Abstract
Chronic obstructive pulmonary disease (COPD) is a complex multi-morbid disorder with significant cardiac mortality. Current cardiovascular risk prediction models do not include COPD. We investigated whether COPD modifies future cardiovascular risk to determine if it should be considered in risk prediction models.
Case-control study using baseline data from two randomized controlled trials performed between 2012 and 2015. Of the 90 eligible subjects, 26 COPD patients with lung hyperinflation were propensity matched for 10-year global cardiovascular risk score (QRISK2) with 26 controls having normal lung function. Patients underwent cardiac magnetic resonance imaging, arterial stiffness and lung function measurements. Differences in pulse wave velocity (PWV), total arterial compliance (TAC) and aortic distensibility were main outcome measures.
PWV (mean difference 1.0 m/s, 95% CI 0.02–1.92; p = 0.033) and TAC (mean difference –0.27 mL/m2/mmHg, 95% CI 0.39–0.15; p < 0.001) were adversely affected in COPD compared to the control group. The PWV difference equates to an age, sex and risk-factor adjusted increase in relative risk of cardiovascular events and mortality of 14% and 15%, respectively.
There were no differences in aortic distensibility. In the whole cohort (n = 90) QRISK2 (β = 0.045, p = 0.005) was associated with PWV in multivariate analysis. The relationship between QRISK2 and PWV were modified by COPD, where the interaction term reached significance (p = 0.014). FEV1 (β = 0.055 (0.027), p = 0.041) and pulse (B = –0.006 (0.002), p = 0.003) were associated with TAC in multivariate analysis.
Markers of cardiovascular outcomes are adversely affected in COPD patients with lung hyperinflation compared to controls matched for global cardiovascular risk. Cardiovascular risk algorithms may benefit from the addition of a COPD variable to improve risk prediction and guide management.
HAPPY London ClinicalTrials.gov: NCT01911910 and HZC116601; ClinicalTrials.gov: NCT01691885
Introduction
Chronic obstructive pulmonary disease (COPD) is predicted to be the third leading cause of death worldwide by 2020 [Citation1]. It is a complex multi-morbid disorder in which up to 37% succumb to cardiovascular causes rather than respiratory failure [Citation2]. The precise mechanisms contributing to cardiovascular risk in COPD are not yet fully elucidated but lung hyperinflation and systemic inflammation are postulated as possible mechanisms [Citation3,Citation4].
Predicting prognosis in COPD has proven difficult. Airflow limitation measured by the forced expiratory volume in 1 s (FEV1) in combination with airflow obstruction (FEV1/Forced vital capacity (FVC)) is the hallmark of COPD. Used in isolation, these parameters only show weak association with all-cause mortality in COPD and therefore been combined in multi-dimensional risk assessments to improve predictive value [Citation5,Citation6]. It has been suggested that a reduction in FEV1, combined with a smoking history is a better predictor of cardiovascular mortality than cholesterol [Citation7]. Despite this, the current global cardiovascular risk scores, which use algorithms for estimating cardiovascular risk, and have been developed and advocated by cardiovascular prevention guidelines to communicate risk and facilitate treatment decisions [Citation8–10], do not factor in COPD severity, raising the possibility that risk estimation may be sub-optimal [Citation11].
Aortic distensibility, total arterial compliance (TAC) and left ventricular mass (LVM) have been identified as cardiac magnetic resonance (CMR) surrogates of cardiovascular risk, a modality which provides unparalleled image quality noninvasively with excellent accuracy and reproducibility [Citation12–14]. Carotid-femoral pulse wave velocity (PWV), a noninvasive bedside measure of global arterial stiffness, is also an independent predictor of coronary artery disease [Citation13,Citation15,Citation16].
The aim of this study was to assess whether differences exist in cardiovascular surrogate markers in COPD compared to controls with normal lung function, when matched for global cardiovascular risk. We hypothesize that differences exist and COPD may be considered as a cardiovascular risk factor.
Material and methods
Patients
This post-hoc case-control analysis utilized baseline data from two randomized controlled trials undertaken between November 2012 to May 2015 at our center with matched protocol for assessed parameters. Patients were propensity matched by QRISK2 score ± 2% (a United Kingdom based validated 10-year cardiovascular risk algorithm) [Citation17]. All participants gave written informed consent. The study was approved by the national Research Ethics Committee (NRES committee – London) and was conducted in accordance with the declaration of Helsinki. Participants were consented for use of data different from those of the original study.
