Abstract
Distal airways disease causes significant morbidity yet remains insufficiently identified. We hypothesize that: (Citation) when spirometry is normal impulse oscillometry may provide information about mechanical properties of the distal airways in a manner comparable to dynamic compliance and (Citation) variation of breathing frequency will influence oscillometric measurements similar to effects of breathing frequency on dynamic compliance. Fifty-three symptomatic subjects with normal large airway function (spirometry) were studied; distal airway dysfunction was identified by presence of frequency dependence of compliance (FDC). Oscillometric parameters evaluated were resistance at 20 Hz (R20) and 5–20 Hz (R5 − 20), reactance at 5Hz (X5), and reactance area (AX). R20 correlated with airway resistance by esophageal manometry (r = 0.74, p< 0.001); X5 correlated with dynamic compliance at a respiratory rate of 60bpm (r = 0.61, p< 0.001); R5-20 and AX correlated with FDC (r = 0.48, p< 0.001; r = 0.53, p< 0.01). IOS indices were further evaluated at increased respiratory rate (RR40). Oscillometric parameters changed minimally at RR40 in normal subjects ΔR20 = 0.20 = 0.08 cmH2O/L/s, ΔR5-20 = 0.10 ± 0.03 cmH2O/L/s, Δ AX = 0.33 ± 0.19 cmH2O/L). However, in symptomatic subjects, while R20 increased minimallyat RR40 (Δ R20 = 0.37 ± 0.10 cmH2O/L/s), R5 − 20 and AX increased markedly (Δ R5 − 20 = 0.54 ± 0.06 cmH2O/L/s, Δ AX = 4.28 ± 0.67 cmH2O/L) and reversed post bronchodilator. IOS evaluates physiology of the distal airways in a manner comparable to dynamic compliance. Elevated respiratory rate influences oscillometric parameters and must be considered when interpreting oscillometric data. IOS provides a non-invasive tool for assessment of distal airway function when spirometry is normal, which can be applied to various clinical settings including early diagnosis of COPD (GOLD stage 0), asthma in clinical remission and occupational/ environmental irritant exposure.
INTRODUCTION
Distal airways disease may cause significant morbidity yet remains insufficiently identified. Distal airways pathology may be an early or sole manifestation of chronic lung diseases such as chronic obstructive pulmonary disease, bronchiectasis, and asthma. However, when airway abnormalities are localized only to the distal airways, clinical-pathologic correlation between patient illness and lung disease has been more difficult to establish (Citation[1]).
Airway function is traditionally evaluated by spirometry, which predominantly identifies abnormal resistance in large airways where flow is turbulent. In contrast, the distal airways have large aggregate cross-sectional area, such that flow velocity is much reduced and airflow is laminar (Citation[2]). Therefore, the distal airways contribute minimally to the total airway resistance and are largely ignored by spirometry leading to the term “silent zone of the lungs” (Citation[1]). Multiple tests to detect distal airways disease (single breath N2, partial flow volume loop and helium isoflow) have been developed which do not necessarily detect airway resistance per se, but likely depend on heterogeneity of airflow distribution.
Mathematical modeling suggests that abnormalities of airflow distribution can also be detected by analysis of frequency-dependent behavior of the respiratory system (Citation[3], Citation[4], Citation[5], Citation[6], Citation[7], Citation[8]). Oscillations of airflow may be induced either by volitional changes in respiratory rate or by externally applied pressure oscillations. Frequency dependence of compliance (FDC) is an established marker of distal airway dysfunction; however, its clinical application is limited by the need for esophageal manometry (Citation[9], Citation[10], Citation[11]). Impulse oscillometry is a non-invasive technique to assess distal airway function involving externally applied pressure oscillations. Interpretation of oscillometric data has been based on theoretical considerations similar to those used to interpret FDC (Citation[3], Citation[4], Citation[5], Citation[6], Citation[7], Citation[8]). Although the interpretation of both oscillometry and FDC is model based, FDC has been correlated with histological documentation of disease in the distal airways providing a rationale for comparison of these tests (Citation[9]).
The present study hypothesizes that, when spirometry is normal, impulse oscillometry will provide a non-invasive method to obtain information about the mechanical properties of the distal airways in a manner similar to dynamic compliance as measured by esophageal manometry. Furthermore, we hypothesize that respiratory rate will influence oscillometric measurements in a manner similar to the effects of respiratory rate on measurements of dynamic compliance. The clinical importance of these observations is that IOS may provide a non-invasive tool for assessment of distal airway function when spirometry is normal, which could be applied in various clinical settings including early diagnosis of COPD (prior to GOLD stage I), asthma in clinical remission and occupational/environmental irritant exposure.
