Abstract
Despite the many benefits of performing physical exercise in patients with chronic obstructive pulmonary disease (COPD), information on the response of acute cardiac autonomic modulation in subjects with moderate and severe COPD during and after an aerobic exercise session at different intensities is unknown. The aim of this study was to evaluate the response of cardiac autonomic modulation in patients with moderate and severe COPD during and after an aerobic exercise session at different intensities. Twenty-seven patients with COPD, divided into: Moderate Group and Severe Group, underwent an aerobic exercise sessions with intensities equivalent to 60% and 90% of velocity corresponding to peak oxygen consumption. The heart rate variability (HRV) indices were analyzed in the time and frequency domains at the following times: at rest, during exercise, immediately after, and 5, 10, and 15 minutes after exercise. In the comparison analysis between the two groups, no differences were observed in any of the HRV indices at different intensities applied. However, it was observed that the exercise caused autonomic changes when the groups were analyzed separately. Sessions of aerobic exercise influence the autonomic modulation in patients with COPD. However, COPD severity did not influence the autonomic nervous system response to exercise and recovery moments; and there was no difference between the exercise intensities
Introduction
In addition to the widely known pulmonary impairment as a result of chronic obstructive pulmonary disease (COPD), cardiovascular phenomenon, such as autonomic neuropathy, is commonly observed in individuals with this disease (Citation1, Citation2). Previous studies have shown that patients with COPD present decreased heart rate variability (HRV), with elevated heart rate, consistent with cardiac sympathetic activation (Citation3). This exaggerated increase in muscle sympathetic nerve activity contributes to muscle hypoperfusion and thus may constitute a peripheral limit to exercise in subjects with heart failure (Citation4), and COPD (Citation5). In addition, increased sympathetic activity and a consequent reduction in parasympathetic activity are related to the pathophysiology of some diseases such as arrhythmias and a high risk of mortality (Citation6, Citation7).
HRV is considered a tool of clinical importance that allows a simple and noninvasive assessment describing the oscillation of intervals between consecutive heart beats (RR intervals), which are related to the influences of the autonomic nervous system (ANS) on the sinus node, which can be used to identify phenomena related to the ANS (Citation8).
It is known that changes in HRV behavior provide a sensitive and early indicator of health impairments (cardiac mortality related arrhythmia, early stages of disorders such as myocardial infarction, and heart failure) (Citation9), in a way that high HRV is a sign of good adaptation, characterizing a healthy individual with efficient autonomic mechanisms, and low HRV is an indicator of abnormal and insufficient ANS adaptation, which may indicate the presence of physiological malfunction (Citation8).
Recently, it has been suggested that changes in cardiac autonomic function may be related to the level of physical activity in patients with COPD (Citation10), besides being an important determinant of physiological responses to physical activity (Citation11), such as in aerobic exercise (Citation12). In patients with COPD, exercise has been shown to be an effective intervention for quality of life, improvement in exercise tolerance and symptom control, as well as functional capacity optimization (Citation13, Citation14). Information regarding cardiac autonomic modulation during and after an acute session of aerobic exercise has been studied in healthy subjects (Citation15–17) and after resistance exercise in subjects with COPD (Citation18, Citation19), however, this information regarding an acute session of aerobic exercise in patients with COPD is unknown.
This knowledge would be of clinical relevance since it is known that these patients present impairments in autonomic modulation and physical exercise is widely indicated as part of pulmonary rehabilitation programs. Furthermore, it becomes critical to understand if the stage of COPD can influence the ANS response to exercise.
Therefore, knowing all aspects of the response of patients with COPD to exercise becomes crucial to perform safe rehabilitation. Considering these aspects, the present study aimed to evaluate the acute response of cardiac autonomic modulation in patients with moderate and severe COPD during and after an aerobic exercise session at different intensities.
We hypothesized that a session of aerobic exercise at different intensities in COPD patients would modify autonomic modulation after its completion, and that these responses would be dependent on the intensity of the exercise performed and the severity of COPD.
Methods
Subjects
To conduct this study, data from 27 patients were used, recruited in the Center for Studies and Assistance in Physiotherapy and Rehabilitation of the Faculty of Science and Technology – FCT/UNESP, Presidente Prudente, São Paulo, Brazil. The patients had a clinical diagnosis of COPD confirmed by spirometry according to the Global Initiative for Obstructive Lung Disease (GOLD): FEV1/FVC <0.70 post-bronchodilator (Citation20). These patients were divided into two groups: Moderate COPD Group, which consisted of patients classified as Stages I and II and Severe COPD Group which consisted of patients with Stages III and IV (Citation20).
