ABSTRACT
Arterial stiffness, a marker for cardiovascular risk, is increased in patients with Chronic Obstructive Pulmonary Disease (COPD) and Obstructive Sleep Apnea (OSA). The specific influence of both on arterial stiffness during sleep is unknown. Nocturnal arterial stiffness (Pulse Propagation Time (PPT) of the finger pulse wave) was calculated in 142 individuals evaluated for sleep apnea: 27 COPD patients (64.7 ± 11y, 31.2 ± 8 kg/m2), 72 patients with cardiovascular disease (CVD group, 58.7 ± 13y, 33.6 ± 6 kg/m2) and 43 healthy controls (HC group 49.3 ± 12y, 27.6 ± 3 kg/m2). Sleep stage related PPT changes were assessed in a subsample of COPD patients and matched controls (n = 12/12). Arterial stiffness during sleep was increased in COPD patients (i.e. shortened PPT) compared to healthy controls (158.2 ± 31 vs. 173.2 ± 38 ms, p = 0.075) and to patients with CVD (161.4 ± 41 ms). Arterial stiffening was particular strong during REM sleep (145.9 ± 28 vs. 172.4 ± 43 ms, COPD vs. HC, p = 0.003). In COPD, time SaO2 < 90% was associated with reduced arterial stiffness (Beta +1.7 ms (1.1–2.3)/10 min, p < 0.001). Sleep apnea did not affect PPT. In COPD, but not in matched controls, arterial stiffness increased from wakefulness to REM-sleep (ΔPPT-8.9 ± 10% in COPD and 3.7 ± 12% in matched controls, p = 0.021). Moreover, REM-sleep related arterial stiffening was correlated with elevated daytime blood pressure (r = −0.92, p < 0.001) and increased myocardial oxygen consumption (r = −0.88, p < 0.01). Hypoxia and REM sleep modulate arterial stiffness. In contrast to healthy controls, REM sleep imposes a vascular load in COPD patients independent of sleep apnea indices, intermittent and sustained hypoxia. The link between REM-sleep, vascular stiffness and daytime cardiovascular function suggests that REM-sleep plays a role for increased cardiovascular morbidity of COPD patients.
Abbreviations
AHI | = | Apnea Hypopnea Index |
ATC | = | Anatomical Therapeutical Chemical |
BMI | = | Body Mass Index |
COPD | = | Chronic Obstructive Pulmonary Disease |
CHF | = | Chronic Heart Failure |
CV | = | Cardiovascular |
CVD | = | Cardiovascular Disease |
DBP | = | Diastolic Blood Pressure |
FEV | = | Functional Expiratory Volume |
GLM | = | General Linear Model |
HC | = | Healthy Controls |
HR | = | Heart Rate |
LTOT | = | Long-Term Oxygen Therapy |
MBP | = | Mean Blood Pressure |
NREM | = | Non Rapid Eye Movement |
N1-N3 | = | NREM sleep stages 1–3 |
ODI | = | Oxygen Desaturation Index |
PP | = | Pulse Pressure |
PPT | = | Pulse Propagation Time |
R | = | Regression Coefficient |
REM | = | Rapid Eye Movement |
RPP | = | Rate Pressure Product |
SBP | = | Systolic Blood Pressure |
T < 90% T < 90% | = | Time of oxygen saturation < 90% |
VC | = | Vital Capacity |
WHO | = | World Health Organization |
Introduction
Patients with Chronic Obstructive Pulmonary Disease (COPD) suffer from increased cardiovascular (CV) risk Citation(1,2). While common risk factors such as age, cigarette smoking and increased systemic inflammation are involved, the coexistence of obstructive sleep apnea has been recently identified as additional risk factor for increased morbidity and mortality in patients with COPD Citation(3). However, the mechanism for this excess risk in patients with COPD remains unclear Citation(4).
Assessment of arterial stiffness is an established method to examine mechanisms involved in the development of CV dysfunction in COPD Citation(4,5,6,7). Arterial stiffness reflect structural properties and varies with functional changes of sympathetic vascular activity such as exercise, adrenergic drugs and hypoxia Citation(8,9). Recently, REM sleep has been shown to be associated with marked increased alpha-adrenergic sympathetic activity of the arterial vascular bed, potentially increasing arterial stiffness Citation(10). REM sleep is also known to be associated with worsening of sustained and/or intermittent hypoxia in both COPD and sleep apnea patients Citation(11,12). While hypoxic vasodilation of the arterial vascular bed may reduce cardiac load Citation(13), intermittent hypoxia has been shown to increase peripheral resistance and arterial stiffness Citation(14,15,16,17). Yet the specific influence of sleep related hypoxia and sympathetic activation on CV dysfunction in patients with COPD remains unknown.
