Abstract
Although many studies have characterized polarity of macrophages in chronic obstructive pulmonary disease (COPD), limited information is available regarding cellular phenotypes of circulating monocytes in this condition. This study aimed to determine the influence of cigarette smoking and COPD on the cellular phenotype of circulating monocytes. Thirty-two patients with COPD and 36 healthy volunteers (n = 17 and 19 in nonsmokers and smokers with normal lung functions, respectively) were enrolled in this study. The expression of two cell surface markers, pro-inflammatory-related S100A9 and anti-inflammatory-related CD163, on classical monocytes was analyzed by flow cytometry. The percentage of CD14strongCD16− classical monocytes in circulating monocytes showed no difference among the three groups. The percentage of S100A9+, S100A9+CD163−, and S100A9+CD163+ cells in classical monocytes was significantly increased in COPD patients relative to nonsmoker controls. In contrast, the levels of S100A9−CD163+ cells were significantly decreased in smokers with normal lung functions and in COPD patients relative to that in nonsmokers. Multivariate analyses revealed an independent association between S100A9+ cell rates and COPD (exponent 1.0336, 95% confidence interval [CI] 1.0063–1.0617, p value < 0.05). In Receiver operating characteristic (ROC) analyses, the ratio of S100A9+CD163−/S100A9−CD163+ cells yielded a receiver operating characteristic-area under the curve of 0.719 (95% CI = 0.567–0.871) for discrimination between smokers with normal lung functions and COPD patients. In conclusion, our results demonstrated increased pro-inflammatory phenotypes in circulating classical monocytes in COPD, providing novel insights to elucidate their roles in the pathogenesis of COPD.
Summary at a glance
In flow cytometric analyses simultaneously using inflammatory-related S100A9 and anti-inflammatory-related CD163 markers, circulating CD14strongCD16− classical monocytes obtained from patients with COPD showed increased inflammatory phenotypes, as compared to healthy volunteers and smokers with normal lung functions.
Introduction
Chronic obstructive pulmonary disease (COPD) is characterized by chronic inflammation in the airway and irreversible airflow blockage, and is mainly caused by cigarette smoking [Citation1–3]. Dyspnea is a major symptom of patients with COPD apart from the presence of general symptoms such as muscle weakness, fatigue, and depression [Citation4–6].COPD is associated with systemic inflammation indicated by an increase in C-reactive protein (CRP) and various pro-inflammatory cytokines [Citation3,Citation7–9]. Currently, COPD is the third leading cause of deaths worldwide [Citation10,Citation11]. Although combination therapy consisting of a long-acting muscarinic antagonist and long-acting beta2 agonist has been used in COPD patients, prognosis of this disease remains poor [Citation12–15]. Therefore, development of biomarkers to detect the early onset of COPD is expected to enable earlier interventions for current smokers with normal lung functions.
Circulating monocytes mediate essential regulatory and effector functions in innate and adaptive immunity, and are divided into three subsets [Citation16,Citation17]. CD14strongCD16− circulating classical monocytes are the predominate subset, accounting for over 90% of all monocytes [Citation17]. The three subsets selectively migrate into inflamed tissues and differentiate into tissue macrophages [Citation18]. Macrophages also constitute a heterogeneous cell population of the innate immune system and can adapt to the local environment, thereby enabling functional diversity. The macrophages are classified as pro-inflammatory M1- and anti-inflammatory M2-type subsets [Citation19–26]. Correspondingly, typical sources of M1 and M2 macrophages were reported to be circulating classical monocytes and CD14diminishCD16+ alternative monocytes, respectively, although cell polarization of macrophages could be mutually transferred, depending on the tissue microenviornment [Citation27].
