ABSTRACT
Systolic heart failure is a common and disabling co-morbidity of chronic obstructive pulmonary disease (COPD) which may increase exercise ventilation due to heightened neural drive and/or impaired pulmonary gas exchange efficiency. The influence of heart failure on exercise ventilation, however, remains poorly characterized in COPD. In a prospective study, 98 patients with moderate to very severe COPD [41 with coexisting heart failure; ‘overlap’ (left ventricular ejection fraction < 50%)] underwent an incremental cardiopulmonary exercise test (CPET). Compared to COPD, overlap had lower peak exercise capacity despite higher FEV1. Overlap showed lower operating lung volumes, greater ventilatory inefficiency and larger decrements in end-tidal CO2 (PETCO2) (P < 0.05). These results were consistent with those found in FEV1-matched patients. Larger areas under receiver operating characteristic curves to discriminate overlap from COPD were found for ventilation (E)-CO2 output
CO2) intercept,
E-
CO2 slope, peak
E/
CO2 ratio and peak PETCO2. Multiple logistic regression analysis revealed that
CO2 intercept ≤ 3.5 L/minute [odds ratios (95% CI) = 7.69 (2.61–22.65), P < 0.001] plus
E-
CO2 slope ≥ 34 [2.18 (0.73–6.50), P = 0.14] or peak
E/
CO2 ratio ≥ 37 [5.35 (1.96–14.59), P = 0.001] plus peak PETCO2 ≤ 31 mmHg [5.73 (1.42–23.15), P = 0.01] were indicative of overlapping. Heart failure increases the ventilatory response to metabolic demand in COPD. Variables reflecting excessive ventilation might prove useful to assist clinical interpretation of CPET responses in COPD patients presenting heart failure as co-morbidity.
Introduction
Exercise intolerance is characteristically multi-factorial in chronic obstructive pulmonary disease (COPD) Citation(1). However, mechanical ventilatory constraints assume a more prominent role as disease progresses as suggested, for instance, by a low sub-maximal ventilation (E) to CO2 output (
CO2) slope, a high
E–
CO2 intercept and increased end-exercise end-tidal pressure for CO2 (PETCO2) (Citation2–6).
Systolic heart failure, in particular, is a common and disabling co-morbidity of COPD (hereafter termed ‘overlap’) (Citation7,8) with a potential to influence on these key exercise responses. In fact, heart failure may increase exercise ventilation in COPD due to heightened neural drive and/or poor pulmonary gas exchange efficiency (Citation9,10). Thus, COPD patients with coexisting heart failure may present with increased E–
CO2 slope and a low
E–
CO2 intercept (Citation2,11,12). End-tidal pressure for CO2 (PETCO2) might be lower in overlap secondary to alveolar hyperventilation (i.e., low ‘mean’ alveolar PCO2) and/or increased ventilation of poorly perfused alveoli (Citation9,13). Unfortunately, the few retrospective studies which looked at the influence of heart failure on ventilatory responses to exercise in COPD compared COPD versus heart failure Citation(2) or heart failure versus overlap (Citation11,12), i.e., they did not contrast COPD patients with and without heart failure. Moreover, those studies did not control for heterogeneities in disease diagnosis, treatment and clinical stability at the time of testing (Citation2,11,12). The influence of heart failure on exercise ventilation in COPD, therefore, remains to be adequately characterized in a prospective study involving stable patients under standardized treatment for both COPD and heart failure.
We therefore set such a study in which we systematically investigated the influence of heart failure on cardiopulmonary exercise test (CPET) responses reflecting excessive ventilation and/or impaired gas exchange efficiency in COPD (Citation2–6). We reasoned that if those abnormalities were more frequently found in overlap than COPD, they might eventually prove useful to assess the contribution of heart failure to exercise intolerance, to determine the effects of interventions aimed to improve ventilatory efficiency (e.g., sildenafil, whole-body and inspiratory muscle training) (Citation14–16), and to assist on risk stratification [as excessive ventilation is a negative prognostic marker in both heart failure Citation(17) and COPD Citation(18)].
