ABSTRACT
The inspiratory pressure is often set by tolerance of chronic obstructive pulmonary disease (COPD) patient during noninvasive pressure support ventilation (PSV). However, physiological effects of this setting remain unclear. This study was undertaken to assess the physiological effect of highest tolerated assist level on COPD patient.
The baseline inspiratory pressure (PS) was titrated by tolerance in 15 severe COPD patients with hypercapnia during acute exacerbation. In addition to the baseline PS, an additional decrease by 25% (PS− = 75% PS) or increase by 25% (PS+ = 125% PS) of PS was applied to the patients. Each level lasted at least 20 minutes. Respiratory rate (RR), tidal volume (Vt), inspiratory effort (PTPpesin/min), and neuro-ventilatory coupling (VE/RMS%) were measured. Asynchrony Index (AI) was calculated.
The Vt and VE/RMS% were significantly increased by PS level (Vt: 561 ± 102 ml, VE/RMS%: 1.06 ± 0.42 L/%, comfort score: 7.5 ± 1.1). The inspiratory muscles were sufficiently unloaded (PTPpesin/min 56.67 ± 32.71 cmH2O.S/min). In comparison with PS, PS+ resulted in a further increase in Vt, VE/RMS% and AI (P < 0.01), with no further reduction in neural drive (RMS) and respiratory muscle activity (P > 0.05).
Increasing inspiratory pressure significantly enhances the VE/RMS% and Vt. However, the inspiratory pressure higher than COPD patient's most tolerated level cannot lead to further reduction in respiratory muscle load and RMS, but more asynchrony events. Physiological data can monitor the patient's responses and the ventilator-patient interaction, which may provide objective criterion to ventilator setting.
Introduction
Noninvasive positive pressure ventilation (NPPV) is the first-line intervention for chronic obstructive pulmonary disease (COPD) patient during acute exacerbation Citation(1,2). NPPV failure is strongly associated with poor outcome Citation(3,4). An optimal setting can improve patient comfort and the success rate Citation(5). Unfortunately, because of lack of the objective standard, inspiratory positive airway pressure (IPAP) is often set by the highest tolerated level of COPD patient Citation(6,7). Objective criterion is needed.
Alternative adjustment in the inspiratory pressure is made to achieve an adequate tidal volume (6–8 ml/Kg) Citation(8) or a satisfactory equilibrium in blood gases Citation(9). However, frequent arterial blood gas (ABG) measurements are invasive and uncomfortable. The improvement of ABG and gas exchange is not the only goal of the NPPV to COPD patients Citation(10–12). More recently, unloading of the inspiratory muscle, patient-ventilator synchrony, and patient's comfort have been analyzed Citation(13,14). The optimal assist level remains unclear Citation(15).
Although pressure support ventilation (PSV) has the risk of asynchrony and over-assistance, it is the most widely used mode of NPPV and has been proven valuable in clinical practice Citation(16,17). As described in our previous report, the impaired neuro-ventilatory coupling of COPD patient is significantly improved by noninvasive PSV, compared with proportional assist ventilation Citation(18). With the well-tolerated inspiratory pressure of noninvasive PSV, the neuro-ventilatory coupling of COPD patient is approximate to that of the age-matched healthy subject Citation(18). The impaired neuro-ventilatory coupling and neural respiratory drive are associated with the respiratory muscle load and dyspnea Citation(19,20). Neural respiratory drive may be a potential biomarker for monitoring the clinical change in acute exacerbation of COPD (AECOPD) patient Citation(21).
We hypothesized that the physiological responses to varying levels of IPAP could be used to identify the optimal assist level in each patient. Our study was undertaken to examine respiratory rate (RR), tidal volume (Vt), inspiratory effort (PTPpesin/min), and neuro-ventilatory coupling during the highest level of IPAP tolerated by the AECOPD patient with hypercapnia. To demonstrate the physiological responses of the COPD patients to the various assist levels from the most tolerated IPAP, three levels of IPAP (75% of baseline IPAP, baseline IPAP, and 125% of baseline IPAP) were applied. The baseline data of the spontaneous breathing (SB) were obtained before ventilation.