Limited information is available regarding cellular phenotypes of circulating monocytes under physiological and pathological conditions [Citation28–31]. In the present study, we explored whether cigarette smoking and COPD influenced circulating monocyte cellular phenotypes by flow cytometric analysis using primary antibodies against S100A9 and CD163. S100A9 and CD163 are markers for pro-inflammatory M1-like and anti-inflammatory M2 monocyte lineages, respectively [Citation32–35]. S100A9 belongs to the S100 family of EF-hand calcium binding proteins and presents as homodimers and/or heterodimers with S100A8 (S100A8/A9) [Citation36]. S100A9 and S100A8/A9 play roles in the development of inflammation, although the individual functions remain to be fully elucidated. S100A9 and S100A8/A9 are distinctively located in the plasma membrane and cytoplasm of monocytes, respectively, while neutrophils, but not monocytes, dominantly secrete S100A8/A9 heterodimers [Citation37–39]. In this study, we targeted cell surface expression of S100A9 and CD163 on CD14strongCD16− classical monocytes because COPD is associated with systemic inflammation and S100A9 homodimers [Citation40]. Our results showed that circulating classical monocytes in otherwise healthy smokers and patients with COPD displayed inflammation-dominant phenotypes. Taken together, our results provide new insights into the pathogenic roles of circulating monocytes in COPD.
Patients and methods
Subject selection
Subjects with COPD, current smokers with normal lung functions, and healthy nonsmokers were prospectively recruited in Iwate Medical University Hospital from April 2016 until March 2019. Healthy volunteers were defined as subjects over 40 years old. Current smokers with normal lung functions were defined as subjects with currently smoking history, no airflow obstruction, and a smoking history of ≥30 pack-years. COPD subjects were defined as being under the lower limit value of healthy subjects (5th percentile) in forced expiratory volume in 1 s (FEV1.0)/forced vital capacity (FVC). Patients with bronchiectasis were excluded. Subjects with inhaled corticosteroid treatment, upper respiratory tract infection, or COPD exacerbation ≤12 weeks of the screening visit were excluded. Patients under treatment with long-acting muscarinic antagonists and/or long-acting beta2 agonists were included in the present study. Patients with stage I/II of COPD were defined as >50% of %FEV1.0. This study was approved by Iwate Medical University (IRB, H29-64 and H28-12). All subjects provided written informed consent.
Isolation of PBMCs
Venous blood was collected into vacutainers containing ethylenediaminetetraacetic acid (EDTA). The blood was layered onto Ficoll-PaqueTM PREMIUM 1.073 (GE Healthcare, Chicago, IL, USA) and centrifuged to separate the peripheral blood monocytes (PBMCs) and plasma. PBMCs were collected, washed with 1% BSA-PBS, and stained for flow cytometric analysis.
Fluorescence-activated cell sorting (FACS)
Peripheral classical monocytes were identified via FACS. Cells obtained from peripheral blood were incubated with mixtures of fluorochrome-conjugated antibodies. Data acquisition and analysis were performed using a BD Canto II Flow Cytometer and BD FACS Diva software. Dead cells were distinguished via 7-amino-actinomycin D (7-AAD) staining. The purity of individual cells was generally greater than 95%. Cells obtained were not applied permeabilization for intrastaining. To identify monocyte lineage cells, surface markers CD14-PE (Biolegend, San Diego, California, clone HCD14) and CD16-APC/Cy7 (Biolegend, clone 3G8) were used. The characterization of pro-inflammatory and anti-inflammatory phenotype was performed using S100A9-FITC (BioRad, Hercules, California, clone MAC387) and CD163-APC (Biolegend, clone RM3/1) antibodies. S100A9 and CD163 expression levels were determined using a positive data set for each antibody, identified by using a matched concentration of mouse IgG1 kappa isotype control for fluorochrome color (Biolegend, clone MOPC21).
Pulmonary function tests
Pulmonary function tests were performed within a month prior to FACS analyses. The values of the FVC and FEV1.0 are also expressed as percentages of the predicted normal values calculated according to sex, weight, and age [Citation41].
Measurement of C-reactive protein
CRP concentration was measured using latex agglutination turbidimetric immunoassays. The detection limit of the assays was 0.1 mg/dL. Two patients with COPD did not participate in the measurement of serum CRP.
Statistical analysis
Statistical significance of differences between two groups was evaluated using the Mann–Whitney U test, Fisher's exact test or Chi-square test. Statistical significance of differences between three groups was evaluated by one-way analysis of variance followed by Tukey test. Stepwise discriminant analysis was used to calculate risk factor coefficients as a multivariate logistic regression analysis. Receiver operating characteristic (ROC) curves were plotted for each rate and differential diagnosis between smokers with normal lung functions and COPD patients, and area under the curve (AUC) was calculated. Spearman's rank correlation coefficient was used to evaluate the relationships of (1) S100A9+ and CD163+ circulating classical monocytes (2) each parameter in pulmonary function test. A p value less than 0.05 indicated statistical significance. Statistical analyses were performed using SPSS Statistics software (SPSS Inc., Chicago, IL, USA). Missing data were managed by removing data units.
