Abstract
We aimed to characterize gender differences in exercise endurance, operating lung volumes and symptoms limitation during exercise in patients with COPD.
Ninety-three women and 93 men with COPD matched for age and disease severity were evaluated during symptom-limited constant-work rate cycle exercise at 75% of peak capacity. Breathing pattern, inspiratory capacity, dyspnoea and leg discomfort Borg scores were recorded during exercise.
Endurance time was shorter in women compared to men. Inspiratory capacity decreased at a similar rate during exercise in women and men (0.71 vs. 0.81 ml· s− 1 for women and men respectively, p = 0.47) despite lower ventilation at end-exercise in women. At end-exercise, women showed lower inspiratory reserve volume (p < 0.005). Dyspnoea responses during exercise occurred with a steep rise near end-exercise, when inspiratory reserve volume approached a critical value, at 10% of total lung capacity, this onset of dyspnoea acceleration occurred earlier in women (p < 0.0001).
At the same relative exercise intensity, women with COPD had lower endurance time than men. Compared to men, women with COPD were disadvantaged during exercise as they reached a critical inspiratory reserve volume earlier, leading to a steep increase in dyspnoea and to exercise termination.
INTRODUCTION
Women appear to be particularly vulnerable to the adverse consequences of smoking on lung function. Women usually have worse lung function for a lesser tobacco exposure (Citation[1], Citation[2]), display more respiratory symptoms and dyspneoa (Citation[3]), have lower peak exercise tolerance (Citation[4], Citation[5]) and worse quality of life than men (Citation[5]). One study investigating gender difference in lung volume at rest showed that women with COPD exhibited more hyperinflation and gas trapping at rest than their men counterpart (Citation[6]).
Little is known about the exercise response in women compared to men with COPD. In the few studies that have addressed this issue, women tended to have similar (Citation[4], Citation[6]) or higher (Citation[7]) exercise tolerance as expressed by peak oxygen consumption (VO2)% of predicted values. The detrimental impact of dynamic hyperinflation on exercise tolerance has been highlighted by studies showing that inspiratory capacity (IC), a reflection of operating lung volumes, is strongly correlated with exercise tolerance (Citation[8]). To date, gender-based differences in the occurrence of dynamic hyperinflation in COPD has not been reported.
Based on the existing data suggesting that women with COPD may be more susceptible to show hyperinflation at rest than men (Citation[6]), we hypothesized that this situation could even worsen during exercise, further contributing to a reduction in exercise tolerance in women. Taking advantage of a large database of exercise tests (Citation[9], Citation[10]) we undertook this study to investigate and compare in women and men with COPD: 1) the tolerance to constant work rate cycling exercise, 2) ventilatory and operating lung volumes responses during constant work rate cycling exercise, 3) dyspnoea and leg discomfort responses during exercise and 4) the determinants of dyspnoea and tolerance to constant work rate cycling exercise.
METHODS
Study subjects and design
This study is a pooled analysis of the exercise data from two large multicentre clinical trials examining the effects of tiotropium on exercise tolerance (Citation[9], Citation[10]). The data presented here was obtained at baseline, when patients were weaned from their bronchodilators and before receiving any study medication. This eliminated any potential bias arising from a differential bronchodilator response between individuals. All subjects signed written informed consent at the time of their assessment and both original studies were approved by local ethics committees. One-hundred and twenty-four women and 335 men with COPD were initially included in these two studies. Original inclusion criteria were: age 40 to 75 years, cigarette smoking > 10 pack-years, forced expiratory volume (FEV1) ≤65% predicted, and functional residual capacity (FRC) measured by body plethysmography ≥120% predicted. Patients with a history of asthma, allergic rhinitis or atopy were not eligible for the trial. Moreover, patients with any recognized contraindication to clinical exercise testing and patients who had participated in a rehabilitation program for COPD within 6 weeks prior to the screening visit were not eligible.
No patients participated in both original studies. For the purpose of the present analysis, three IC data points or more (one at rest, one at submaximal exercise and one at end-exercise) during the constant work rate endurance test were necessary to examine changes in lung volume during exercise. Because IC maneuvres were performed at two-minute intervals, only patients with a constant work rate endurance time ≥4 minutes (93 women and 283 men) were considered in the patient selection process, representing, respectively, 75% and 84% of the women and men included in the original studies. Ninety-three men matching the age (within 5 years) and FEV1% predicted (within 5% predicted) of the 93 women were selected from the database, providing a 1 to 1 women to men ratio for this study. As a secondary analysis, the 93 women were also matched to 93 men for age (within 5 years) and FEV1 liters (within 100 ml).
