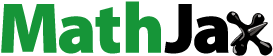
Abstract
While the mechanisms underlying exercise limitations and symptoms during leg activities have been investigated in detail, knowledge of potential differences between leg and arm activities are not well understood and results from individual studies are contradictory. Thus, the aim of the present study was to synthesize physiological and symptomatic responses during activities involving the arms relative to activities involving the legs in people with Chronic Obstructive Pulmonary Disease (COPD). Any study with a cross-sectional comparison of acute physiological (cardiorespiratory, metabolic) and symptomatic responses to activities performed with the arms versus the legs were included. Studies were sub-grouped based on the type of activity performed (cycle ergometer, resistance exercises, or functional test/activities). Eighteen studies with 423 individuals with COPD were included. Leg cycle ergometer resulted in greater tidal volume (137 mL), minute ventilation (4.8 L/min), and oxygen consumption (164 mL/min) than arm cycle ergometer, while symptomatic responses were similar. Resistance exercises resulted in similar physiological and symptomatic responses irrespective of whether the legs or the arms were involved while studies on functional activities report different results depending on the type and intensity of the activity performed. With the exception of cycle ergometer activities, physiological and symptomatic responses do not seem to depend on whether the arms or the legs are used, but rather seem to be task and intensity dependent. These novel findings suggest, for example, that strategies used to increase exercise tolerance should not be dependent on whether the arms or the legs are used, but rather the intensity of specific activity performed.
Introduction
COPD (COPD) is a disease characterized by persistent respiratory symptoms and airflow limitation [Citation1]. In addition to this central limitation, a common peripheral consequence of COPD is limb muscle dysfunction [Citation2], which affects the function of the leg and arm muscles [Citation2, Citation3]. In comparison to healthy individuals, people with COPD present reduced aerobic capacity [Citation4], reduced mechanical efficiency (ME) (work per unit oxygen consumed) [Citation5], increased fatigue for the same absolute oxygen consumption [Citation6], as well as greater reliance on glycolysis during exercise [Citation7] when performing leg activities, such as walking or cycling. Arm activities are also poorly tolerated by many individuals with COPD, mainly when the arms are unsupported and raised above shoulder height. For example, similar peak oxygen consumption (V̇ᴏ2) and minute ventilation (V̇e) has been seen during arm (arm elevation) and leg (knee extension) resistance exercises when expressed as a percentage of maximal oxygen consumption obtained from a cardiopulmonary exercise test [Citation8]. Compared to healthy individuals, people with COPD have demonstrated increased hyperinflation as well as increased perceived dyspnea, despite lower cardiorespiratory responses during maximal arm exercises [Citation9]. Since leg and arm activities often are problematic for individuals with COPD [Citation10, Citation11], pulmonary rehabilitation guidelines recommend the inclusion of training modalities that target both arms and legs [Citation9, Citation10].
Before starting an exercise training program, an exercise assessment is needed to individualize the exercise prescription, ensure the safety of the intervention and evaluate the potential need for adjunct strategies to increase exercise tolerance [Citation10]. Identifying a single variable for exercise intolerance in COPD is difficult; it is likely multifactorial including both physiological and symptomatic factors as well as dependent on the mode of activity [Citation10, Citation12].
To date, several comparisons between arm and leg activities have been made, but with somewhat conflicting results. For example, McKeough et al. [Citation13] found that at a given V̇ᴏ2, dyspnea response was comparable during arm and leg cycling in individuals with COPD, and there was no difference in V̇ᴏ2 peak. However, in contrast, Carter et al. [Citation14], found greater peak V̇ᴏ2 during leg cycling than arm cycling, while Porto et al. [Citation15] found that, at a given V̇ᴏ2, dyspnea and hyperinflation was greater during arm exercises than during leg exercises in individuals with COPD. These seemingly contradictory messages highlight the need for a systematic review of studies that have evaluated physiological responses and symptoms during arm and leg activities in individuals with COPD. Determining physiological responses and symptoms of arm versus leg activities will help us understand the mechanisms behind the difficulties that people with COPD experience when performing physical activities. This knowledge will also assist prescription of individualized exercise training, for example, evaluating the potential need for adjunct strategies to increase exercise tolerance.
Objectives
The primary objective of this systematic review and meta-analysis was to synthesize the physiological responses during activities involving the arms relative to activities involving the legs in individuals with COPD. A secondary objective of this systematic review was to synthesize the exertional symptoms during activities involving the arms relative to activities involving the legs in individuals with COPD.
Methods
The systematic review is reported in line with the preferred reporting items for systematic review/meta-analysis (PRISMA) [Citation16]. It is registered with the International Prospective Register of Systematic Reviews (registration number: CRD42017074476), and a protocol with detailed information about the methods used has been published [Citation17].