Volunteers who had at least one of the following conditions were not included in the study: smoking, presence of disabling pathological conditions, and/or unstable heart disease that could influence the performance of physical activity, clinical instability (exacerbations and changes in medication in previous 30 days), the use of drugs that influence autonomic activity of the heart, and participation in any other physical training program in the previous 6 months.
Subjects were informed about the procedures of the study and all of them signed an informed consent form. All procedures were approved by the Ethics Committee of the Institution (CAAE: 01114912.0.0000.5402).
Study design
The experimental protocol consisted of an initial evaluation and all subjects performed a cardiopulmonary exercise test for aerobic exercise prescription. Subsequently, assessment of autonomic function was carried out while the patients performed protocols of aerobic exercise at 60% and 90% of the velocity corresponding to the peak oxygen uptake (vVO2peak). The order of the sessions at different intensities (60% and 90% vVO2peak) of exercise was randomized by a draw for all patients. The initial evaluation consisted of patient identification, anthropometric measurements (Citation21), pulmonary function assessment (Citation22), and a cardiopulmonary exercise test in a room with a temperature between 21 °C and 23 °C and relative humidity between 50% and 60% (Citation23), always in the morning to minimize the interference of circadian rhythm.
For all visits, patients were instructed to: avoid consuming caffeine and alcohol for 24 hours before the procedures; eat a light meal before testing, and avoid physical exercise on the day before evaluations.
Cardiopulmonary exercise test
For aerobic exercise prescription, patients performed a cardiopulmonary exercise test on a treadmill (Inbrasport ATL 2000) with an initial velocity of 2.0 km hour−1, a constant inclination of 3%, and 0.5 km hour−1 increments every 2 minutes. The test was performed until voluntary exhaustion (Citation24, Citation25).
In addition, ventilatory variables were obtained using a gas analyzer (VO2000, Medical Graphics, USA). The medium airflow was used in all tests and gas samples were taken every 10 seconds (Aerograph®, Michegan, USA).
The speed corresponding to the peak VO2 (vVO2peak) was taken as the highest intensity reached during the test. In the case of the patient reaching exhaustion before the end of the stage, vVO2peak was adjusted by the equation proposed by Kuipers et al. (Citation26).
Aerobic exercise session
The aerobic exercise session was performed at two intensities: protocols at 60% of vVO2peak and at 90% of vVO2peak. The exercise sessions were carried out on different days with an interval of two days. The duration of exercise was 30 minutes at 60% of the vVO2peak, while at 90% of the vVO2peak, exercise was performed until patient voluntary exhaustion, since the participants could not perform 30 minutes of aerobic exercise at this intensity.
Heart rate variability (HRV)
For the HRV analysis, patient heart rate (HR) was recorded beat by beat using a Polar S810i heart rate monitor (Polar Electro, Kempele, Finland) (Citation27).
An elastic catchment strap was placed on the chest of the volunteer, at the height of the xiphoid process, and a heart rate receiver on their wrist (Polar Electro, Kempele, Finland). HR was recorded with the patients in the sitting position for 20 minutes, during exercise performance, and during recovery. The assessment of HRV was performed on two separate days for each exercise performed.
For HRV indices analysis, 256 consecutive RR intervals were used, selected from the most stable five minutes of the following times: rest, during exercise, immediately after (IA), and five, 10, and 15 minutes after exercise; which were submitted to digital filtering using Polar Precision Performance SW software, complemented by manual filtering for elimination of premature ectopic beats and artifacts. Only series with more than 95% of sinus beats were included in the study (Citation28, Citation29).
The indices calculated in the time and frequency domains are presented in . Kubios software version 2.033 was used to calculate these indices (Citation30).
Table 1. Clinical representation of HRV indices in time domain and frequency domain.
Statistical analysis
For the population profile data analysis, the descriptive statistical method was used and the results are presented as mean, standard deviation, median, and 25–75% interquartile range values. Data normality was assessed by the Shapiro–Wilk test.
Comparisons of HRV indices between protocols and time intervals were performed by the technique of analysis of variance for repeated measures in the two-factor model. The repeated measurement data were checked for violations of sphericity using the Mauchly test and the Greenhouse–Geisser correction was used when sphericity was violated.
The Bonferroni post-test for parametric distribution or Dunnett's post-test for nonparametric distribution was used for the times analysis (rest vs. immediately after, and 5, 10, and 15 minutes after). Statistical significance was set at 5% for all analyzes and calculations performed using the software SPSS - version 22 (SPSS Inc., Chicago, IL, USA).
Results
Twenty-seven patients with COPD were included in this study (Stage I n = 1, Stage II n = 11, Stage III n = 12, and Stage IV n = 3). The anthropometric and spirometric data of the patients are presented in . No patient presented clinical and/or electrocardiography events that prevented completion of the tests.
