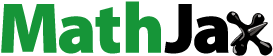
Abstract
We previously showed that functional electrical stimulation during cycle ergometry (FES-cycling) increased oxygen consumption (VO2), indicating that metabolism during exercise was increased. However, the effects on muscle oxygenation have never been studied. The aim of this secondary analysis was to analyse changes in muscle oxygenation during an FES-cycling session. Eight patients with chronic obstructive pulmonary disease who were participating in a pulmonary rehabilitation programme were enrolled. Each participant carried out 30 minutes of cycle ergometry with a constant load at 50% of peak oxygen uptake, either (i) with FES or (ii) without (Placebo-FES). Oxygenation of the vastus lateralis (VL) muscle over time was measured using near-infrared spectroscopy (NIRS) during both sessions. External power output on the cycle ergometer was the same in both conditions. There were no differences in dyspnoea between the groups, although the concentrations of deoxygenated haemoglobin and myoglobin (deoxy(Hb + Mb)) in the VL were significantly greater during Placebo-FES than FES-Cycling (respectively +212 ± 65% vs. +84 ± 29%; p < 0.001), as was the decrease in muscle oxygen saturation (StO2) (p < 0.001). When adjusted for VO2, there was a greater increase over time in the deoxy(Hb + Mb)/VO2 ratio during Placebo-FES than FES-cycling (p < 0.0001). FES-cycling could be a useful strategy to decrease muscular deoxy(Hb + Mb) and limit decreases in muscle StO2, however this should be confirmed in larger studies.
Introduction
In chronic obstructive pulmonary disease (COPD), the loss of respiratory function and associated muscle dysfunction reduce the capacity to carry out activities of daily living in affected individuals. Several authors reported gross reductions in the volume and strength of the quadriceps muscle, the number of capillaries within the muscle and its oxidative capacity, as well as changes in muscle fibre distribution.Citation1–3 Pulmonary rehabilitation programmes encourage patients to participate in physical exercise to improve physical exercise capacity and reduce exertional dyspnoea. Oxygen therapy and non-invasive ventilation are sometimes used to facilitate fitness training for patients with severe dyspnoea.Citation4,Citation5
In a previous study, we evaluated a new approach to facilitate physical exercise.Citation6 Rather than relieving dyspnoea by providing ventilatory support, we increased muscle work directly through electrical stimulation of the quadriceps, synchronised with pedalling movements on a cycle ergometer (FES-cycling). The results showed that FES-cycling increased oxygen consumption (VO2), indicating that exercise metabolism was increased. We hypothesised that this increase in VO2 was due to an increased recruitment of the quadriceps via the electrical stimulation. However, to our knowledge, no studies have yet investigated the effects of FES-cycling on muscle in individuals with COPD.
The aim of this secondary analysis was to compare changes in muscle oxygenation during training sessions with FES-cycling and with Placebo-FES. Muscle oxygenation during exertion was measured non-invasively, in real-time using near-infrared spectroscopy (NIRS).
Method
Design
This study involved analysis of secondary data from a study published by Medrinal et al.Citation6 The original study was a single-centre, cross-over, controlled, randomised and single-blind trial. Each participant completed two 30-minute cycle ergometer sessions (with functional electrical stimulation (FES-cycling) and without (Placebo-FES)). The session orders were randomised (by a co-worker who was not directly involved in the study) and placed into sealed envelopes for each participant. The investigator did not open these envelopes until just before the first session.Citation7
The original study was registered on ClinicalTrials.gov (NCT02594722) and the present work required a modification to the initial protocol, which was granted by our local ethics committee (Comité de Protection des Personnes Nord-Ouest III). The modification involved the addition of muscle oxygenation analysis for each of the last ten participants included in the study. All participants provided written consent, in accordance with the Declaration of Helsinki, and the study was conducted, analysed and reported according to the Consolidation Standards of Reporting Trials (CONSORT) statement for randomised controlled trials.