In the current study, we examined arterial stiffness during sleep in a convenient sample of COPD and non-COPD patients who were evaluated for the coexistence of obstructive sleep apnea. We hypothesized that arterial stiffness increases with the degree of intermittent hypoxia, and that this increase in arterial stiffness is greater in patients with COPD compared to controls with and without comorbid CV disease. Furthermore, we hypothesized that REM sleep imposes a CV load that is greater in patients with COPD compared to controls. Therefore, we conducted a subset analysis in carefully matched COPD and non-COPD patients to determine the effect of sleep stages on arterial stiffness and daytime cardiac function.
Method
Study settings
The cohort and the pulse wave analysis methodology have been reported in detail before Citation(18,19,20). Six hundred and thirty one patients from five clinical sleep centers in Germany (Nuremberg, Solingen, Berlin, Wuppertal) and Sweden (Göteborg) were referred for a sleep diagnostic test for suspected sleep disordered breathing. Only patients investigated with standard polysomnography without atrial fibrillation or PAP treatment were eligible for the current study (n = 361). The study was conducted according to the amended Declaration of Helsinki and the protocol had been approved by local ethic committees (e-supplement). Oral and written informed consent was obtained.
Study subjects and controls
We identified 27 individuals with clinically diagnosed stable COPD (no exacerbation within pat 4 weeks and not on long-term oxygen therapy). Information on COPD diagnosis was obtained by the pulmonary physician from patient history, the physical status, the concomitant medication, additional information from the hospital chart/the referral as well as from pulmonary function test data () where COPD was defined as FEV1/VC ratio below the 5th percentile of population-based predicted values for age and sex (Global Lung Initiative 2012 reference data base Citation(21)). Those individuals without COPD, yet with CV disease (at least hypertension) and treated with at least one drug (according to Anatomical Therapeutic Chemical (ATC) code class C), were classified as “CVD-group” (n = 72). Subjects without CVD, not prescribed CV medication and lifelong non-smoking history were identified as “Healthy controls” (HC, n = 43) (see flowchart in ). Pulmonary function test data were used to exclude COPD in the HC and CVD groups ().
Table 1. Anthropometric and clinical characteristics in patients with COPD (n = 27) and 2 non-COPD control groups (n = 115). Shown are means ± standard deviation.
Figure 1. Study flow chart. From a pool of polysomnographic sleep studies two analyses have been performed in the current study: Analysis 1 targteted overnight PPT in 142 patients divided in 3 subgroups; analysis 2 targeted sleep stage based PPT analysis performed in 12 COPD patients and 12 matched controls.
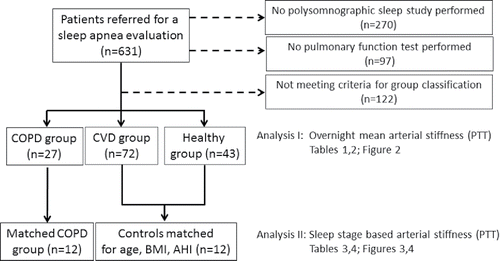
A subsequent sleep stage based analysis of arterial stiffness was performed in 12 COPD patients (2 females) and 12 matched controls (4 females) recruited from the groups stated above (). Matching criteria included age (±3 years), Body Mass Index (BMI) (±3 kg/m2), and sleep apnea severity (Apnea Hypopnea Index (AHI) of ±5 events/hr for AHI < 15/hr, ±10 events/hr for AHI > 15–50 events/hr and ±15 for AHI>50 events/hr) and overnight mean saturation in COPD individuals < 95%.