Table 2. Anthropometric, spirometric data and aerobic parameters of the participants.
In the comparison analysis between the two groups (Moderate and Severe) there were no significant differences in any of the HRV indices at the different intensities applied, i.e., both groups showed similar behavior, both during the exercise at 60% and 90% of vVO2peak and during recovery.
In the SDNN analysis, which represents the sympathetic and parasympathetic activity (overall variability), the Moderate Group at 60% presented an increase immediately after the exercise (p < 0.0001) and at 90% there was a decrease during exercise (p = 0.0002). The Severe Group at 60% presented an increase immediately after the exercise (p < 0.0001) and at 90% of vVO2peak a decrease during exercise (p < 0.0001) was observed, and .
Figure 1. Behavior of SDNN (A, B) and RMSSD (C, D) during aerobic exercise and recovery period in 60% and 90% of vVO2peak intensities. *Significant difference compared to rest (p < 0.05). Values are expressed as mean ± standard deviation. EX, during exercise; IA, immediately after exercise; 5, five minutes after exercise; 10, ten minutes after exercise; 15, fifteen minutes after exercise.
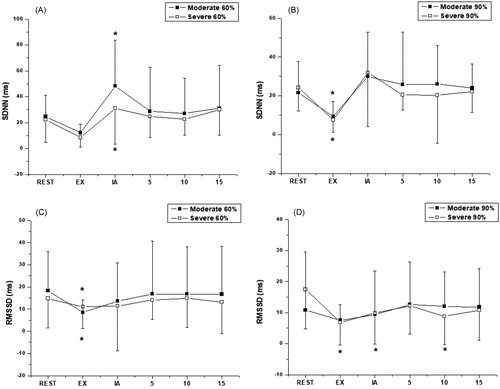
For the RMSSD index, which represents parasympathetic activity, in the analysis of exercise intensity at 60% of vVO2peak in the Moderate Group, a significant decrease was observed during exercise (p < 0.0001) compared to baseline. As for the intensity at 90% of vVO2peak there were no significant differences (p < 0.1868). In the Severe Group at the intensity of 60% vVO2peak a significant decrease occurred in this index during exercise (p < 0.0363) and at 90% there was a decrease during exercise, immediately after, and 15 minutes after exercise compared to baseline (p = 0.0001), and and .
Table 3. Behavior of RMSSD and SDNN during aerobic exercise and recovery period in 60% and 90% of vVO2peak intensities.
In the Moderate Group, the LF index in ms, which reflect the combined action of vagal and sympathetic components of the heart with sympathetic predominance, there was an increase at 15 minutes after exercise at 60% of vVO2peak (p < 0.0001). At 90% in the same group, there was a decrease during exercise compared to baseline (p < 0.0001). The Severe Group at 60% intensity showed a decrease in this index during exercise when compared to baseline (p < 0.0001), which also occurred at the intensity of 90%vVO2peak (p < 0.0001), and .
Figure 2. Behavior of LF (ms) (A, B) and HF (ms) (C, D) during aerobic exercise and recovery period in 60% and 90% of vVO2peak intensities. *Significant difference compared to rest (p < 0.05). Values are expressed as mean ± standard deviation. EX, during exercise; IA, immediately after exercise; 5, five minutes after exercise; 10, ten minutes after exercise; 15, fifteen minutes after exercise.
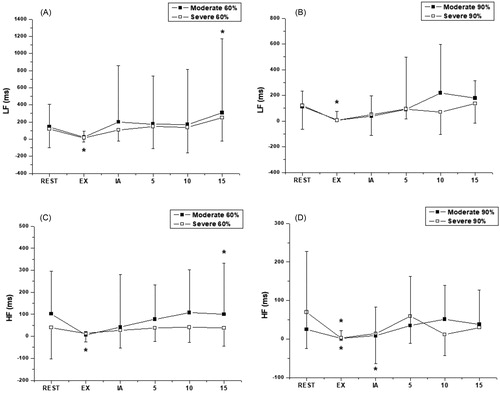
In the HFms2 index, which corresponds to the respiratory modulation and is an indicator of the performance of the vagus nerve on the heart, at the intensity of 60% of vVO2peak, for the Moderate Group, we observed a significant increase at 15 minutes after exercise compared to baseline (p < 0.0001). At the higher intensity there was a decrease in this index during exercise compared to baseline (p = 0.0065), which also occurred at the 60% intensity in the Severe Group (p < 0.0001). At 90% there was also decreased parasympathetic activity during exercise and immediately after exercise (p < 0.0001), and and .
Table 4. Behavior of LFms2 and HFms2, respectively during aerobic exercise and recovery period in 60% and 90% of vVO2peak intensities.
