffic Inj Prev. 22(5):396–400. doi:10.1080/15389588.2021.1910243
- Viano DC, Burnett R, White S, Stephens G, Parenteau CS. 2021a. Dual-recliner ABTS seats in severe rear sled tests with the 5th, 50th and 95th hybrid III. SAE 2021-01-0917. Warrendale, PA: Society of Automotive Engineers.
- Viano DC, Burnett R, White S. 2019b. FRED II quasistatic seat testing rearward: An improved method based on the SAE H-point Manikin. SAE 2019-01-1032. Warrendale, PA: Society of Automotive Engineers.
- Viano DC, editor. 2003a. The debate between stiff and yielding seats: a new generation of yielding seats with high retention in rear crashes. Warrendale, PA: Society of Automotive Engineers.
- Viano DC. 2003d. Influence of seat properties on occupant dynamics in severe rear crashes. Traffic Inj Prev. 4(4):324–336.
- Viano DC, Parenteau CS, Burnett R, Prasad P. 2018. Occupant responses in conventional and ABTS seats in high-speed rear sled tests with a normally seated dummy. Traffic Inj Prev. 19(1):54–59. doi:10.1080/15389588.2017.1347782
- Viano DC, Parenteau CS, Burnett R. 2012. Influence of belt pretensioning on dummy responses in 40 km/h rear impact sled tests. Traffic Inj Prev. 13(1):65–71. doi:10.1080/15389588.2011.629558
- Viano DC, Parenteau CS, Prasad P, Burnett R. 2008. Occupant responses in high-speed rear crashes: analysis of government-sponsored tests. SAE 2008-01-0188. Warrendale, PA: Society of Automotive Engineers.
- Viano DC, Parenteau CS, White S. 2019a. Influence of DISH, ankylosis, spondylosis and osteophytes on serious-to-fatal fracture-dislocation of the spine and spinal cord injury in rear impacts. SAE 2019-01-1028. Warrendale, PA: Society of Automotive Engineers.
- Viano DC, Parenteau CS. 2021. Change in seating position of children in towaway crashes from 1989–2019. Accid Anal Prev. 159:106280. doi:10.1016/j.aap.2021.106280
- Viano DC, Parenteau CS. 2016b. Effectiveness of the revision to FMVSS 301: FARS and NASS-CDS analysis of fatalities and severe injuries in rear impacts. Accid Anal Prev. 89(1–8):1–8.
- Viano DC, Parenteau CS. 2010. Ejection and severe injury risks by crash type and belt use with focus on rear impacts. Traffic Inj Prev. 11(1):79–86. doi:10.1080/15389580903479186
- Viano DC, Parenteau CS. 2008c. Fatalities by seating position and principal direction of force for 1st, 2nd and 3rd row occupants. SAE 2008-01-1850. Warrendale, PA: Society of Automotive Engineers.
- Viano DC, Parenteau CS. 2008a. Fatalities of children 0-7 years old in the 2nd row. Traffic Inj Prev. 9(3):231–237. doi:10.1080/15389580801957671
- Viano DC, Parenteau CS. 2008b. Field accident data analysis of 2nd row children and individual case review for safety priorities. SAE 2008-01-1851. Warrendale, PA: Society of Automotive Engineers.
- Viano DC, Parenteau CS. 2022a. Injury by delta v in front, near-side, far-side and rear impacts: analysis of 1994-2015 NASS-CDS. SAE 2022-01-0835. Warrendale, PA: Society of Automotive Engineers.
- Viano DC, Parenteau CS. 2015b. NASS-CDS analysis of high retention seat performance in rear impacts. Traffic Inj Prev. 16(5):491–497.
- Viano DC, Parenteau CS. 2008d. Serious injury in very-low and very-high speed rear impacts. SAE 2008-01-1485. Warrendale, PA: Society of Automotive Engineers.
- Viano DC. 2008. Seat design principles to reduce neck injuries in rear impacts. Traffic Inj Prev. 9(6):552–560. doi:10.1080/15389580802381939
- Viano DC, Parenteau CS. 2022b. Significance of tractor-trailer impacts to the rear of light vehicles. Traff Inj Prev. 23(4):169–175. doi:10.1080/15389588.2022.2033974
- Viano DC, Parenteau CS. 2016a. Update of “Field accident data analysis of 2nd row children and individual case reviews. SAE 2008-01-1851. ProBiomechanics Report to NHTSA.” 6-3-16.
- Viano DC, Parenteau CS. 2015a. Update on the effectiveness of high retention seats in preventing fatal injury in rear impacts. Traffic Inj Prev. 16(2):154–158.
- Viano DC. 2002. Role of the seat in rear crash safety. SAE Book, ISBN 0-7680-0847-6. Warrendale, PA: Society of Automotive Engineers, SAE R-317:1-491.
- Viano DC, White SD. 2016. Seat strength in rear body block tests. Traffic Inj Prev. 17(5):502–507. doi:10.1080/15389588.2015.1111513
- Viano DC. 2022b. Analysis of the lack of restraint with and without belt pretensioning in 40.2 km/h rear impacts. Traff Inj Prev. 1–7. doi:10.1080/15389588.2022.2121143
- Viano DC. 2003b. Effectiveness of high-retention seats in preventing fatality: Initial results and trends. SAE 2003-01-1351. Warrendale, PA: Society of Automotive Engineers.
- Viano DC. 2011. Fracture-dislocation of the thoracic spine in extension with upright seats in severe rear crashes. SAE 2011-01-0274. Warrendale, PA: Society of Automotive Engineers.
- Viano DC. 1989a September. Head injury research needs. Testimony submitted to the interagency head injury task force. Washington, DC: Department of Health and Human Services; p. 58–61.
- Viano DC. 2003c. High retention seat performance in quasistatic seat tests. SAE 2003-01-0173. Warrendale, PA: Society of Automotive Engineers.
- Viano DC. 2022a. Motion sequence criteria for favorable occupant kinematics in rear impacts. Traff Inj Prev. 1–7. doi:10.1080/15389588.2022.2138709
- Viano DC. 1990 Mar 8. Testimony Before the Subcommittee on Health and the Environment of the Committee on Energy and Commerce. House of Representatives Hearings about the Federal Programs on Injury Control and Disability Prevention. Serial. No 101-139.
- Viano DC. 1989b Oct 17. Testimony Before the United States Senate Committee on Environment and Public Works Subcommittee on Water Resources, Transportation, and Infrastructure Concerning Senate Bill S.1007. National Highway Fatality and Injury Reduction Act of 1989.
- White S, Viano DC, Burnett R. 2019. Seat performance and occupant moving out of the shoulder belt in ABTS in rear impacts. SAE 2019-01-1031. Warrendale, PA: Society of Automotive Engineers.
- Williams AF. 1999 May 5–9. The Haddon matrix: its contribution to injury prevention and control. 3rd National Conference on Injury Prevention and Control.
- Wilson R. 1983. Vehicle safety improvement program (VSIP). General Technical Committee, General Motors Corporation. 3-7-83