Eligibility criteria for inclusion of studies
We considered all prospective and retrospective study designs, including but not limited to cross-sectional, cohort, case-control and experimental study designs. We included studies that presented a cross-sectional comparison of physiological responses and symptoms of arm versus leg activities in individuals with a diagnosis of COPD confirmed by spirometry following published guidelines [Citation1, Citation18–21]. For studies with multiple measurement points, such as intervention studies, baseline data were used. Exposure included any type of upper limb/arm activity, either unsupported or supported; including but not limited to aerobic and resistance training/activities targeting the upper limbs/arms. Comparators included any type of lower leg activity; including but not limited to aerobic and resistance training/activities targeting the legs. Primary outcomes included, but were not limited to 1) Cardiorespiratory and metabolic responses: heart rate (HR), peripheral capillary oxygen saturation (Spᴏ2%), V̇ᴏ2, carbon dioxide production (V̇co2), and concentration of blood lactate; 2) Respiratory responses: tidal volume (Vt), (V̇e), respiratory rate (RR), minute ventilation and maximum voluntary ventilation ratio (V̇e/MVV), dynamic hyperinflation (DH), end-expiratory lung volume (EELV), end-inspiratory lung volume (EILV), and inspiratory capacity (IC); 3) Biomechanics: muscle activity, chest wall kinematics, mechanical efficiency (ME), peak load and total workload. Secondary outcomes included: subjective ratings of exertional symptoms (i.e. dyspnea and fatigue) measured with the revised Borg category-ratio 0–10 scale (CR10) [Citation22] or another scale with similar properties.
There was no restriction related to year of publication; all articles from inception to 2017-10-01 of selected databases were considered if written in English, Swedish, Spanish, or Portuguese. We included relevant conference abstracts if all information could be retrieved. If not, they were excluded. Studies that included a mixed population where individuals with COPD were not the primary diagnosis were excluded if data could not be attained separately for individuals with COPD. Studies including activities involving the whole body or arms and legs simultaneous were excluded.
Search methods
Potentially relevant studies were identified from CINAHL (EBSCO interface, 1981 onwards) EMBASE (OVID interface, 1980 onwards), Physiotherapy Evidence Database (PEDro) (Neuroscience Research Australia (NeuRA) 1929 onwards), PubMed (U.S. National Library of Medicine, 1946 onwards), and Cochrane Central Register of Controlled Trials (Wiley, 2010 onwards). A search for “grey” literature was performed through clinicaltrials.gov, where observational studies, as well as randomized controlled trials, can be registered. Also, we searched the Cochrane Database of Systematic Reviews (CDSR) and PROSPERO, to identify existing or ongoing reviews on the topic.
Search strategy
The search strategy was developed with the assistance of a Health Science Librarian and reviewed by experts in the fields of physiotherapy and lung diseases. To ensure literature saturation, comprehensive searches were constructed of both indexes terming (MeSH terms), “free text” terms and synonyms. We also hand-searched the reference lists of included studies or relevant reviews identified through the search. Lastly, the “related articles” function in PubMed was used on included studies or relevant reviews. We used the following search terms to search trials registers and databases: Pulmonary Disease, Chronic Obstructive; COPD; COPD; COAD; Chronic Obstructive Airway Disease; Chronic Obstructive Lung Disease; Airflow Obstruction, Chronic; Airflow Obstructions, Chronic; Chronic Airflow Obstructions; Upper Extremity; Extremities, Upper; Upper Extremities; Upper Limb; Limb, Upper; Extremity, Upper; arm; arms; shoulder; Lower Extremity; Extremities, Lower; Lower Extremities; Lower Limb; Limb, Lower; Limbs, Lower; Lower Limbs; Extremity, Lower; Hip; Knee; Leg; Legs; Thigh. The complete search strategy used for searches in PubMed can be found in online supplementary file 1.
Study records
The results from the literature searches were uploaded to COVIDENCE, an internet-based software program that facilitates management of studies, including removal of duplicates. The inclusion of articles was then performed in three steps with one reviewer screening titles (EF), two reviewers were involved in screening of abstracts (EF & AN), and four reviewers were involved screening of full-text articles (EF [all full-texts] & AN/VPL/TJF [one third each]). In case of disagreement or uncertainty, the decision was reached through discussions between reviewers. Agreement between assessors was assessed mathematically using a Kappa statistic (K value). Special attention to identifying possible duplicates was taken to minimize risk for biased results. The selection process is presented in a PRISMA flow chart diagram (). A standardized data extraction form, based on the eligibility criteria for inclusion of studies, was used by two reviewers to extract data independently from full-text copies of all included studies.
Study quality assessment
Assessment of the methodological quality of the studies was done by the 14 most relevant components from the checklist by Downs and Black [Citation23]:
Is the hypothesis/aim/objective of the study clearly described?
Are the main outcomes to be measured clearly described in the introduction or methods section?
Are the characteristics of the patients included in the study clearly described? Are the distributions of principal confounders in each group of subjects to be compared clearly described?
Are the main findings of the study clearly described?
Does the study provide estimates of the random variability in the data for the main outcomes?
Have the characteristics of patients lost to follow-up been described?
Have actual probability values been reported (for example .035 rather than <.05) for the main outcomes except where the probability value is <.001?
Were the subjects asked to participate in the study representative of the entire population from which they were recruited?
Were those subjects who were prepared to participate representative of the entire population from which they were recruited?
Were the statistical tests used to assess the main outcomes appropriate?
Were the main outcome measures used accurate (valid and reliable)?
Were losses of patients to follow-up taken into account?
Did the study have sufficient power to detect a clinically important effect/difference, where the probability value for a difference is due to chance, is less than 5%?
A standardized form was used by two independent reviewers, and each item was graded as yes = 1, no = 0, or unable to determine (UTD) = 0. Giving a possible total score of 14 points, no grading based on scores was used. In case of disagreement or uncertainty, the decision was reached through discussions between reviewers.