COPD group
The COPD group consisted of 45 consecutive patients recruited to a clinical trial involving stable hyperinflated COPD patients [Citation18]. The diagnosis of COPD was confirmed according to published criteria using the lower limit of normal for FEV1 and ratio of FEV1 to FVC for all COPD patients [Citation19]. Patients were aged over 40 years with at least 15 pack-year smoking history and evidence of lung hyperinflation on body plethysmograph (residual volume >120% of predicted) with no history of COPD exacerbation in the preceding 4 weeks. All patients with known cardiovascular disease (7 individuals) or atrial fibrillation (2 individuals) were excluded, leaving 36 evaluable hyperinflated COPD patients. There was a washout period of at least 48 h for long acting beta-2 agonists, 4 days for long acting muscarinic antagonists and at least 6 h for the short acting bronchodilators prior the CMR and PVW assessments.
Control group with known cardiovascular risk
The control group was drawn from imaging subgroup of 96 out of the total of study population of 402 participants with global 10-year cardiovascular risk of ≥10% based on QRISK2, recruited to the Heart Attack Prevention Program for You (HAPPY) London primary prevention randomized controlled trial aiming to reduce cardiovascular risk in a cohort free of preexisting cardiovascular disease [Citation20]. Only those that underwent CMR imaging with normal spirometry and absence of respiratory disease or atrial fibrillation were included, leaving 54 evaluable subjects.
Spirometry
Spirometry was performed using equipment meeting the minimum performance recommendations of the American Thoracic Society/European Respiratory Society task force (Microlab3500, Micromedical, UK) [Citation21]. At least three valid spirometry efforts were attempted, but not more than eight. Residual volume, total lung capacity and functional residual capacity z-scores for the COPD group were calculated from published reference ranges [Citation22].
Static lung volumes, measured using whole body plethysmography (ZAN500, Germany) and carbon monoxide transfer factor, via a single breath hold technique (CPL PFT, United States), were assessed according to manufacturers’ instructions [Citation18].
Cardiovascular magnetic resonance
CMR images were analyzed from baseline scans performed on a 1.5 T CMR system (Achieva, Philips, Netherlands) using a Software release 3.2 and Cardiac package installed. ISS performed analysis for the COPD group, while MYK performed analysis for the control group. Ventricular (both groups) and atrial (COPD group only) volumes and function data were acquired according to local protocol and international guidance [Citation23]. All participants were specifically advised to refrain from caffeine, alcohol and smoking for at least 8 h prior to the CMR and PWV assessments. The endocardial contours of the ventricle and atria were manually segmented and summed for the whole ventricle using semi-automated software (CVI42, Circle Cardiovascular imaging Inc., Calgary, Canada) to quantify end-diastolic (EDV), end-systolic volumes (ESV), ejection fraction and stroke volume (SV) for the left atrium and both left (LV) and right (RV) ventricles. Values were indexed (denoted by letter “I”) to body surface area as determined by the Mosteller formula [Citation24]. Cardiac Index was calculated according to the following formula: SVI × pulse. Epicardial contours were manually segmented at end-diastole for the left ventricle to allow the calculation of indexed LV mass (LVMI).
Local arterial stiffness: CMR aortic distensibility measurements
Two SSFP cine images were acquired during end-expiration in planes perpendicular to the thoracic aorta at the level of the pulmonary artery (thoracic ascending aorta (TAA) and thoracic descending aorta (TDA), with further image acquired 10 cm below this for the abdominal aorta (ABA). Brachial blood pressure was measured using a CMR compatible oscillatory sphygmomanometer (Vicorder, Skidmore medical, UK) and central blood pressure estimated using a validated transfer function used in calculating distensibility where distensibility (%/mmHg) = [(maximum area – minimum area)/pulse pressure × minimum area] × 100 [Citation25,Citation26]. Minimum and maximum values for cross sectional areas were derived using an in-house validated automated endoluminal border-tracking program written in MatLab (v.7.5).