Baseline lung function, lung volumes and incremental exercise testing were performed during an initial screening visit. Patients performed one constant work rate cycle exercise test (CET) on two separate occasions; results of the second test were reported herein.
Lung function evaluation
Spirometry and body plethysmography were performed according to the recognized standards (Citation[11],Citation12,Citation13). FEV1, forced vital capacity (FVC), FEV1/FVC, FRC, total lung capacity (TLC) and residual volume (RV) values were then compared to the predicted values of Quanjer et al. (Citation[13]). Predicted IC was calculated by subtracting the predicted values of FRC from the predicted value of TLC (Citation[14]). Maximal voluntary ventilation (MVV) was calculated by multiplying FEV1 by 35 (Citation[15]).
Exercise testing
The specific details of the incremental exercise test and the CET have previously been reported (Citation[16], Citation[17]). The incremental exercise test started with 3 min of unloaded pedaling after which work rate was set at 10 W with increments of 10 W/min until symptom limitation. Peak work capacity (Wpeak) was defined as the highest work rate maintained for 30s.
The CET started with 1 min of unloaded pedaling and workload was set at 75% of Wpeak. Patients were instructed to cycle until symptom limitation at a pedaling rate of 50–70 rpm. Ventilatory and gas exchange parameters were collected breath-by-breath during exercise using commercially available cardiopulmonary exercise testing equipment. Values were recorded at rest and during the third 30 s period of each 2 min interval during exercise. End-exercise values were recorded as the average of the last 30 s of exercise. At rest, during each 2 min interval during exercise and at the end of exercise, subjects rated the intensity of dyspnoea and leg discomfort using the modified Borg scale (Citation[18]) and then performed an IC manoeuvre. The IC was used to track changes in operating lung volumes during exercise as previously described (Citation[14]). Immediately after completing exercise, subjects were asked to identify the primary reason for stopping exercise.
Statistical analysis
Results are shown as mean ± SD unless otherwise specified. Baseline characteristics of women and men were compared using ANOVAs. Results are expressed as five time points: for each subject, time points in seconds corresponding to baseline, 25%, 50%, 75% and 100% of endurance time were used in the analysis. ANOVAs were used to compare women and men at the different time points. The rate of hyperinflation during the constant work rate cycle exercise test (CET) was calculated by dividing the fall in IC by CET time. Pearson correlations were used to correlate indexes of lung function and hyperinflation with endurance time and VO2 at the end of CET. Statistical significance was set at p < 0.05.
RESULTS
Patient characteristics
Anthropometric measurements, baseline lung function and incremental exercise test results are summarized in . Despite that women and men were well matched for age and FEV1% predicted value, women displayed less smoking history than men. When expressed in% predicted values, women had a higher TLC and FRC. There was no gender difference in IC (% predicted) but IC/TLC ratio was lower in women than men. Peak VO2 (ml· kg− 1· min− 1) and work rate (watt) were lower in women, but when expressed in% predicted, women had a greater peak VO2 and work rate than men.
Table 1 Baseline characteristics of patients.
Constant-work-rate exercise test
Women exercised for a shorter time (p < 0.0005) at a lower absolute workload (p < 0.0001) than men (). Heart rate (HR) at the end of CET was available for 61 women and 44 men. There was no significant gender difference in HR whether expressed in absolute (129 ± 20 and 127 ± 17 beats*min− 1, in women and men, respectively) or relative values (81 ± 12 and 80 ± 10% of predicted values, in women and men, respectively).
Figure 1 Constant-work rate endurance time and workload in women and men matched for FEV1% predicted values. Values are mean ± SD. Constant-work rate endurance time is in seconds (s) and work rate is in Watts (w). Women are shown as the white column and men as the black column.
