Abstract
In patients with moderate-to-severe Chronic Obstructive Pulmonary Disorder (COPD), pulmonary hyperinflation can occur at rest and increase during episodes of exacerbation. Among other mechanical constraints, changes in position and configuration of the diaphragm are also induced by increased end-expiratory lung volume. Both descent and flattening of diaphragm might damage the phrenic nerves by stretching their fibers. The study aimed to investigate the phrenic nerve conduction in COPD patients in stable conditions and during COPD exacerbation. In a group of 11 COPD patients without relevant comorbidities in stable conditions and subsequently in another group of 10 COPD patients during in-hospital COPD exacerbation and recovery, measurements of functional respiratory parameters and assessment of phrenic nerves motor conduction by bilateral electric stimulation were performed concurrently. Significant increase in phrenic nerves latency (p < 0.05), but similar amplitude of motor compound muscle action potential (cMAP) was observed in stable COPD patients vs. matched controls (p < 0.05). However, in COPD patients with resting pulmonary hyperinflation as reliably detected by substantial Inspiratory Capacity reduction (<80% pred.), the mean bilateral latency was longer vs. COPD patients without pulmonary hyperinflation (p < 0.02). During COPD exacerbation, in contrast with mean latency, the mean amplitude of phrenic nerves cMAP improved at discharge when compared with in-hospital admission (p < 0.05). In stable COPD patients the velocity of phrenic nerve conduction was impaired mostly in the presence of pulmonary hyperinflation, while during COPD exacerbation where dynamic pulmonary hyperinflation abruptly occurs, the reversible decrease of cMAP amplitude does suggest a temporary, acute axonal damage of phrenic nerves, potentially contributing to diaphragmatic dysfunction in these circumstances.
Introduction
Chronic pulmonary hyperinflation and/or acute and acute-on-chronic dynamic pulmonary hyperinflation induces changes in position and configuration of the diaphragm that may impair its muscular function as pressure and flow generator, especially when rapidly occurring [Citation1, Citation2].
Descent and flattening of the diaphragm in face to chronically or acutely increased operative lung volumes during tidal breathing because of the presence or development of pulmonary hyperinflation may be deleterious for its mechanical function, due to shortening of the fibers, increase on curvature radius, reduction of the zone of apposition and change in the mechanical arrangement of costal and crural components [Citation3]. Limited attention, however, has been paid on the concomitant diaphragmatic functional damage possibly caused by abnormal motor nervous conduction due to the phrenic nerve stretching.
In the past, only a few studies were published looking at this possibility in stable COPD patients demonstrating consistent abnormal prolongation in the latency of the phrenic nerve conduction, but with controversial results related to the motor compound muscle action potential (cMAP) of the diaphragm under these circumstances [Citation4–6]. To our knowledge, however, no studies so far have been performed in acute COPD exacerbation in non-intubated COPD patients, assessing the function of phrenic nerves.
The study aimed to investigate the phrenic nerve conduction either in stable COPD patients, seeing the effect of chronic pulmonary hyperinflation, or during acute exacerbation where dynamic hyperinflation may acutely occur or increase.
Methods
Subjects
This study was performed at the Respiratory Medicine Unit of the University of Brescia—Spedali Civili Hospital of Brescia, Italy, from October 2018 to April 2019. To be included in the study, subjects had to be aged 45–80 years and have had a previous diagnosis of chronic obstructive pulmonary disorder (COPD) supported by the presence of risk factors, clinical judgment and objective measurements of lung function according to ATS/ERS criteria (FEV1/VC% < LLN) [Citation7].
In the first part of the study, the patients had to be in stable conditions and regularly treated only with long-acting bronchodilators. Excluded from the study were those with acute exacerbation in the previous three months and chronic respiratory failure, suffering from notable chronic comorbidities (heart failure, ischemic cardiac disease, chronic atrial fibrillation, overlap with OSA, Parkinson’s disease and/or other neurological diseases, diabetes mellitus, and/or other endocrine diseases). Eight age and sex-matched, healthy subjects were also evaluated as controls.