Data extraction
Data extraction was individually performed by two review authors (EF and HVS) with a standardized and pilot tested data extraction form. Disagreements were resolved by discussion between the two review authors; if no agreement could be reached, it was planned a third author would decide (AN). All extracted data were double checked with the included studies.
Data items
Study: author(s) name, title, publication year, inclusion and exclusion criteria, setting and identification of measurements taken without prior intervention.
Participants: age, gender, diagnosis of COPD, forced expiratory volume in 1 s, body mass index, comorbidities, sample size, and missing values.
Exposure (arm activity): type of activity, number of participants, intensity and duration.
Comparator (leg activity): type of activity, number of participants, intensity and duration.
Data analysis
When appropriate, we performed a meta-analysis using Review Manager (RevMan). A meta-analysis was considered when at least two studies reported sufficient data for the same outcome using the same exposure and control. Thus meta-analysis was only done with data from studies that included cycle ergometer tests. Because of the nature of the exposure analyzed in this review, we expected heterogeneity between the studies. Therefore, we performed meta-analyses using a random-effect model rather than a fixed-effect model. The levels of statistical heterogeneity were assessed using the I2 score from each analysis. I2 values from 0% to 25% were interpreted as the meta-analysis having a low level of heterogeneity, values from 26% to 50% as a moderate level, values from 51% to 75% as a high level, and from 76% to 100% as a considerable level [Citation24].
Outcomes included and not included in the meta-analysis are descriptively reported in tables. For a uniform presentation was data presented as means with standard deviations (SD), mean difference, and effect size. If a standard error was presented in the original study, a SD was calculated as:
And, the effect size was estimated as the difference in means divided by the pooled SD:
Alterations to study protocol
No additional search was done after 2017-10-01, but notifications of new studies were received, and one study was included after this date.
Results
Results of the search
In total, 483 potentially relevant articles were found after database searches and imported to Covidence. After removal of duplicates 329 articles were screened, of which 18 studies with a total of 423 individuals with COPD met the inclusion criteria (). The agreement between reviewers regarding the inclusion of studies after initial full-text screening was high (Cohens Kappa 0.80), and all disagreements were resolved by discussion. Quality assessment scores are shown in . An overview of the outcomes used to compare the differences between arm versus leg activities is seen in . To improve data reporting and interpretability of the findings, the included studies were categorized in three groups according to the type of activity performed: 1) studies that compared arm cycle ergometer versus leg cycle ergometer, 2) studies that compared resistance training exercises between arms and legs, and 3) studies that compared arm functional tests with functional tests involving the legs.
Table 1. Downs and black scores for individual studies.
Table 2. Incremental cycle ergometer tests.
Table 3. Constant load ergometer.
Table 4. Resistance training exercises
Table 5. Functional tests/activities
Quality assessment
Downs and Black scores are given in . Of the 18 assessed studies, the mean score was 10.3 out of the total 14 (range: 8 to 13). The item related to study sample being or not representative of the entire population from which they were recruited (based on random or consecutive sampling), and the item concerning study power could often not be determined and therefore reduced the total score. Also, in some studies, the characteristics of included participants were not clearly described, due to the lack of explicit inclusion and exclusion criteria.
Cycle ergometer
Seven studies [Citation13, Citation14, Citation25–29] () compared gas exchange outcomes during incremental arm versus leg cycle ergometer tests, and nine outcomes were identified as suitable for meta-analysis; V̇ᴏ2, HR, Vt, RR, V̇e, V̇e/MVV, load, Spᴏ2%, and dyspnea (). The analyses demonstrate lower V̇ᴏ2, Vt, V̇e, V̇e/MVV, and workload during seated arm cycle ergometer compared to leg cycle ergometer (). No significant differences were demonstrated for HR, RR, Spᴏ2%, and dyspnea during arm cycle ergometer compared to leg cycle ergometer (). The heterogeneity estimation demonstrated low heterogeneity (I2 <18%) for all variables except workload (I2 70%). Additional outcomes are presented in .
Figure 2. Random-effects meta-analysis on the mean difference of oxygen consumption between arm and leg cycle ergometer tests at peak level reported as mL/minute.
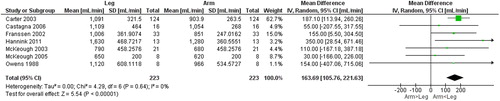
Figure 3. Random-effects meta-analysis on the mean difference in heart rate between arm and leg cycle ergometer tests at peak level reported as beats/minute.

Figure 4. Random-effects meta-analysis on the mean difference of tidal volume between arm and leg cycle ergometer tests at peak level reported as mL.
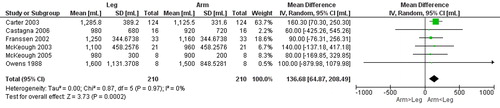
Figure 5. Random-effects meta-analysis on the mean difference of respiratory rate between arm and leg cycle ergometer tests at peak level reported as breaths/minute.

Figure 6. Random-effects meta-analysis on the mean difference in ventilation between arm and leg cycle ergometer tests at peak level reported as litres/minute.