Overnight pulse wave analysis
A modified pulse oximeter was applied during standard polysomnography (Somnolab, Weinmann, Hamburg, Germany). As described elsewhere Citation(18,20), Pulse Propagation Time (PPT) was computed as the time difference between the maximum of the reflection (diastolic) wave trefl and the time point of the maximum of the systolic pulse wave tmain (, e-supplement). PPT, referred to as “arterial stiffness”, and hypoxic measures (oxygen desaturation index (ODI) 4%, time below oxygen saturation <90% (T<90%)) were analyzed as means over the entire night and in the sleep stages NREM/REM and wakefulness within the sleep period. Data were computed for Rapid Eye Movement (REM) sleep, Non REM stages 1, 2, and 3 (N1, N2, and N3, respectively) as well as during wakefulness (wake). PPT is given both in milliseconds (ms) and in percentage of the value obtained during wakefulness (defined as 100%).
Assessment of sleep and breathing
Sleep stages and breathing events were scored according to American Academy of Sleep Medicine criteria Citation(22) including the alternative hypopnea criteria. Intermittent hypoxia was defined as ODI4% whereas sustained hypoxia was defined as T<90%. In the sleep stage based analysis, T<90% was calculated for NREM and REM sleep. In addition, ODI2% was calculated as a measure of intermittent hypoxia for each sleep stage based on our previous findings of a strong association with cardiovascular dysfunction Citation(18,19).
Assessment of cardiovascular risk factors
Comorbidities as well as concomitant medication were assessed by clinical interview and hospital medical charts/information from the referral. Office blood pressure (SBP/DBP) and heart rate (HR) were determined during supine rest Citation(23). Mean blood pressure (MAP) and pulse pressure (PP) were calculated. The Rate Pressure Product (RPP), a measure of cardiac load/myocardial oxygen demand, was calculated according to the formula: RPP = HR*SBP Citation(24). Fasting lipids and smoking status were determined.
Data analysis and statistics
Descriptive statistics are presented as mean ± standard deviation. Statistical significance was set at p < 0.05, two tailed. Between group differences were tested by ANOVA, Student t-test, Kruskal Wallis test, or the Chi-square test (for equally/not equally distributed data and distributions, respectively). Within group differences were tested by means of the paired t-test or the Wilcoxon Signed Rank test. Post hoc between group comparisons were corrected with the Bonferroni method. Pearson´s and Spearmans correlation analysis assessed associations between PPT and daytime CV measures (e.g. blood pressure) as well as measures of nocturnal hypoxia. Significant predictors of arterial stiffness in univariate analysis (age, blood pressure, gender, comorbidities, sleep variables) were used in General Linear Models (GLM) to explore independent predictors of overnight PPT as a measure of arterial stiffness. The analysis was divided into the three subgroups (COPD, CV disease and healthy controls) and followed thereby the study inclusion criteria described above. Numbers of individuals included in the analysis are stated in the result section. Analyses were performed using IBM-SPSS (version 22.0, Chicago, USA).
Results
Anthropometric and clinical characteristics are shown in . CV and metabolic comorbidities were more prevalent in COPD patients and in the CVD group. Compared to healthy controls, the degree of sustained hypoxia (mean oxygen saturation and T < 90%) was greater in the COPD group and slightly elevated in the CVD group. Clinical significant sleep apnea (AHI ≥ 15) occurred in approximately a third of COPD patients (29.6%). Lung function test performed in COPD patients showed pathological values compared to normal values in the CVD and HC groups ().
Mean overnight PPT varied over the three patient groups (ANOVA, p = 0.098) and PPT tended to be shorter in COPD patients compared to healthy controls (158.2 ± 31 vs. 173.2 ± 38 ms, p = 0.075), while PPT in patients with CVD (161.4 ± 41 ms) did not differ from PPT in the COPD group. Arterial stiffening was particular strong during REM sleep in COPD patients (145.9 ± 28 vs. 172.4 ± 43 ms, COPD vs. HC, p = 0.003). In COPD patients, sustained hypoxia, assessed as time of oxygen saturation <90% (T < 90%) during the entire sleep period, correlated strongly with PPT (). In GLM analysis, T < 90% T < 90% negatively predicted arterial stiffness in both, COPD and HC patients (), whereas AHI or ODI as measures of apnea related intermittent hypoxia did not. Moreover, independent predictors of overnight mean PPT included established CV risk factors like age, BMI, and blood pressure.
Figure 2. Association between pulse propagation time (PPT in milliseconds) and time below 90% oxygen saturation (in minutes) for the three study groups: Healthy Controls group, Cardiovascular Disease group (CVD) and Chronic Obstructive Pulmonary Disease group (COPD). Spearmen rho correlation factor (R) and significance level are shown.