In the second part of the study, a number of COPD patients consecutively admitted to the Hospital because of COPD exacerbation, and not requiring tracheal intubation and mechanical ventilation were investigated as soon as possible and at the moment of discharge. The exclusion criteria were the same above mentioned. None of the COPD patients enrolled in the study was in long term oxygen therapy.
Study design
In the morning, at rest and breathing room air, each patient had an arterial blood gas sample for pH, arterial gas partial pressures (PaO2 and PaCO2), and plasma bicarbonates (HCO3−) measurements in a seated position. Subsequently, after 8-hour wash-out from short-acting bronchodilators and 24-hour wash-out from long-acting bronchodilators, each patient performed spirometry (Biomedin Instruments, Padua, Italy) wearing a nose clip and breathing through a flanged mouthpiece. Slow vital capacity (SVC) and inspiratory capacity (IC) were measured twice using a bell spirometer in sitting position.
To the extent that the reduction of IC is believed a mirror of the increase of end-expiratory lung volume (see FRC) when TLC is preserved, an IC decrease of more than 20% of predicted at rest is considered as a reliable cut off to detect the presence of significant pulmonary hyperinflation and in longitudinal studies, it has been shown to be an independent predictor of respiratory and all-cause mortality in COPD [Citation8].
Lung volumes were measured with a pressure-constant plethysmograph (Biomedin Instruments, Padua, Italy). During the procedure, patients panted at a 0.7 Hertz frequency. Three acceptable tracings of mouth pressure versus box volume changes were averaged to achieve a final measurement of functional residual capacity (FRC). Total lung capacity (TLC) and residual volume (RV) were computed subsequently.
In each circumstance, the best values were collected for analysis. All tests were performed according to the ERS-ATS recommendations [Citation7]. Predicted values of lung function parameters were those proposed by the European Community for Coal and Steel [Citation9].
Subsequently, the phrenic nerves motor conduction was assessed in a semi-recumbent position by bilateral cervical electric stimulation. At the beginning of tidal inspiration under visual inspection, the phrenic nerve was supramaximal stimulated on both sides at the posterior border of the sternocleidomastoid muscle 3 cm above clavicle with a hand-held bipolar probe, being the cathode placed distally to the anode. The intensity of each stimulus was between 30 and 50 mA, and the duration of rectangular pulses was between 0.1 and 0.2 sec, conventionally at 2 Hz. The supramaximal stimulation of the phrenic nerves was checked by progressively increasing the intensity of the electric stimulus, looking at the elicited response and was ascertained by reaching a plateau on the amplitude of this response.
The compound motor action potential (cMAP) of the diaphragm was recorded by placing the active recording adhesive surface electrode 5 cm superior to the tip of the xiphoid process and the reference electrode over the ipsilateral costal margin 16 cm from the active electrode connected to an electromyogram machine (Nicolet VikingQuest EMG/NCS/EP system). Filters were set at 2 Hz to 10 kHz. Initial sensitivity was set at 0.2 mV and sweep speed at 5 ms/div. Afterward, both the latency and the amplitude of the cMAP response of either side were measured, and mean values of two acceptable measurements obtained in each side (right and left) and their sum (right plus left side) were used for analysis [Citation10].
The same protocol, except measurement of lung volumes, was followed in exacerbated COPD patients, as soon as possible after hospitalization and at the discharge time.
The study was approved by the local Ethic Committee, and all participants signed written informed consent upon enrolling.
Statistics
Unless specified otherwise, data are expressed as the mean ± standard deviation. In the first part of the study, the comparisons of the variables of interest between stable COPD and control groups were assessed by the Student’s unpaired t-test or the Mann-Whitney test if the normal distribution could not be assumed. Subsequently, analysis among different groups (COPD with normal IC, COPD with reduced IC and controls) was performed by using to one-way ANOVA and if significant by multiple orthogonal comparisons according to Bonferroni test to assess the inter-group significance. In the second part of the study, comparisons of different variables within the same group of exacerbated COPD patients, when admitted in the Hospital and at discharge, were performed by the Student’s paired t-test or the Wilcoxson signed-rank test if the normal distribution could not be assumed.