Population
Participants were recruited from patients enrolled in a pulmonary rehabilitation programme in the Groupe Hospitalier du Havre. The inclusion criterion was stage 2, 3 or 4 COPD, according to the GOLD classification. Exclusion criteria were: exacerbation of respiratory function less than four weeks previously, implanted with a pacemaker, contraindications to either functional electrical stimulation or to undertaking 30 minutes of pedalling, or an inability to pedal.
Interventions
Before participating in the study (during the 48 hours before the first session), a pre experimental exercise test was carried out to titrate the external power output that corresponded to 50% of the peak VO2 achieved during the incremental exercise testing. This external power output was then used in both conditions.
Each session of cycle ergometry began with a 2-minute warm-up. Participants were then instructed to maintain a frequency of 50–60 rpm for 30 minutes. The cycle ergometer used was a MotoMed Letto II® (RECK-Technik, Germany). A 30-minute break was allowed between the cycle ergometry sessions in order to ensure that cardio-respiratory and muscle function returned to their baseline states.
FES-cycling
Functional electrical stimulation was applied and coordinated with the cycling using a stimulator that was relayed to a computer (RehaMove®, Hasomed, Germany). For all participants, the electrical stimulation was set to a frequency of 35 Hz and impulse length of 300 μs, in accordance with conventional electrotherapy protocols.Citation8 Rectangular RehaMove® electrodes (5 × 9 cm) were placed at each extremity of both quadriceps muscles (total of four electrodes). They were used to produce an intermittent, rectangular current with sufficient intensity to induce a visible or palpable muscle contraction that was tolerable for the participant.
Placebo-FES
For the placebo sessions, the conditions were the same as for the FES-cycling: both used the same electrical stimulation device and cycle ergometer and the electrodes remained in situ during both sessions, regardless of the session order. However, in the placebo condition, the electrical stimulation was set to a frequency of 2Hz and impulse length of 300 μs, with an intensity that was too low to induce a contraction.Citation9
Measurements
During each cycle ergometry session, muscle oxygenation was measured on the vastus lateralis (VL) muscle using a commercially available NIRS device (Portamon, Artinis Medical Systems, The Netherlands). The Portamon device was a continuous-wave system, which simultaneously used the modified Beer-Lambert and spatially resolved spectroscopy methods. This system was composed of three optodes (positioned 30, 35 and 40 mm from the receptor) that emitted two different wavelengths of light (760 and 850 nm) which were captured by a receptor. The maximum penetration depth for this device is 20 mm. In biological tissue, diffusion is an important phenomenon. The actual length of the optical trajectory may be greater than the physical distance between the emitter and the detector. In order to account for the longer optical trajectory, a correction factor is incorporated. This factor, called differential pathlength factor (DPF), accounts for the increase in optical pathlength due to scattering in the tissue. A DPF value of 4 was used in the present study. The NIRS device was fixed using an elastic bandage to minimise movement during cycling and the bandage was black to avoid light-related errors.Citation10
The signal emitted from the optodes was captured at a frequency of 1 Hz and measured the concentration of (1) haemoglobin (Hb) and myoglobin (Mb) oxygenated (oxy(Hb + Mb)), (2) Hb + Mb deoxygenated (deoxy(Hb + Mb)) and (3) total haemoglobin and myoglobin (Total(Hb + Mb)) in the area of interrogation. Total(Hb + Mb) is the sum of oxy(Hb + Mb) and deoxy(Hb + Mb). This represents the volume of blood in the part of the muscle evaluated by the NIRS.Citation11,Citation12 The tissue saturation index provided an estimate of the oxygen saturation of the tissue (StO2) defined as:
StO2 = 100×(oxy(Hb + Mb)/total(Hb + Mb).
The contribution of Hb and Mb in vivo to the NIRS signal is not well known for muscle oxygenation, which made the data difficult to interpret. Absorption by Hb or Mb in large vessels is high. NIRS therefore provides information regarding oxygen status, particularly in the small blood vessels (arterioles, capillaries and venules) and myocytes which absorb NIRS to a lesser extent.