Methods
Subjects
One-hundred and fifty-three patients were consecutively referred for cardiopulmonary assessment jointly performed by the same respirologists and cardiologists in 3 outpatient clinics dedicated to the care of COPD and/or heart failure (Brazil and Canada) from 01 April 2012 to 30 November 2014. Disease treatment was optimized carefully before study entry and patients underwent CPET only after an agreement had been reached between respirologists and cardiologists regarding disease stability. As outlined in , 98 sedentary males with COPD [post-bronchodilator FEV1/forced vital capacity (FVC) ratio < 0.70] and a previous history of cigarette smoking (at least 10 pack-years) underwent CPET. Forty-one patients presenting with echocardiographic left ventricular ejection fraction ≤ 45% in combination with symptoms indicative of heart failure (such as orthopnoea, paroxysmal nocturnal dyspnoea, fatigue, peripheral oedema, nocturia more than twice a night, or any combination of these symptoms) comprised the overlap group Citation(19). Regarding heart failure treatment, 24 patients were on the maximal recommended doses of carvedilol (50 mg/day if weight <85 kg and 100 mg/day if weight >85 kg) with the remaining patients receiving at least 25 mg/day. Daily mode doses for the most frequently used medications in each class were: captopril (150 mg), losartan (125 mg), spironolactone (50 mg) and furosemide (120 mg). Participants were asked to report chronic dyspnoea [modified (Citation1–5) Medical Research Council (MRC) scale score 2–4] and intolerance to physical exertion (NYHA class 2 or 3). Main exclusion criteria included: long-term O2 therapy, recent (within a year) rehabilitation, osteomuscular limitation to cycling, type I or non-controlled type II diabetes mellitus, and peripheral arterial disease associated with claudication. All subjects gave written informed consent and the study was approved by the appropriate Medical Ethics Committee (Sao Paulo Hospital, Hospital de Clinicas of Porto Alegre and Kingston General Hospital).
Measurements
Lung function
Spirometry, transfer factor and static lung volumes were evaluated (1085 ELITE D™, Medical Graphics). Resting blood gases were obtained from samples from the radial artery.
Cardiopulmonary exercise testing
Patients performed a symptom-limited incremental CPET on an electronically braked cycle ergometer using the Vmax229d System (SensorMedics). The rate of work rate increment was individually selected according to reported exercise tolerance (typically 5–10 W). Oxygen uptake (O2, L/minute), carbon dioxide output (
CO2, L/minute), respiratory exchange ratio (RER,
CO2/
O2), minute ventilation (
E, L/minute), end-tidal partial pressure for carbon dioxide (PETCO2, mmHg), tidal volume (VT, L) and breathing frequency (f, cycles/minute) were averaged at 20-second intervals. Peak
E was also expressed relative to the estimated maximal voluntary ventilation [MVV (L/minute) = FEV1 × 35].
E/
CO2 nadir was the lowest test data point. Slope and intercept of the linear
E–
CO2 relationship were determined by linear regression (Citation5,6). Serial inspiratory capacity (IC, L) manoeuvres tracked the operating lung volumes Citation(1). Lactate threshold was estimated by the gas exchange method (V-slope). Arterial oxygen saturation was measured non-invasively by pulse oximetry (SpO2, %). Breathlessness and leg effort scores were rated according to the 10-point Borg category ratio scale.
Statistical analysis
Values are reported as mean ± standard deviation (SD) unless otherwise specified (IBM® SPPS® Statistics version 22.0.0.0). Overlap and COPD patients were contrasted by non-paired t, Mann–Whitney test or a χ2 test for differences in proportions. Receiver operating characteristic (ROC) curve analyses selected the optimal threshold values to differentiate overlap from COPD based on CPET responses. Univariate and multivariate binary logistic regression analyses evaluated the discriminative value of CPET variables using ‘overlap’ as the outcome. Results were expressed as odds ratios with the corresponding 95% confidence intervals.