Global arterial stiffness: PWV and TAC
PWV were obtained using Vicorder device as described previously, according to manufacturer’s guidelines [Citation27]. Briefly, for PWV measurements the path-length was calculated from the suprasternal notch to a defined point on the upper part of the femoral cuff. The foot-to-foot transit time was measured as described previously and values for cfPWV were derived automatically [Citation28]. Measurements were performed in a supine position, after 10 min rest, outside the CMR scanner and prior to lung function maneuvers. All measurements were repeated at least twice and the mean value of consistent measures was derived. TAC was derived by the following formula: SVI/central pulse pressure
Statistics
Matching of the groups was performed using SAS (SAS Institute Inc., Cary, NC, US, Version 9.3). Patients were matched by QRISK2 score ± 2% to test the initial hypothesis. Statistical analysis was performed using SPSS 21.0 for Mac (SPSS Inc., Chicago, Illinois, USA). The distribution of the data was assessed visually. Continuous variables were expressed as mean ± SD for parametric variables and median (interquartile range) for non-parametric variables. Differences between the COPD and controls were assessed using paired t-tests. Univariate followed by multivariate linear regression analysis was used to evaluate associations between patient variable and the surrogate endpoints that showed differences between the groups. Cohort adjusted effects were obtained by including COPD status as a factor in the models. Variables for inclusion in the multivariate models were selected using all-subsets variable selection using the Bayesian information criterion to select the final model. A term for COPD status was forced in to all models to account for differences between the two studies. Models were validated using 5-fold cross validation repeated 100 times. Where an association was found, further regression analyses were performed on the interaction terms to establish whether the presence or absence of COPD as a binary variable had any impact on the relationship between QRISK2 and the cardiovascular surrogates. Thus, the matched design was used initially to look at differences between the two groups. Subsequently, we performed a multivariate analysis using all the data from both cohorts and further analyzed the data to control for variables that were different between the two groups (including hypertension, diabetes and smoking). The extent of intra-observer agreement was assessed using Bland-Altman method on 20 randomly selected patients (10 from each cohort) for the CMR measures and on 16 of the HAPPY London cohort for Vicorder measures of PWV and aortic pulse pressure [Citation29]. Statistical significance was defined as a two-sided p < 0.05.
Results
Of the 36 eligible COPD patients, 26 were successfully matched for 10-year global cardiovascular risk ± 2% based on QRISK2 score with 26 of the 54 HAPPY London participants with normal lung function. Baseline demographics and pulmonary function of the 52 matched individuals are shown in . As expected, there were no differences in QRISK2 score (p = 0.693), age (p = 0.447), sex (p = 0.161), blood pressure (p = 0.447), renal function (p = 0.055) or cholesterol treatment (p = 0.449) between the groups. The control group had a higher prevalence of diabetes, whilst the COPD group had more impaired pulmonary function and significant smoking history leading to similar QRISK2 scores.
Table 1. Demographic and pulmonary function characteristics of COPD and control groups matched for global cardiovascular risk.
Inter-observer agreement
The Bland Altman plots (Supplementary Figure S1) confirmed acceptable agreement between ISS and MYK measurements of PWV (bias 0.43 m/s, limits of agreement (LOA) –0.9, 1.76, Intra-class correlation coefficient (ICC) 90.5%), aortic pulse pressure (bias –1.14 mmHg, LOA –22.9, 23.2, ICC 86.4%), aortic relative area change (TAA bias 9.3 × 10−3, LOA –0.06, 0.82, ICC 80.2%; TDA bias 1.4 × 10−3, LOA –0.02,0.02, ICC 97.9%; ABA bias –2.9 × 10−3 LOA –0.02, 0.03, ICC 99.2%) left ventricle end-diastolic volume index (LVEDVI) (bias –3.6 mL/m2 LOA –12.5, 5.8, ICC 96.6%), left ventricular mass index (LVMI) (bias –2.9 g/m2, LOA –13.65, 7.83, ICC 87.5%) and left ventricular SV index (LVSVI) (bias 2.0 mL/m2 LOA –2.5, 8.5, ICC 92.5%).
Arterial stiffness
Global arterial stiffness measures of PWV and TAC were adversely affected in COPD compared to the matched control group. PWV was higher in the COPD group compared to controls with a mean difference of 1.0 m/s (95% CI 0.1, 1.9; p = 0.033), whereas TAC was lower by –0.27 mL/m2/mmHg (95% CI –0.4, –0.2; p < 0.001 (; ).
Figure 1. Differences in PWV, TAC and their relationship to QRISK2 in COPD compared to controls matched for cardiovascular risk. Markers of cardiovascular outcomes adversely affected in COPD patients compared to controls matched for global cardiovascular risk.
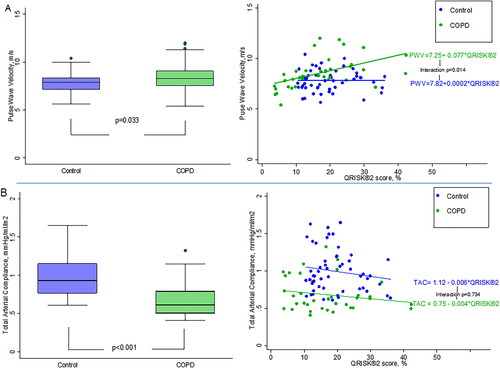
Table 2. Comparison of cardiovascular endpoints between COPD and control group matched for global cardiovascular risk.