The concentrations of oxy(Hb + Mb), deoxy(Hb + Mb) and total(Hb + Mb) were expressed in arbitrary units (A.U) by the NIRS. To facilitate intra- and inter-participant comparisons, changes in oxy(Hb + Mb), deoxy(Hb + Mb) and total(Hb + Mb) during exertion were converted into percentage change from the participant’s baseline values. StO2 (ratio of oxy(Hb + Mb) to total(Hb + Mb)) was expressed as an absolute %. StO2 was expressed as the Δ of change from baseline.
Participants also wore a face mask relayed to an infrared gas analyser to measure breath-by-breath gas exchange (Vmax Vyntus™ CPX, Carefusion) during both sessions.
Primary outcome
The primary outcome measure was the change in oxygenation of the VL muscle over time in comparison to baseline values (ΔDeoxy(Hb + Mb)) during both cycling sessions. We chose to analyse change in deoxy(Hb + Mb) for the following reasons: (1) In contrast with oxy(Hb + Mb) and total(Hb + Mb), changes in deoxy(Hb + Mb) are relatively insensitive to alterations in skin blood volume or increases in flow during exercise. Changes in deoxy(Hb + Mb) are often associated with the change in deoxygenation of the muscle under the sensor. (2) Changes in deoxy(Hb + Mb) are often considered as a non-invasive means of measuring microvascular extraction of O2.Citation13 Nevertheless, this depends on certain factors: first the balance between O2 delivery (QO2) and O2 uptake (VO2); and second, changes in haematocrit, capillary–venous haem concentration, and/or volume of small blood vessels. Variations in these factors can reduce the association between deoxy(Hb + Mb) and the microvascular extraction of O2. Nevertheless, it appears that the effect of these factors is reduced during exertion, probably because muscle contractions limit vascular dilatation and microvascular hemoconcentration.Citation14
Secondary outcomes
Secondary outcome measures were changes in Oxy(Hb + Mb), Total(Hb + Mb) and StO2 of the VL muscle during exertion (compared with baseline).
The kinetics of deoxy(Hb + Mb) (time constant, amplitude, plateau) were analysed for both cycling sessions. The change in deoxy(Hb + Mb) was measured each second. The time courses were modelled using the following non-linear functionCitation15,Citation16:
Here “deoxy(Hb + Mb)rest” represented the initial level of deoxy(Hb + Mb) at rest, “deoxy(Hb + Mb)ss” represented the steady state of deoxy(Hb + Mb) during exertion, and τ (time constant) represented the time course of the monoexponential deoxy(Hb + Mb) curve. The amplitude of the deoxy(Hb + Mb) (deoxy(Hb + Mb)ampl) corresponds to the difference between deoxy(Hb + Mb)ss and deoxy(Hb + Mb)rest.
Cardiac frequency (CF), dyspnoea (as measured on the modified Borg scale from 0 to 10), lower limb fatigue (VAS 0–10) and oxygen consumption (VO2) were also evaluated. The deoxy(Hb + Mb)/VO2 ratio was calculated to normalise the variations in muscle oxygen as a function of VO2.Citation17 This ratio represents a balance between the excess oxygen extracted and O2 consumption.
Statistical analysis
Data are expressed in numbers and percentages for categorical variables and as means and standard deviations for continuous, quantitative variables. The normality of the distribution of each variable analysed was evaluated using the Standardised Skewness and Kurtosis scores. Mean or median values during exertion were analysed using a paired Student t-test or a Wilcoxon matched pair signed rank test. When significant differences were found, oxygen concentration kinetics were modelled using non-linear models. The time courses of change in the deoxy(Hb + Mb)/VO2 ratio were compared using a Two-Way Anova. The decline in StO2 from baseline was analysed at 25, 50, 75 and 100% of exercise time and compared using a Two-Way Anova. All analyses were carried out using GraphPad Prism 5 software. A p value <0.05 was considered significant.