Results
Subject resting characteristics
Subjects were well matched for general characteristics, including age and body mass index (P > 0.05; ). Overlap patients, however, reported more dyspnoea (mMRC scores) and physical limitation (NYHA scores) on daily life. As previously reported (Citation7,8), there was a greater burden of co-morbidities in overlap compared to COPD. Treatment for COPD did not differ between the groups. COPD patients showed a typical obstructive ventilatory defect with concomitant air trapping and lung hyperinflation, i.e., reduced FEV1 and FEV1/FVC with increased residual volume (RV) and RV/total lung capacity (TLC). As expected from coexisting heart failure, those abnormalities were lessened in overlap due to a restrictive component (as suggested by reduced FVC and preserved TLC) (P < 0.05). Resting IC, however, did not differ between the groups due to greater reductions in expiratory reserve volume in overlap (data not shown). Despite lower transfer factor values, overlap patients had better arterial oxygenation and lower PaCO2 than COPD. Similar results were found in FEV1-matched COPD and overlap patients ().
Table 1. Subject resting characteristics.
Metabolic and cardiovascular responses to exercise
Peak work rate and peak O2 were significantly lower in overlap compared to COPD. Moreover, overlap showed considerable evidence of impaired submaximal aerobic metabolism as suggested by shallower
O2–work rate slope and lower estimated lactate threshold (i.e., lower gas exchange threshold, P > 0.05; ). As expected from pharmacological β-blocking (), overlap patients showed significantly lower peak heart rates. Similar results were found contrasting FEV1-matched COPD and overlap patients ().
Table 2. Physiological and sensory responses to incremental CPET.
Ventilatory and gas exchange responses to exercise
Compared to COPD, overlap patients had greater peak ventilatory reserve (lower peak E/MVV ratio) despite greater ventilatory inefficiency (higher peak
E/
CO2 ratio and
E-
CO2 slope) (). In addition, lower
E-
CO2 intercept was found in overlap when considering both the whole group and FEV1-matched sub-groups (). Overlap also showed smaller decrements in IC from rest to peak exercise, i.e., exercise-induced dynamic hyperinflation. These findings were associated with larger decrements in peak PETCO2 and attenuated exercise-induced O2 desaturation (). As anticipated, dyspnoea was the main exercise-limiting symptom in COPD. Conversely, leg effort ratings were higher than dyspnoea scores at exercise termination in overlap. Similar results were found when contrasting FEV1-matched COPD and overlap patients ().
shows the comparative analysis among ROC curves with higher AUCs to discriminate overlap from COPD (E–
CO2 slope and intercept, peak
E/
CO2 ratio and peak PETCO2). Based on the suggested optimal thresholds for these and other key physiological variables, odds ratios for overlapping prediction were calculated by univariate logistic regression analysis. As shown in , particularly high odds ratios were found for
E-
CO2 intercept ≤3.5 L/minute,
E-
CO2 slope ≥ 34, peak
E/
CO2 ratio ≥ 37 and peak PETCO2 ≤ 31 mmHg (P < 0.0001). Multivariate logistic regression models for overlapping prediction were then obtained for variables derived from the linear
E-
CO2 plot (i.e., slope and intercept) (‘model 1’, ) or discrete end-exercise variables (
E/
CO2 ratio and PETCO2) (‘model 2’, ). Attenuated O2 desaturation (peak SpO2 ≤ 89%) did not significantly add predictive value to those variables (P > 0.05). For instance, (left panels) shows that only 8/50 (16%) subjects with
E-
CO2 intercept > 3.5 L/minute and
E-
CO2 slope < 34 were overlap patients. Moreover, whereas 7/41 patients (17%) with peak
E/
CO2 < 37 and peak PETCO2 ≥ 31 mmHg were overlap, 16/19 (84.3%) subjects with higher peak
E/
CO2 and lower PETCO2 had overlap (P < 0.001). Matching patients by FEV1 produced similar results ().
Figure 2. Receiver operating characteristic (ROC) curve analysis to discriminate overlap from COPD. Circles depict the optimal thresholds according to individual variables. *P < 0.05. †P < 0.05 versus peak PETCO2. E, ventilation;
CO2, carbon dioxide output; PET, end-tidal partial pressure.