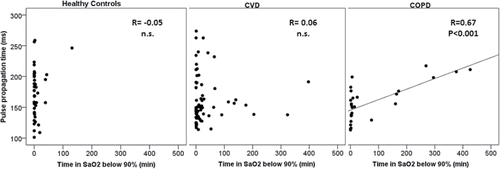
Table 2. Independent predictors of mean overnight Pulse Propagation Time (PPT) obtained in 3 different General Linear Models for (a) 27 COPD patients, (b) 72 CVD patients and (c) 43 healthy controls. Beta values indicate the numerical influence on PPT for each factor listed in the table.
For the subgroup analysis of sleep stage based PPT changes, the COPD and control groups were well matched with regard to anthropometric and clinical data (). The changes observed in arterial stiffness over the different sleep stages are exemplified in one recording example of a patient with COPD (, e-supplement). For the entire group, PPT analysis by sleep stages followed two different patterns in COPD and control subjects (, PPT-% change from wakefulness level (reference 100%)): First, arterial stiffness decreased (= PPT increased) from wake to deep sleep (N3) (% change in PPT 8.5 ± 7%, p = 0.002) as well as from light to deep sleep (N1 to N3) ΔPPT 6.6 ± 9, p = 0.044) in controls but not in COPD patients (ΔPPT 1.3 ± 12% and 4.9 ± 9%, n.s., respectively). Second, COPD patients decreased PPT significantly from wakefulness to REM sleep whereas controls increased PPT values slightly when compared to wakefulness (ΔPPT −8.9 ± 10% in COPD and 3.7 ± 12% in matched controls, between group difference p = 0.021). Third, REM sleep PPT was lower in COPD patients compared with controls (% of awake PPT 91 ± 12% vs. 108 ± 12%, between group difference p = 0.019). Changes in PPT by sleep stage did not associate with pulmonary function (FVC/VC ratio), the amount of intermittent hypoxia (ODI 2% for NREM and REM sleep), or sustained hypoxia (mean SaO2 or time of SaO2 < 90%) (, e-supplement).
Table 3. Anthropometric and clinical data in patients with COPD and matched controls. Shown are means ± standard deviation.
Figure 3. Percentage of change in pulse propagation time (PPT, mean and standard error of the means (SEM)) from wakefulness (= 100%) in patients with COPD and matched non-COPD controls. Asterix indicate significant differences (all p < 0.05): Within group increase in PPT from wake to N3 (non-COPD controls) and PPT decrease from wake to REM (COPD patients); between group difference in REM sleep PPT between COPD patients and non-COPD controls. N1, N2, N3 and REM indicate the sleep stages NREM1-3 and REM. Please note, a decrease in PPT corresponds to an increase in arterial stiffness and vice versa.
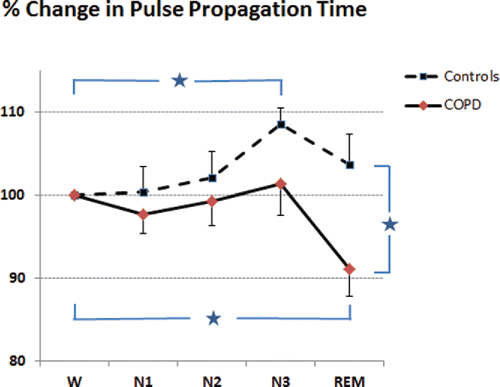
Finally, REM sleep related arterial stiffening was associated with elevated systolic and mean blood pressure, pulse pressure, and signs of increased myocardial oxygen consumption (RPP) only in the COPD group (, ). Intermittent or sustained nocturnal hypoxia did not associate significantly with daytime hemodynamic variables in this case control sub-study.
Table 4. Correlations between the change in pulse wave propagation time from wakefulness to REM-sleep and daytime hemodynamic parameters.
Discussion
We report three major findings: First, we found that hypoxia and REM sleep modulate overnight arterial stiffness in COPD and healthy controls. Second, in contrast to healthy controls, REM sleep increased arterial stiffness in COPD patients independent of sleep apnea indices, intermittent and sustained hypoxia. Third, the REM-sleep dependent increases of arterial stiffness, and not measures of hypoxia, predicted daytime arterial blood pressure and myocardial oxygen consumption in patients with COPD. Our data suggest that REM sleep imposes a vascular load in COPD patients independent of sleep apnea indices, intermittent and sustained hypoxia.