METHODS
The present study retrospectively analyzed data obtained from 53 specially selected subjects with persistent respiratory symptoms (cough, dyspnea and or wheeze) despite normal spirometry and chest radiograph. Patients were referred from the Bellevue Hospital WTC Environmental Health Center, medicine, chest, asthma and occupational health clinics for evaluation of respiratory symptoms. Records were reviewed to determine symptoms, medical and smoking history and radiographic findings. Inclusion criteria were: normal spirometry, indicating normal large airway function and no evidence of parenchymal disease on radiographic evaluation.
Spirometry
Spirometry and lung volumes measured with body plethysmography were performed in accord with ATS/ERS recommendations (Citation[12]). Normal spirometry was defined as FEV1 and FVC >80% predicted and FEV1/FVC ≥ 77% (Citation[13]). This high value for FEV1/FVC was chosen to ensure that subjects with even mild large airway dysfunction were excluded from the study.
Lung compliance
All 53 subjects underwent esophageal manometry for measurement of lung compliance utilizing an esophageal balloon (Ackrad Laboratories; Cooper Surgical Company Trumbull CT) positioned in the distal third of the esophagus (Citation[14]). Static lung compliance (Cst,1) was measured after two inspiratory capacity maneuvers by periodically occluding the airway during exhalation from total lung capacity to functional residual capacity. Dynamic compliance (Cdyn,1) was determined at increasing respiratory frequencies and expressed as a ratio to the Cst,l (Citation[11]). Breath-by-breath data were analyzed to calculate Cdyn,1 and transpulmonary resistance (RL) by multiple linear regression of the equation of motion (Citation[14]). Constancy of Cdyn during successive breaths at each frequency was required and only trials with a regression coefficient >0.96 were considered for analysis (Citation[9], Citation[11]). Data included: lung resistance (RL), static compliance (Cst,1), dynamic compliance (Cdyn,1), and frequency dependence of compliance (FDC). FDC was defined as Cdyn,l/Cst,l < 0.8 at a respiratory rate of 60/min (RR60).
Impulse oscillometry
Impulse oscillometry (IOS) was measured with the Jaeger Impulse Oscillation System (Jaeger USA; Yorba Linda, CA) (Citation[15]). Measurements were performed with patients in the seated position during tidal breathing with a nose clip while firmly supporting the cheeks. Respiratory resistance and reactance were calculated by analyzing airflow and pressure oscillations between frequencies of 5 to 35 Hz. Parameters selected included: (1) resistance at an oscillation frequency of 20 Hz (R20); (2) reactance at 5 Hz (X5); (3) frequency dependence of resistance from 5 to 20 Hz (R5 − 20); and (4) The magnitude of low frequency reactance expressed as reactance area (AX). AX was calculated as the area above the reactance curve from 5 Hz to the resonant frequency. For the purpose of comparing IOS to compliance, the following assumptions were made based on the models of DuBois et al., Otis et al. and Mead (Citation[4], Citation[5], Citation[6]). R20 was assumed to reflect large airway resistance and therefore we examined its relationship to RL; X5 was assumed to reflect elastance and therefore we examined its relationship to Cst,1 and Cdyn,1; oscillation frequency dependent parameters (R5 − 20 and AX) were assumed to reflect abnormalities in peripheral airways and therefore we examined their relationship to FDC.
IOS was performed at baseline breathing (rate 12–22/min) and at an elevated respiratory rate of 40 breaths/minute (RR40). Only trials with constant tidal volumes and a coherence > 0.85 at oscillation frequencies of 10 Hz and higher were considered for analysis. Reproducibility between trials (variability <10%) was required for R5, X5 and AX at all respiratory rates. IOS was repeated following albuterol administration at both respiratory rates.