No significant differences were detected in LF in normalized units. Regarding the HF index in normalized units, we did not observe significant differences, except for a decrease at 10 minutes after exercise at 90% in the Severe Group (p < 0.0001). The LF/HF ratio showed no significant differences ().
Table 5. Behavior of LFnu, HFnu, and LF/HF ratio, respectively during aerobic exercise and recovery period in 60% and 90% of vVO2peak intensities.
Discussion
The results of this study demonstrated that the performance of aerobic sessions in patients with COPD influenced HRV indices at the analyzed moments. Differences were observed during exercise and recovery in relation to rest. Furthermore, there was no difference between the protocols in either moment or protocol interactions. In addition, no differences were found when comparing moderate and severe COPD.
During exercise, in both COPD stages, in both protocols, RMSSD and HFms2, which indicate parasympathetic modulation (Citation8), decreased compared to rest. These values were lower in the protocol at 90% of vVO2peak, suggesting that, during exercise, the inhibition of parasympathetic response in these patients is related to the intensity of the performed effort. This behavior represents alterations of higher proportions during a protocol of higher intensity, regardless of the severity of the disease.
The SDNN index, which represents the overall variability, as well as the LFms index, which reflects predominantly sympathetic modulation, also decreased during exercise at both intensities, this response was not influenced by disease severity. An increase in heart rate during exercise due to increased metabolic demand has been associated with decreased HRV as a result of reduced vagal activity during exercise (Citation31).
Martinmaki et al. (Citation16) also observed a reduction in LFms2 and HFms2 values during aerobic exercise of low and high intensity in healthy subjects, and this decrease probably reflects a reduction in vagal activity in response to increased metabolic demand. Regarding exercise intensity, previous studies (Citation31, Citation32) have also observed higher values of LFms2 and HFms2 during lower compared to higher intensity exercise.
However, when analyzing LF and HF indices in normalized units, we found no significant decrease during exercise. Previous studies have demonstrated that LF and HF, expressed in absolute values, decrease during exercise (Citation31–33), while the results for normalized LF and HF have been inconsistent (Citation32, Citation34–36). Casadei et al. (Citation32) observed, in 11 healthy men, that the spectral component of high frequency (HF) decreased at the beginning of exercise, consistent with a reduction in vagal activity. Moreover, the normalized units increased with the intensity of exercise and therefore, do not adequately reflect the autonomic changes during exercise. Our findings corroborate previous studies (Citation16, Citation37) suggesting that LF(nu) and HF(nu) do not indicate sympathetic and vagal outflow during exercise.
Vagal participation influences the majority of HRV indices and can be well assessed almost exclusively through the HF component and the spectral analysis using time domain indices, such as RMSSD, while sympathetic participation influences the low frequency (LF) component, which is less evident in the time domain. This fact demonstrates the importance of using the frequency domain indices for the analysis of HRV.
After the end of the exercise, parasympathetic modulation, reflected by RMSSD and HFms2, immediately increased in both protocols. These values were lower in the 90% of vVO2peak protocol, suggesting slower recovery after higher intensity exercise, although no significant differences were observed between the protocols or between the COPD stages. During recovery, there was also a decrease in the HF (nu) index compared to rest.
These results corroborate the findings of Casonatto et al. (Citation17), who evaluated a session of aerobic exercise in healthy subjects. The authors observed that in higher intensity exercise, the parasympathetic recovery, represented by RMSSD, seems to occur later and that sympathetic withdrawal compensates this potential delay in recovery. A similar response was observed in the study of Kaikkonen et al. in sedentary women (Citation38) and Gladwell et al. in untrained young adults (Citation39). Guiraud et al. (Citation40) compared the effects of a high-intensity interval exercise (HIIE) protocol and moderate-intensity continuous exercise (MICE) on ANS activity in chronic heart failure patients. The authors observed that HF power values remained higher after HIIE compared with MICE at the 24th hour. The HR reduction post-HIIE exercise was directly correlated with vagal modulation enhancement as assessed by an increase in HF power, suggesting that beneficial effects on HRV are probably related to the mode and intensity of HIIE.
Studies have shown the effects of resistance exercise on post-exercise recovery; a single session on cardiac autonomic modulation in subjects with COPD. Nicolino et al. (Citation19) observed that the HFms2 index remained raised throughout the 15 min of recovery from exercise at both 60% and 90% of one maximum repetition in subjects with COPD. In addition, there was no statistical difference between the training protocols, which showed that the recovery of autonomic modulation in this population was similar regardless of the exercise intensity. Vanderlei et al. (Citation18) observed, through the behavior of the RMSSD index, that the initial 5 minutes of recovery demonstrated a decrease in values in relation to rest. These findings indicate sympathetic modulation caused acutely by resistance training, as these values approached baseline conditions at other moments, demonstrating a reactivation of parasympathetic modulation in subjects with COPD.