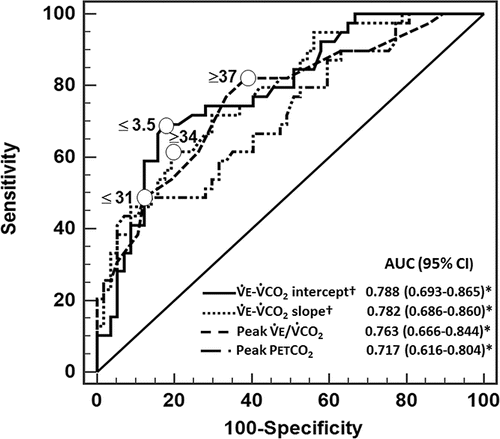
Table 3. Univariate and multivariate logistic regression modelling for overlap prediction
Discussion
This is the first study to prospectively investigate the influence of heart failure with reduced left ventricular ejection fraction on exercise ventilation in COPD. Results indicate that lower maximal exercise capacity in COPD-heart failure overlap was associated with attenuated exercise-induced dynamic hyperinflation, greater ventilatory inefficiency and larger decrements in peak PETCO2. A low E-
CO2 intercept (≤3.5 L/minute) coupled with a high
E-
CO2 slope (≥34) or a high peak
E/
CO2 ratio (≥37) plus a low peak PETCO2 (≤31 mmHg) were more frequently found in overlap than COPD even when between-group differences in FEV1 were taken into consideration. Thus, CPET responses reflecting a heightened ventilatory response to metabolic demand might prove useful in different clinical scenarios involving overlap patients, e.g., to assess the contribution of heart failure to exercise intolerance, to determine the effects of interventions and to assist in prognostic stratification.
There is growing recognition that identification and treatment of co-morbidities are highly relevant to clinical management of COPD. Heart failure in COPD (‘overlap’), in particular, has been associated with increased morbidity, poor quality of life and greater utilization of health care resources. Moreover, overlap frequently compounds with other systemic co-morbidities () thus contributing to poor prognosis (Citation7,8,20). Considering that exercise intolerance is the hallmark of both COPD and heart failure Citation(21), exercise-based evaluations such as CPET are likely to provide relevant information for clinical management of overlap patients.
In this context, the most consistent abnormality found in overlap was an excessive ventilatory response to metabolic demand, expressed as relatively higher E-
CO2 slope, lower
E-
CO2 intercept or higher peak
E/
CO2 ratio (; and ). Physiological dead space (i.e., dead space/VT ratio) and neural ventilatory drive, the main determinants of exercise ventilation Citation(22), are typically increased in patients with COPD or heart failure (Citation3–6,9–11). Increased physiological dead space in these patients might be due to elevated dead space (e.g., augmented ventilation/perfusion mismatch, larger emphysematous areas) and/or lowered VT secondary to mechanical constraints. Overlap patients showed slightly, but significantly, lower PaCO2 values at rest () which might chronically reduce CO2 set-point. Interestingly, similar responses to CPET as described herein in overlap might also be found in patients with coexisting COPD and pulmonary hypertension Citation(23) thereby suggesting that heightened pulmonary arterial pressures may also play a role in increasing exercise ventilation in overlap. Moreover, increased activation of group III-IV skeletal muscle afferents could be involved in the heightened ventilatory responses to exercise Citation(24). It is thus conceivable that negative interactions among those abnormalities may underlie greater ventilatory inefficiency in overlap compared to COPD alone.
Overlap patients had lower exercising PETCO2 responses compared to COPD (). PETCO2 is biased to represent poorly ventilated lung units with longer time constants (and higher alveolar CO2 pressures) in patients with COPD Citation(9). In contrast, low PaCO2 (i.e., alveolar hyperventilation), shallow and fast breathing pattern, more homogenous lung emptying, and poor pulmonary perfusion secondary to impaired cardiac output can reduce exercising PETCO2 in overlap (Citation18,25). Heightened ventilation of areas with better preserved ventilation/perfusion matching might also explain why overlap presented with higher resting and exercising SpO2 despite relatively lower transfer factor and, possibly, lower mixed venous O2 saturation. Additional studies with direct measurements of arterial blood gases are warranted to determine at which extent high dead space/VT ratio versus low CO2 set-point might mechanistically explain high ventilatory inefficiency and low PETCO2 in overlap.