Correlations between variables were performed using Pearson’s linear regression, and the coefficients of determination were computed.
Statistical significance was accepted if p ≤ 0.05. Statistical analyses were performed using Graph Pad Prism 6.0 (Graph Pad Software, La Jolla, CA) and SPSS 23.00 (IBM, Armonk, NY).
Results
Eleven COPD patients and 8 matched healthy subjects as controls were evaluated in the first part of the study. The demographic and respiratory functional characteristics of the stable COPD patients and controls are shown in . Four COPD patients had an IC < 80% pred. and were considered as affected by pulmonary hyperinflation. They showed higher lung volumes and significantly lower FEV1 than COPD with IC ≥ 80% pred., that is without pulmonary hyperinflation.
Table 1. Anthropometric characteristics and lung function parameters in healthy control subjects and all patients with COPD and in COPD patients divided according to the presence or absence of pulmonary hyperinflation at rest.
Values of latency and amplitude of cMAP elicited by supramaximal phrenic nerves stimulation were shown in for Controls, all COPD and COPD subgroups divided according to the presence (IC < 80% pred.) or absence (IC ≥ 80% pred.) of pulmonary hyperinflation, separately for the right and left side and as the sum of the two sides. The latency was increased for COPD vs. Controls (p < 0.01), while the amplitude of cMAP was similar (n.s.). Within the COPD group, a much longer latency was observed in those with resting pulmonary hyperinflation (p < 0.02), whereas the latency values were not different when comparing COPD patients without pulmonary hyperinflation and Controls (). A significant inverse relationship was observed between IC (% pred.) and latency (r2 = 0.29; p < 0.05) in all COPD patients (). Similarly, a significant direct relationship was also found between FRC (% pred.) and latency (r2 = 0.24; p < 0.05). These findings do suggest chronic damage to the myelinic sheath of the phrenic nerves with a decreased velocity of conduction in stable COPD patients with pulmonary hyperinflation.
Figure 1. Individual values of mean latency (panel a) and mean amplitude (panel b) of cMAP in the stable COPD patients and matched control subjects enrolled in the study and subgroups of COPD patients divided according to the presence (IC < 80% pred.) or absence (IC ≥ 80% pred.) of pulmonary hyperinflation at rest. IC = Inspiratory Capacity.
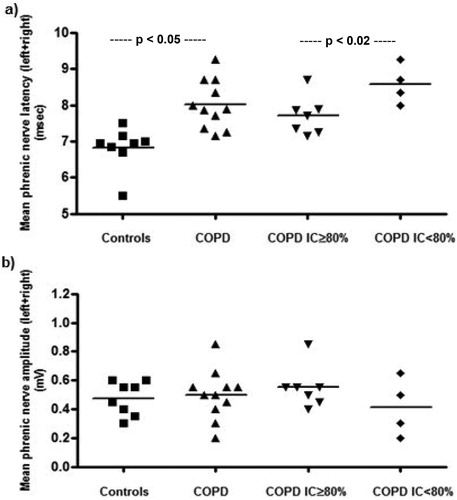
Figure 2. Correlation between values of mean latency of cMAP and resting IC (as % pred.) in stable COPD patients, suggesting an association between the prolonged velocity of conduction of phrenic nerves and degree of chronic pulmonary hyperinflation.
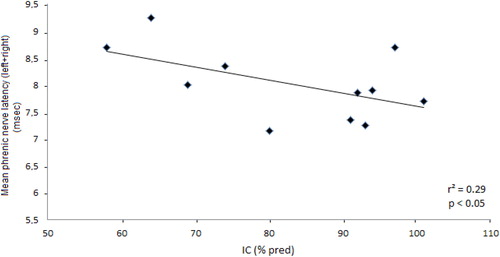
Table 2. Phrenic nerve conduction parameters in control subjects and all COPD patients, and in COPD patients divided according to the presence or absence of pulmonary hyperinflation at rest.