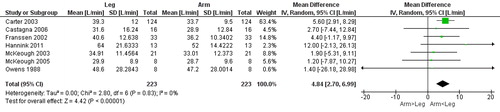
Figure 7. Random-effects meta-analysis on the mean difference of work load between arm and leg cycle ergometer tests at peak level reported in watt.
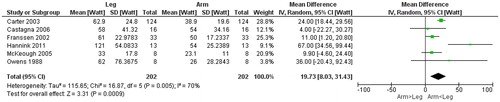
Figure 8. Random-effects meta-analysis on the mean difference of dyspnea between arm and leg cycle ergometer tests at peak level reported the revised Borg category-ratio 1–10 scale.

Figure 9. Random-effects meta-analysis on the mean difference of ventilatory reserve between arm and leg cycle ergometer tests at peak level reported in %.

Figure 10. Random-effects meta-analysis on the mean difference of oxygen saturation between arm and leg cycle ergometer tests at peak level reported in %.

Figure 11. Random-effects meta-analysis on the mean difference of mechanical efficiency between arm and leg cycle ergometer tests at 50% of peak level reported as %.

Also, three studies investigated physiological responses to continuous work during submaximal levels of exercise (that is, performed at a % of the mode specific peak]) during cycle ergometer [Citation25–27] (). During submaximal continuous work, the ME was higher for arm cycle ergometer (). Not included in the meta-analyses due to differences in test procedures, Hannink et al. [Citation27] found lower workload, V̇ᴏ2, and Vt, similar DH (change in IC pre- to post-test), and higher HR and RR, during arm- compared to leg cycle ergometer at iso-Ve. Both inspiratory and expiratory time was significantly reduced during arm cycle ergometer and contributed to a higher RR [Citation27]. Additional outcomes are presented in .
Resistance exercises
Six studies [Citation8, Citation30–34] () reported comparisons of resistance exercises performed with the arms and the legs, using free weights, weight lifting equipment [Citation8], elastic bands [Citation33–35], isometric testing equipment [Citation31] or without equipment [Citation30]. Two studies reported gas exchange outcomes [Citation8, Citation34], three studies reported symptoms during exercise [Citation8, Citation32, Citation33], one study reported fatigability during isometric contractions [Citation31], and one study used near-infrared spectroscopy to determine the oxygen recovery constant [Citation30]. Robles et al. [Citation8] and Nyberg et al. [Citation34] found similar V̇ᴏ2, and V̇e during arm (arm elevation) and leg (knee extension) resistance exercises when expressed as a percentage of V̇ᴏ2 peak obtained from a cardiopulmonary exercise test. These findings were similar in both studies, even though the design of the resistance exercise protocol used varied (muscle strength [high-load/low-repetition] [Citation8] versus muscle endurance [low-load/high-repetition] [Citation34]). However, when expressed as V̇ᴏ2 per kg work performed, Nyberg et al. [Citation34] found that oxygen consumption per Kg work performed was significantly higher during arm elevation (214 [SD 88] ml/kg/min) than during leg extension (53 [SD 15] ml/kg/min). The same research group also presented dyspnea and muscle fatigue ratings during low-load/high-repetition resistance training using elastic bands in two separate trials, demonstrating similar exertional dyspnea (pooled mean ratings: arm 4.0 [SD 1.7], leg 4.0 [SD 1.5]) and muscle fatigue (arm 5.2 [SD 1.6], leg 5.2 [SD 1.4]) during arm and leg resistance exercises [Citation32, Citation33]. These ratings on dyspnea and muscle fatigue are comparable to the ratings of perceived exertion (arm 5 [SD 2.5], leg 4 [SD 3.1]) reported by Robles et al. [Citation8]. Furthermore, Miranda et al. [Citation31] investigated the fatigability of the quadriceps muscle during an isometric knee extension exercise performed at 60° knee flexion and the middle deltoid during an isometric shoulder abduction exercise performed with the shoulder placed at 90° abduction. Both exercises were performed at equivalent work-loads (60% of maximal voluntary isometric contractions, to the limit of tolerance) and fatigability was examined continuously during the exercises using electromyography. They found a greater decline in median frequency throughout an isometric local muscle endurance test at 60% of maximal voluntary contraction for the middle deltoid compared to the quadriceps femoris. When corrected for time of the endurance test, the dyspnea and fatigue scores were higher for the middle deltoid during the isometric shoulder abduction exercise than for the quadriceps femoris during the isometric knee extension exercise [Citation31].
Lastly, Adami et al. [Citation30] performed repeated intermittent arterial occlusions after a brief (10–15 sec) isometric muscle contractions of the medial forearm and medial gastrocnemius and used near-infrared spectroscopy to determine the recovery rate of muscle oxygen consumption. They found no differences between arm and leg muscles [Citation30].
Functional tests/activities
Seven studies compared functional tests performed with the arms and the legs [Citation13, Citation15, Citation29, Citation36–39] (). Six studies reported gas exchange outcomes [Citation13, Citation15, Citation29, Citation36–38] and one study reported changes in spirometric values pre- and post-activity [Citation39]. Celli et al. [Citation36] compared a test involving moving rings up and down in a pegboard with an unloaded leg cycle ergometer. De Souza et al. [Citation37] and Porto et al. [Citation15] compared a diagonal arm movement with a dumbbell with treadmill walking. Marrara et al. [Citation38] and Silva et al. [Citation39] compared functional activities such as blackboard erasing and storing pots on a shelf, with treadmill walking. While McKoeogh et al [Citation13, Citation29] compared the unsupported upper limb exercise test (UULEX) to an incremental leg cycle ergometer test.