The effect of hypoxia during sleep on vascular circulation has been studied extensively both in humans and animal models Citation(25–30). While there is consensus that hypoxia generates vasodilation through release of endothelial vasodilatory substances such as NO Citation(13), the overall effect of hypoxia on arterial stiffness remains unclear. This uncertainty may primarily be due to the extent of counter-regulatory increase of sympathetic nervous system activity in response to hypoxia. Indeed, sympathetic activity during sleep will increase vascular muscle tone and highly likely even arterial stiffness Citation(10,26,31). Depending on the balance between these opposing factors the net effect may be either a relaxation or stiffening of the vascular bed. We found that NREM hypoxia was associated with prolonged PPT values. In contrast, REM sleep hypoxia had no additional effect on PPT. A possible explanation is that, sympathetic nerve activity is known to increase during REM sleep Citation(10,32) independently of hypoxia Citation(33,34). Our data strongly suggest that during REM sleep vasoconstrictive forces dominate over the hypoxic vasodilatory component in COPD patients. Taken together, our data demonstrate that REM sleep imposes a vascular load in COPD patients independent of sustained hypoxia. The COPD patients were recruited from patients with suspected sleep apnea and had similar sleep apnea characteristics when compared to a population based study of individuals with mild airway obstruction Citation(35) and a clinical cohort sample with moderate to severe COPD Citation(36). Several studies have demonstrated that sleep apnea in the absence of COPD is associated with increased vascular stiffness, both during sleep and daytime Citation(14–17). We have demonstrated that intermittent hypoxia had no independent effect on arterial stiffness in COPD. This may indicate that intermittent hypoxia in COPD may not be the major contributor for the increased CV morbidity and mortality.
Our study confirmed a strong association between traditional risk factors of cardiovascular disease (including age, blood pressure, obesity) and overnight PPT as a measure of arterial stiffness Citation(8,9,37). We extended these findings by demonstrating that data in patients with COPD showed that sleep stage related changes in arterial stiffness had a strong linear association with daytime hemodynamic measures like daytime arterial blood pressure and RPP—a measure of myocardial oxygen consumption. Vascular stiffening during REM sleep may be an independent predictor of CV morbidity in COPD.
There are several strengths and limitations to the current study. This is to our knowledge the first study addressing the changes of arterial stiffness during sleep in COPD patients. Our approach to identify COPD patients and controls, all based on pulmonary function test data, with and without CV disease provided three well defined subsamples allowing for the analysis of different comorbid influences on arterial stiffness. Moreover, we successfully matched COPD and non-COPD control patients in order to identify vascular stiffness pattern during REM sleep. Another strength resides in a careful matching of individuals in the sub-study. Our method to calculate arterial stiffness by an oximeter based pulse wave propagation time analysis has been validated against applanation tonometry of the radial artery and assessment of aortic pulse wave velocity (18, data on file). Indeed, pulse wave analysis has previously been applied for vascular function assessments in COPD and OSA patients Citation(38–40). Third, our study used comprehensive investigations for cardiovascular risk status comparable to Framingham and EU SCORE CV risk classifications Citation(18).
Limitations include the lack of a population based healthy control group and the limited number of COPD patients. Our study is therefore more likely to underestimate the true disease impact of COPD on the cardiovascular system. In our case control sub-analysis, the clinical characteristics of the COPD patients and their matched controls differed with regard to smoking status and CHF prevalence (). However, our analysis revealed no systematic change in arterial stiffness by CHF disease status. Lastly, the mechanisms or the causal relationship between sleep related changes in arterial stiffness in COPD could not be investigated with the current study design. Methods which can assess local or systemic sympathetic activity during NREM and REM sleep may be used in future studies.
The present work has several important clinical implications. First, the data strongly suggest that sleep imposes a specific load to the cardiovascular system in COPD and this influence may provide diagnostic information for CV risk estimation in COPD. Further mechanistic studies are needed to fully understand NREM and REM specific modulations of arterial stiffness in COPD and other chronic conditions characterized by hypoxia and inflammation. Second, the results suggest a potential vasorelaxing effect of sustained hypoxia during sleep. If the observed changes in PPT are associated with significant hemodynamic consequences, it may explain the deleterious effects of hypoxia on mortality and morbidity in patients with COPD. Third, assessment of PPT by finger pulse oximetry has a number of advantages like robustness, feasibility, non-invasiveness and validated use during sleep. The diagnostic potential for utilizing sleep for cardiovascular monitoring is given.