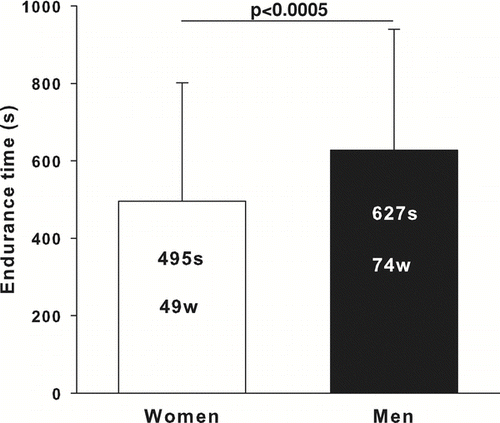
The time courses of VO2 and VE during constant work-rate exercise are depicted in . VO2 and VE were significantly higher in men throughout the CET (p < 0.0001) but showed a similar pattern between genders when expressed in relative units (as a fraction of peak VO2 during incremental exercise or maximal voluntary ventilation [MVV], respectively). VO2 at the end of CET expressed in% of VO2 at the end of incremental exercise was similar between genders (99 ± 5 and 97 ± 8%, in women and men, respectively). IC was significantly higher in men compared to women at all time points during the CET. SpO2 was not different between genders for the duration of the CET.
Figure 2 Time course of oxygen consumption (VO2, Panel A), VO2 during constant work rate exercise (CET)/peak VO2 during incremental exercise testing (Panel B), ventilation (VE, Panel B), VE/maximum voluntary ventilation (MVV) ratio (Panel C), inspiratory capacity (IC, Panel D), O2 pulse saturation (SpO2, Panel E) in relation to endurance time during CET in women and men matched for FEV1% pred. Values are mean ± SEM. * p < 0.0001, †p < 0.05. White circles represent women and black circles represent men.
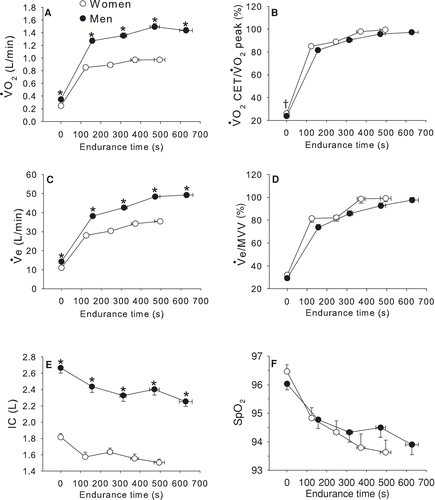
The operating lung volumes during exercise expressed in absolute and relative value are shown in . At peak exercise time, women showed a lower inspiratory reserve volume (IRV) (0.38 ± 0.26 vs. 0.52 ± 0.34 L, p < 0.005). When lung volumes were expressed in% of TLC, women and men exhibited similar changes in operational lung volumes (). The drop in IC was alinear with most of the fall occurring during the first two minutes of exercise. At the end of the CET, IC had decreased by 0.30 ± 0.30 L (15.7 ± 15.9% from baseline) and 0.40 ± 0.47 L (13.7 ± 21.3% from baseline) in women and men, respectively (p = 0.07). IC decreased at a same rate in both genders when expressed in absolute values (0.71 vs. 0.81 ml· s− 1 for women and men respectively, p = 0.47) and in% change from baseline (0.038 vs. 0.028%· s− 1 for women and men respectively, p = 0.17).
Figure 3 Operating lung volumes expressed in L (panel A) and in % of TLC (panel B) in relation to endurance time for women and men matched for FEV1% predicted values. Values are mean ± SEM. Definition of abbreviations: TLC: total lung capacity, IRV: inspiratory reserve volume, VT: tidal volume, IC: inspiratory capacity. White circles represent women and black circles represent men.
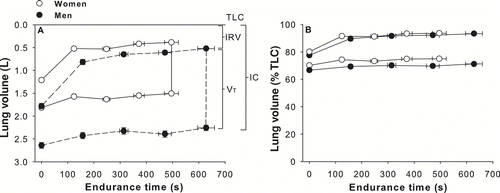
illustrates VE, tidal volume (VT), VT/IC, respiratory rate (RR), expiratory time and IRV% TLC in women and men in relation to VO2 during the CET. The increase in VE in relation to V˙O2 was similar among genders but was achieved differently: women showed significantly lower VT at all time points during the test (p < 0.0001) and higher respiratory rate at 75% (p < 0.05) and 100% of CET time (p < 0.05). Despite a more rapid and shallow breathing pattern, tidal volume in women represented a larger fraction of IC at 25% and 75% of CET. Expiratory time was significantly shorter in women at baseline and at 50% of CET. IRV% TLC was similar between genders.