Materials and methods
The investigative protocol was approved by the Institutional Ethics Committee of Chinese State Key Laboratory of Respiratory Diseases and informed consents were obtained from all the patients who participated. Our study was registered in Clinicaltrials.gov (NCT01782768) on April 29, 2013.
Patients
Fifteen severe AECOPD patients with respiratory failure were recruited consecutively. The diagnosis of AECOPD was made according to the Global Initiative for Chronic Obstructive Lung Disease (GOLD) guideline Citation(2). All the subjects received NPPV after hospital admission. They were naïve to the objective of the research. All patients received oxygen therapy (2 L/min) and other regular treatments (inhale bronchodilator and systematic corticosteroids). The studies were conducted on patients 3−5 days after hospital admission. The basic information of patients is illustrated in . ABGs data (PaCO2, PaO2, and pH) were measured at the time of study. Respiratory functions (forced vital capacity [FVC] and forced expiratory volume in one second [FEV1]) were measured at the time of recovery. The exclusion criteria are given in the online supplementary materials.
Table 1. The baseline data of the COPD patients with hypercapnia.
Measurements
The flow was measured with a heated pneumotachograph (Fleisch No 1 Pneumotachograph Lausanne Switzerland) connected to a differential pressure transducer (ML141, ADInstruments, Australia). The pneumotachograph was inserted between the face mask and the plateau valve (Respironics USA) of the NPPV circuit. Volume was calculated by numerical integration of the flow signal.
Pulse oxygen saturation (SPO2) was continuously monitored by pulse oximetry (Pulse Oximeter, Heal force, Shenzhen China). Pressure at the airway opening (Paw) was measured with pressure transducer (HY Ruishibo Medical Technology Co. Ltd; Guangzhou, China) connected to one port of the face mask.
Changes in pleural (Ppl) and abdominal (Pab) pressures were obtained from changes in esophageal (Pes) and gastric (Pga) pressures, respectively, by the balloon catheter technique. The two catheters were connected to pressure transducer. The design of balloon catheter and the positioning followed the ATS/ERS Statement on Respiratory Muscle Testing Citation(22). The occlusion test was performed while the patient was breathing through a mouthpiece to verify the correct positioning of the esophageal balloon Citation(22); it was satisfactory in every instance.
The calculation of pressure-time product of inspiratory muscles per minute (PTPPes in/min) was conducted using the time integral of the inspiratory muscles pressure as described Citation(23,24). The pressure developed by the inspiratory muscles was calculated as the difference between esophageal (Pes) pressure and the recoil pressure of the chest wall Citation(23,24).
A multi-pair esophageal electrode catheter with 10 coils, which provided five pairs of electrodes, was used to record the diaphragm electromyogram (EMGdi). The electrode design, positioning, and signal processing were performed as described by Luo et al. Citation(20,25). Raw signals of EMGdi were converted to root mean square (RMS). RMS is the index of the total of EMGdi power Citation(22). EMGdi was normalized to the largest value obtained from maximal maneuvers (RMS%) Citation(26). The largest value of the RMS (RMSmax) obtained from maximal maneuvers was measured in the experiment. More details are presented in the online supplementary materials.
Ventilatory settings
A face mask of fitted size for each patient's face was used. In each patient, the NPPV was delivered by the same ventilator (BiPAP Vision; Respironics USA). “Rise time” was set at 0.1 seconds. The inspiratory trigger, rise time, and expiratory cycling-off criterion were kept constant during the ventilation. The backup respiratory rate (RR) was 10 bpm. The ventilator circuit was equipped with plateau valve (Respironics USA) to prevent rebreathing of CO2. Before starting the protocol, the titration of the IPAP level was performed based on patient's tolerance. A default level of 4 cm H2O of expiratory positive airway pressure (EPAP) was applied to each patient. All the details of the setting can be found in the online supplementary materials.
Experimental procedure
The patient was studied in the afternoon in a semirecumbent position. After the application of topical anesthesia (2% xylocaine), the patient was asked to swallow two balloon-tipped catheters and the multi-pair esophageal electrode catheter through the nose into their right position.