Results
Data for FES-cycling and Placebo-FES sessions from eight participants were analysed. Originally ten subjects were eligible for participation, but one withdrew consent on the day of the test and a technical issue rendered another participant’s NIRS signal uninterpretable.
The mean age of the participants was 60 years, the mean FEV1 was 52% of the predicted value and the mean distance walked on the 6MWT was 81% of the predicted distance (see ).
The results for the metabolic parameters, dyspnoea and lower limb fatigue are provided in .
Table 2. Differences in physiological variables
The Δdeoxy(Hb + Mb) was significantly greater for the placebo intervention compared with FES-Cycling (respectively +212 ± 65% vs. +84 ± 29%; p < 0.001).
The kinetics of deoxy(Hb + Mb) concentrations are shown by a non-linear regression in . Changes in amplitude of the deoxy(Hb + Mb) over time were significantly greater for the placebo intervention compared with FES-Cycling (deoxy(Hb + Mb)ampl placebo vs. deoxy(Hb + Mb)ampl FES, respectively 294 (95% CI 284–304) % vs. 113 (95% CI 106–119) %; p < 0.01). No significant differences were found for the kinetics time constant of deoxy(Hb + Mb) between the interventions (see ). Individual patient data and R square values are presented in Supplemental Data and .
Figure 1. Comparison of the kinetics of oxygen extraction between FES-cycling (grey line) and Placebo-FES (black line). There was no significant difference in Deoxy (Hb + Mb) time constant kinetics between groups. There was a significant difference between the amplitude of oxygen extraction (p < 0.01); Change in deoxy(Hb + Mb) over time is modelled by a non-linear regression).
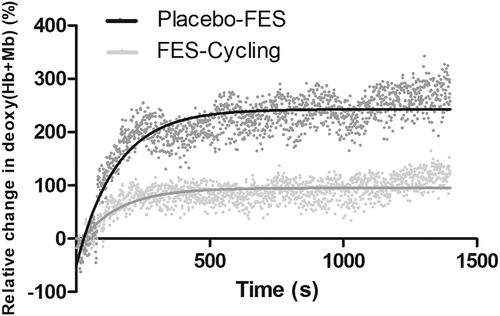
When adjusted for VO2, the two-way ANOVA revealed a greater increase over time in the deoxy(Hb + Mb)/VO2 ratio for the Placebo-FES intervention than for FES-cycling (p < 0.0001) (see ).
Figure 2. Comparison of Deoxy(Hb + Mb) as a function of oxygen consumption between FES-Cycling (solid line) and Placebo-FES (dashed line). (A) The grey area represents the warm-up period and the white area shows the active phase (50% of the VO2 peak). (B) The Deoxy(Hb + Mb)/VO2 ratio was analysed at 25, 50, 75 and 100% of exercise time. The significant increase in the ratio in the Placebo-FES group shows an excess in oxygen extraction for a given VO2 ((p < 0.0001); the difference between conditions over time was analysed using two-way ANOVA).
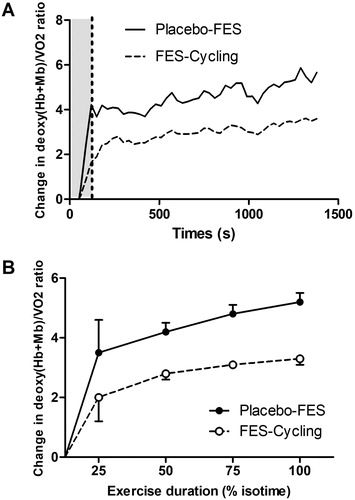
With regard to the other NIRS variables, there was no significant difference between the interventions for the Δtotal(Hb + Mb) of Δoxy(Hb + Mb) during exertion, however StO2 decreased significantly during the placebo intervention (p < 0.01) (see ).