In conclusion, REM sleep imposes a vascular load in COPD patients independent of sleep apnea indices, intermittent and sustained hypoxia. REM sleep was associated with strong arterial stiffening only in COPD patients suggesting that mechanisms other than hypoxia may affect the CV system. The link between REM-sleep and daytime cardiovascular function suggests that REM-sleep plays a role for increased cardiovascular morbidity of COPD patients. Future studies exploring overnight PPT may provide new insights in the early identification of harmful CV outcome in COPD.
Declaration of interest
1. | Dr. Grote reports personal fees from Weinmann GMBH and grants from the Swedish Heart and Lung Foundation during the conduct of the study; personal fees from Resmed, Philips, Mundipharma, and Breas as well as grants from the Resmed and Philips Foundations outside the submitted work. | ||||
2. | Dr. Hedner reports grants from ResMed, grants from Phillips Respironics, payments related to speaker activities from AstraZeneca, Novartis, Takeda and Jazz Pharmaceuticals outside the submitted work. | ||||
3. | Dr. Fietze reports personal fees from Somnomed and grant support from Heinen and Löwenstein, Philips, Resmed, Weinmann and Hoffrichter. | ||||
4. | Dr. Penzel is shareholder of The Siestagroup GmbH and Advanced Sleep Research. His institution has received grants from Cidelec, Apnex, Imthera, Itamar, Nomics, Heinen and Löwenstein, Philips, Resmed, Weinmann. | ||||
5. | Dr. Randerath reports personal fees from Inspire, personal fees from Philips Respironics, personal fees from Resmed, personal fees from Weinmann, outside the submitted work. | ||||
6. | Dr. Sanner reports grants from Weinmann outside the submitted work. | ||||
7. | Dr. Ficker reports personal fees from Weinmann during the conduct of the study. | ||||
8. | Dr. Schneider reports personal fees from Linde Healthcare, TNI Medical, Fisher & Paykel Healthcare, InSleep, Philipps Respironics, Apnex. My institution has received grants from Imthera, Resmed, InSleep, TNI Medical and Fisher & Paykel Healthcare. | ||||
9. | Dr. Sommermeyer has nothing to disclose. |
E-supplement_L_Grote_Arterial_Stiffness_in_COPD_revision_3_clean_file.pdf
Download PDF (538.1 KB)ICOP-1365119_E-supplement_L_Grote_Arterial_Stiffness_in_COPD_revision_3_red_marked.pdf
Download PDF (626.1 KB)Acknowledgments
Contribution: The corresponding author (LG) takes responsibility for the content of the manuscript, including the data and analysis. All co-authors except for HS were involved in the collection of data. LG and HS planned and performed the data analysis, they also wrote the first draft of the manuscript. All co-authors reviewed the data and were involved in the preparation of the final manuscript.
Funding sources of the study: This study was supported by the Swedish Heart and Lung Foundation (HLF 20110445, 20120429 and 20130488), the German Ministry for Education and Science (BMBF), the National Institute of Health (R01 HL105546), and Weinmann Geräte für Medizin GmbH&Co.KG.
References
- Müllerova H, Agusti A, Erqou S, Mapel DW. Cardiovascular comorbidity in COPD: Systematic literature review. Chest. 2013; 144(4):1163–1178.
- Sin Sin DD, Man SF. Chronic obstructive pulmonary disease as a risk factor for cardiovascular morbidity and mortality. Proc Am Thorac Soc. 2005; 2(1):8–11.
- Marin JM, Soriano JB, Carrizo SJ, Boldova A, Celli BR. Outcomes in patientswith chronic obstructive pulmonary disease and obstructive sleep apnea: the overlap syndrome. Am J Respir Crit Care Med. 2010; 182(3):325–331.
- Maclay JD, MacNee W. Cardiovascular disease in COPD: mechanisms. Chest. 2013; 143(3):798–807.