At the first period, the stable signals of unassisted SB (about 5 minutes) were recorded. After this period, the patient was placed on the three levels (PS, PS−, PS+) of noninvasive PSV in random sequence. The IPAP was initially titrated by patient's tolerance (PS). To demonstrate the physiological responses of varying assist levels of PSV, a lower (PS−) and a higher (PS+) level of IPAP corresponding to 75% and 125% of PS, respectively, were also implemented. During experiment, each level lasted at least 20 minutes until the breathing was stable. All the raw signals were recorded. The stable, computer-stored data of the last 15 minutes at each level were calculated and analyzed. At each period the patients scored their comfort sensation with an arbitrary scale ranging from 0 (worst) to 10 (best). The leaks were monitored by the display of the ventilator and the computer during the procedure. When the leak was observed, the mask was carefully adjusted to prevent from leaking.
Patient-ventilator interaction
By detecting the waveform of EMGdi and airway opening pressure (Paw), three main types of asynchronies were quantified according to previously published definitions Citation(27,28): (1) ineffective efforts; (2) auto-triggering, and (3) double triggering. Of note, the double triggering was defined as one neural inspiration triggering two breath cycles. Asynchrony index (AI) was defined as the number of asynchrony events divided by the total RR.
Statistical analysis
The results were expressed as mean ± SD. Differences of the physiological data between spontaneous breathing and each assisted level (SB, PS−, PS, PS+) were evaluated by repeated-measures Analysis of variance (ANOVA), with Least Significant Difference (LSD) post hoc analysis for multiple comparison. A p value<0.05 was considered significant. Statistical analyses were performed using SPSS 17.0 (SPSS Inc., Chicago, IL, USA)
Results
All patients tolerated mask PSV well. No visible leak was detected. The raw signals are shown in . Comfort score was available for each patient. The highest score was obtained at PS level (PS: 7.5 ± 1.1 vs PS−: 4.5 ± 1.3, p < 0.01; PS vs PS+: 5.4 ± 1.1, p < 0.01). The mean value of the most tolerated IPAP was 15.7 ± 1.9 cm H2O.
Vt was significantly higher with PS and PS+ than with SB. The minute ventilation remained constant regardless of the assistance level (PS−, PS, PS+). RR decreased with the increasing assist level. During the ventilation, the pulse oximetry (SPO2) was >98%. There was no significant difference in the SPO2 among three assisted levels.
Table 2. Comparison of differences in ventilatory pattern (Vt, RR, and VE) from spontaneous breathing (SB) with each three levels(PS−, PS, PS+).
As illustrated in , and , in comparison with SB, significant decrease in RMS% and increase in VE/RMS% were observed in PS and PS+, respectively (p < 0.01).
Figure 1. Neural respiratory drive during SB, PS−, PS, PS+ in COPD patient with hypercapnia. Neural respiratory drive is assessed by RMS%. RMS = root mean square, the index of the total of diaphragm electromyogram power. PS = the highest respiratory positive airway pressure set by patient's tolerance. SB = spontaneous breathing. PS− = 75% PS. PS+ = 125% PS. RMS% of the healthy contemporary subject = 9.8 ± 3.2%.
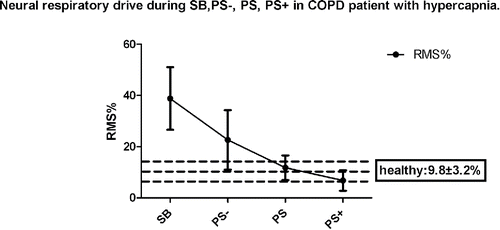
Figure 2. Neuro-ventilatory coupling during SB, PS−, PS, PS+ in COPD patient with hypercapnia. PS− = 75% PS. PS+ = 125%PS. VE = minute ventilation. SB = spontaneous breathing. RMS = root mean square (the index of the total of diaphragm electromyogram powder). Neuro-ventilatory coupling is assessed by VE/RMS%. VE/RMS% of the healthy contemporary subjects = 1.0 ± 0.7 L/%.
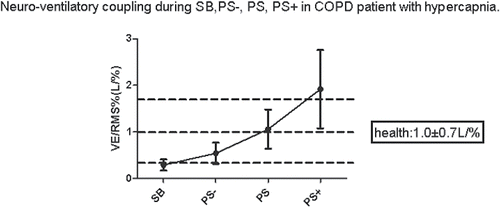
Table 3. The neural respiratory drive (RMS%) and neuro-ventilatory coupling (VE/RMS%) at spontaneous breathing (SB) and each three levels (PS−, PS, PS+).