Five studies reported a lower V̇ᴏ2 [Citation13, Citation15, Citation29, Citation36, Citation37], four studies reported a lower HR [Citation13, Citation29, Citation36, Citation37], and four studies reported a lower V̇e [Citation13, Citation29, Citation36, Citation37] during arm activity compared to leg activity (the difference not always statistically significant (). De Souza et al. [Citation37], Marrara et al. [Citation38], and Mc Keough et al. [Citation29] also reported lower ratings of dyspnea during arm activity. Silva et al. [Citation39] found a lower change in HR and dyspnea (pre-post activity), and a lower proportion of participants with DH after the arm activity when comparing storing pots and walking for 5 minutes. In contrast, Celli et al. [Citation36] reported a greater proportion of participants developing dyssynchronous breathing during arm activity (rings movement in a pegboard) (arm 5/12, leg 0/12) compared to an unloaded cycle ergometer. Porto et al. [Citation15] investigated arm and leg activities effect on hyperinflation at similar levels of V̇ᴏ2 consumption and found a higher V̇e, dyspnea, and a higher DH (change in IC pre- to post-test) during arm activity.
Discussion
The main findings of this systematic review are that people with COPD perform leg activities at a higher absolute load compared to arm activities, and that leg activities often generate greater central physiological responses. However, exertional dyspnea and limb muscle fatigue do not seem to depend on whether the arms or the legs are used. Instead, dyspnea and limb muscle fatigue are more related to the relative intensity of the specific task performed.
Cycle ergometer
The results from the meta-analysis revealed that V̇ᴏ2 peak was significantly higher (mean difference 164 ml/min, arm/leg ratio 0.86) during leg- compared to arm-cycle ergometer in people with COPD. The difference in V̇ᴏ2 peak between arm and leg cycle ergometer is, however, considerably less than previously found in healthy young people. An absolute difference of 890 ml/min and arm/leg ratio of 0.70 have been reported in a systematic review comparing incremental arm and leg cycle ergometer tests [Citation40]. The smaller difference in V̇ᴏ2 peak between leg- and arm cycle ergometer in people with COPD could be related to differences in muscle force capacities [Citation3] and is in line with previous research showing that leg muscles often are more affected than arm muscles among people with COPD [Citation2], even though this is not a universal finding [Citation3]. Furthermore, these findings should not be interpreted as evidence of a preserved arm function compared to healthy controls [Citation9].
Another interesting finding is the lower Vt (−137 mL) and V̇e (−4.8 L) at a similar RR during arm compared to leg cycle ergometer. Observations that may be explained by a decreased function of the respiratory muscles during arm compared to leg cycle ergometer. For example, during arm activities the elastic load of the inspiratory muscles may change [Citation41], as a result the accessory muscles of respiration are less available to assist with ventilation, as they are recruited also for postural support [Citation36]. Thus, as a result, the function of the respiratory muscles is reduced during arm cycle ergometer which is not seen to the same extent during leg cycle ergometer.
Resistance exercises
The differences in central physiological responses seen in arm and leg cycle ergometer () does not seem to be present during resistance training exercises [Citation8, Citation34], thus indicating that physiological responses are task specific. For example, in contrast to the higher central physiological responses observed during leg cycle ergometer in comparison to arm cycle ergometer, similar physiological responses (V̇e, V̇ᴏ2, and HR) were seen between arm and leg resistance exercises [Citation8, Citation34]. Similarly as for physiological responses, no differences were seen in perceived symptoms between arm and leg resistance exercises despite differences in absolute workloads [Citation8, Citation32, Citation33]. The higher fatigability of the middle deltoid found by Miranda et al. [Citation31] is in agreement to what is found in a healthy population [Citation42].
Functional tests/activities
The comparisons made of functional activities are more difficult to interpret due to the heterogeneity of the different activities performed. Nevertheless, it seems that functional leg activities lead to greater physiological responses (For example, V̇ᴏ2 [Citation13, Citation15, Citation29, Citation36, Citation37], HR [Citation13, Citation29, Citation36, Citation37], V̇e [Citation13, Citation29, Citation36, Citation37], and hyperinflation [Citation39]), as well as more perceived symptoms (dyspnea) [Citation29, Citation37, Citation38], than arm activities. However, only the studies of McKeough et al. [Citation13, Citation29] used activities that involved both arms (other studies used single-arm activities) whereas all studies used a control activity that involved both legs. Thus, considering that bilateral arm activities have been shown to lead to greater physiological responses and exertional symptoms than single arm activities [Citation34], these findings should be interpreted with caution as they may be different if both the leg and arm conditions are performed using the same setup (both bilateral execution of arm and leg activities).
Also, the relative intensity of the activity is most likely the most important factor. For example, Porto et al. [Citation15] stopped the leg activity when the V̇ᴏ2 was equal to V̇ᴏ2 peak during arm activity, thus resulting in that the arm activity was maximal and the leg activity submaximal. The observed difference in relative intensity might explain higher physiological responses (V̇e, RR, and IC reduction) and more dyspnea during arm activity in this study [Citation15].