Figure 3. Comparison of tissue saturation (StO2) of the VL muscle during FES-Cycling (dashed line) and Placebo-FES (solid line). (A) The grey area represents the warm-up period and the white area shows the active phase (50% of the VO2 peak). (B) The decline from baseline of StO2 was analysed at 25, 50, 75 and 100% of exercise time. ΔStO2 of the VL during exertion was significantly lower during Placebo-FES (p < 0.0001). The difference between the two interventions over time was analysed by a two-way ANOVA).
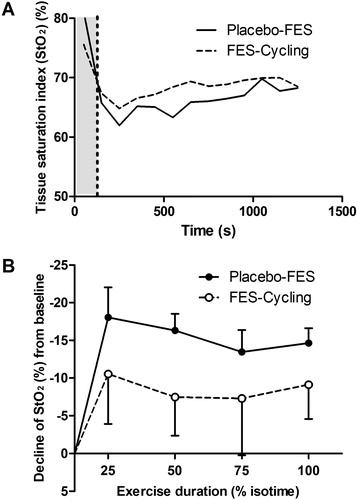
Discussion
This study is the first to evaluate the effects of FES-cycling on skeletal muscle oxygenation in individuals with COPD. Muscle and tissue deoxygenation (deoxy(Hb + Mb)) occurred to a significantly lower extent during the FES-Cycling sessions compared with the FES-Placebo (+84 ± 29% vs +212 ± 65%; respectively, p < 0.001), although there was no concomitant change in kinetics.
This smaller increase in deoxy(Hb + Mb) could reflect a lower level of voluntary muscle activity during the FES-cycling sessions. This idea is supported by several facts: firstly, the VL muscle (from which the NIRS measurements were taken) is mainly active during the extension phase of cycling (1st quadrant).Citation18 Changes in deoxy(Hb + Mb) are often associated with the microvascular extraction of O2, thus this is suggestive of less voluntary muscle activity.Citation13 Secondly, there was a significant decrease in StO2 over time during the placebo intervention, indicating that the availability of oxygen to the muscles was reduced and oxygen consumption was increased.Citation19–21
No differences were found in muscle fatigue between the two interventions. Paillard et al. showed that supplementation of voluntary contractions by electrical stimulation reduces muscle fatigue (measured by an isokinetic dynamometer).Citation22 It is possible that a reduced level of voluntary muscle activity could decrease the energetic cost and oxygen demand, thus reducing muscle fatigue. In the present study, muscle fatigue was measured on a VAS, which is subjective, rather than on an isokinetic dynamometer, which is more objective.Citation23
The deoxy(Hb + Mb)/VO2 ratio was significantly lower during the FES-Cycling than during the placebo intervention (p < 0.0001). This could be attributed to two factors. (1) We previously evoked the fact that there was significantly less deoxy(Hb + Mb) under the sensor in the FES-Cycling group. This decrease in deoxy(Hb + Mb) is partially responsible for the decrease in the deoxy(Hb + Mb)/VO2 ratio. (2) There was a significant increase in VO2 in the FES-Cycling group. This further amplified the decrease in the deoxy(Hb + Mb)/VO2 ratio. These results are surprising since theoretically, if we consider the hypothesis that deoxy(Hb + Mb) is associated with the microvascular extraction of O2 under the sensor during exertion, an increase in VO2 could lead to an increase in deoxy(Hb + Mb). In the present case, this did not occur. It must be noted that we only measured variations in muscle oxygenation of the VL with NIRS. It is likely that the decrease in deoxy(Hb + Mb) in the FES-cycling group is due to a smaller degree of activity of the VL (reflecting voluntary activity). Furthermore, we propose that the significant increase in VO2 was due to the increase in the number of motor units recruited in the rectus femoris muscle by the electrical stimulation, which was superimposed on the voluntary contractions. We cannot confirm this hypothesis since the NIRS system used only measured voluntary VL activity, and not electrically-induced rectus femoris activity. Nevertheless, these results suggest that the metabolic load of the quadriceps muscle was increased by the FES, without an associated increase in dyspnoea. This indicates that FES-cycling could be an appropriate method for patients with severe COPD who have difficulty initiating a pulmonary rehabilitation programme.