In contrast with PS, 25% increase of the baseline IPAP caused a remarkable increase in VE/RMS% (p < 0.0001), but the RMS% remained similar (PS+: 6.78 ± 4.00% vs PS: 11.75 ± 4.81%, p = 0.929). Furthermore, in four patients, no transdiaphragmic pressure (Pdi) or EMGdi activity was observed, with positive esophageal pressure (Pes) and gastric pressure (Pga), suggesting that the respiratory muscles were completely passive (Figure S5-PS + B is presented in the online supplementary materials). The AI was significantly lower in PS− and PS compared with that in PS+ (p < 0.01, ).
Table 4. Comparison of differences in peak airway pressure, inspiratory effort (PTPPes in/min), and asynchrony index from spontaneous breathing (SB) with each three levels (PS−, PS, PS+).
As shown in , inspiratory muscles were more unloaded at each level compared with SB (p < 0.01). However, a non-significant decrease of the PTPPes in/min was observed when 25% increase of the baseline IPAP was applied in comparison with PS (p = 0.082).
The respiratory physiological parameters, in particular PTPPes in/min and RMS%, altered quickly within one minute after the assist level was changed.
Discussion
In the present study, the highest tolerated inspiratory pressure applied to COPD patients was basically very close to the average value applied to the patients in the previous randomized physiological study Citation(29). During ventilation, increasing tidal volume and reducing RR are the best ways to increase the alveolar ventilation. A reduction in Vt and an increase in RR are very sensitive markers of an overloaded respiratory muscle pump Citation(30).
Our data demonstrated that the well-tolerated inspiratory support could lead to a marked reduction in inspiratory effort and significant improvement in neuro-ventilatory coupling. As previously observed, increasing the inspiratory pressure from 10 cm H2O to 20 cm H2O caused a significant decrease in respiratory muscle load in COPD patients during NPPV Citation(31). Furthermore, IPAP from 15 to 20 cm H2O was more effective in reducing inspiratory muscle workload Citation(32). With the mean IPAP value of 16 cm H2O, the mean values of the neural drive and neuro-ventilatory coupling in COPD patients receiving NPPV were similar to those in healthy subjects of comparable age (COPD patients: RMS: 11.75 ± 4.81%, VE/RMS%: 1.06 ± 0.42 L/% vs healthy subjects in SB: RMS: 9.8 ± 3.2%, VE/RMS% 1.0 ± 0.7 L/%) Citation(18). In the present study, the inspiratory muscle burden decreased substantially during PS to only about 20% of the level during SB. The AI in PS was much lower in comparison with that in PS+, both of which were not in the high incidence category, of which the AI is higher than 10% Citation(27).
As previously described, the significant improvement in ABGs could be achieved by 12 cm H2O IPAP value Citation(7). In addition, Nava et al. reported that the mean IPAP value of 17.6 cm H2O, ranging from 15 to 20 cm H2O, can produce a satisfactory equilibrium in ABGs of COPD patients Citation(9). Mean IPAP value of 14 cm H2O with a range of 11.5–15.2 cm H2O can produce a tidal volume (Vt) of 6–8 ml/kg of predicted ideal body weight Citation(14). Based on the results of the present study, in combination with those reported by other groups, it is reasonable to speculate that a satisfactory ABGs value and an improved gas exchange in AECOPD patients with respiratory failure can be achieved with an IPAP value of 15.7 ± 1.9 cm H2O, which was set by the tolerance.
To avoid the frequent invasive measurement of placing arterial catheters for sampling arterial blood, ABGs data were not obtained during ventilation. At the range of 10–20 cm H2O, varying levels of IPAP could not significantly influence the ABGs tensions and minute ventilation (VE) during short-term ventilation Citation(9,14). However, with increasing assist level, marked reduction in inspiratory effort and significant improvement in neuro-ventilatory coupling are observed Citation(9,14). We demonstrated the acute physiological effect of the most tolerated IPAP on COPD patients with hypercapnia.