The slower recovery of HRV may be related to increased sympathetic activity after high-intensity exercise, which, in a previous study (Citation41), was suggested to occur during the first minutes after exercise cessation. In addition, a sympathoadrenal response to maximal exercise may induce a six-fold increase in the norepinephrine levels from sympathetic nerve terminals, which influences the cardiovascular adjustments during and immediately after high-intensity exercise (Citation42).
The SDNN index, which indicates the overall variability, that is, sympathetic and parasympathetic modulation (Citation8), was increased after exercise in the 60% of vVO2peak protocol compared to rest. Possible mechanisms that promote immediate changes in cardiac function after exercise include quick changes in pre-load, post-load, and heart contractility (Citation43, Citation44). Together with the loss of central control and baroreflex activation, these mechanisms contribute to fast vagal reactivation, which can be observed by an increase in RMSSD and HFms2 after exercise, leading to HR recovery and HRV increase (Citation31, Citation45, Citation46), and may be related to the observed increase in SDNN.
The LFms2 and LF(nu) increased in the recovery period at both intensities and this response was not influenced by disease severity. Increased sympathetic activity after exercise has been described in the literature. Nicolino et al. (Citation19) observed that the LFms2 index remained raised during 15 min of recovery in subjects with COPD after resistance exercise at both 60% and 90% of one maximum repetition. Studies have found that when the intensity increases, HF remains lower and LF and the LF/HF ratio are higher in comparison with baseline (Citation47, Citation48). This tendency reflects the slow return of sympathetic activity to baseline (Citation35, Citation49), which were not achieved within 5 minutes (Citation35), 9 minutes (Citation31), or even 1 hour (Citation47, Citation48) after the end of exercise, depending on its intensity and duration. Furlan et al. (Citation41) performed a single session of maximal exercise in athletes and observed LF predominance 24 hours after exercise.
Regarding the LF/HF ratio, which provides information about the sympathetic and vagal balance, it was not possible to identify differences in either the exercise or recovery periods. These findings corroborate the study of Casonatto et al. (Citation17) in healthy subjects where the LF/HF ratio presented no statistically significant differences after exercise.
This study has some limitations that should be considered. The exercise sessions did not have the same duration. Additionally, patients' respiratory rate was not controlled during HR capitation, which may have influenced the HF index. Furthermore, there is controversy in the literature regarding the alleged predominance of sympathetic activity quantified in LF (Citation50), therefore, further studies are needed to confirm the changes in sympathetic activity. Another limitation of the study is the absence of data regarding the measurement of arterial oxygen saturation in the protocols performed, which may have an impact on HRV (Citation51), and which was used only for monitoring individuals with COPD during exercise.
This study is a useful clinical tool for cardiorespiratory professionals, since the results indicate that monitoring of HRV indices, a noninvasive and inexpensive method, can be useful to evaluate the clinical manifestations of the disease and verify the effects of physical exercise on the ANS, which is a widely used therapeutic in pulmonary rehabilitation protocols for these patients.
In addition, the study has great clinical relevance, since we observed that the severity of COPD did not influence the response of ANS during exercise at different intensities, or in the moments of recovery. It can be observed in this sample that the higher intensity protocols (90% vVO2peak) promoted more evident alterations in the autonomic modulation than the severity of the disease.
These results may contribute to the clinical practice of professionals working in rehabilitation and exercise programs, highlighting the importance of considering the behavior of ANS at the highest exercise intensity, since patients can present autonomic alterations of greater magnitude and may require a longer recovery period, although in this study no significant differences were observed between the protocols. This fact should be taken into consideration when prescribing exercises and their respective moments of recovery, since an increase in sympathetic modulation in patients with COPD is associated with a higher rate of morbidity and mortality (Citation52) and low levels of parasympathetic reactivation in the recovery period can be considered an important risk factor for cardiac mortality (Citation53).
Conclusion
Single sessions of aerobic exercise influence the autonomic modulation in patients with COPD. However, COPD severity did not influence ANS responses to exercise and recovery moments; and there was no difference between the protocols.
Declaration of interest
The authors report no conflicts of interest.
Additional information
Funding
References
- Chhabra SK, De S. Cardiovascular autonomic neuropathy in chronic obstructive pulmonary disease. Respir Med. 2005;99(1):126–33. doi:10.1016/j.rmed.2004.06.003.
- Volterrani M, Scalvini S, Mazzuero G, Lanfranchi P, Colombo R, Clark AL, Levi G. Decreased heart rate variability in patients with chronic obstructive pulmonary disease. Chest. 1994;106(5):1432–7. doi:10.1378/chest.106.5.1432.