Local arterial stiffness measured using aortic distensibility, although numerically lower in COPD compared to controls in all three regions analyzed, showed no statistical differences (mean difference TAA: –0.41%/mmHg ×10−3 95% CI –0.9, 0.1, p = 0.088; TDA –0.29%/mmHg ×10−3 95% CI –0.8, 0.2 p = 0.216; ABA –0.27%/mmHg ×10−3 95% CI –1.2, 0.6, p = 0.536).
Ventricular mass, size and function
No differences in LVMI were identified between groups (mean difference 2.8 g/m2; 95% CI –2.5, 8.1, p = 0.291) (). Chamber size was smaller in COPD group compared to the controls with mean differences in LVEDVI and RVEDVI of –14.1 mL/m2 (95% CI –22.1, –6.3, p = 0.001) and –13.0 (95% CI –23.9, –2.9, p = 0.022), respectively. There was a corresponding lower LVSVI (mean difference –10.3 mL/m2 95% CI –15.4, –5.3, p < 0.001) but no differences in LV ejection fraction. Despite lower SV, cardiac index was similar as a consequence of a higher heart rate in the COPD group (76 ± 14 vs. 63 ± 11 beats/min, p = 0.001).
Baseline demographics and pulmonary function for the COPD and control groups making up the 90-patient cohort are shown in Supplementary Table S1. The results of the univariate and multivariate analyses for PWV and TAC for the whole 90 patients are shown in and Citation4, respectively. In the first model (where QRISK2 is entered but age, sex and SBP are excluded) QRISK2 was associated with PWV in the multivariate analysis, whereby a 10% increase in QRISK2 was associated with 0.45 m/s higher PWV when adjusting for other co-variates in the model. However, the relationship between QRISK2 and PWV differed when stratified according to presence or absence of COPD (COPD group r2 = 0.260; control group r2 = 0.003, which was significant when an interaction term was included in the model (p = 0.014) (.
Table 3. Univariate and cohort adjusted predictors of PWV and TAC.
Table 4. Multivariate predictors of PWV and TAC.
In the second multivariate model (which includes age, sex and SBP but not QRISK2), age and SBP enter the model. A 10-year increase in age is associated with a 0.7 m/s higher PWV, while a 10 mmHg increase in SBP results in a 0.30 m/s higher PWV. There is a significant interaction between SBP and COPD group (p = 0.019). A 10 mmHg increase in SBP associated with a significant (0.40 m/s, SE = 0.08) increase for COPD, with no significant effect (0.07 m/s, SE = 0.12) for controls. The R2 for the model including the interaction term is 49.3%, suggesting a better fit with the individual components of age and SBP in the model rather than QRISK2 (R2 = 21.5%). Differences remained significant between the COPD and control groups following sensitivity analysis to control for the baseline differences (Supplementary Table S2).
Discussion
The principle novel findings of our study are that PWV and TAC, known independent predictors of cardiovascular disease, are adversely affected in stable hyperinflated COPD over and above a cohort considered to have equivalent global cardiovascular risk but normal lung function. There appears to be an interaction between COPD and QRISK2 with regard to its relationship to PWV.
Concerns have previously been raised about the accuracy of a number of different scoring systems and possible over-estimation of risk in the general population [Citation30]. We have found PWV in our COPD cohort to be 1.0 m/s higher than in matched non-COPD subjects which would equate to an age, sex and risk-factor adjusted increase in relative risk of cardiovascular events and mortality of 14% and 15%, respectively [Citation31,Citation32]. Furthermore, we have shown a clear interaction between COPD and the QRISK2 in relation to PWV (). COPD has an estimated UK prevalence of 13.5% in those over 35 years of age and when assessing cardiovascular risk in this group using smoking status alone may not optimally predict cardiovascular risk in this common disease. Whilst it has been shown that, the addition of Framingham risk score to FEV1 improves risk stratification for cardiovascular events compared to FEV1 alone, our findings importantly suggest that the inclusion of COPD may improve the predictive ability of cardiovascular risk scores and should be further confirmed in larger population studies [Citation33]. Secondly, this interaction implies that COPD could potentially act as a modifiable risk factor. This is a concept supported by previous post-hoc analyses of large randomized controlled trials and two more recent randomized controlled trials where the treatment of COPD with conventional therapies have led to a reduction in PWV [Citation34–37].
The impact of blood pressure in COPD patients appears to be exaggerated based on our findings and suggests the need for tighter control in this group for primary prevention of cardiovascular events, although randomized controlled trials in this area are lacking [Citation38].
Non-pharmacological treatment (including increased physical activity and smoking cessation) and antihypertensive medication need to be integral in the reduction of future CVD in this group. Relying on current CVD risk scoring systems alone, such as QRISK2, may not optimally identify high-risk individuals who may not receive guideline based treatments that are based on risk thresholds [Citation11].