Oxygen consumption per unit of work performed
The amount of oxygen consumed per unit of work performed was investigated in two cycle-ergometer and one resistance exercise study, with somewhat conflicting results [Citation25, Citation26, Citation34]. Healthy controls consumed more oxygen per unit of work during arm activities compared to leg activities in all three studies [Citation25, Citation26, Citation34]. For individuals with COPD, Franssen et al. [Citation26] found a higher ME (i.e. less oxygen consumption per unit of work) during arm compared to leg cycle ergometer, while Castagna et al. [Citation25] found no difference in ME between the arms and the legs during cycle ergometer. Furthermore, Nyberg et al. [Citation34] found a higher oxygen consumption per unit of work during shoulder flexion compared to leg extension resistance exercises (i.e. more oxygen used per unit of arm work). The latter is in line with observations among the healthy controls within included studies [Citation25, Citation26, Citation34]. These somewhat contradictory findings could, at least partly, be explained by differences in degree of muscle dysfunction across studies. For example, the participants in the study by Franssen et al. [Citation26] in which a higher ME during arm activities was found also had the greatest reduction in leg muscle function (∼70% lower) when compared with the healthy control group. Of importance, the reduction in leg muscle function was seemingly greater than the reduction in arm muscle function (∼54% lower), thus indicating that the higher ME during arm activities might be related to a more preserved arm function. In contrast, the participants in the study by Nyberg et al [Citation34] had the smallest reduction in lower limb function (∼30% lower) compared with the healthy control group of the three studies [Citation25, Citation26, Citation34], and importantly, the reduction was similar not different between arm and leg muscles.
Methodological considerations
The number of studies that explicitly address the question of differences in physiological responses to arm and leg activities is rather limited. Additionally, differences in the protocols of arm and leg activities, outcomes, and the possible diversities of included individuals with COPD are all potential sources of bias in this systematic review and makes the synthesis of results challenging. The diversity between included studies is explained by differences in the specific research questions, for example regarding disease severity or specific activities, highlighting that the comparison of arm and leg activities could be addressed in numerous ways. In the outcomes included for meta-analyses, high-level of heterogeneity was found for W peak, and several studies had wide confidence intervals for the outcomes, but the studies showed agreement on mean values, and we are confident on the trends.
Clinical considerations
The results from our systematic review supports previous statements that walking or cycling are good choices of exercises to increase cardiopulmonary fitness in patients with COPD [Citation10] since the legs can generate greater central physiological responses compared to the arms. However, if performed at maximal effort, both arm cycle ergometer [Citation25, Citation26] and resistance exercises using the arms [Citation34] did lead to V̇e/MVV greater than 80% (an indication of ventilatory limitation) [Citation43] as well as similar levels of limb fatigue and dyspnea (the latter being a primary contributing factor to exercise tolerance in COPD) [Citation44], compared to leg activities. Thus indicating that also arm activities might be used to target cardiopulmonary fitness. From a clinical perspective, these findings also indicate that adjunct strategies used to facilitate leg training in symptom-limited individuals, such as oxygen supplementation [Citation14], noninvasive ventilation [Citation15] or partitioning exercise [Citation45, Citation46] may be relevant also during arm activities to increase exercise tolerance, even though this needs to be further investigated. Important to notice is that of the included studies that reported Spᴏ2%, all was >85, thus conclusions from this systematic review should not be extrapolated to people with respiratory insufficiency.
Conclusions
To conclude, central physiological responses were in general larger during leg- compared to arm activities except for resistance training exercises, while, about the perception of exertional dyspnea and limb fatigue we conclude that this seems to be task and intensity dependent. Thus, considering the importance of dyspnea as a factor for exercise intolerance, these findings suggest, for example, that strategies used to increase exercise tolerance should not be dependent on whether the arms or the legs are used, but rather the intensity of specific activity performed. Furthermore, the choice of how to prescribe exercise training is multifaceted and we should follow the specificity principle of training and tailor our prescription to the individual, either to maximize central capacity or to maximize specific functions.
Authors’ contributions
AN and TJF are the guarantors and contributed equally to the systematic review. EF, VPL, TJF and AN contributed to the development of the eligibility criteria, the risk of bias assessment strategy, development of search strategy and data extraction criteria. EF, VPL, TJF and AN screened articles for inclusion. EF and HVS extracted the data. EF, HVS and AN drafted the manuscript. EF, HVS, VPL, TJF and AN read, provided feedback and approved the final protocol.
Declaration of interest
The authors have no conflicts of interest to declare.
Additional information
Funding
References
- Beaver WL, Wasserman K, Whipp BJ. A new method for detecting anaerobic threshold by gas exchange. J Appl Physiol. 1986;60(6):2020–2027. doi:10.1152/jappl.1986.60.6.2020.
- Maltais F, Decramer M, Casaburi R, et al. An official American Thoracic Society/European Respiratory Society statement: update on limb muscle dysfunction in chronic obstructive pulmonary disease. Am J Respir Crit Care Med. 2014;189(9):e15–e62. doi:10.1164/rccm.201402-0373ST.
- Gosselink R, Troosters T, Decramer M. Distribution of muscle weakness in patients with stable chronic obstructive pulmonary disease. J Cardiopulm Rehabil. 2000;20(6):353–360. doi:10.1097/00008483-200011000-00004.