The anthropometric and functional characteristics of 10 COPD patients who were hospitalized because of COPD exacerbation and enrolled in the second part of the study are illustrated in in the acute phase and at discharge. The time between the initial (as soon as possible after hospitalization) and final (at discharge) assessment was, in average, 5.9 ± 3.0 days. A mild amelioration of gas exchange and greater improvement of functional parameters of airflow obstruction, with a significant increase in IC (p < 0.05) were observed, suggesting a decrease of dynamic pulmonary hyperinflation. Concurrently, a significant increase of the right, left and mean amplitude of cMAP was found at discharge (p < 0.05), suggesting a recovery of this parameter, while the mean (right plus left side) latency remained unchanged, although the latency after the phrenic nerve stimulation at the left side was decreased at discharge (p < 0.05) ( and ).
Figure 3. Individual values of amplitude and latency of cMAP during exacerbation (E) and at discharge (D) from the Hospital in a group of COPD patients hospitalized because of COPD exacerbation.
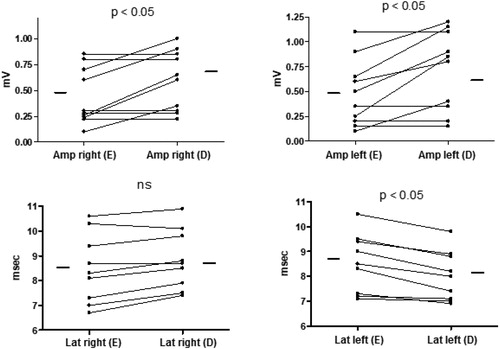
Table 3. Anthropometric characteristics, lung function parameters, and arterial blood gas values in a group of patients with COPD during exacerbation and at discharge.
Table 4. Phrenic nerve conduction parameters in a group of COPD patients during exacerbation and at discharge.
No significant relationships were found between changes in amplitude of cMAP or latency and changes in FEV1, FVC, VC, and IC (both in absolute and % predicted values).
Discussion
The findings of this study confirm in accordance with what previously shown that the latency of cMAP after electric stimulation of phrenic nerves in stable COPD patients is abnormally prolonged, as compared to matched healthy subjects [Citation4–6]. Generally, a slight prolongation of latency reflects demyelination. In this context, the presence of resting pulmonary hyperinflation instead of COPD per se seems to play a major role, and this was not clearly established in the past.
Therefore, the sufferance of the myelinic sheath on both sides of phrenic nerves could be more linked to the chronic traction on the phrenic nerve branches following the unfavorable position of the diaphragm as suggested by Younis El-tantawi et al. [Citation11], rather than representing a peculiar aspect of the motor peripheral polyneuropathy often affecting the COPD patients [Citation12]. Indeed, a gradual, protract elongation of nerves may cause histologic changes involving myelin, axon degeneration, and regeneration and endoneurial collagen deposition [Citation13]. Just once a significantly increased amplitude of cMAP in stable COPD patients has been described in the literature, ascribing it to the flattening of the diaphragm with a questionable explanation or to an increased diaphragmatic muscle mass [Citation6]. In contrast, in the current study, the amplitude of cMAP, although slightly reduced, was not significantly different in stable COPD patients from controls, in line with what reported in several other studies [Citation5, Citation11, Citation12].
The novelty of the present study was the improvement of the amplitude of cMAP during phrenic nerve stimulation after recovery from a moderate-to-severe COPD exacerbation, not requiring tracheal intubation and mechanical ventilation, suggesting its acute worsening during an exacerbation, where dynamic pulmonary hyperinflation may suddenly develop or increase. This could be likely caused by reversible axonal damage because of the mechanical stretching of phrenic nerves following the descent of both hemi-diaphragms due to a rapid increase in end-expiratory lung volume. The possible role of severe hypoxemia and/or respiratory acidosis, because of acute hypercapnia, appears less probable since this did not occur in our patients.