A particularly interesting finding of the present study was the lower inspiratory capacity from rest to peak exercise in overlap compared to COPD (). It thus seems that heart failure, similar to other conditions associated with a restrictive pattern (such as obesity) Citation(26), may have a deflating effect in COPD. This rather unexpected effect of heart failure allowed overlap patients to increase VT despite their significantly lower TLC (). Consequently, lower operating lung volumes may have allowed overlap patients to meet the increased ventilatory demands of exercise while delaying the reaching of a critical inspiratory reserve volume associated with limiting dyspnoea Citation(1). Although it could be argued that the total (resistive plus elastic) work of breathing – and the attendant inspiratory neural drive – would be greater in overlap patients Citation(27), it is noteworthy that breathlessness assumed a secondary role (to leg effort) as limiting symptom in overlap. Thus, attenuated volume constraints and preservation of ventilatory demand/capacity ratio may explain lower dyspnoea/ventilation ratio in overlap compared to COPD. These findings were confirmed in a sub-group of FEV1-matched patients and thus cannot be ascribed solely to between-group differences in airflow limitation (at least as indicated by FEV1).
Another key finding of the current study was the shift from dyspnoea to leg effort as the major limiting symptom to exercise in overlap compared to COPD (). Thus, although the primary central insult differs in the isolated diseases, structural and functional aberrations appear to conflate at the periphery (i.e., skeletal muscle) and may play a dominant role in determining physical capacity in overlap (Citation21,28). Impaired cardiac output and poor muscle blood flow Citation(28), unfavourable metabolic and hormonal milieu, increased proportion of less efficiency type II fibres, intrinsic muscle abnormalities and severe deconditioning might be mechanistically linked to those findings Citation(29). From a practical perspective, targeting the peripheral skeletal muscles might be particularly relevant for improving exercise intolerance in this patient population.
There are some clinical scenarios in which we envisage our data might eventually prove useful. Our results suggest that heart failure likely contributes to exercise pathophysiology in COPD in the presence of markedly impaired ventilatory efficiency (E-
CO2 intercept ≤ 3.5 L/minute,
E-
CO2 slope ≥ 34, peak
E/
CO2 ratio ≥ 37) and/or reduced peak PETCO2 (≤31 mmHg). Although those CPET responses clearly lack sensitivity and specificity to separate overlap from COPD (), their presence might suggest the need of additional cardiologic investigations in patients with a high pre-test likelihood of heart failure (e.g., previous myocardial infarction, suspicious imaging findings, and out-of-proportion (to FEV1) exercise intolerance). These variables might also be useful targets to evaluate the efficacy of ‘cardiovascular’ interventions in overlap patients, e.g., cardiac resynchronization therapy or further β-blockage to improve ventilatory inefficiency. Considering the relevance of poor ventilatory efficiency and reduced PETCO2 in predicting poor prognosis in heart failure (Citation17,25), it is conceivable that they may also prove useful to predict survival in overlap. In fact, Alencar et al. recently provided preliminary evidence that
E-
CO2 slope ≥ 34 is an independent predictor of mortality in overlap Citation(20).
Our study presents with some important methodological particularities compared to previous studies which looked at exercise responses in overlap. Patients were seen prospectively by the same respirologists and cardiologists with their treatment being optimized carefully before CPET. In contrast, previous retrospective studies did not control for heterogeneities in COPD and heart failure treatment. For instance, overlap patients followed by cardiologists are less likely to receive adequate bronchodilator treatment; conversely, respirologists are frequently reluctant to prescribe β-blockers (Citation7,8). Thus, the observed differences in overlap versus COPD cannot be ascribed to insufficient treatment for either disease. In fact, pharmacological β-blocking precluded us to test peak heart rate or O2 pulse to discriminate COPD from overlap. Whether coexisting COPD and heart failure with preserved left ventricular ejection Citation(30) similarly impact on CPET responses remains to be investigated. Our results might not be applicable to end-stage COPD with severe resting hypercapnia and/or hypoxaemia. Nevertheless, severely disabled patients are usually unable to tolerate physical exertion and thus are not routinely submitted to incremental CPET.