- Green HJ, Bombardier E, Burnett M, et al. Organization of metabolic pathways in vastus lateralis of patients with chronic obstructive pulmonary disease. Am J Physiol Regul Integr Comp Physiol. 2008;295(3):R935–R941. doi:10.1152/ajpregu.00167.2008.
- Richardson RS, Leek BT, Gavin TP, et al. Reduced mechanical efficiency in chronic obstructive pulmonary disease but normal peak V̇ᴏ2 with small muscle mass exercise. Am J Respir Crit Care Med. 2004;169(1):89. doi:10.1164/rccm.200305-627OC.
- Mador MJ, Bozkanat E, Kufel TJ. Quadriceps fatigue after cycle exercise in patients with COPD compared with healthy control subjects. Chest 2003;123(4):1104–1111. doi:10.1378/chest.123.4.1104.
- Saey D, Lemire BB, Gagnon P, et al. Quadriceps metabolism during constant workrate cycling exercise in chronic obstructive pulmonary disease. J Appl Physiol. 2011;110(1):116. doi:10.1152/japplphysiol.00153.2010.
- Robles P, Araujo T, Brooks D, et al. Does limb partitioning and positioning affect acute cardiorespiratory responses during strength exercises in patients with COPD? Respirology 2017;22(7):1336–1342. doi:10.1111/resp.13056.
- Lima VP, Iamonti VC, Velloso M, et al. Physiological responses to arm activity in individuals with chronic obstructive pulmonary disease compared with healthy controls: a systematic review. J Cardiopulm Rehabil Prev. 2016;36(6):402–412. doi:10.1097/HCR.0000000000000190.
- Spruit MA, Singh SJ, Garvey C, et al. An official American Thoracic Society/European Respiratory Society statement: key concepts and advances in pulmonary rehabilitation. Am J Respir Crit Care Med. 2013;188(8):e13–e64. doi:10.1164/rccm.201309-1634ST.
- Annegarn J, Meijer K, Passos VL, et al. Problematic activities of daily life are weakly associated with clinical characteristics in COPD. J Am Med Dir Assoc. 2012;13(3):284–290. doi:10.1016/j.jamda.2011.01.002.
- Man WD, Soliman MG, Gearing J, et al. Symptoms and quadriceps fatigability after walking and cycling in chronic obstructive pulmonary disease. Am J Respir Crit Care Med. 2003;168(5):562–567. doi:10.1164/rccm.200302-162OC.
- McKeough ZJ, Alison JA, Bye P. Arm exercise capacity and dyspnea ratings in subjects with chronic obstructive pulmonary disease. J Cardiopulm Rehabil. 2003;23(3):218. doi:10.1097/00008483-200305000-00010.
- Carter R, Holiday DB, Stocks J, et al. Peak physiologic responses to arm and leg ergometry in male and female patients with airflow obstruction. Chest 2003;124(2):511–518. doi:10.1016/S0012-3692(15)33332-8.
- Porto EF, Castro AAM, Velloso M, et al. Exercises using the upper limbs hyperinflate COPD patients more than exercises using the lower limbs at the same metabolic demand. Monaldi Arch Chest Dis. 2009;71(1):21–26. doi:10.4081/monaldi.2009.372.
- Liberati A, Altman DG, Tetzlaff J, et al. The PRISMA statement for reporting systematic reviews and meta-analyses of studies that evaluate healthcare interventions: explanation and elaboration. BMJ 2009;339:b2700.
- Frykholm E, Lima VP, Janaudis-Ferreira T, et al. Physiological responses to arm versus leg activity in patients with chronic obstructive pulmonary disease: a systematic review protocol. BMJ Open. 2018;8(2):e019942. doi:10.1136/bmjopen-2017-019942.
- Siafakas NM, Vermeire P, Pride NB, et al. Optimal assessment and management of chronic obstructive pulmonary disease (COPD). The European Respiratory Society Task Force. Eur Respir J. 1995;8(8):1398–1420. doi:10.1183/09031936.95.08081398.
- Celli B, Macnee W, Agusti A, et al. Standards for the diagnosis and treatment of patients with COPD: a summary of the ATS/ERS position paper. Eur Respir J. 2004;23(6):932–946. doi:10.1183/09031936.04.00014304.
- Celli B, Snider G, Heffner J, et al. Standards for the diagnosis and care of patients with chronic obstructive pulmonary disease. Am J Respir Crit Care Med. 1995;152(5 Pt 2):S77–S121.
- COPD Guidelines Group of the Standards of Care Committee of the BTS. BTS guidelines for the management of chronic obstructive pulmonary disease. Thorax 1997;52:1.
- Borg G. Perceived exertion and pain scales. Champaign (IL): Human Kinetics; 1998.
- Downs SH, Black N. The feasibility of creating a checklist for the assessment of the methodological quality both of randomised and non-randomised studies of health care interventions. J Epidemiol Commun Health. 1998;52(6):377–384. doi:10.1136/jech.52.6.377.
- Higgins JPT, Thompson SG, Deeks JJ, et al. Measuring inconsistency in meta-analyses. Br Med J. 2003;327(7414):557–560. doi:10.1136/bmj.327.7414.557.