Although a diaphragmatic needle EMG was not performed in our patients for ethical reasons in order to rule out myopathy of the diaphragm as the origin of reduced cAMP amplitude at the onset of AECOPD, this possibility seems unlikely because of the relatively rapid improvement of this parameter.
Unexpectedly, no significant positive correlation was found between changes in IC (increase) and cAMP amplitude (increase) during the recovery from acute exacerbation. Unfortunately, we were not able to measure plethysmographic lung volumes during AECOPD to obtain the FRC variations (decrease) that are more strictly associated with Dynamic Hyperinflation reduction than those of IC because, in these circumstances, TLC may also change [Citation14].
To our knowledge, only one study assessed the phrenic nerve conduction in COPD patients with acute exacerbation who, however, were sedated, intubated, and mechanically ventilated because of the severity of respiratory failure [Citation15]. In this group of 20 COPD patients investigated during AECOPD and after weaning the latency of cMAP was always higher than controls but was not different during AECOPD and after weaning. In contrast, the amplitude of cMAP was significantly lower in COPD than controls during AECOPD and after weaning, but, similarly to the latency, its value did not change after weaning. In these conditions (the median duration of mechanical ventilation was 7 days), however, a myogenic nature of reduced cMAP amplitude due to acute inflammatory systemic effects, severe blood-gas abnormalities, electrolyte imbalances, drugs, and critical illness myopathy should be considered, but also in this study, a diaphragmatic needle EMG was not performed to exclude it.
Therefore, it appears that during AECOPD the decrease in cMAP amplitude may recover if AECOPD is moderate in severity and duration because likely sustained by temporary axonal damage due to the acute traction of the phrenic nerves, while in contrast if AECOPD is severe or very severe requiring mechanical ventilation and prolonged time to recover, the decrease in cMAP amplitude may longer persist, perhaps because partly caused by diaphragmatic myopathy.
Limits of the study
We did not perform the simultaneous evaluation of diaphragmatic function in terms of EMG, measurement of volitional or non-volitional trans-diaphragmatic pressure and ultrasound assessment of diaphragmatic displacement and contractility in our COPD patients during the examination of phrenic nerves conduction. This is a potential shortcoming of the study because a muscular dysfunction of the diaphragm cannot be ruled out as responsible for the transient cMAP amplitude reduction during an acute exacerbation. However, this seems unlikely since, during COPD exacerbation, the trans-diaphragmatic pressure throughout inspiration increases due to high neuromuscular drive to assist tidal breathing in front of greater mechanical impedance [Citation16]. In any case, the acute decrease of maximal trans-diaphragmatic pressure (Pdi, max), usually occurring under these circumstances [Citation16], seems more a consequence of a conformational change of the diaphragm (due to the rapid increase of end-expiratory lung volume) rather than an intrinsic abnormality of its fibers (due to inflammation, injury, drugs, etc.) leading to a decreased contractility, also in relation to the transient nature of the phenomenon.
Finally, the relatively small sample size is another limitation of the study, making more difficult to find a significant correlation among the different variables.
Conclusions
In stable conditions, the latency of cMAP is consistently prolonged in COPD patients suggesting damage to the myelinic sheath of phrenic nerves. Rather than a peculiar aspect of COPD-related peripheral neuropathy, this might be more related to persistent elongation secondary to prolonged mechanical traction associated with resting chronic pulmonary hyperinflation.
During moderate-to-severe COPD exacerbation, a reversible impairment of cMAP amplitude, a measure of the number the diaphragmatic muscle fibers activated by phrenic nerves, may indicate transient axonal damage. The concomitant acute dynamic pulmonary hyperinflation and the associated descent of the diaphragm may likely contribute to diaphragmatic dysfunction in AECOPD also through the injurious stretching of the phrenic nerves.