Data are presented as raw data and are compared to an upper limit of normal selected from prior publications. Although a single value for an upper limit of normal was selected for all parameters, these values represent a conservative estimate because they approximate 150% of previously published mean values in normal subjects (Citation[15], Citation[16], Citation[17], Citation[18], Citation[19], Citation[20]). An upper limit of normal for R20 (3.2 cmH2O/L/s) was chosen to approximate the upper limit of the 95% confidence interval. A conservative upper limit of normal for R5 − 20 was chosen at 0.76 cmH2O/L/s. For AX and X5 there are limited published data; conservative upper limits of normal of 3.6 cmH2O/L and -1.2 cmH2O/L/s, respectively were selected. Historic normative data from our laboratory obtained in 62 asymptomatic non-smoking subjects without lung disease, and in whom spirometry was normal, fell within these normal limits. Although these normal data were not obtained as part of a controlled study, these subjects had similar age, height and gender distribution to the subjects of the present study. Eighteen of these subjects also performed oscillometry at an elevated respiratory rate.
Statistical analysis
Data were summarized as means ± standard deviation (SD) or standard error (SE). Differences between groups were assessed utilizing a Student's t-test or the Mann-Whitney U-test. Correlation coefficients were derived from linear regressions except for the relationship between reactance and lung compliance which was assessed using a nonlinear power function regression since reactance is a function of respiratory elastance (1/C). Analyses were performed utilizing SPSS for Windows version 13.0. This study was approved by the institutional review boards of the NYU Medical School and Bellevue Hospital.
RESULTS
summarizes clinical characteristics and pulmonary function in the 53 subjects. In addition to dust exposure, potential etiologies for distal airway dysfunction included cigarette smoking in 18%, history of asthma in 11% and/or morbid obesity in 24%. Chest roentgenograms were normal in all subjects. By design, all subjects had normal spirometry. Lung volumes (n = 44) were within normal limits.
Table 1 Clinical characteristics; n = (53)
Static lung compliance was normal in all subjects (0.21 ± 0.01 l/cmH20). Cdyn,l/Cst,l decreased with increasing breathing frequency (0.83 ± 0.03 at RR20, 0.75 ± 0.02 at RR40 and 0.68 ± 0.02 at RR60), confirming the presence of FDC. Following bronchodilator administration, Cdyn,l/Cst,l, improved at all respiratory rates (p < 0.001 at RR40 and RR60).
illustrates that impulse oscillometry also demonstrated abnormalities in airway function despite normal spirometry. The top panel shows that airway resistance as assessed by R20 was elevated and responded to bronchodilator (3.47 ± 0.11 to 3.09 ± 0.13 cmH_2O/L/s; p < 0.001). The center panel shows that X_5 was abnormal and responded to bronchodilator (-1.37 ± 0.10 to -1.01 ± 0.07 cmH2O/L/s; p < 0.001). The bottom panel shows that frequency dependent parameters (R5-20 and AX), were also abnormal (0.90 ± 0.11 cmH2O/L/s and 6.32 ± 0.91 cmH2O/L, respectively) and responded to inhaled albuterol (0.68 ± 0.07 cmH_2O/L/s and 3.33 ± 0.47 cmH2O/L, p < 0.001, respectively).
Figure 1 Baseline impulse oscillometry parameters: R20 (top panel), X5 (center panel) and frequency dependent parameters: R5 − 20 and AX (bottom panel). The dotted line represents the published upper limit of normal for each parameter. Mean values ± SE are graphed pre (•) and post (◯) bronchodilator. Values were abnormal and returned toward normal following bronchodilator. *p < 0.001.
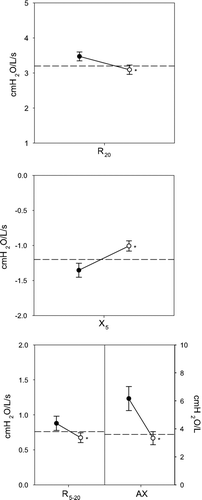
examines the relation between R20 obtained by oscillometry and RL calculated from esophageal manometry. A tight correlation between pre-bronchodilator values for R20 and RL was demonstrated (r = 0.74 p < 0.001). In contrast, R5 − 20 correlated weakly with RL (r = 0.36, data not shown).
Figure 2 The relationship between R20 obtained by oscillometry and RL calculated from esophageal manometry is illustrated. A tight correlation between R20 and RL was demonstrated. Regression line is plotted (r = 0.74; p < 0.001).