In conclusion, heart failure with reduced left ventricular ejection fraction had discernible effects on selected physiological responses to exertion in patients with COPD. Thus, CPET provided a cluster of ventilatory and gas exchange variables that were more frequently found in overlap than COPD vis-à-vis an unusually high ventilatory response to metabolic demand (low E-
CO2 intercept, high
E-
CO2 slope and high peak
E/
CO2 ratio) and low peak PETCO2 values (reflecting alveolar hyperventilation and/or lung perfusion abnormalities). These variables might prove eventually useful in practice to assist the clinical interpretation of CPET responses in COPD patients presenting heart failure with reduced left ventricular ejection fraction as co-morbidity.
References
- O'Donnell DE, Laveneziana P, Webb K, Neder JA. Chronic obstructive pulmonary disease: clinical integrative physiology. Clin Chest Med 2014;35:51–69.
- Teopompi E, Tzani P, Aiello M, Ramponi S, Visca D, Gioia MR, et al. Ventilatory response to carbon dioxide output in subjects with congestive heart failure and in patients with COPD with comparable exercise capacity. Respir Care 2014;59:1034–1041.
- Gargiulo P, Apostolo A, Perrone-Filardi P, Sciomer S, Palange P, Agostoni P. A non invasive estimate of dead space ventilation from exercise measurements. PloS One 2014;9:e87395.
- Teopompi E, Tzani P, Aiello M, Gioia MR, Marangio E, Chetta A. Excess ventilation and ventilatory constraints during exercise in patients with chronic obstructive pulmonary disease. Respir Physiol Neurobiol 2014;197:9–14.
- Neder JA, Arbex FF, Alencar MC, et al. Exercise ventilatory inefficiency in mild to end-stage COPD. Eur Respir J 2015;45:377–87.
- Poon CS, Tin C, Song G. Submissive hypercapnia: why COPD patients are more prone to CO2 retention than heart failure patients. Respir Physiol Neurobiol 2015;216:86–93.
- Rutten FH, Cramer M-JM, Lammers J-WJ, Grobbee DE, Hoes AW. Heart failure and chronic obstructive pulmonary disease: an ignored combination? Eur J Heart Fail 2006;8:706–711.
- Macchia A, Rodriguez Moncalvo JJ, Kleinert M, Comignani PD, Gimeno G, Arakaki D, et al. Unrecognised ventricular dysfunction in COPD. Eur Respir J 2012;39:51–58.
- Wasserman K, Zhang YY, Gitt A, Belardinelli R, Koike A, Lubarsky L, et al. Lung function and exercise gas exchange in chronic heart failure. Circulation 1997;96:2221–2227.
- Wensel R, Georgiadou P, Francis DP, Bayne S, Scott AC, Genth-Zotz S, et al. Differential contribution of dead space ventilation and low arterial pCO2 to exercise hyperpnea in patients with chronic heart failure secondary to ischemic or idiopathic dilated cardiomyopathy. Am J Cardiol 2004;93:318–323.
- Apostolo A, Laveneziana P, Palange P, Agalbato C, Molle R, Popovic D, et al. Impact of chronic obstructive pulmonary disease on exercise ventilatory efficiency in heart failure. Int J Cardiol 2015;189:134–140.
- Guazzi M, Myers J, Vicenzi M, Bensimhon D, Chase P, Pinkstaff S, et al. Cardiopulmonary exercise testing characteristics in heart failure patients with and without concomitant chronic obstructive pulmonary disease. Am Heart J 2010;160:900–905.
- Hansen JE, Ulubay G, Chow BF, Sun X-G, Wasserman K. Mixed-expired and end-tidal CO2 distinguish between ventilation and perfusion defects during exercise testing in patients with lung and heart diseases. Chest 2007;132:977–983.