Author contributions
Study design: C. T. Data collection: S. M., P. B., B. G., L. P. Data analysis: R. M. and L. B. Interpretation of results: all authors. Initial draft: CT. Review of the manuscript for intellectual content: all authors.
Acknowledgments
The Authors acknowledge Mr. M. Guerini, University technician, for his invaluable technical support.
References
- Troyer D. A. Effect of hyperinflation on the diaphragm. Eur Respir J. 1997;10:708–713.
- Decramer M. Hyperinflation and respiratory muscle interaction. Eur Respir J. 1997;10(4):934–941. doi:10.1183/09031936.94.07101744.
- O’Donnell DE, Webb KA, Neder JA. Lung hyperinflation in COPD: applying physiology to clinical practice. COPD Res Pract. 2015;1(1):4–16. doi:10.1186/s40749-015-0008-8.
- Lu Z, Tang X, Huang X. Phrenic nerve conduction and motor evoked potential diaphragmatic: evaluation of respiratory dysfunction. Chin Med J. 1998;111(6):496–499.
- Hopkinson NS, Sharshar T, Ross ET, et al. Corticospinal control of respiratory muscles in chronic obstructive pulmonary disease. Respir Physiol Neurobiol. 2004;141(1):1–12. doi:10.1016/j.resp.2004.04.003.
- Podnar S, Harlander M. Phrenic nerve conduction studies in patients with chronic obstructive pulmonary disease. Muscle Nerve. 2013;47(4):504–509. doi:10.1002/mus.23617.
- Miller MR, Crapo R, Hankinson J, et al. General considerations for lung function testing. Eur Respir J. 2005;26(1):153–1611. doi:10.1183/09031936.05.00034505.
- Tantucci C, Donati P, Nicosia F, et al. Inspiratory capacity predicts mortality in patients with chronic obstructive pulmonary disease. Respir Med. 2008;102(4):613–619. doi:10.1016/j.rmed.2007.11.004.
- Quanjer PH, Tammeling GJ, Cotes JE, Pedersen OF, et al. Lung volumes and forced ventilatory flows. Eur Respir J. 1993; 6(Suppl 16):5–40. doi:10.1183/09041950.005s1693.
- Markand ON, Kincaid JC, Pourmand RA, et al. Electrophysiologic evaluation of diaphragm by transcutaneous phrenic nerve stimulation. Neurology. 1984;34(5):604–614. doi:10.1212/wnl.34.5.604.
- El-Tantawi GAY, Imam MH, Morsi TS. Phrenic nerve conduction abnormalities correlate with diaphragmatic descent in chronic obstructive pulmonary disease. COPD J Chronic Obstruct Pulm Dis. 2015;12(5):516–524. doi:10.3109/15412555.2014.993465.
- El-Rifai AW, El-Shazil SM. Peripheral neuropathy in stable chronic obstructive pulmonary disease: is the phrenic nerve more affected compared with other peripheral nerves? Al-Azhar Assiut Med J. 2017;15(1):52–58. doi:10.4103/AZMJ.AZMJ_5_17.
- Topp KS, Boyd BS. Structure and biomechanics of peripheral nerves: nerve responses to physical stresses and implications for physical therapist practice. Phys Ther. 2006;86(1):92–109. doi:10.1093/ptj/86.1.92.
- Parker CM, Voduc N, Aaron SD, et al. Physiological changes during symptom recovery from moderate exacerbations of COPD. Eur Respir J. 2005;26(3):420–428. doi:10.1183/09031936.05.00136304.
- Wang Y, Liu N, Zhang Z. Respiratory electrophysiologic studies in chronic obstructive pulmonary disease. Medicine. 2019;98(1):1–7.
- Ceriana P, Vitacca M, Carlucci A, et al. Changes of respiratory mechanics in COPD patients from stable state to acute exacerbations with respiratory failure. COPD J Chronic Obstruct Pulm Dis. 2017;14(2):150–155. doi:10.1080/15412555.2016.1254173.