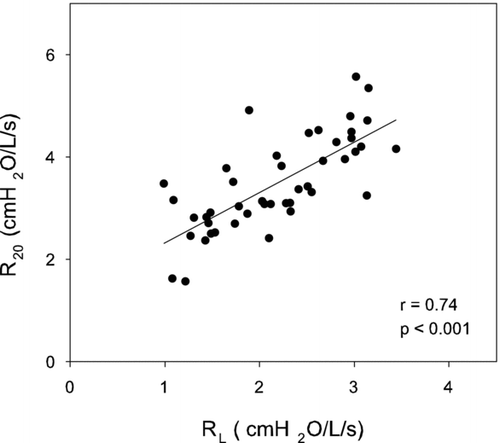
illustrates the comparison of X5 obtained by oscillometry to measurements of static and dynamic lung compliance obtained by esophageal manometry. The left panel demonstrates that X5 was poorly correlated with static lung compliance, (r = 0.40, p < 0.01). This relationship improved when X5 was related to Cdyn,l at progressively increasing respiratory rates. The correlation was maximized at RR60 (r = 0.61, p < 0.001, right panel).
Figure 3 X5 during baseline breathing is plotted for each subject as a function of Cst,l (left panel) and Cdyn,1 at RR60 (right panel). The relationship between X5 and lung compliance was assessed using a nonlinear inverse regression since reactance is a function of elastance (1/C). There is a statistically significant relationship between X5 and Cst,l, but with a large variance. The relationship is highly significant when X5 is related to Cdyn,1 at RR60.
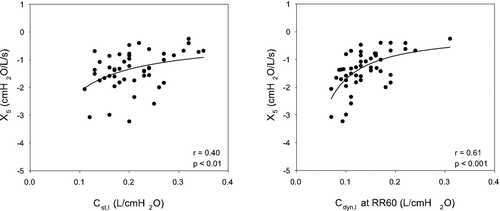
(top panel) plots frequency dependence of reactance expressed as AX as a function of Cdyn,l /Cst,l at a respiratory rate of 60 bpm. As Cdyn,l /Cst,l at RR60 decreased indicating worsening FDC, there was a corresponding increase in AX. The correlation between these parameters was statistically significant (r = 0.51, p < 0.001). Similarly, frequency dependence of resistance assessed as R5 − 20 was related to Cdyn,l /Cst,l at RR60 (r = 0.47, p < 0.001; not shown). The bottom panel shows that these two frequency dependent oscillometric parameters are tightly correlated (r = 0.92, p < 0.0001).
Figure 4 The top panel illustrates a tight correlation between AX and Cdyn,l/Cst,l at RR60 (power function regression). The bottom panel illustrates that the two frequency dependent oscillometric parameters (AX and R5 − 20) are tightly correlated in a linear fashion.
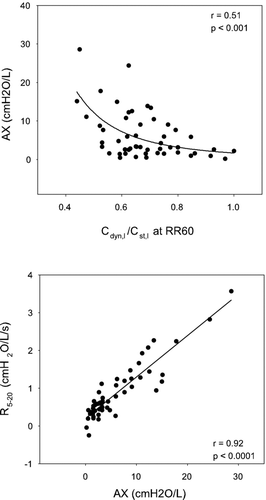
Oscillometric parameters at increased respiratory rate
illustrates the effects of increased respiratory rate on oscillometric parameters. The top panels illustrate data from 18 laboratory normal subjects in whom measurements at elevated respiratory rates were available. R20, R5 − 20 and AX were within normal limits at baseline and increased minimally at RR40 but did not exceed the upper limit of normal (Δ R20 = 0.20 ± 0.08 cmH2O/L/s, p < 0.05; Δ R5 − 20 = 0.10 ± 0.03 cmH2O/L/s, p < 0.01; and Δ AX = 0.33 ± 0.19 cmH2O/L, p = 0.10). The lower 3 panels illustrate similar data in the symptomatic subjects. The baseline mean values for R20, R5 − 20 and AX were elevated as shown previously (). At RR40, R20 increased minimally (Δ R20 = 0.37 ± 0.10 cmH2O/L/s, p < 0.001), similar to observations in normal subjects. Following bronchodilator administration, R20 remained at baseline values despite the increased respiratory rate. In contrast to observations in normal subjects, elevated breathing rates resulted in marked augmentation of frequency dependent parameters (Δ R5 − 20 = 0.54 ± 0.06 cmH2O/L/s, p < 0.001; Δ AX = 4.28 ± 0.67 cmH2O/L, p < 0.001). This enhancement was obliterated following bronchodilator administration in all subjects.