Figure 4 Time course of ventilation (VE, panel A), tidal volume in L (VT, panel B) and as a fraction of inspiratory capacity (VT/IC, panel C), respiratory rate (RR, panel D), expiratory time (panel E), and inspiratory reserve volume (IRV) in% of total lung capacity (IRV/TLC, panel F) in relation to oxygen consumption (VO2) during the constant workrate endurance test in women and men matched for FEV1% predicted values. Values are mean ± SEM. Arrows indicate the time at which the threshold of dyspnoea acceleration occurred. * p < 0.0001, †p < 0.05, § p < 0.01. White circles represent women and black circles represent men.
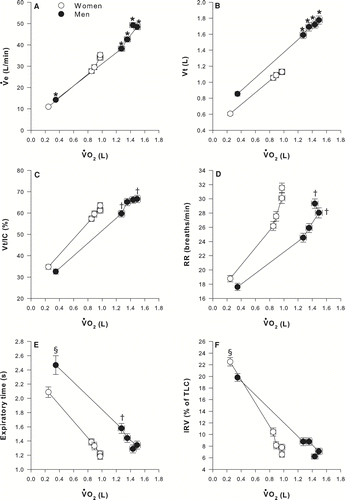
Determinants of dyspnoea and leg discomfort
The relationship between dyspnoea and leg discomfort, CET time, VE, VO2 and IRV are depicted in . Dyspneoa and leg discomfort Borg scores were higher in men compared to women at all time points during the CET except baseline. At the end of CET, Borg scores for dyspnoea (5.3 ± 2.1 vs. 6.5 ± 2, p < 0.0001) and leg discomfort (4.9 ± 2.4 vs. 6.0 ± 2.5, p < 0.05) were significantly lower in women (). Locus of symptom limitation was different between women and men (χ2 = 8.82, p < 0.05). Breathing discomfort was the main limiting factor in 39% and 57% of women and men, respectively, while the combination of dyspnoea with leg discomfort was the primary limiting symptom in 46% and 27% of women and men, respectively.
Figure 5 Time course of Borg dyspneoa and leg discomfort in relation to endurance time (panels A&B), dyspneoa in relation to ventilation (VE) in L/min (panel C) and in% of maximum voluntary ventilation (VE/MVV, panel D), dyspneoa and leg discomfort in relation to oxygen consumption (VO2, panels E&F) and dyspneoa in relation to inspiratory reserve volume (IRV) in L (panel G) and as a% of total lung capacity (IRV/TLC, panel H) in women and men matched for FEV1% pred. Values are mean ± SEM. Arrows indicate the time at which the threshold of dyspnoea acceleration occurred. White circles represent women and black circles represent men.
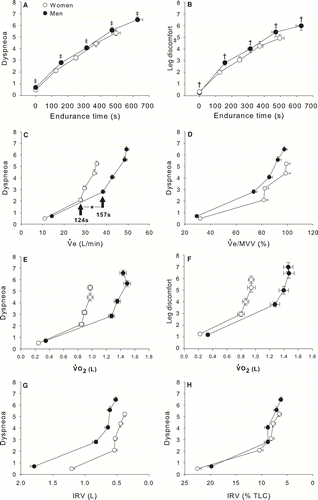
For a given VE, or VO2, women experienced more dyspnoea than men. This was particularly true at VE above 30 L/min or VO2 above 0.8 L. The increases in dyspnoea and leg discomfort Borg scores were alinear: toward the end of exercise, a threshold of dyspneoa and leg discomfort acceleration became evident in both genders. This threshold occurred earlier in women compared to men (p < 0.0001). There were striking similarities in the dyspnoea/VE% MVV and in the dyspnoea/IRV% TLC relationships between genders ( and ). In both genders, there was a steep rise in dyspnoea when VE/MVV ratio approached 80% and when IRV reached a critical limit, within 10% of TLC.
Determinants of endurance time and VO2 at end-exercise
Predictors of endurance time to CET and VO2 at the end of CET are shown in . VO2 at the end of CET and endurance time to CET time were correlated in women only. VO2 showed more and stronger correlations with lung function and hyperinflation indices than endurance time to CET in both genders. In women, only resting IRV% of TLC was significantly correlated with CET time.
Table 2 Correlates of endurance time and VO2 (% predicted) during cycle endurance test (CET).
Men and women matched for FEV1 in liters
shows baseline characteristics for the men matched with the 93 women for FEV1 in liters. Despite similar FEV1 (L), men had higher FVC (L), FRC (L), RV (L), TLC (L) and IC (L). Peak VO2 in ml· kg− 1· min− 1 was similar between groups, although women's peak VO2 exceeded that of men when expressed in% of predicted values. Men achieved higher peak work rate than women in watts, but women's were higher when predicted values were considered.