Muscle and tissue oxy(Hb + Mb)) and muscle and tissue blood volume (total(Hb + Mb)) both increased over time, with no differences in these variables between the FES-cycling and Placebo interventions. The increase in these factors over time during both interventions could be the result of local vasodilatation induced by the exercise and the recruitment of arterioles and capillaries. These results are in agreement with data from a study carried out in healthy subjects during moderate intensity exercise (40% and 60% of VO2peak).Citation24,Citation25
This work, a secondary analysis of a randomised, controlled cross-over study, provided new information regarding muscle activity during a novel training method: FES-cycling. The design limited confounding factors relating to the NIRS and successfully demonstrated that NIRS is an easy-to-use and non-invasive technique that is suitable for the continuous measurement of muscle activity and exercise intensity. Participants were evaluated under the same conditions and on the same day, but with sufficient rest time in between sessions; there was no evidence to suggest that there was an effect of session order or a carry-over effect.Citation6 The NIRS hardware was well fixed to the participant to limit data artefacts being recorded during the exercise and, in addition, the NIRS was not removed between the sessions.
This study has several limitations: (1) only eight participants underwent NIRS measurements, (2) the contribution of Hb and Mb in vivo to the NIRS signal is not well known for muscle oxygenation which made data difficult to interpret. Despite this, the Mb desaturation apparently contributes to less than 20% of the total NIRS desaturation signal during exercise,Citation26 (3) catheters were not used to precisely measure changes in femoral arterial and venous oxygen, although StO2 results were linearly related to femoral venous saturation indicating that NIRS could be used as a measure of oxygen consumption as a function of local blood flow,Citation27 (4) changes in oxygenation of the rectus femoris muscle were not measured, which might have shown greater activity in this muscle during FES-cycling due to the combined electrical stimulation and voluntary activity and (5) changes in deoxy(Hb + Mb) can be influenced by certain factors (QO2/VO2) such as changes in haematocrit, capillary–venous haem concentration, and/or volume of small blood vessels). Although the influence of these factors is reduced during exertion, we wish to highlight the complexity of interpreting changes in deoxy(Hb + Mb).Citation14
Conclusions
The results of this secondary analysis suggest oxygen extraction was greater during the Placebo-FES intervention than during FES-cycling, possibly due to a higher level of voluntary muscle activity with no electrical stimulation. Nevertheless, VO2 was greater in the FES-cycling group. This was probably due to a greater recruitment of muscle fibres in the whole of the quadriceps muscle with the supplementation of voluntary muscle activity with electrical stimulation, resulting in an increase in the metabolic load of the quadriceps with no increase in dyspnoea. The results of this secondary analysis of our previous study suggest FES-cycling could decrease muscle deoxy(Hb + Mb) and limit decreases in muscle StO2, thus opening the door to future research in this new type of training.
Disclosure of interest
The authors report no conflict of interest
Acknowledgments
We thank Johanna Robertson and Jennifer Dandrea Palethorpe for translation and constructive criticism.
Additional information
Funding
References
- Bernard S, LeBlanc P, Whittom F, Carrier G, Jobin J, Belleau R, Maltais F. Peripheral muscle weakness in patients with chronic obstructive pulmonary disease. Am J Respir Crit Care Med. 1998;158(2):629–634. doi: 10.1164/ajrccm.158.2.9711023.
- Couillard A, Prefaut C. From muscle disuse to myopathy in COPD: potential contribution of oxidative stress. Eur Respir J. 2005;26(4):703–719. doi: 10.1183/09031936.05.00139904.
- Maltais F, Decramer M, Casaburi R, Barreiro E, Burelle Y, Debigaré R, Dekhuijzen PNR, Franssen F, Gayan-Ramirez G, Gea J, et al. An official American Thoracic Society/European Respiratory Society statement: update on limb muscle dysfunction in chronic obstructive pulmonary disease. Am J Respir Crit Care Med. 2014;189(9):e15–62. doi: 10.1164/rccm.201402-0373ST.