Figure 5 Effects of increased respiratory rate on oscillometric parameters are shown for 18 normal (top 3 panels) and 53 symptomatic subjects (lower 3 panels). Mean data ± SE are presented pre (•) and post (◯) bronchodilator. The dotted line represents the published upper limit of normal for each parameter. Increased respiratory rate had minimal effect on oscillometric parameters in normal subjects. In contrast, in the 53 symptomatic subjects R20 increased minimally, as in normal subjects, but frequency dependent parameters R5 − 20 and AX increased markedly. This enhancement was obliterated following bronchodilator administration despite elevated respiratory rate. Statistically significant increases at RR40 compared with baseline are illustrated as *p < 0.05.
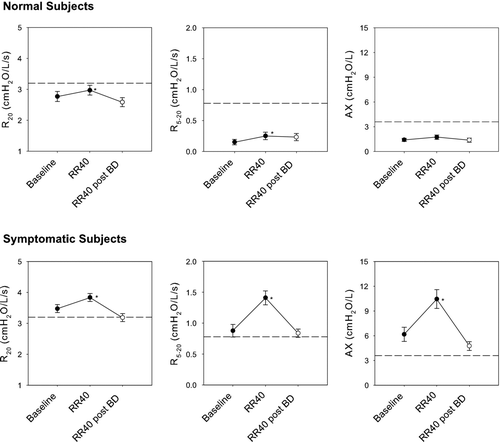
relates the change in IOS parameters at increased respiratory rate to presence or absence of FDC. The left panel illustrates that R20 increased minimally at increasing respiratory rates in normal and in symptomatic subjects without (n = 7) and with FDC (n = 46). The magnitude of this increase in R20 was similar in all 3 groups. The right panel illustrates similar data for frequency dependence of reactance (AX). While AX increased minimally at increased respiratory rate in both normal subjects and symptomatic subjects without FDC (0.33 ± 0.19 and 1.21 ± 0.40 cmH2O/L, respectively, p = ns), marked augmentation of AX was observed in the symptomatic subjects with FDC (4.66 ± 0.73 cmH2O/L, p < 0.001).
Figure 6 The change in R20 and AX at increased respiratory rate relative to baseline are shown for normal subjects (n = 18), subjects without FDC (n = 7) and with FDC (n = 46). R20 increased minimally and with a similar magnitude in all groups. Conversely, AX increased significantly in subjects with FDC compared with both normal subjects and subjects without FDC. * p < 0.05.
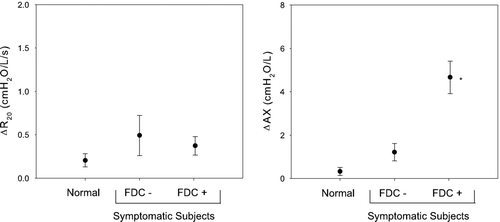
DISCUSSION
The present study assessed whether impulse oscillometry may provide a non-invasive alternative to direct measurements of lung compliance using esophageal manometry in symptomatic subjects with isolated distal airway dysfunction. Mathematical modeling based on initial studies of Otis et al. and Dubois et al. suggests that both tests may provide data that reflect flow resistive properties, respiratory elastance, and airflow distribution (Citation[5], Citation[6], Citation[7], Citation[8], Citation[16], Citation[21], Citation[22], Citation[23], Citation[24], Citation[25]). The data support that: (1) R20 reflects flow resistive properties as assessed by RL; (2) abnormalities of X5 reflect abnormalities of dynamic lung compliance; (3) frequency-dependent parameters (R5 − 20 and AX) are tightly linked to FDC. These frequency-dependent oscillometric parameters vary with increasing respiratory rate similar to the effects of respiratory rate on dynamic lung compliance.
Theoretically, the frequency spectrum of IOS permits evaluation of respiratory resistance from the upper to the distal airways (Citation[7], Citation[16], Citation[26], Citation[27], Citation[28]). This assumption has been tested by means of mathematical modeling and utilization of airway capsules and retrograde catheters (Citation[28], Citation[29]). These studies suggest that resistance at high oscillation frequencies (R20) predominantly reflects resistance in the proximal airways (Citation[30], Citation[31], Citation[32], Citation[33]). In the present study, resistance at 20 Hz was increased and tightly correlated to RL measured by esophageal manometry despite normal large airway function as evaluated by spirometry and plethysmographically measured airway resistance. Therefore, an elevated R20 may reflect either flow resistive properties in airways more peripheral than those evaluated by spirometry and plethysmography or conversely may represent a more sensitive test of large airway dysfunction.