Table 3 Baseline characteristics of patients matched for FEV1 in liters.
shows analysis for women and men matched for FEV1 in liters. Women still show a reduced endurance time on the CET (panel A), along with a reduced VE (L/min, panel B), IC (L) and Vt (L) during the test (panel C). When volumes were plotted according to% of TLC, both genders behave similarly (panel D). At the end of the CET, IC had decreased by 0.30 ± 0.30 L (15.7 ± 15.9% from baseline) and 0.40 ± 0.46 L (16.4 ± 22.9% from baseline) in women and men, respectively (p = 0.1). IC decreased at a same rate in both genders when expressed in absolute values (0.71 vs. 0.91 ml· s− 1 for women and men respectively, p = 0.17) and in% change from baseline (0.038 vs. 0.039%· s− 1 for women and men respectively, p = 0.83). At all time points during the CET, dyspneoa was higher in men compared to women. The onset of dyspneoa acceleration occurred earlier in women (panel E). The dyspnoea relationships were practically identical in both genders, with a steep increase in dyspnoea occurring when IRV approached 10% of TLC.
Figure 6 Constant-work rate endurance time and workload (panel A), time course of ventilation (VE) in L/min (Panel B), operating lung volumes in L (panel C) and in% of TLC (panel D) along with Borg dyspnoea ratings in relation to ventilation (Panel E) and inspiratory reserve volume (panel F) are illustrated for women and men matched for FEV1 in liters. Values are mean ± SEM for panels C & D and mean ± SD for panels A, B, E & F. † p < 0.05, * p < 0.0001, ‡ p < 0.005, § p < 0.01. Women are shown in white and men in black.
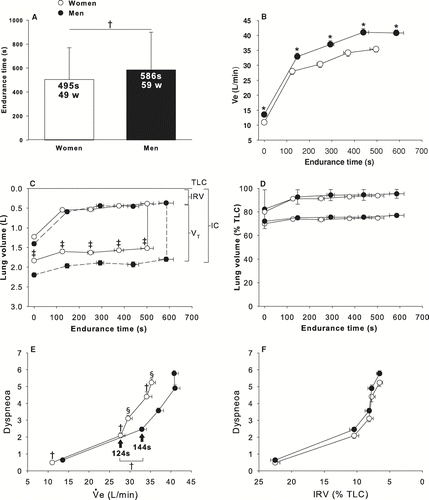
DISCUSSION
This is the first study to investigate gender differences in exercise tolerance and operating lung volumes in patients with COPD during a constant-workload exercise test. The main study findings can be summarized as followed: 1) at an identical relative workload and VO2 during the CET, women with COPD had lower exercise tolerance than men; 2) the rate of dynamic hyperinflation was similar between genders, despite lower ventilation in women; 3) given the similar rate of dynamic hyperinflation between genders and a lower IC in women, the expansion in VT and VE were rapidly constrained in women; 4) the dyspnoea and leg discomfort responses were clearly alinear with a steep rise in both symptoms toward the end of exercise; and 5) the threshold of dyspnoea acceleration occurred earlier in women and was tightly related to VE expressed as% MVV and IRV expressed as% TLC. These conclusions remained unchanged whether women and men were matched for FEV1 expressed in% predicted value or in liters.
Women were less overweight, had less smoking history but showed more lung hyperinflation than men. These results are consistent with previous reports suggesting that women may be more susceptible to the adverse effects of smoking and that the phenotypic expression of COPD varies between genders (Citation[2], Citation[4], Citation[6]). Women had lower exercise tolerance as expressed by lower weight-adjusted peak VO2. However, VO2 was higher in women when expressed in% of predicted values. Therefore, women in this study could be viewed as slightly less disabled based on the predicted values, although weight-adjusted peak VO2 may be more meaningful to characterize the ability to sustain the demand of daily life.
Gender differences in CET performance
The performance in CET in women compared to men can be viewed from two different perspectives. Because of smaller lungs, women have a reduced capacity to ventilate and are likely to become mechanically constrained earlier than men during exercise. When the exercise responses were normalized for lung size, as in and , women and men had matching responses. This would indicate that the general physiologic principle, that exercise is limited by exhausted ventilatory reserve and mechanical constraints to further expansion in tidal volume (as a result of dynamic hyperinflation), equally apply to women and men with COPD. From this perspective, we acknowledge that women behave similarly than men.