Mechanisms proposed for the association between PWV and COPD include systemic inflammation and the effects of hyperinflation on neurohumoral activation [Citation3]. Computed tomography defined emphysema has been associated with PWV, whilst reports linking systemic inflammation to PWV in COPD have been inconsistent [Citation39]. Sabit et al. found relationships with Interleukin (IL)-6, whereas a more recent study found no relationship with leukocytes, C-reactive protein, IL-6, IL-8 or soluble tumor necrosis factor receptor pathway 2. Whilst we also found no relationship with leucocytes, we have found a relationship between PWV and fibrinogen, a marker of systemic inflammation in both COPD and cardiovascular disease [Citation40,Citation41]. An accurate understanding of the role of fibrinogen in the relationship between COPD and cardiovascular disease is under evaluation, but if confirmed could act as a potential therapeutic target [Citation42].
TAC has been shown to be a predictor of cardiovascular events, in normal individuals free from cardiovascular disease, hypertensives and elderly [Citation43,Citation44]. However, unlike PWV we found no relationship with QRISK2 in univariate or multivariate analysis. Arterial stiffness measures are surrogate measures of end-organ disease representing an index of the summed effects of aging and exposure. However, these surrogate measures have varying abilities to predict particular types of cardiovascular events. Whilst the QRISK2 score is designed to predict both the risk of myocardial infarction and stroke, TAC when measured using CMR has been shown to be independently associated with non-fatal cardiac events only, including hospitalization for congestive heart failure and arrhythmia [Citation14]. This may in part explain the lack of relationship.
We have confirmed the findings of previous studies, which were limited by lacking suitable control groups and/or the inclusion of patient populations with more severe disease, that COPD patients have smaller cardiac chambers and SVs, and maintain cardiac output through a compensatory increase in heart rate [Citation45–48]. The cause of the smaller cardiac chamber size is thought to be a pre-load effect [Citation18,Citation45,Citation46,Citation49]. Our group has previously demonstrated that lung deflation in the short-term result in at least partial reversal of these effects, with decompression of the cardiac chambers, improvements in SV, cardiac output and atrial ejection fraction [Citation50]. Long-term implications of these findings on heart failure and arrhythmia, increased in COPD, are as yet unknown, but may identify another therapeutic target for the prevention of cardiac co-morbidity in COPD [Citation51]. At present COPD remains a risk factor for heart failure mortality and has been incorporated into risk scores accordingly [Citation52]. The findings presented here add weight to the belief that the same should considered for cardiovascular risk. COPD prevalence is higher than rheumatoid arthritis, a condition, which is already included in QRISK2 score. An estimated 1 million COPD patients in the UK alone are undiagnosed [Citation31]. The inclusion of COPD could potentially improve risk estimation, provision of lifestyle advise and intervention [Citation53], and promote the early diagnosis of COPD through increased usage of pulmonary function testing and the availability of pulmonary function data on primary and secondary care databases.
Limitations
The results have to be interpreted in the context of the study design. This is a post-hoc cross-sectional analysis so we are able to establish association but not causation. Given the relatively small sample size, these findings should be interpreted with caution and replicated on a larger scale. The COPD cohort in our study all had RV > 120% of predicted, thus further research is required to see if the results can be generalized to patients with milder COPD, lower RV or those with differing clinical phenotypes. Although the COPD and control group were matched for global cardiovascular risk, it is difficult to accurately quantify the impact of the variations in individual risk factors and medication use on the outcome measures. Our primary purpose was to investigate the applicability of cardiovascular risk scores to patients with COPD by way of assessing surrogates of cardiac risk and as such our investigation regarding the proposed mechanisms surrounding increased risk have not been exhaustive.
Conclusion
PWV and TAC are adversely affected in hyperinflated COPD compared to a group matched for global cardiovascular risk. The relationship between cardiovascular risk scores and PWV appears to be modified by COPD. Further research is needed to assess if CVD risk algorithms may benefit from the addition of a COPD variable to improve risk prediction and guide management, given its common occurrence and associated high cardiovascular morbidity and mortality.
Declaration of interest
This work forms part of the research areas contributing to the translational research portfolio of the NIHR Barts Biomedical Research Center. Data were collected and analyzed by the investigators.
N.C.B is now an employee of GlaxoSmithKline (GSK)
Authors contribution
MYK and ISS conceived and designed this study. MYK and ISS finalized the protocol. MYK, ISS, RB, NCB, and SEP collected the data. MYK, ISS, SEP, and RB performed the cardiac magnetic resonance analysis. MYK, ISS and JC, performed the statistical analysis. MYK, ISS, SEP, NCB, and JC analyzed and interpreted the data. MYK and ISS wrote the first draft. All authors reviewed, edited, and approved the final draft of the manuscript and agreed in the decision to submit for publication.