- Mills NL, Miller JJ, Anand A, Robinson SD, Frazer GA, Anderson D, et al. Increased arterial stiffness in patients with chronic obstructive pulmonary disease: a mechanism for increased cardiovascular risk. Thorax. 2008; 63(4):306–311.
- Vivodtzev I, Tamisier R, Baguet JP, Borel JC, Levy P, Pépin JL. Arterial stiffness in COPD. Chest. 2014; 145(4):861–875.
- Maclay JD, McAllister DA, Mills NL, Paterson FP, Ludlam CA, Drost EM, et al. Vascular dysfunction in chronic obstructive pulmonary disease. Am J Respir Crit Care Med. 2009; 15; 180(6):513–520.
- Van Bortel LM, Duprez D, Starmans-Kool MJ, Safar ME, Giannattasio C, Cockcroft J, et al. Clinical applications of arterial stiffness, Task Force III: recommendations for user procedures. Am J Hypertens. 2002; 15(5):445–452.
- Chirinos JA. Arterial stiffness: basic concepts and measurement techniques. J Cardiovasc. Transl Res. 2012; 5(3):243–255.
- Lavie P, Schnall RP, Sheffy J, Shlitner A. Peripheral vasoconstriction during REM sleep detected by a new plethysmographic method. Nat Med 2000; 6:606.
- Flenley DC. Sleep in chronic obstructive lung disease. Clin Chest Med. 1985; 6(4):651–661.
- Biselli PJ, Grossman PR, Kirkness JP, Patil SP, Smith PL, Schwartz AR, et al. The Effect of Increased Lung Volume in Chronic Obstructive Pulmonary Disease on Upper Airway Obstruction during Sleep. J Appl Physiol 1985; 2015; 5:jap.00455.2014.
- Halliwill JR. Hypoxic regulation of blood flow in humans. Skeletal muscle circulation and the role of epinephrine. Adv Exp Med Biol. 2003; 543:223–36.
- Phillips CL, Butlin M, Wong KK, Avolio AP. Is obstructive sleep apnoea causally related to arterial stiffness? A critical review of the experimental evidence. Sleep Med Rev. 2013; 17(1):7–18.
- Scholze A, Lamwers S, Tepel M, Sanner BM. Nasal continuous positive airway pressure: influence on digital volume pulse in obstructive sleep apnoea patients. Eur Respir J. 2012; 39(5):1127–1135.
- Jelic S, Bartels MN, Mateika JH, Ngai P, DeMeersman RE, Basner RC. Arterial stiffness increases during obstructive sleep apneas. Sleep. 2002; 25(8):850–855.
- Wons AM, Kohler M. Established vascular effects of continuous positive airway pressure therapy in patients with obstructive sleep apnoea-an update. J Thorac Dis. 2015; 7(5):912–919.
- Sommermeyer D, Zou D, Eder DN, Hedner J, Ficker JH, Randerath W, et al. The use of overnight pulse wave analysis for recognition of cardiovascular risk factors and risk: a multicentric evaluation. J Hypertens. 2014; 32(2):276–285.
- Grote L, Sommermeyer D, Zou D, Eder DN, Hedner J. Oximeter-based autonomic state indicator algorithm for cardiovascular risk assessment. Chest. 2011; 139(2):253–259.
- Sommermeyer D, Zou D, Ficker JH, Randerath W, Fischer, C, Penzel T, et al. Detection of cardiovascular risk from a photoplethysmographic signal using a matching pursuit algorithm. Med Biol Eng Comput. 2016 Jul; 54(7):1111–1121.
- Quanjer PH, Stanojevic S, Cole TJ, Baur X, Hall GL, Culver BH, Enright PL, Hankinson JL, Ip MSM, Zheng J, Stocks J. Multi-ethnic reference values for spirometry for the 3–95-yr age range: the global lung function 2012 equations. Eur Resp J 2012; 40:1324–1343
- Iber C, Ancoli-Israel S, Chesson A, Quan S, for the American Academy of Sleep Medicine. The AASM Manual for the Scoring of Sleep and Associated Events: Rules, Terminology and Technical Specifications. 1st ed. American Academy of Sleep Medicine, Westchester, IL; 2007.
- O'Brien E, Asmar R, Beilin L, Imai Y, Mallion JM, Mancia G, et al. european society of hypertension working group on blood pressure monitoring. european society of hypertension recommendations for conventional, ambulatory and home blood pressure measurement. J Hypertens. 2003; 21(5):821–848.