The ability of IOS to evaluate elastic properties of the respiratory system was addressed by comparing oscillometric assessment of reactance to direct measurement of lung compliance. Modeling indicates that reactance varies as a function of oscillation frequency and is determined by the balance between inertive and elastic forces. At low oscillation frequencies, below resonant frequency, the influence of inertance is minimized and reactance is primarily a function of elastance (Citation[6], Citation[7]). Since lower oscillation frequencies may penetrate more peripherally into the tracheobronchial tree, X5 would theoretically reflect elastance of the distal airways (Citation[7], Citation[16], Citation[26], Citation[27], Citation[28], Citation[29], Citation[30], Citation[31], Citation[32], Citation[33]). Similarly, abnormalities of dynamic lung compliance induced by increasing respiratory rate reflect abnormalities of distal airway mechanics. In accord with these considerations, a tight correlation was seen between abnormalities of X5 and abnormalities of Cdyn,l at RR60, suggesting that oscillometry may be a useful clinical alternative for evaluation of dynamic lung compliance.
Prior studies have demonstrated that a common mechanism leading to both frequency dependence of resistance and FDC may be uneven distribution of airflow due to heterogeneity of distal airway mechanics (Citation[6], Citation[7], Citation[16], Citation[21], Citation[23], Citation[24], Citation[25], Citation[26], Citation[28], Citation[34]). Kjelgaard et al. and Van Den Elshout et al. demonstrated a relationship between frequency dependence of resistance and FDC (Citation[35], Citation[36]). However, these studies included subjects with large airway disease which could have influenced the distribution of airflow in the distal airways. In the present study, subjects with large airway disease were excluded, therefore the correlation between R5 − 20 and FDC indicates that isolated distal airway dysfunction is assessed similarly by both tests. In addition, the correlation between AX and FDC confirms that both oscillation frequency dependent parameters and breathing frequency dependent parameters assess distal airway dysfunction similarly. Although the link between both FDC and oscillation frequency dependent parameters to peripheral heterogeneity remains model based, FDC has been correlated with histologic documentation of disease in distal airways (Citation[9]).
The effect of varying respiratory rate on IOS parameters has not been previously evaluated. The present study assesses IOS at elevated respiratory rate as a method to increase heterogeneity of distribution of airflow in the distal airways. Increasing respiratory rate would increase the measured resistance in areas of turbulent flow (e.g., large airways). In contrast, airflow would remain laminar in distal airways due to the large aggregate cross-sectional area, and enhancement of abnormalities can be attributed to heterogeneous distribution of airflow. In normal subjects, increasing respiratory rate had minimal effect on all measured IOS parameters in accord with normal baseline resistance and homogenous distribution of airflow.
Conversely, in the symptomatic subjects there was a significant effect of respiratory rate on IOS parameters. Despite elevated R20 at baseline, the observed small increase seen at RR40 was similar to observations in normal subjects. In contrast, elevated breathing rate resulted in marked augmentation of frequency-dependent parameters (R5 − 20 and AX) which differed from the minimal effect observed in the normal subjects. This augmentation of R5 − 20 and AX was seen predominantly in the symptomatic subjects with FDC in accord with these oscillometric parameters reflecting heterogeneity of distal airway mechanics rather than resistance per se. Thus, increasing respiratory rate may enhance heterogeneity of peripheral airflow distribution in a manner similar to breathing frequency on dynamic compliance, thereby increasing sensitivity of IOS to detect peripheral airway abnormalities. In addition, these findings indicate that an influence of respiratory rate on IOS parameters must be considered in the clinical setting for determination of degree of abnormality and for evaluation of longitudinal data.
Assessment of IOS at RR40 requires justification because of potential interaction between breathing rate and oscillation frequency. A maximum respiratory rate of 40 (0.67 Hz) was chosen to minimize distortion of oscillometric data by tidal fluctuations in pressure and airflow. Furthermore, a respiratory rate of 40 is frequently observed in adult patients with respiratory disease and in pediatric populations where IOS is used clinically. An additional factor which may affect the results is alteration of chest wall mechanical properties at elevated respiratory rate with consequent distortion of IOS data. In the present study, the validity of IOS data obtained at increased respiratory rate is supported by demonstrating: (1) minimal effect of respiratory rate on oscillometric parameters in normal subjects, (2) enhancement of IOS abnormalities at elevated respiratory rate only in subjects where distal airway disease was present and confirmed by FDC and (3) obliteration of this enhancement following administration of bronchodilator.