With the 25% increase in IPAP (PS+ = 125% PS), the neural respiratory drive was not remarkably decreased, whereas a greater improvement in the neuro-ventilatory coupling was observed, comparing to that with PS. In four subjects, the respiratory muscles were completely passive, suggesting an excess of pressure support, which might represent a potential harm of pressure support as many studies have already demonstrated Citation(27,33). With the increase in PSV assist level, a progressive increase in Vt values is observed Citation(9,34), which subsequently results in overdistention. Overdistention, in turn, contributes to the downregulation of the activity of the respiratory drive centers Citation(9,34). It is illustrated by the marked alteration of the neuro-ventilatory coupling observed in PS+, with no significant change in neural drive. A higher assist level can augment Vt, decrease respiratory rate, and worsen patient-ventilator asynchrony Citation(33,34). However, Ekkernkamp et al. Citation(35) reported that a higher mean IPAP value (>25 cm H2O) is more effective in improving gas exchange and decreasing the inspiratory effort in stable COPD patients. Although the mean IPAP value of 19.2 ± 2.6 cm H2O is not high, we speculate that an IPAP value of more than 25 cm H2O may induce overdistention in more severe AECOPD patients. More research should be needed to identify the physiological benefit of the mean IPAP value of more than 25 cm H2O.
The patient with respiratory failure is faced with a “choice” between avoiding respiratory muscle fatigue and correcting abnormal ABG tensions. The choice is different from patient to patient. For example, in patients with cystic fibrosis (CF), some CF patients “choose” to reduce EMG activation and maintain Vt whereas others maintain EMG and increase Vt Citation(36). In the present study, the severe AECOPD patients with hypercapnia seem to prefer reducing EMG activation and maintaining Vt. Respiratory muscle fatigue may be the determining factor in these patients. The impaired neuro-ventilatory coupling is associated with higher inspiratory work and respiratory muscle fatigue Citation(37). The respiratory muscles are unloaded and impaired neuro-ventilatory coupling is improved by the noninvasive PSV. With an appropriated inspiratory pressure, the patients feel most comfortable.
Critiques of the method
Comfort scale
As described in previous studies, an arbitrary scale was used to assess the patient's comfort sensation in our trail Citation(14,38,39). The comfort score is usually related to accurate setting of the ventilator inspiratory trigger or sensitivity, interface, and circuit Citation(5). In the present study, the patient was ventilated with the same ventilator (with the same triggering sensitivity, “rise time” and cycling-off criterion). The same type of interface and circuit were applied. Visible leak was not detected. The comfort score may be highly associated with dyspnea due to the neuro-ventilatory coupling and respiratory muscle load Citation(18,39,40). In mechanically ventilated patients, the comfort sensation such as dyspnea can be measured using rating scale Citation(39). Test-retest reliability and intraclass correlation are satisfactory Citation(41). The inspiratory pressure adjustments targeted at dyspnea reduction significantly improve respiratory comfort Citation(42).
Patient selection
Fifteen patients were recruited in the same hospital and therefore subjected to selection bias. We followed the design of previous studies Citation(9,43). However, the number of patient in the present study is more than that in previous studies Citation(9,43,44) The goal of this study is to provide pilot data to demonstrate objective criterion for inspiratory pressure setting to COPD patients with hypercapnia.
Clinical implication
Our findings are potentially clinically relevant. In mechanical ventilation, monitoring the flow signal, EMGdi, and inspiratory muscles activity can detect the patient's physiological responses and the patient-ventilator interaction. Despite the potential clinical usefulness, the technique has limited application as it requires the placement of esophageal and gastric electrodes Citation(21,26). Parasternal electromyogram (EMGpara) signals, recorded from surface electrodes, have a direct relationship with respiratory muscle load Citation(45) and may be used as a noninvasive alternative to the invasive esophageal EMGdi measurement[46,47]. Although the interference from other chest wall muscles cannot be excluded, the neural drive and the inspiratory muscles activity could be monitored eventually by surface electrode with development of the noninvasive technique for surface EMGdi Citation(21). We believe all these physiological signals will be obtained by noninvasive technique during noninvasive ventilation in the future. These physiological data may provide the objective criterion to optimize the ventilator setting.