Acknowledgments
We would like to thank all the participants in the study and the staff at the William Harvey Heart Center and The Barts cardiovascular magnetic resonance imaging department for their contribution in obtaining the data and assisting in the successful completion of both the COPD and HAPPY London studies.
Data sharing
Anonymized individual participant data from study HZC 116601 (NCT01691885) and related documents can be requested for further research from www.clinicalstudydatarequest.com.
Additional information
Funding
References
- Murray CJ, Lopez AD. Global mortality, disability, and the contribution of risk factors: global burden of disease study. Lancet. 1997;349(9063):1436–1442. doi:10.1016/S0140-6736(96)07495-8.
- Sin DD, Anthonisen NR, Soriano JB, et al. Mortality in COPD: role of comorbidities. Eur Respir J. 2006;28(6):1245–1257. doi:10.1183/09031936.00133805.
- Stone IS, Barnes NC, Petersen SE. Chronic obstructive pulmonary disease: a modifiable risk factor for cardiovascular disease? Heart 2012;98(14):1055–1062. doi:10.1136/heartjnl-2012-301759.
- Christakis NA, Escarce JJ. Survival of Medicare patients after enrollment in hospice programs. N Engl J Med. 1996;335(3):172–178. doi:10.1056/NEJM199607183350306.
- Celli BR, Cote CG, Marin JM, et al. The body-mass index, airflow obstruction, dyspnea, and exercise capacity index in chronic obstructive pulmonary disease. N Engl J Med. 2004;350(10):1005–1012. doi:10.1056/NEJMoa021322.
- Puhan MA, Hansel NN, Sobradillo P, et al. Large-scale international validation of the ADO index in subjects with COPD: an individual subject data analysis of 10 cohorts. BMJ Open. 2012;2(6):e002152. doi:10.1136/bmjopen-2012-002152.
- Hole DJ, Watt GC, Davey Smith G, et al. Impaired lung function and mortality risk in men and women: findings from the Renfrew and Paisley prospective population study. BMJ 1996;313(7059):711–715. Discussion 715–6. doi:10.1136/bmj.313.7059.711.
- Collins GS, Altman DG. Predicting the 10-year risk of cardiovascular disease in the United Kingdom: independent and external validation of an updated version of QRISK2. BMJ 2012;344:e4181. doi:10.1136/bmj.e4181.
- Conroy RM, Pyörälä K, Fitzgerald AP, et al. Estimation of ten-year risk of fatal cardiovascular disease in Europe: the SCORE project. Eur Heart J. 2003;24(11):987–1003. doi:10.1016/S0195-668X(03)00114-3.
- Stone NJ, Robinson JG, Lichtenstein AH, et al. ACC/AHA guideline on the treatment of blood cholesterol to reduce atherosclerotic cardiovascular risk in adults: a report of the American College of Cardiology/American Heart Association Task Force on Practice Guidelines. J Am Coll Cardiol. 2013;2014:2889–2934.
- Khanji MY, Bicalho VVS, van Waardhuizen CN, et al. Cardiovascular risk assessment: a systematic review of guidelines. Ann Intern Med. 2016;165(10):713–722. doi:10.7326/M16-1110.
- Redheuil A, Wu CO, Kachenoura N, et al. Proximal aortic distensibility is an independent predictor of all-cause mortality and incident CV events: the MESA study. J Am Coll Cardiol. 2014;64(24):2619–2629. doi:10.1016/j.jacc.2014.09.060.
- Laurent S, Boutouyrie P, Asmar R, et al. Aortic stiffness is an independent predictor of all-cause and cardiovascular mortality in hypertensive patients. Hypertension 2001;37(5):1236–1241. doi:10.1161/01.HYP.37.5.1236.
- Maroules CD, Khera A, Ayers C, et al. Cardiovascular outcome associations among cardiovascular magnetic resonance measures of arterial stiffness: the Dallas heart study. J Cardiovasc Magn Reson. 2014;16(1):33. doi:10.1186/1532-429X-16-33.
- Weber T, Auer J, O’Rourke MF, et al. Arterial stiffness, wave reflections, and the risk of coronary artery disease. Circulation 2004;109(2):184–189. doi:10.1161/01.CIR.0000105767.94169.E3.
- Willum Hansen T, Staessen JA, Torp-Pedersen C, et al. Prognostic value of aortic pulse wave velocity as index of arterial stiffness in the general population. Circulation 2006;113(5):664–670. doi:10.1161/CIRCULATIONAHA.105.579342.