- White WB. Heart rate and the rate-pressure product as determinants of cardiovascular risk in patients with hypertension. Am J Hypertens. 1999; 12:50S–55S.
- Coccagna G, Mantovani M, Brignani F, Parchi C, Lugaresi E. Continuous recording of the pulmonary and systemic arterial pressure during sleep in syndromes of hypersomnia with periodic breathing. Bull Physiopathol Respir. 1972; 8(5):1159–1172.
- Hedner J, Ejnell H, Sellgren J, Hedner T, Wallin G. Is high and fluctuating muscle nerve sympathetic activity in the sleep apnoea syndrome of pathogenetic importance for the development of hypertension? J Hypertens Suppl. 1988; 6(4):S529–S531.
- Schneider H, Schaub CD, Andreoni KA, Schwartz AR, Smith PL, Robotham JL, et al. Systemic and pulmonary hemodynamic responses to normal and obstructed breathing during sleep. J Appl Physiol 1985; 1997 Nov;83(5):1671–1680.
- Schneider H, Schaub CD, Chen CA, Andreoni KA, Schwartz AR, Smith PL, et al. Neural and local effects of hypoxia on cardiovascular responses to obstructive apnea. J Appl Physiol 1985. 2000; 88(3):1093–1102.
- Fletcher EC. Invited review: Physiological consequences of intermittent hypoxia: systemic blood pressure. J Appl Physiol 1985. 2001; 90(4):1600–1605.
- Fletcher EC, Levin DC. Cardiopulmonary hemodynamics during sleep in subjects with chronic obstructive pulmonary disease. The effect of short- and long-term oxygen. Chest. 1984; 85(1):6–14.
- Zou D, Grote L, Eder DN, Peker Y, Hedner J. Obstructive apneic events induce alpha-receptor mediated digital vasoconstriction. Sleep. 2004; 27(3):485–489.
- Somers VK, Dyken ME, Mark AL, Abboud FM. Sympathetic-nerve activity during sleep in normal subjects. N Engl J Med. 1993 Feb 4; 328(5):303–307.
- Trinder J, Kleiman J, Carrington M, Smith S, Breen S, Tan N, et al. Autonomic activity during human sleep as a function of time and sleep stage. J Sleep Res. 2001; 10(4):253–264.
- Herscovici S, Pe'er A, Papyan S, Lavie P. Detecting REM sleep from the finger: an automatic REM sleep algorithm based on peripheral arterial tone (PAT) and actigraphy. Physiol Meas. 2007 Feb; 28(2):129–140
- Sanders MH, Newman AB, Haggerty CL, Redline S, Lebowitz M, Samet J, et al. Sleep Heart Health Study. Sleep and sleep-disordered breathing in adults with predominantly mild obstructive airway disease. Am J Respir Crit Care Med. 2003 Jan 1; 167(1):7–14.
- Soler X, Gaio E, Powell FL, Ramsdell JW, Loredo JS, Malhotra A, et al. High Prevalence of Obstructive Sleep Apnea in Patients with Moderate to Severe Chronic Obstructive Pulmonary Disease. Ann Am Thorac Soc. 2015;12(8):1219–1225.
- Mancia G, De Backer G, Dominiczak A, Cifkova R, Fagard R, Germano G, et al. European society of hypertension; european society of cardiology. 2007 ESH-ESC Guidelines for the management of arterial hypertension: the task force for the management of arterial hypertension of the european society of hypertension (ESH) and of the european society of cardiology (ESC). Blood Press. 2007; 16(3):135–232.
- Clarenbach CF, Stoewhas AC, van Gestel AJ, Latshang TD, Lo Cascio CM, Bloch KE, et al. Comparison of photoplethysmographic and arterial tonometry-derived indices of arterial stiffness. Hypertens Res. 2012; 35(2):228–233.
- Millasseau SC, Kelly RP, Ritter JM, Chowienczyk PJ. Determination of age-related increases in large artery stiffness by digital pulse contour analysis. Clin Sci (Lond) 2002; 103(4):371–377.
- Gunarathne A, Patel JV, Hughes EA, Lip GY. Measurement of stiffness index by digital volume pulse analysis technique: clinical utility in cardiovascular disease risk stratification. Am J Hypertens 2008; 21(8):866–872.