Conclusion
In summary, the highest inspiratory pressure tolerated by the COPD patients with hypercapnia can appropriately improve the neuro-ventilatory coupling, significantly enhance tidal volume, and sufficiently unload the inspiratory muscles. Increasing assist level significantly enhances the neuro-ventilatory coupling and the tidal volume, but cannot lead to more reduction in respiratory muscle load and neural respiratory drive. The rate of asynchrony is lower during inspiratory pressure set by tolerance compared to higher assist level. Physiological data during ventilation may provide objective criterion to ventilator setting. Recently, surface electromyography (EMG) of the parasternal muscles can be used as a noninvasive alternative to the invasive measurement. Further investigation is needed.
Abbreviations lists | ||
AI = | = | asynchrony index |
ABG = | = | arterial blood gas |
AECOPD = | = | Acute exacerbation of COPD |
BMI = | = | body mass index |
COPD = | = | chronic obstructive pulmonary disease |
EMGdi = | = | diaphragm electromyogram |
EPAP = | = | expiratory positive airway pressure |
FEV1 = | = | forced expiratory volume in one second |
FRC = | = | functional residual capacity; |
IPAP = | = | inspiratory positive airway pressure |
NPPV = | = | noninvasive positive pressure ventilation |
NRD = | = | neural respiratory drive |
RR = | = | Respiratory rate |
PSV = | = | pressure support ventilation |
PTPPes in/min = | = | pressure-time product of inspiratory muscles per minute |
RMS = | = | root mean square |
SB = | = | spontaneous breathing |
VE = | = | minute ventilation |
Vt = | = | tidal volume |
online_supplementary_material1_1_.doc
Download MS Word (48.5 KB)Acknowledgments
The authors thank Mei Jiang (State Key Laboratory of Respiratory Diseases, Guangzhou Medical University, China) for her assistance in statistical analysis of data; Zhan-hong Xie, Ze-guang Zheng, and Yun-xiang Zeng for their assistance in enrolling patients.
Funding
The study was funded by Guangzhou institute of Respiratory Disease State Key Laboratory of Respiratory Disease
Author contribution
All authors have made substantial contribution to the work.
Declaration of interest
All authors have no conflicts of interest to disclose.
References
- Thys F, Roeseler J, Reynaert M, Liistro G, Rodenstein DO. Noninvasive ventilation for acute respiratory failure: a prospective randomised placebo-controlled trial. Eur Respir J 2002; 20(3): 545–555.
- Vestbo J, Hurd SS, Agusti AG, Jones PW, Vogelmeier C, nzueto A, et al. Global strategy for the diagnosis, management, and prevention of chronic obstructive pulmonary disease: GOLD executive summary. Am J Respir Crit Care Med 2013; 187(4): 347–365.
- Ferrer M, Esquinas A, Leon M, Gonzalez G, Alarcon A, Torres A. Noninvasive ventilation in severe hypoxemic respiratory failure: a randomized clinical trial. Am J Respir Crit Care Med 2003; 168(12): 1438–1444.
- Schnell D, Timsit JF, Darmon M, Vesin A, Goldgran-Toledano D, Dumenil AS, et al. Noninvasive mechanical ventilation in acute respiratory failure: trends in use and outcomes. Intensive Care Med 2014; 40(4): 582–591.
- Mehta S, Hill NS. Noninvasive ventilation. Am J Respir Crit Care Med 2001; 163(2): 540–577.
- Porta R, Appendini L, Vitacca M, Bianchi L, Donner CF, Poggi R, et al. Mask proportional assist vs pressure support ventilation in patients in clinically stable condition with chronic ventilatory failure. Chest 2002; 122(2): 479–488.
- Kramer N, Meyer TJ, Meharg J, Cece RD, Hill NS. Randomized, prospective trial of noninvasive positive pressure ventilation in acute respiratory failure. Am J Respir Crit Care Med 1995; 151(6): 1799–1806.
- Fernandez-Vivas M, Caturla-Such J, de la Rosa JG, Acosta-Escribano J, Alvarez-Sanchez B, Canovas-Robles J. Noninvasive pressure support versus proportional assist ventilation in acute respiratory failure. Intensive Care Med 2003; 29(7): 1126–1133.
- Nava S, Bruschi C, Rubini F, Palo A, Iotti G, Braschi A. Respiratory response and inspiratory effort during pressure support ventilation in COPD patients. Intensive Care Med 1995; 21(11): 871–879.