- Guazzi M, Tumminello G, Di Marco F, Fiorentini C, Guazzi MD. The effects of phosphodiesterase-5 inhibition with sildenafil on pulmonary hemodynamics and diffusion capacity, exercise ventilatory efficiency, and oxygen uptake kinetics in chronic heart failure. J Am Coll Cardiol 2004; 44:2339–2348.
- Davey P, Meyer T, Coats A, Adamopoulos S, Casadei B, Conway J, et al. Ventilation in chronic heart failure: effects of physical training. Br Heart J 1992; 68:473–477.
- Dall'Ago P, Chiappa GR, Guths H, Stein R, Ribeiro JP. Inspiratory muscle training in patients with heart failure and inspiratory muscle weakness: a randomized trial. J Am Coll Cardiol 2006; 47:757–763.
- Myers J, Arena R, Dewey F, Bensimhon D, Abella J, Hsu L, et al. A cardiopulmonary exercise testing score for predicting outcomes in patients with heart failure. Am Heart J 2008;156:1177–1183.
- Neder JA, Alharbi A, Berton DC, Alencar MC, Arbex FF, Hirai DM, et al. Exercise ventilatory inefficiency adds to lung function in predicting mortality in COPD. COPD 2016 Apr 14:1–9[Epub ahead of print].
- Rutten FH, Moons KG, Cramer MJ, Grobbee DE, Zuithoff NP, Lammers JW, et al. Recognising heart failure in elderly patients with stable chronic obstructive pulmonary disease in primary care: cross sectional diagnostic study. BMJ 2005; 331(7529):1379.
- Alencar MC, Arbex FF, Souza A, Mazzuco A, Sperandio PA, Rocha A, et al. Does exercise ventilatory inefficiency predict poor outcome in heart failure patients with COPD? J Cardiopulm Rehab Prev (in press).
- Gosker HR, Lencer NHMK, Franssen FME, van der Vusse GJ, Wouters EFM, Schols AMWJ. Striking similarities in systemic factors contributing to decreased exercise capacity in patients with severe chronic heart failure or COPD. Chest 2003;123:1416–1424.
- Whipp BJ, Ward SA, Wasserman K. Ventilatory responses to exercise and their control in man. Am Rev Respir Dis 1984;129:S17–20.
- Thirapatarapong W, Armstrong HF, Bartels MN. Comparing cardiopulmonary exercise testing in severe COPD patients with and without pulmonary hypertension. Heart Lung Circ 2014; 23:833–840.
- Olson TP, Joyner MJ, Eisenach JH, Curry TB, Johnson BD. Influence of locomotor muscle afferent inhibition on the ventilatory response to exercise in heart failure. Exp Physiol 2014; 99:414–426.
- Arena R, Guazzi M, Myers J, Chase P, Bensimhon D, Cahalin LP, et al. Prognostic value of capnography during rest and exercise in patients with heart failure. Congest Heart Fail 2012; 18:302–307.
- O'Donnell DE, Ciavaglia CE, Neder JA. When obesity and chronic obstructive pulmonary disease collide. Physiological and clinical consequences. Ann Am Thorac Soc 2014; 11:635–644. 34.
- Cross TJ, Sabapathy S, Beck KC, Morris NR, Johnson BD. The resistive and elastic work of breathing during exercise in patients with chronic heart failure. Eur Respir J 2012;39:1449–1457.
- Oliveira MF, Arbex FF, Alencar MC, Souza A, Sperandio PA, Medeiros W, et al. Heart failure impairs muscle blood flow and endurance exercise tolerance in COPD. COPD 2016 Jan 20:1–9 [Epub ahead of print].
- Coats AJ, Clark AL, Piepoli M, Volterrani M, Poole-Wilson PA. Symptoms and quality of life in heart failure: the muscle hypothesis. Br Heart J 1994;72(2 Suppl):S36–S39.
- Marcun R, Stankovic I, Vidakovic R, Farkas J, Kadivec S, Putnikovic B, et al. Prognostic implications of heart failure with preserved ejection fraction in patients with an exacerbation of chronic obstructive pulmonary disease. Intern Emerg Med 2015 [Epub ahead of print].