- Andreas S, Anker SD, Scanlon PD, Somers VK. Neurohumoral activation as a link to systemic manifestations of chronic lung disease. Chest. 2005;128(5):3618–24. doi:10.1378/chest.128.5.3618.
- Notarius CF, Ando S, Rongen GA, Floras JS. Resting muscle sympathetic nerve activity and peak oxygen uptake in heart failure and normal subjects. European Heart Journal. 1999;20(12):880–7. doi:10.1053/euhj.1998.1447.
- Haarmann H, Folle J, Nguyen XP, Herrmann P, Heusser K, Hasenfuss G, Andreas S, Raupach T. Sympathetic activation is associated with exercise limitation in COPD. COPD. 2016;13(5):589–94. doi:10.3109/15412555.2015.1136272.
- Sztajzel J. Heart rate variability: a noninvasive electrocardiographic method to measure the autonomic nervous system. Swiss Med Wkly. 2004;134(35–36):514–22. doi:10.4414/smw.2004.10321.
- Tukek T, Yildiz P, Atilgan D, Tuzcu V, Eren M, Erk O, Demirel S, Akkaya V, Dilmener M, Korkut F. Effect of diurnal variability of heart rate on development of arrhythmia in patients with chronic obstructive pulmonary disease. Int J Cardiol. 2003;88(2–3):199–206. doi:10.1016/S0167-5273(02)00402-3.
- Vanderlei LC, Pastre CM, Hoshi RA, Carvalho TD, Godoy MF. Basic notions of heart rate variability and its clinical applicability. Rev Bras Cir Cardiovasc. 2009;24(2):205–17. doi:10.1590/S0102-76382009000200018.
- Lombardi F. Clinical implications of present physiological understanding of HRV components. Card Electrophysiol Rev. 2002;6(3):245–9.
- Camillo CA, Pitta F, Possani HV, Barbosa MV, Marques DS, Cavalheri V, Probst VS, Brunetto AF. Heart rate variability and disease characteristics in patients with COPD. Lung. 2008;186(6):393–401. doi:10.1007/s00408-008-9105-7.
- Goldberger JJ, Le FK, Lahiri M, Kannankeril PJ, Ng J, Kadish AH. Assessment of parasympathetic reactivation after exercise. Am J Physiol Heart Circ Physiol. 2006;290(6):H2446–52. doi:10.1152/ajpheart.01118.2005.
- Hautala AJ, Makikallio TH, Kiviniemi A, Laukkanen RT, Nissila S, Huikuri HV, Tulppo MP. Cardiovascular autonomic function correlates with the response to aerobic training in healthy sedentary subjects. Am J Physiol Heart Circ Physiol. 2003;285(4):H1747–52. doi:10.1152/ajpheart.00202.2003.
- Cheng ST, Wu YK, Yang MC, Huang CY, Huang HC, Chu WH, Lan CC. Pulmonary rehabilitation improves heart rate variability at peak exercise, exercise capacity and health-related quality of life in chronic obstructive pulmonary disease. Heart Lung. 2014;43(3):249–55. doi:10.1016/j.hrtlng.2014.03.002.
- Borghi-Silva A, Mendes R, Trimer R, Oliveira C, Fregonezi G, Resqueti V, Arena R, Sampaio-Jorge L, Costa D. Potential effect of 6 versus 12-weeks of physical training on cardiac autonomic function and exercise capacity in chronic obstructive pulmonary disease. Eur J Phys Rehabil Med. 2015;51(2):211–21.
- Coote JH. Recovery of heart rate following intense dynamic exercise. Exp Physiol. 2010;95(3):431–40. doi:10.1113/expphysiol.2009.047548.
- Martinmaki K, Rusko H. Time-frequency analysis of heart rate variability during immediate recovery from low and high intensity exercise. Eur J Appl Physiol. 2008;102(3):353–60. doi:10.1007/s00421-007-0594-5.
- Casonatto J, Tinucci T, Dourado AC, Polito M. Cardiovascular and autonomic responses after exercise sessions with different intensities and durations. Clinics (Sao Paulo). 2011;66(3):453–8. doi:10.1590/S1807-59322011000300016
- Vanderlei FM, Zandonadi F, de Lima FF, Silva BSA, Freire A, Ramos D, Ramos EMC. Acute effects of different types of resistance training on cardiac autonomic modulation in COPD. Respir Care. 2018;63(8):1050–9. doi:10.4187/respcare.05898.