- Hippisley-Cox J, Coupland C, Vinogradova Y, et al. Predicting cardiovascular risk in England and Wales: prospective derivation and validation of QRISK2. BMJ 2008;336(7659):1475–1482. doi:10.1136/bmj.39609.449676.25.
- Stone IS, Barnes NC, James W-Y, et al. Lung deflation and cardiovascular structure and function in chronic obstructive pulmonary disease. A randomized controlled trial. Am J Respir Crit Care Med. 2016;193(7):717–726. doi:10.1164/rccm.201508-1647OC.
- Quanjer PH, Stanojevic S, Cole TJ, et al. Multi-ethnic reference values for spirometry for the 3-95-yr age range: the global lung function 2012 equations. Eur Respir J. 2012;40(6):1324–1343. doi:10.1183/09031936.00080312.
- Khanji MY, Balawon A, Boubertakh R, et al. Personalized E-coaching in cardiovascular risk reduction: a randomized controlled trial. Ann Glob Health 2019;85(1):107.
- Miller MR, Hankinson J, Brusasco V, et al. Standardisation of spirometry. Eur Respir J. 2005;26(2):319–338. doi:10.1183/09031936.05.00034805.
- Gutierrez C, Ghezzo RH, Abboud RT, et al. Reference values of pulmonary function tests for Canadian Caucasians. Can Respir J. 2004;11(6):414–424. doi:10.1155/2004/857476.
- Mosteller RD. Simplified calculation of body-surface area. N Engl J Med. 1987;317(17):1098.doi:10.1056/NEJM198710223171717.
- O'Rourke MF. Influence of ventricular ejection on the relationship between central aortic and brachial pressure pulse in man. Cardiovasc Res. 1970;4:291–300. doi:10.1093/cvr/4.3.291.
- Sanz J, Kariisa M, Dellegrottaglie S, et al. Evaluation of pulmonary artery stiffness in pulmonary hypertension with cardiac magnetic resonance. JACC Cardiovasc Imaging. 2009;2(3):286–295. doi:10.1016/j.jcmg.2008.08.007.
- Stone IS, John L, Petersen SE, et al. Reproducibility of arterial stiffness and wave reflections in chronic obstructive pulmonary disease: the contribution of lung hyperinflation and a comparison of techniques. Respir Med. 2013;107(11):1700–1708. doi:10.1016/j.rmed.2013.06.008.
- Hickson SS, Butlin M, Broad J, et al. Validity and repeatability of the Vicorder apparatus: a comparison with the SphygmoCor device. Hypertens Res. 2009;32(12):1079–1085. doi:10.1038/hr.2009.154.
- Bland JM, Altman DG. Statistical methods for assessing agreement between two methods of clinical measurement. Lancet. 1986;1:307–310. doi:10.1016/S0140-6736(86)90837-8.
- DeFilippis AP, Young R, Carrubba CJ, et al. An analysis of calibration and discrimination among multiple cardiovascular risk scores in a modern multiethnic cohort. Ann Intern Med. 2015;162(4):266–275. doi:10.7326/M14-1281.
- Shahab L, Jarvis MJ, Britton J, et al. Prevalence, diagnosis and relation to tobacco dependence of chronic obstructive pulmonary disease in a nationally representative population sample. Thorax 2006;61(12):1043–1047. doi:10.1136/thx.2006.064410.
- Vlachopoulos C, Aznaouridis K, O'Rourke MF, et al. Prediction of cardiovascular events and all-cause mortality with central haemodynamics: a systematic review and meta-analysis. Eur Heart J. 2010;31(15):1865–1871. doi:10.1093/eurheartj/ehq024.
- Lee HM, Lee J, Lee K, et al. Relation between COPD severity and global cardiovascular risk in US adults. Chest 2012;142(5):1118–1125. doi:10.1378/chest.11-2421.
- Celli B, Decramer M, Kesten S, et al. Mortality in the 4-year trial of tiotropium (UPLIFT) in patients with chronic obstructive pulmonary disease. Am J Respir Crit Care Med. 2009;180(10):948–955. doi:10.1164/rccm.200906-0876OC.
- Pepin J-L, Cockcroft JR, Midwinter D, et al. Long-acting bronchodilators and arterial stiffness in patients with COPD: a comparison of fluticasone furoate/vilanterol with tiotropium. Chest 2014;146(6):1521–1530. doi:10.1378/chest.13-2859.
- Dransfield MT, Cockcroft JR, Townsend RR, et al. Effect of fluticasone propionate/salmeterol on arterial stiffness in patients with COPD. Respir Med. 2011;105(9):1322–1330. doi:10.1016/j.rmed.2011.05.016.