- Garrod R, Paul EA, Wedzicha JA. Supplemental oxygen during pulmonary rehabilitation in patients with COPD with exercise hypoxaemia. Thorax. 2000;55(7):539–543.
- Camillo CA, Osadnik CR, van Remoortel H, Burtin C, Janssens W, Troosters T. Effect of “add-on” interventions on exercise training in individuals with COPD: a systematic review. ERJ Open Res. 2016;2(1). doi: 10.1183/23120541.00078-2015
- Medrinal C, Prieur G, Combret Y, Quesada AR, Debeaumont D, Bonnevie T, Gravier FE, Dupuis Lozeron E, Quieffin J, Contal O, et al. Functional electrical stimulation-a new therapeutic approach to enhance exercise intensity in chronic obstructive pulmonary disease patients: a randomized, controlled crossover trial. Arch Phys Med Rehabil. 2018;99(8):1454–1461. doi: 10.1016/j.apmr.2018.02.002
- Medrinal C, Prieur G, Debeaumont D, Robledo Quesada A, Combret Y, Quieffin J, Contal O, Lamia B. Comparison of oxygen uptake during cycle ergometry with and without functional electrical stimulation in patients with COPD: protocol for a randomised, single-blind, placebo-controlled, cross-over trial. BMJ Open Respir Res. 2016;3(1):e000130. doi: 10.1136/bmjresp-2016-000130.
- Vivodtzev I, Debigaré R, Gagnon P, Mainguy V, Saey D, Dubé A, Paré M-È, Bélanger M, Maltais F. Functional and muscular effects of neuromuscular electrical stimulation in patients with severe COPD: a randomized clinical trial. Chest. 2012;141(3):716–725. doi: 10.1378/chest.11-0839.
- Minogue CM, Caulfield BM, Lowery MM. Whole body oxygen uptake and evoked knee torque in response to low frequency electrical stimulation of the quadriceps muscles: V•O2 frequency response to NMES. J Neuroeng Rehabil. 2013;10(1):63. doi: 10.1186/1743-0003-10-63.
- Adami A, Cao R, Porszasz J, Casaburi R, Rossiter HB. Reproducibility of NIRS assessment of muscle oxidative capacity in smokers with and without COPD. Respir Physiol Neurobiol. 2017;235:18–26. doi: 10.1016/j.resp.2016.09.008.
- Van Beekvelt MC, Colier WN, Wevers RA, Van Engelen BG. Performance of near-infrared spectroscopy in measuring local O(2) consumption and blood flow in skeletal muscle. J Appl Physiol (1985). 2001;90(2):511–519. doi: 10.1152/jappl.2001.90.2.511.
- Ferreira LF, Koga S, Barstow TJ. Dynamics of noninvasively estimated microvascular O2 extraction during ramp exercise. J Appl Physiol (1985). 2007;103(6):1999–2004. doi: 10.1152/japplphysiol.01414.2006.
- Grassi B, Pogliaghi S, Rampichini S, Quaresima V, Ferrari M, Marconi C, Cerretelli P. Muscle oxygenation and pulmonary gas exchange kinetics during cycling exercise on-transitions in humans. J Appl Physiol (1985). 2003;95(1):149–158. doi: 10.1152/japplphysiol.00695.2002.
- Adami A, Koga S, Kondo N, Cannon DT, Kowalchuk JM, Amano T, Rossiter HB. Changes in whole tissue heme concentration dissociates muscle deoxygenation from muscle oxygen extraction during passive head-up tilt. J Appl Physiol (1985). 2015;118(9):1091–1099. doi: 10.1152/japplphysiol.00918.2014.