- Nicolino J, Ramos D, Leite MR, Rodrigues FM, de Alencar Silva BS, Tacao GY, de Toledo AC, Vanderlei LC, Ramos EM. Analysis of autonomic modulation after an acute session of resistance exercise at different intensities in chronic obstructive pulmonary disease patients. Int J Chronic Obstr Pulm Dis. 2015;10:223–9. doi:10.2147/copd.s64345.
- Vestbo J, Hurd SS, Agusti AG, Jones PW, Vogelmeier C, Anzueto A, Barnes PJ, Fabbri LM, Martinez FJ, Nishimura M, et al. Global strategy for the diagnosis, management, and prevention of chronic obstructive pulmonary disease: GOLD executive summary. Am J Respir Crit Care Med. 2013;187(4):347–65. doi:10.1164/rccm.201204-0596PP.
- Lohman T, Roache A, Martorell R. Anthropometric standardization reference manual. Med Sci Sports Exerc. 1992;24(8):952.
- Miller MR, Hankinson J, Brusasco V, Burgos F, Casaburi R, Coates A, Crapo R, Enright P, van der Grinten CP, Gustafsson P, et al. Standardisation of spirometry. Eur Respir J. 2005;26(2):319–38. doi:10.1183/09031936.05.00034805.
- Borghi-Silva A, Arena R, Castello V, Simoes RP, Martins LE, Catai AM, Costa D. Aerobic exercise training improves autonomic nervous control in patients with COPD. Respir Med. 2009;103(10):1503–10. doi:10.1016/j.rmed.2009.04.015.
- Borghi-Silva A, Baldissera V, Sampaio LM, Pires-DiLorenzo VA, Jamami M, Demonte A, Marchini JS, Costa D. L-carnitine as an ergogenic aid for patients with chronic obstructive pulmonary disease submitted to whole-body and respiratory muscle training programs. Braz J Med Biol Res. 2006;39(4):465–74. doi:10.1590/S0100-879X2006000400006.
- Kendrick KR, Baxi SC, Smith RM. Usefulness of the modified 0–10 Borg scale in assessing the degree of dyspnea in patients with COPD and asthma. J Emerg Nurs. 2000;26(3):216–22.
- Kuipers H, Verstappen F, Keizer H, Geurten P, Van Kranenburg G. Variability of aerobic performance in the laboratory and its physiologic correlates. Int J Sports Med. 1985;6(4):197–201. doi:10.1055/s-2008-1025839.
- Vanderlei LC, Silva RA, Pastre CM, Azevedo FM, Godoy MF. Comparison of the Polar S810i monitor and the ECG for the analysis of heart rate variability in the time and frequency domains. Braz J Med Biol Res. 2008;41(10):854–9. doi:10.1590/S0100-879X2008005000039.
- Heart rate variability: standards of measurement, physiological interpretation and clinical use. Task Force of the European Society of Cardiology and the North American Society of Pacing and Electrophysiology. Circulation. 1996;93(5):1043–65.
- Godoy M, Takakura I, Correa P. The relevance of nonlinear dynamic analysis (Chaos theory) to predict morbidity and mortality in patients undergoing surgical myocardial revascularization. Arq Ciênc Saúde. 2005;12:167–71.
- Tarvainen MP, Niskanen JP, Lipponen JA, Ranta-Aho PO, Karjalainen PA. Kubios HRV – heart rate variability analysis software. Comput Methods Programs Biomed. 2014;113(1):210–20. doi:10.1016/j.cmpb.2013.07.024.
- Arai Y, Saul JP, Albrecht P, Hartley LH, Lilly LS, Cohen RJ, Colucci WS. Modulation of cardiac autonomic activity during and immediately after exercise. Am J Physiol. 1989;256(1 Pt 2):H132–41. doi:10.1152/ajpheart.1989.256.1.H132.
- Casadei B, Cochrane S, Johnston J, Conway J, Sleight P. Pitfalls in the interpretation of spectral analysis of the heart rate variability during exercise in humans. Acta Physiol Scand. 1995;153(2):125–31. doi:10.1111/j.1748-1716.1995.tb09843.x.
- Pichon AP, de Bisschop C, Roulaud M, Denjean A, Papelier Y. Spectral analysis of heart rate variability during exercise in trained subjects. Med Sci Sports Exerc. 2004;36(10):1702–8. doi:10.1249/01.MSS.0000142403.93205.35.
- Bernardi L, Salvucci F, Suardi R, Solda PL, Calciati A, Perlini S, Falcone C, Ricciardi L. Evidence for an intrinsic mechanism regulating heart rate variability in the transplanted and the intact heart during submaximal dynamic exercise? Cardiovasc Res. 1990;24(12):969–81.
- Perini R, Orizio C, Baselli G, Cerutti S, Veicsteinas A. The influence of exercise intensity on the power spectrum of heart rate variability. Eur J Appl Physiol Occup Physiol. 1990;61(1–2):143–8.