It is useful to look at the current exercise data from a clinical perspective. During daily living, individuals face the necessity of completing tasks that require the same absolute effort, irrespective of age, body size or gender. A shorter endurance time to CET in the present study indicates that women with COPD may have difficulties in the performance of activity of daily living that require endurance capacity. Furthermore, at VO2 above 0.8 L/min, women experienced more dyspnoea and leg discomfort, were utilizing a greater fraction of their IC to generate VT, and were breathing closer to TLC (). When faced with a given metabolic demand in the completion of activities of daily living, women are therefore clearly disadvantaged compared to men. These findings may help to explain why women with COPD are generally more dyspnoeic during daily activities compared to men (Citation[19]).
Why did women stop constant work rate cycling exercise before men? The threshold of dyspnoea acceleration occurred earlier in women () and was likely a key contributor of their exercise limitation. Resting IC (expressed in absolute value), which was already lower in women, was further compromised by the occurrence of dynamic hyperinflation at a similar rate than men. Because of a lower IRV, women were breathing closer to TLC than men at the end of CET. Breathing at elevated lung volumes allows higher expiratory flow rates, but it can also worsen dyspnoea by restricting VT expansion and by placing an additional load on the respiratory muscles, ultimately leading to neuromechanical uncoupling (Citation[20]).
The link between dyspnoea and breathing at volumes near TLC was nicely illustrated by the dyspnoea/IRV% TLC relationships, which were practically identical between genders (). The robust association between dyspneoa and IRV across studies (Citation[10], Citation[20]) suggests that breathing near TLC is an extremely potent dyspnoea-generating stimulus. Although the threshold of dyspnoea acceleration occurred at a very similar IRV in% TLC in both genders, women reached a critical IRV earlier than men (), leading to a steep rise in dyspnoea and to exercise termination.
From the patient's perspective, exercise limitation occurs when dyspnoea and leg discomfort become intolerable (Citation[21], Citation[22]); the perception of these symptoms is thus likely to influence the capacity to sustain a given exercise task. Lower dyspneoa and leg discomfort Borg scores at the end of CET in women compared to men suggest that women were less tolerant to the discomfort of exercise. Fit individuals usually reach higher symptom scores during exercise compared to unfit individuals less accustomed to the uncomfortable sensations of exercise (Citation[21]), a reduced tolerance to the sensations of exercise could be related to lower fitness in women.
In our study, women had a lower peak VO2 max, ml· kg− 1· min− 1 than men indicating that they would be less likely to undergo and endure intense physical exercise. In consequence, their exposure and tolerance to severe dyspnoea and/or leg discomfort were likely reduced. In fact, for a similar VO2 in L/min, Borg dyspnoea scores were higher in women than men (), further supporting the fact that women might be less inclined to engage in intense daily activities than men. The current data do not allow a definitive conclusion on this issue and comparative assessment of activity of daily living in women versus men with COPD would be of interest.
Dynamic hyperinflation during constant work-rate cycling exercise
Three factors govern the magnitude of dynamic hyperinflation in a given individual: VE (or VT); expiratory time; and the time constant of the respiratory system (Citation[23]). The rate of hyperinflation was similar between women and men despite a much lower VE in women. Shortened expiratory time in women () was certainly a factor that contributed to the similar rate of dynamic hyperinflation between genders. We cannot rule out that a longer time constant of the respiratory system in women also contributed to the similar rate of dynamic hyperinflation between genders, despite different VE. A longer time constant in women could result from increased airway resistance due to smaller airway caliber (Citation[2], Citation[24]) than in men. Greater lung compliance could also increase the susceptibility to dynamic hyperinflation in women. A substudy of the NETT trial reported less parenchymal abnormalities in emphysematous women compared to men, suggesting that for a given degree of airflow limitation, emphysema may be less severe in women (Citation[2]). However, hyperinflation and/or air trapping were inclusion criteria for this trial (Citation[25]) thus minimizing any potential existing gender difference in the susceptibility to hyperinflation and gas trapping.
POTENTIAL LIMITATIONS
We acknowledge the retrospective nature of this study. However, using unique sets of exercise data, we were able to evaluate exercise physiology in a large cohort of women and men with COPD matched for age and FEV1% predicted value. We are confident about the validity of this observation emerging from a large sample of men and women with COPD whose exercise testing was carefully conducted in experienced clinical centers. The fact that similar findings were observed when women were compared to men matched for FEV1 in% predicted value or in liters support the robustness of the study's conclusions.