- Kallet RH, Diaz JV. The physiologic effects of noninvasive ventilation. Respir Care 2009; 54(1): 102–115.
- Liesching T, Kwok H, Hill NS. Acute applications of noninvasive positive pressure ventilation. Chest 2003; 124(2): 699–713.
- Antonio ME. Noninvasive mechanical ventilation. In: Enrica B, Michele V, Pressure support ventilation. Springer-Verlag Berlin Heidelberg, 2010; 21–26.
- Wysocki M, Richard JC, Meshaka P. Noninvasive proportional assist ventilation compared with noninvasive pressure support ventilation in hypercapnic acute respiratory failure. Crit Care Med 2002; 30(2): 323–329.
- Schmidt M, Kindler F, Cecchini J, Poitou T, Morawiec E, ersichini R, et al. Neurally adjusted ventilatory assist and proportional assist ventilation both improve patient-ventilator interaction. Crit Care 2015; 19: 56.
- Terzi N, Piquilloud L, Roze H, Deslandes-Boutmy E, Kindler F, Mayaux J, et al. Clinical review: Update on neurally adjusted ventilatory assist–report of a round-table conference. Crit Care 2012; 16(3): 225.
- Barbas CS, Isola AM, Farias AM, Cavalcanti AB, Gama AM, Duarte AC, et al. Brazilian recommendations of mechanical ventilation 2013. Part I. Rev Bras Ter Intensiva 2014; 26(2): 89–121.
- Nava S, Bruschi C, Fracchia C, Braschi A, Rubini F. Patient-ventilator interaction and inspiratory effort during pressure support ventilation in patients with different pathologies. Eur Respir J 1997; 10(1): 177–183.
- Zhang J, Luo Q, Zhang H, Chen R. Effect of noninvasive proportional assist vs pressure support ventilation on neuroventilatory coupling in chronic obstructive pulmonary patients with hypercapnia. Intensive Care Med 2014; 40(9): 1390–1391.
- Gorini M, Spinelli A, Ginanni R, Duranti R, Gigliotti F, Scano G. Neural respiratory drive and neuromuscular coupling in patients with chronic obstructive pulmonary disease (COPD). Chest 1990; 98(5): 1179–1186.
- Jolley CJ, Luo YM, Steier J, Reilly C, Seymour J, Lunt A, et al. Neural respiratory drive in healthy subjects and in COPD. Eur Respir J 2009; 33(2): 289–297.
- Murphy PB, Kumar A, Reilly C, Jolley C, Walterspacher S, Fedele F, et al. Neural respiratory drive as a physiological biomarker to monitor change during acute exacerbations of COPD. Thorax 2011; 66(7): 602–608.
- ATS/ERS Statement on respiratory muscle testing. Am J Respir Crit Care Med 2002; 166(4): 518–624.
- Jubran A, Van de Graaff WB, Tobin MJ. Variability of patient-ventilator interaction with pressure support ventilation in patients with chronic obstructive pulmonary disease. Am J Respir Crit Care Med 1995; 152(1): 129–136.
- Jubran A, Tobin MJ. Pathophysiologic basis of acute respiratory distress in patients who fail a trial of weaning from mechanical ventilation. Am J Respir Crit Care Med 1997; 155(3): 906–915.
- Luo YM, Moxham J, Polkey MI. Diaphragm electromyography using an oesophageal catheter: current concepts. Clin Sci (Lond) 2008; 115(8): 233–244.
- Qin YY, Steier J, Jolley C, Moxham J, Zhong NS, Luo YM. Efficiency of neural drive during exercise in patients with COPD and healthy subjects. Chest 2010; 138(6): 1309–1315.
- Thille AW, Rodriguez P, Cabello B, Lellouche F, Brochard L. Patient-ventilator asynchrony during assisted mechanical ventilation. Intensive Care Med 2006; 32(10): 1515–1522.
- Piquilloud L, Vignaux L, Bialais E, Roeseler J, Sottiaux T, Laterre PF, et al. Neurally adjusted ventilatory assist improves patient-ventilator interaction. Intensive Care Med 2011; 37(2): 263–271.