- Calverley PMA, Anderson JA, Celli B, et al. Cardiovascular events in patients with COPD: TORCH study results. Thorax 2010;65(8):719–725. doi:10.1136/thx.2010.136077.
- Chen W, Thomas J, Sadatsafavi M, et al. Risk of cardiovascular comorbidity in patients with chronic obstructive pulmonary disease: a systematic review and meta-analysis. Lancet Respir Med. 2015;3(8):631–639. doi:10.1016/S2213-2600(15)00241-6.
- McAllister DA, Maclay JD, Mills NL, et al. Arterial stiffness is independently associated with emphysema severity in patients with chronic obstructive pulmonary disease. Am J Respir Crit Care Med. 2007;176(12):1208–1214. doi:10.1164/rccm.200707-1080OC.
- Sabit R, Bolton CE, Edwards PH, et al. Arterial stiffness and osteoporosis in chronic obstructive pulmonary disease. Am J Respir Crit Care Med. 2007;175(12):1259–1265. doi:10.1164/rccm.200701-067OC.
- Duvoix A, Dickens J, Haq I, et al. Blood fibrinogen as a biomarker of chronic obstructive pulmonary disease. Thorax 2013;68(7):670–676. doi:10.1136/thoraxjnl-2012-201871.
- Agusti A, Sobradillo P, Celli B. Addressing the complexity of chronic obstructive pulmonary disease: from phenotypes and biomarkers to scale-free networks, systems biology, and P4 medicine. Am J Respir Crit Care Med. 2011;183(9):1129–1137. doi:10.1164/rccm.201009-1414PP.
- Lilly SM, Jacobs D, Bluemke DA, et al. Resistive and pulsatile arterial hemodynamics and cardiovascular events: the multiethnic study of atherosclerosis. J Am Heart Assoc. 2014;3(6):e001223–e001223.
- Fagard RH, Pardaens K, Staessen JA, et al. The pulse pressure-to-stroke index ratio predicts cardiovascular events and death in uncomplicated hypertension. J Am Coll Cardiol. 2001;38(1):227–231. doi:10.1016/S0735-1097(01)01362-6.
- Barr RG, Bluemke DA, Ahmed FS, et al. Percent emphysema, airflow obstruction, and impaired left ventricular filling. N Engl J Med. 2010;362(3):217–227. doi:10.1056/NEJMoa0808836.
- Watz H, Waschki B, Meyer T, et al. Decreasing cardiac chamber sizes and associated heart dysfunction in COPD: role of hyperinflation. Chest 2010;138(1):32–38. doi:10.1378/chest.09-2810.
- Grau M, Barr RG, Lima JA, et al. Percent emphysema and right ventricular structure and function: the multi-ethnic study of atherosclerosis-lung and multi-ethnic study of atherosclerosis-right ventricle studies. Chest 2013;144(1):136–144. doi:10.1378/chest.12-1779.
- Schoos MM, Dalsgaard M, Kjaergaard J, et al. Echocardiographic predictors of exercise capacity and mortality in chronic obstructive pulmonary disease. BMC Cardiovasc Disord. 2013;13(1):84. doi:10.1186/1471-2261-13-84.
- Jörgensen K, Müller MF, Nel J, et al. Reduced intrathoracic blood volume and left and right ventricular dimensions in patients with severe emphysema: an MRI study. Chest 2007;131(4):1050–1057. doi:10.1378/chest.06-2245.
- Smith BM, Prince MR, Hoffman EA, et al. Impaired left ventricular filling in COPD and emphysema: is it the heart or the lungs? The multi-ethnic study of atherosclerosis COPD study. Chest 2013;144(4):1143–1151. doi:10.1378/chest.13-0183.
- Ceelen F, Hunter RJ, Boubertakh R, et al. Effect of atrial fibrillation ablation on myocardial function: insights from cardiac magnetic resonance feature tracking analysis. Int J Cardiovasc Imaging. 2013;29(8):1807–1817. doi:10.1007/s10554-013-0287-6.
- Müllerova H, Agusti A, Erqou S, et al. Cardiovascular comorbidity in COPD: systematic literature review. Chest 2013;144(4):1163–1178. doi:10.1378/chest.12-2847.
- Pocock SJ, Ariti CA, McMurray JJV, et al. Predicting survival in heart failure: a risk score based on 39 372 patients from 30 studies. Eur Heart J. 2013;34(19):1404–1413. doi:10.1093/eurheartj/ehs337.
- Khanji MY, van Waardhuizen CN, Bicalho VVS, et al. Lifestyle advice and interventions for cardiovascular risk reduction: a systematic review of guidelines. Int J Cardiol. 2018;263:142–151. doi:10.1016/j.ijcard.2018.02.094.