The duration of a constant work rate exercise is intimately linked to its intensity according to the power-duration relationship (Citation[26]).
Because women reached a higher% predicted peak work rate during incremental exercise compared to men, they also exercised at a higher% predicted workload during CET. In other words, women might have been exercising in a different zone of the power-duration relationship. This appears unlikely because both genders were exercising at the same relative workload (75% Wpeak) and VO2 as a fraction of peak VO2 reached during incremental exercise was similar between genders throughout the CET (). Given the potential pitfalls and imprecision of the equations used to predict peak exercise work rate (Citation[27]), we submit that it should be more accurate to describe exercise intensity based on measured peak VO2 values than on work rate expressed in% predicted value. The fact that both the dyspnoea/exercise duration () and the leg discomfort/exercise duration () relationship reported in women and men were similar also suggests that both genders were exercising at the same relative intensity.
CONCLUSIONS
The present study demonstrates a reduced tolerance to CET in women compared to men with COPD, despite being matched for age and FEV1% predicted. This lower exercise tolerance could be explained by an earlier threshold of dyspnoea acceleration associated with breathing at high lung volume, near TLC. Our data also suggest a lower tolerance to dyspnoea and leg discomfort in women with COPD. Despite lower absolute ventilation, women had a similar rate of hyperinflation to men, suggestive of a higher susceptibility to dynamic hyperinflation. This study underlines differences in the expression of COPD across genders and suggests that appropriate considerations should be given to the more severe functional limitations in women.
Declaration of Interests
L. Laviolette is a parttime consultant for Boehringher-Ingelheim Canada. DE O'Donnell has received research funding support from BI/Pfizer and has served on speaker's bureaus, consultation panels and advisory boards for BI/Pfizer. K. Webb has no conflicts of interest to report. AL Hamilton is an employee of Boehringer-Ingelheim Canada. S. Kesten is an employee of Boehringher-Ingelheim. FM received fees for speaking at conferences sponsored by Boehringer Ingelheim, Pfizer and GlaxoSmithKline. He served on an advisory board for GlaxoSmithKline and Altana Pharma. He has received research funding from GlaxoSmithKline, Boehringer Ingelheim, Altana Pharma and Merck. The authors alone are responsible for the content and writing of the paper.
ACKNOWLEDGMENTS
We wish to acknowledge the contributions of each investigator and study team in each participating centres of the two multicentre clinical trials that have evaluated the impact of tiotropium on exercise tolerance in patients with COPD. We would also like to thank Inge Leimer and Gaétan Daigle for their statistical assistance.
Financial support: This study was supported by Boehringer Ingelheim Pharma GmbH & Co. KG, Ingelheim, Germany and Pfizer, Inc., New York, NY. François Maltais is a research scholar of the Fonds de la Recherche en Santé du Québec.
REFERENCES
- Prescott E, Bjerg A M, Andersen P K, Lange P, Vestbo J. Gender difference in smoking effects on lung function and risk of hospitalization for COPD: results from a Danish longitudinal population study. Eur Respir J 1997; 10(4)822–827
- Martinez F J, Curtis J L, Sciurba F, Mumford J, Giardino N D, Weinmann G, Kazerooni E, Murray S, Criner G J, Sin D D, Hogg J, Ries A L, Han M, Fishman A P, Make B, Hoffman E A, Mohsenifar Z, Wise R. Sex differences in severe pulmonary emphysema. Am J Respir Crit Care Med 2007; 176(3)243–252
- Langhammer A, Johnsen R, Gulsvik A, Holmen T L, Bjermer L. Sex differences in lung vulnerability to tobacco smoking. Eur Respir J 2003; 21(6)1017–1023
- Chatila W M, Wynkoop W A, Vance G, Criner G J. Smoking patterns in African Americans and whites with advanced COPD. Chest 2004; 125(1)15–21
- de Torres J P, Campo A, Casanova C, Guirre-Jaime A, Zulueta J. Gender and chronic obstructive pulmonary disease in high-risk smokers. Respiration 2006; 73(3)306–310