The results of the present study have broad implications for the diagnosis of airway disease when standard tests are normal. This study evaluated distal airway function by 2 methods in a group of subjects with chronic respiratory symptoms despite normal large airway function. Oscillometric measurements were abnormal and tightly correlated with direct assessment of dynamic lung compliance. Since chest roentgenograms, spirometry and static compliance were normal in all subjects, the observed abnormalities of IOS and their correlation with dynamic compliance demonstrates the feasibility of IOS to detect disease at a stage when abnormalities are localized only to the distal airways. Both oscillometric and compliance data were reversible following bronchodilator administration indicating clinical relevance. Thus, this study demonstrates that IOS evaluates the physiologic properties of the distal airways in a manner comparable to FDC, and therefore may provide a non-invasive clinical tool which can be applied to various clinical settings including early diagnosis of COPD (GOLD stage 0), effects of cigarette smoking, asthma in clinical remission and occupational/environmental irritant exposure (Citation[15], Citation[35], Citation[37]).
Declaration of interest
The authors report no conflicts of interest. The authors alone are responsible for the content and writing of the paper.
Funding: NIEHS ES0260
REFERENCES
- Mead J. The lung's “quiet zone”. N Engl J Med 1970; 282: 1318–1319
- Pedley T J, Schroter R C, Sudlow M F. The prediction of pressure drop and variation of resistance within the human bronchial airways. Respir Physiol 1970; 9: 387–405
- Macklem P T. The physiology of small airways. Am J Respir Crit Care Med 1998; 157: S181–S183
- Mead J. Contribution of compliance of airways to frequency-dependent behavior of lungs. J Appl Physiol 1969; 26: 670–673
- Dubois A B, Brody A W, Lewis D H, Burgess B FJ. Oscillation mechanics of lungs and chest in man. J Appl Physiol 1956; 8: 587–594
- Otis A B, McKerrow C B, Bartlett R A, Mead J, McIlroy M B, Selverstone N J, Radford E P. Mechanical factors in distribution of pulmonary ventilation. J Appl Physiol 1956; 8: 427–443
- Peslin R, Fredberg J J. Oscillation mechanics of the respiratory system. Handbook of physiology. The respiratory system. Mechanics of Breathing, P Macklem, J Mead. American Physiological Society, Bethesda, MD 1986; 145–166, Chapter 11
- Lutchen K R, Saidel G M, Primiano F PJ, Horowitz J G, Deal E C, Jr. Mechanics and gas distribution in normal and obstructed lungs during tidal breathing. Am Rev Respir Dis 1984; 130: 974–979
- Woolcock A J, Vincent N J, Macklem P T. Frequency dependence of compliance as a test for obstruction in the small airways. J Clin Invest 1969; 48: 1097–1106
- Cosio M, Ghezzo H, Hogg J C, Corbin R, Loveland M, Dosman J, Macklem P T. The relations between structural changes in small airways and pulmonary-function tests. N Engl J Med 1978; 298: 1277–1281
- del Hoz R E, Berger K I, Klugh T T, Friedman-Jimenez G, Goldring R M. Frequency dependence of compliance in the evaluation of patients with unexplained respiratory symptoms. Respir Med 2000; 94: 221–227
- Miller M R, Hankinson J, Brusasco V, Burgos F, Casaburi R, Coates A, Crapo R, Enright P, van der Grinten C P, Gustafsson P, Jensen R, Johnson D C, MacIntyre N, McKay R, Navajas D, Pedersen O F, Pellegrino R, Viegi G, Wanger J ATS, Brusasco V, van der Grinten C PM. Standardisation of spirometry. Eur Respir J 2005; 26: 319–338
- Knudson R J, Slatin R C, Lebowitz M D, Burrows B. The maximal expiratory flow-volume curve. Normal standards, variability, and effects of age. Am Rev Respir Dis 1976; 113: 587–600
- Officer T M, Pellegrino R, Brusasco V, Rodarte J R. Measurement of pulmonary resistance and dynamic compliance with airway obstruction. J Appl Physiol 1998; 85: 1982–1988
- Oppenheimer B W, Goldring R M, Herberg M E, Hofer I S, Reyfman P A, Liautaud S, Rom W N, Reibman J, Berger K I. Distal airway function in symptomatic subjects with normal spirometry following World Trade Center dust exposure. Chest 2007; 132: 1275–1282