- Vitacca M, Nava S, Confalonieri M, Bianchi L, Porta R, Clini E, et al. The appropriate setting of noninvasive pressure support ventilation in stable COPD patients. Chest 2000; 118(5): 1286–1293.
- Yang KL, Tobin MJ. A prospective study of indexes predicting the outcome of trials of weaning from mechanical ventilation. N Engl J Med 1991; 324(21): 1445–1450.
- Nava S, Ambrosino N, Rubini F, Fracchia C, Rampulla C, Torri G, et al. Effect of nasal pressure support ventilation and external PEEP on diaphragmatic activity in patients with severe stable COPD. Chest 1993; 103(1): 143–150.
- Vanpee D, El KC, Rousseau L, Jamart J, Delaunois L. Effects of nasal pressure support on ventilation and inspiratory work in normocapnic and hypercapnic patients with stable COPD. Chest 2002; 122(1): 75–83.
- Colombo D, Cammarota G, Bergamaschi V, De Lucia M, Corte FD, Navalesi P. Physiologic response to varying levels of pressure support and neurally adjusted ventilatory assist in patients with acute respiratory failure. Intensive Care Med 2008; 34(11): 2010–2018.
- Leung P, Jubran A, Tobin MJ. Comparison of assisted ventilator modes on triggering, patient effort, and dyspnea. Am J Respir Crit Care Med 1997; 155(6): 1940–1948.
- Ekkernkamp E, Kabitz HJ, Walker DJ, Schmoor C, Storre JH, Windisch W, et al. Minute ventilation during spontaneous breathing, high-intensity noninvasive positive pressure ventilation and intelligent volume assured pressure support in hypercapnic COPD. COPD 2014; 11(1): 52–58.
- Serra A, Polese G, Braggion C, Rossi A. Non-invasive proportional assist and pressure support ventilation in patients with cystic fibrosis and chronic respiratory failure. Thorax 2002; 57(1): 50–54.
- Hart N, Hunt A, Polkey MI, Fauroux B, Lofaso F, Simonds AK. Comparison of proportional assist ventilation and pressure support ventilation in chronic respiratory failure due to neuromuscular and chest wall deformity. Thorax 2002; 57(11): 979–981.
- Schmidt M, Banzett RB, Raux M, Morelot-Panzini C, Dangers L, Similowski T, et al. Unrecognized suffering in the ICU: addressing dyspnea in mechanically ventilated patients. Intensive Care Med 2014; 40(1): 1–10.
- O'Donnell DE, Banzett RB, Carrieri-Kohlman V, Casaburi R, Davenport PW, Gandevia SC, et al. Pathophysiology of dyspnea in chronic obstructive pulmonary disease: a roundtable. Proc Am Thorac Soc 2007; 4(2): 145–168.
- Powers J, Bennett SJ. Measurement of dyspnea in patients treated with mechanical ventilation. Am J Crit Care 1999; 8(4): 254–261.
- Schmidt M, Demoule A, Polito A, Porchet R, Aboab J, Siami S, et al. Dyspnea in mechanically ventilated critically ill patients. Crit Care Med 2011; 39(9): 2059–2065.
- Xirouhaki N, Kondili E, Mitrouska I, Siafakas N, Georgopoulos D. Response of respiratory motor output to varying pressure in mechanically ventilated patients. Eur Respir J 1999; 14(3): 508–516.
- Piquilloud L, Tassaux D, Bialais E, Lambermont B, Sottiaux T, Roeseler J, et al. Neurally adjusted ventilatory assist (NAVA) improves patient-ventilator interaction during non-invasive ventilation delivered by face mask. Intensive Care Med 2012; 38(10): 1624–1631.
- Duiverman ML, van Eykern LA, Vennik PW, Koeter GH, Maarsingh EJ, Wijkstra PJ. Reproducibility and responsiveness of a noninvasive EMG technique of the respiratory muscles in COPD patients and in healthy subjects. J Appl Physiol (1985) 2004; 96(5): 1723–1729.
- Suh ES, Mandal S, Harding R, Ramsay M, Kamalanathan M, Henderson K, et al. Neural respiratory drive predicts clinical deterioration and safe discharge in exacerbations of COPD. Thorax 2015; 70(12): 1123–1130.