- Baty F, Ritz C, van Gestel A, Brutsche M, Gerhard D. Modeling the oxygen uptake kinetics during exercise testing of patients with chronic obstructive pulmonary diseases using nonlinear mixed models. BMC Med Res Methodol. 2016;16:66. doi: 10.1186/s12874-016-0173-8
- Barker AR, Trebilcock E, Breese B, Jones AM, Armstrong N. The effect of priming exercise on O2 uptake kinetics, muscle O2 delivery and utilization, muscle activity, and exercise tolerance in boys. Appl Physiol Nutr Metab. 2014;39(3):308–317. doi: 10.1139/apnm-2013-0174.
- McNarry MA, Harrison NK, Withers T, Chinnappa N, Lewis MJ. Pulmonary oxygen uptake and muscle deoxygenation kinetics during heavy intensity cycling exercise in patients with emphysema and idiopathic pulmonary fibrosis. BMC Pulm Med. 2017;17(1):26. doi: 10.1186/s12890-017-0364-z
- da Silva JCL, Tarassova O, Ekblom MM, Andersson E, Rönquist G, Arndt A. Quadriceps and hamstring muscle activity during cycling as measured with intramuscular electromyography. Eur J Appl Physiol. 2016;116(9):1807–1817. doi: 10.1007/s00421-016-3428-5.
- DeLorey DS, Kowalchuk JM, Paterson DH. Relationship between pulmonary O2 uptake kinetics and muscle deoxygenation during moderate-intensity exercise. J Appl Physiol (1985). 2003;95(1):113–120. doi: 10.1152/japplphysiol.00956.2002.
- DeLorey DS, Kowalchuk JM, Paterson DH. Effect of age on O(2) uptake kinetics and the adaptation of muscle deoxygenation at the onset of moderate-intensity cycling exercise. J Appl Physiol (1985). 2004;97(1):165–172. doi: 10.1152/japplphysiol.01179.2003.
- DeLorey DS, Kowalchuk JM, Paterson DH. Adaptation of pulmonary O2 uptake kinetics and muscle deoxygenation at the onset of heavy-intensity exercise in young and older adults. J Appl Physiol (1985). 2005;98(5):1697–1704. doi: 10.1152/japplphysiol.00607.2004.
- Paillard T, Margnes E, Maitre J, Chaubet V, François Y, Jully JL, Gonzalez G, Borel L. Electrical stimulation superimposed onto voluntary muscular contraction reduces deterioration of both postural control and quadriceps femoris muscle strength. Neuroscience. 2010;165(4):1471–1475. doi: 10.1016/j.neuroscience.2009.11.052.
- Nyberg A, Saey D, Maltais F. Why and how limb muscle mass and function should be measured in patients with chronic obstructive pulmonary disease. Ann Am Thorac Soc. 2015;12(9):1269–1277. doi: 10.1513/AnnalsATS.201505-278PS.
- Kime R, Im J, Moser D, Nioka S, Katsumura T, Chance B. Noninvasive determination of exercise-induced vasodilation during bicycle exercise using near infrared spectroscopy. Med Sci Monit. 2009;15(3):CR89–94.
- Kounalakis SN, Geladas ND. Cardiovascular drift and cerebral and muscle tissue oxygenation during prolonged cycling at different pedalling cadences. Appl Physiol Nutr Metab. 2012;37(3):407–417. doi: 10.1139/h2012-011.
- Ferrari M, Muthalib M, Quaresima V. The use of near-infrared spectroscopy in understanding skeletal muscle physiology: recent developments. Philos Trans A Math Phys Eng Sci. 2011;369(1955):4577–4590. doi: 10.1098/rsta.2011.0230.
- Vogiatzis I, Habazettl H, Louvaris Z, Andrianopoulos V, Wagner H, Zakynthinos S, Wagner PD. A method for assessing heterogeneity of blood flow and metabolism in exercising normal human muscle by near-infrared spectroscopy. J Appl Physiol (1985). 2015;118(6):783–793. doi: 10.1152/japplphysiol.00458.2014.