- Goldman M D, Saadeh C, Ross D. Clinical applications of forced oscillation to assess peripheral airway function. Respir Physiol Neurobiol 2005; 148: 179–194
- Niven A S, Backenson T J, Goldman M D, Hnatiuk O W, Weisman I M. Resistance measured by forced oscillation (IOS) is mouthpiece dependent and reduced in normal subjects using a free flow mouthpiece. Am J Respir Crit Care Med 2003; 167: A419
- Kohlhaufl M, Brand P, Scheuch G, Schulz H, Haussinger K, Heyder J. Impulse oscillometry in healthy nonsmokers and asymptomatic smokers: effects of bronchial challenge with methacholine. J Aerosol Med 2001; 14: 1–12
- Shiota S, Katoh M, Fujii M, Aoki S, Matsuoka R, Fukuchi Y, Shiota S, Katoh M, Fujii M, Aoki S, Matsuoka R, Fukuchi Y. Predictive equations and the reliability of the impulse oscillatory system in Japanese adult subjects. Respirology 2005; 10: 310–315
- Skloot G, Goldman M, Fischler D, Goldman C, Schechter C, Levin S, Teirstein A. Respiratory symptoms and physiologic assessment of ironworkers at the World Trade Center disaster site. Chest 2004; 125: 1248–1255
- Fredberg J J, Mead J. Impedance of intrathoracic airway models during low-frequency periodic flow. J Appl Physiol 1979; 47: 347–351
- Peslin R, Duvivier C, Gallina C. Total respiratory input and transfer impedances in humans. J Appl Physiol 1985; 59: 492–501
- Lutchen K R, Gillis H. Relationship between heterogeneous changes in airway morphometry and lung resistance and elastance. J Appl Physiol 1997; 83: 1192–1201
- Lutchen K R, Greenstein J L, Suki B. How inhomogeneities and airway walls affect frequency dependence and separation of airway and tissue properties. J Appl Physiol 1996; 80: 1696–1707
- Lutchen K R, Hantos Z, Petak F, Adamicza A, Suki B. Airway inhomogeneities contribute to apparent lung tissue mechanics during constriction. J Appl Physiol 1996; 80: 1841–1849
- Grimby G, Takishima T, Graham W, Macklem P, Mead J. Frequency dependence of flow resistance in patients with obstructive lung disease. J Clin Invest 1968; 47: 1455–1465
- Pimmel R L, Tsai M J, Winter D C, Bromberg P A. Estimating central and peripheral respiratory resistance. J Appl Physiol 1978; 45: 375–380
- Kappos A D, Rodarte J R, Lai-Fook S J. Frequency dependence and partitioning of respiratory impedance in dogs. J Appl Physiol 1981; 51: 621–629
- Macklem P T, Mead J. Resistance of central and peripheral airways measured by a retrograde catheter. J Appl Physiol 1967; 22: 395–401
- Petak F, Hantos Z, Adamicza A, Daroczy B. Partitioning of pulmonary impedance: modeling vs. alveolar capsule approach. J Appl Physiol 1993; 75: 513–521
- Lutchen K R, Suki B, Zhang Q, Petak F, Daroczy B, Hantos Z. Airway and tissue mechanics during physiological breathing and bronchoconstriction in dogs. J Appl Physiol 1994; 77: 373–385
- Suki B, Petak F, Adamicza A, Hantos Z, Lutchen K R. Partitioning of airway and lung tissue properties: comparison of in situ and open-chest conditions. J Appl Physiol. 1995; 79: 861–869
- Kaminsky D A, Irvin C G, Lundblad L, Moriya H T, Lang S, Allen J, Viola T, Lynn M, Bates J H, Kaminsky D A, Irvin C G, Lundblad L, Moriya H T, Lang S, Allen J, Viola T, Lynn M, Bates J HT. Oscillation mechanics of the human lung periphery in asthma. J Appl Physiol 2004; 97: 1849–1858
- Landser F J, Clement J, Van de Woestijne K P. Normal values of total respiratory resistance and reactance determined by forced oscillations: influence of smoking. Chest 1982; 81: 586–591
- Kjeldgaard J M, Hyde R W, Speers D M, Reichert W W. Frequency dependence of total respiratory resistance in early airway disease. Am Rev Respir Dis 1976; 114: 501–508
- van den Elshout F J, van Herwaarden C L, Folgering H T. Oscillatory respiratory impedance and lung tissue compliance. Respirat Med 1994; 88: 343–347
- Clement J, Landser F J, Van de Woestijne K P. Total resistance and reactance in patients with respiratory complaints with and without airways obstruction. Chest 1983; 83: 215–220