- Warren JH, Jaffe RS, Wraa CE, Stebbins CL. Effect of autonomic blockade on power spectrum of heart rate variability during exercise. Am J Physiol. 1997;273(2 Pt 2):R495–502. doi:10.1152/ajpregu.1997.273.2.R495.
- Perini R, Milesi S, Fisher NM, Pendergast DR, Veicsteinas A. Heart rate variability during dynamic exercise in elderly males and females. Eur J Appl Physiol. 2000;82(1–2):8–15. doi:10.1007/s004210050645.
- Kaikkonen P, Nummela A, Rusko H. Heart rate variability dynamics during early recovery after different endurance exercises. Eur J Appl Physiol. 2007;102(1):79–86. doi:10.1007/s00421-007-0559-8.
- Gladwell VF, Sandercock GR, Birch SL. Cardiac vagal activity following three intensities of exercise in humans. Clin Physiol Funct Imaging. 2010;30(1):17–22. doi:10.1111/j.1475-097X.2009.00899.x.
- Guiraud T, Labrunee M, Gaucher-Cazalis K, Despas F, Meyer P, Bosquet L, Gales C, Vaccaro A, Bousquet M, Galinier M, et al. High-intensity interval exercise improves vagal tone and decreases arrhythmias in chronic heart failure. Med Sci Sports Exerc. 2013;45(10):1861–7. doi:10.1249/MSS.0b013e3182967559.
- Furlan R, Piazza S, Dell'Orto S, Gentile E, Cerutti S, Pagani M, Malliani A. Early and late effects of exercise and athletic training on neural mechanisms controlling heart rate. Cardiovasc Res. 1993;27(3):482–8.
- McArdle WD, Katch FI, Katch VL. Exercise physiology: nutrition, energy, and human performance. Philadelphia (PA): Lippincott Williams & Wilkins; 2010.
- Miles DS, Sawka MN, Hanpeter DE, Foster JE, Jr., Doerr BM, Frey MA. Central hemodynamics during progressive upper- and lower-body exercise and recovery. J Appl Physiol Respir Environ Exerc Physiol. 1984;57(2):366–70. doi:10.1152/jappl.1984.57.2.366.
- Plotnick GD, Becker LC, Fisher ML. Changes in left ventricular function during recovery from upright bicycle exercise in normal persons and patients with coronary artery disease. Am J Cardiol. 1986;58(3):247–51. doi:10.1016/0002-9149(86)90056-1.
- O'Leary DS. Autonomic mechanisms of muscle metaboreflex control of heart rate. J Appl Physiol (1985). 1993;74(4):1748–54. doi:10.1152/jappl.1993.74.4.1748.
- Oida E, Moritani T, Yamori Y. Tone-entropy analysis on cardiac recovery after dynamic exercise. J Appl Physiol (1985). 1997;82(6):1794–801. doi:10.1152/jappl.1997.82.6.1794.
- Terziotti P, Schena F, Gulli G, Cevese A. Post-exercise recovery of autonomic cardiovascular control: a study by spectrum and cross-spectrum analysis in humans. Eur J Appl Physiol. 2001;84(3):187–94. doi:10.1007/s004210170003.
- Hayashi N, Nakamura Y, Muraoka I. Cardiac autonomic regulation after moderate and exhaustive exercises. Ann Physiol Anthropol. 1992;11(3):333–8.
- Perini R, Orizio C, Comande A, Castellano M, Beschi M, Veicsteinas A. Plasma norepinephrine and heart rate dynamics during recovery from submaximal exercise in man. Eur J Appl Physiol Occup Physiol. 1989;58(8):879–83.
- Billman GE. The LF/HF ratio does not accurately measure cardiac sympatho-vagal balance. Front Physiol. 2013;4:26. doi:10.3389/fphys.2013.00026.
- Botek M, Krejci J, De Smet S, Gaba A, McKune AJ. Heart rate variability and arterial oxygen saturation response during extreme normobaric hypoxia. Auton Neurosci: Basic Clin. 2015;190:40–5. doi:10.1016/j.autneu.2015.04.001.
- Andreas S, Haarmann H, Klarner S, Hasenfuss G, Raupach T. Increased sympathetic nerve activity in COPD is associated with morbidity and mortality. Lung. 2014;192(2):235–41. doi:10.1007/s00408-013-9544-7.
- Albert CM, Mittleman MA, Chae CU, Lee IM, Hennekens CH, Manson JE. Triggering of sudden death from cardiac causes by vigorous exertion. N Engl J Med. 2000;343(19):1355–61. doi:10.1056/nejm200011093431902.