- Laviolette L, Lacasse Y, Doucet M, Lacasse M, Marquis K, Saey D, Le Blanc P, Maltais F. Chronic obstructive pulmonary disease in women. Can Respir J 2007; 14(2)93–98
- Carter R, Holiday D B, Stocks J, Grothues C, Tiep B. Predicting oxygen uptake for men and women with moderate to severe chronic obstructive pulmonary disease. Arch Phys Med Rehabil 2003; 84(8)1158–1164
- O'Donnell D E, Revill S M, Webb K A. Dynamic hyperinflation and exercise intolerance in chronic obstructive pulmonary disease. Am J Respir Crit Care Med 2001; 164(5)770–777
- Maltais F, Hamilton A, Marciniuk D, Hernandez P, Sciurba F C, Richter K, Kesten S, O'Donnell D. Improvements in symptom-limited exercise performance over 8 h with once-daily tiotropium in patients with COPD. Chest 2005; 128(3)1168–1178
- O'Donnell D E, Fluge T, Gerken F, Hamilton A, Webb K, Aguilaniu B, Make B, Magnussen H. Effects of tiotropium on lung hyperinflation, dyspnoea and exercise tolerance in COPD. Eur Respir J 2004; 23(6)832–840
- American Thoracic Society. Standards for the diagnosis and care of patients with chronic obstructive pulmonary disease. Am J Respir Crit Care Med 1995; 152(5)S77–121
- Coates A L, Peslin R, Rodenstein D, Stocks J. Measurement of lung volumes by plethysmography. Eur Respir J 1997; 10(6)1415–1427
- Quanjer P H, Tammeling G J, Cotes J E, Pedersen O F, Peslin R, Yernault J C. Lung volumes and forced ventilatory flows. Report Working Party Standardization of Lung Function Tests, European Community for Steel and Coal. Official Statement of the European Respiratory Society. Eur Respir J Suppl 1993; 16: 5–40
- O'Donnell D E, Lam M, Webb K A. Measurement of symptoms, lung hyperinflation, and endurance during exercise in chronic obstructive pulmonary disease. Am J Respir Crit Care Med 1998; 158(5)1557–1565
- Spiering B A, Judelson D A, Rundell K W. An evaluation of standardizing target ventilation for eucapnic voluntary hyperventilation using FEV1. J Asthma 2004; 41(7)745–749
- O'Donnell D E, Webb K A. Exertional breathlessness in patients with chronic airflow limitation. The role of lung hyperinflation. Am Rev Respir Dis 1993; 148(5)1351–1357
- O'Donnell D E, Lam M, Webb K A. Spirometric correlates of improvement in exercise performance after anticholinergic therapy in chronic obstructive pulmonary disease. Am J Respir Crit Care Med 1999; 160(2)542–549
- Borg G A. Psychophysical bases of perceived exertion. Med Sci Sports Exerc 1982; 14(5)377–381
- de Torres J P, Casanova C, Hernandez C, Abreu J, Guirre-Jaime A, Celli B R. Gender and COPD in patients attending a pulmonary clinic. Chest 2005; 128(4)2012–2016
- O'Donnell D E, Hamilton A L, Webb K A. Sensory-mechanical relationships during high-intensity, constant-work-rate exercise in COPD. J Appl Physiol 2006; 101(4)1025–1035
- Hamilton A L, Killian K J, Summers E, Jones N L. Muscle strength, symptom intensity, and exercise capacity in patients with cardiorespiratory disorders. Am J Respir Crit Care Med 1995; 152(6)2021–2031
- Killian K J, Le Blanc P, Martin D H, Summers E, Jones N L, Campbell E J. Exercise capacity and ventilatory, circulatory, and symptom limitation in patients with chronic airflow limitation. Am Rev Respir Dis 1992; 146(4)935–940
- Bancalari E, Clausen J. Pathophysiology of changes in absolute lung volumes. Eur Respir J 1998; 12(1)248–258
- Brooks L J, Byard P J, Helms R C, Fouke J M, Strohl K P. Relationship between lung volume and tracheal area as assessed by acoustic reflection. J Appl Physiol 1988; 64(3)1050–1054
- Fishman A, Martinez F, Naunheim K, Piantadosi S, Wise R, Ries A, Weinmann G, Wood D E. A randomized trial comparing lung-volume-reduction surgery with medical therapy for severe emphysema. N Engl J Med 2003; 348(21)2059–2073
- Neder J A, Jones P W, Nery L E, Whipp B J. Determinants of the exercise endurance capacity in patients with chronic obstructive pulmonary disease. The power-duration relationship. Am J Respir Crit Care Med 2000; 162(2)497–504
- ATS/ACCP. Statement on Cardiopulmonary Exercise Testing. Am J Respir Crit Care Med 2003; 167(2)211–277