- Castagna O, Boussuges A, Vallier JM, et al. Is impairment similar between arm and leg cranking exercise in COPD patients? Respir Med. 2007;101(3):547–553. doi:10.1016/j.rmed.2006.06.019.
- Franssen FME, Wouters EFM, Baarends EM, et al. Arm mechanical efficiency and arm exercise capacity are relatively preserved in chronic obstructive pulmonary disease. Med Sci Sports Exerc. 2002;34(10):1570–1576. doi:10.1097/00005768-200210000-00007.
- Hannink JD, Van Helvoort HA, Dekhuijzen PN, et al. Similar dynamic hyperinflation during arm and leg exercise at similar ventilation in chronic obstructive pulmonary disease. Med Sci Sports Exerc 2011;43:996–1001. doi:10.1249/MSS.0b013e318205e2be
- Owens GR, Thompson FE, Sciurba FC, et al. Comparison of arm and leg ergometry in patients with moderate chronic obstructive lung disease. Thorax 1988;43(11):911. doi:10.1136/thx.43.11.911.
- McKeoughl ZJ, Alison JA, Bayfield MS, et al. Supported and unsupported arm exercise capacity following lung volume reduction surgery: a pilot study. Chron Respir Dis. 2005;2(2):59–65. doi:10.1191/1479972305cd074oa.
- Adami BA, Corvino VR, Casaburi AR, et al. Muscle oxidative capacity is low in the upper and lower limbs of COPD patients. Med Sci Sports Exerc. 2017;49:1045. doi:10.1249/01.mss.0000519869.59500.28.
- Miranda EF, Malaguti C, Marchetti PH, et al. Upper and lower limb muscles in patients with COPD: similarities in muscle efficiency but differences in fatigue resistance. Respir Care. 2014;59(1):62. doi:10.4187/respcare.02439.
- Nyberg A, Lindström B, Rickenlund A, et al. Low‐load/high‐repetition elastic band resistance training in patients with COPD: a randomized, controlled, multicenter trial. Clin Respir J. 2015;9(3):278–288. doi:10.1111/crj.12141.
- Nyberg A, Saey D, Martin M, et al. Acute effects of low-load/high-repetition single-limb resistance training in COPD. Med Sci Sports Exerc. 2016;48(12):2353–2361. doi:10.1249/MSS.0000000000001027.
- Nyberg A, Saey D, Martin M, et al. Cardiorespiratory and muscle oxygenation responses to low-load/high-repetition resistance exercises in COPD and healthy controls. J Appl Physiol. 2018;124(4):877. doi:10.1152/japplphysiol.00447.2017.
- Nyberg A, Saey D, Martin M, et al. Muscular and functional effects of partitioning exercising muscle mass in patients with chronic obstructive pulmonary disease - a study protocol for a randomized controlled trial. Trials 2015;16(1):194. doi:10.1186/s13063-015-0698-x.
- Celli BR, Rassulo J, Make BJ. Dyssynchronous breathing during arm but not leg exercise in patients with chronic airflow obstruction. N Engl J Med. 1986;314(23):1485–1490. doi:10.1056/NEJM198606053142305.
- de Souza GF, Castro AA, Velloso M, et al. Lactic acid levels in patients with chronic obstructive pulmonary disease accomplishing unsupported arm exercises. Chron Respir Dis. 2010;7(2):75–82. doi:10.1177/1479972310361833.
- Marrara KT, Marino DM, de Held PA, et al. Different physical therapy interventions on daily physical activities in chronic obstructive pulmonary disease. Respir Med. 2008;102(4):505–511. doi:10.1016/j.rmed.2007.12.004.
- Silva CS, Nogueira FR, Porto EF, et al. Dynamic hyperinflation during activities of daily living in COPD patients. Chron Respir Dis. 2015;12(3):189–196. doi:10.1177/1479972315576143.
- Larsen RT, Christensen J, Tang LH, et al. A systematic review and meta-analysis comparing cardiopulmonary exercise test values obtained from the arm cycle and the leg cycle respectively in healthy adults. Int J Sports Phys Ther. 2016;11(7):1006. doi:10.1097/CPT.0000000000000081.
- Dolmage TE, Maestro L, Avendano MA, et al. The ventilatory response to arm elevation of patients with chronic obstructive pulmonary disease. Chest 1993;104(4):1097–1100. doi:10.1378/chest.104.4.1097.
- Senefeld J, Yoon T, Bement MH, et al. Fatigue and recovery from dynamic contractions in men and women differ for arm and leg muscles. Muscle Nerve. 2013;48(3):436–439. doi:10.1002/mus.23836.
- Forman DE, Myers J, Lavie CJ, et al. Cardiopulmonary exercise testing: relevant but underused. Postgrad Med. 2010;122(6):68–86. doi:10.3810/pgm.2010.11.2225.
- Ambrosino N, Strambi S. New strategies to improve exercise tolerance in chronic obstructive pulmonary disease. Eur Respir J. 2004;24(2):313–322. doi:10.1183/09031936.04.00002904.
- Dolmage TE, Goldstein RS. Effects of one-legged exercise training of patients with COPD. Chest 2008;133(2):370–376. doi:10.1378/chest.07-1423.
- Dolmage TE, Goldstein RS. Response to one-legged cycling in patients with COPD. Chest 2006;129(2):325–332. doi:10.1378/chest.129.2.325.