Results
Patient characteristics
Patient characteristics are shown in . Specifically, there was a greater number of male in current smokers with normal lung functions relative to nonsmoker healthy volunteers, and in patients with COPD relative to nonsmokers and smokers with normal lung functions, respectively (nonsmoker vs. COPD, p < 0.0001; smoker with normal lung functions vs. COPD, p < 0.0001). Healthy volunteers were significantly younger than patients with COPD (nonsmoker vs. COPD, p < 0.001; smoker with normal lung functions vs. COPD, p < 0.01). The number of patients with exacerbation of COPD during the current one year tended to be increased in stage III/IV COPD relative to stage I/II COPD (p = 0.055 in Supplementary Material Table S1).
Table 1. Patient characteristics.
Characterization of circulating classical monocytes
Circulating monocytes were divided via the specific gravity centrifugal method, and we identified three subsets of monocytes as CD14-strong positive CD16-negative cells—classical monocytes, CD14-strong positive CD16-positive cells—intermediate monocytes, and CD14-weak CD16-positive cells—alternative monocytes by flow cytometry. The ratio of dot plot number of classical monocytes to all monocytes did not differ across the three groups ( and Supplementary Material Figure S1).
Figure 1. Representative flow cytometric analyses for peripheral blood circulating monocytes. Cells obtained from the peripheral blood monocytes (PBMCs) were used for analyses, and doublets and debris were excluded. Representative plots illustrate the gating strategy in samples obtained from COPD patients. (Top). CD14+CD16−—classical monocytes, CD14+CD16+—intermediate monocytes, and CD14diminishCD16+—alternative monocytes were identified. (Bottom left and right). Dot plots represent isotype controls and primary antibodies against S100A9 and CD163 in classical monocytes.
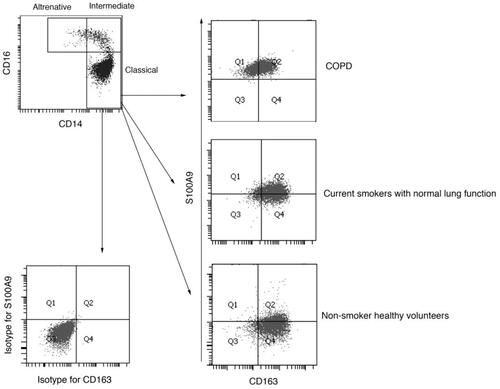
S100A9 and CD163 expression in classical monocytes
S100A9 and CD163 expression was assessed in circulating classical monocytes (). Using primary antibodies against S100A9 and CD163, FACS dot plots demonstrated that classical monocytes divided into four distinct categories, including S100A9+CD163− (Gate 1), S100A9+CD163+ (Gate 2), S100A9−CD163− (Gate 3), and S100A9−CD163+ cells (Gate 4) ().
S100A9 and CD163 expression in healthy volunteers
We explored which background factors related to healthy volunteers could influence S100A9 and CD163 expression on classical monocytes. In classical monocytes, subjects over 65 years old showed a significant increase in S100A9+CD163− cells (Gate 1) (). S100A9−CD163+ cells were significantly decreased in current smokers with normal lung functions relative to never smokers, whereas the ratio of S100A9+CD163−/S100A9−CD163+ increased. There were no differences related to sex for each parameter.
Table 2. Impact of background factors on cell polarization of circulating classical monocytes.
Comparison of S100A9+ and CD163+ cells in circulating classical monocytes in three groups
The rates of S100A9+ cells (Gate 1 + 2), S100A9+CD163− cells (Gate 1), and S100A9+CD163+ double-positive cells (Gate 2) showed a significant increase in COPD relative to nonsmoker healthy volunteers (). In addition, the rates of S100A9+ cells (Gate 1 + 2) were increased in COPD relative to smokers with normal lung functions. In contrast, the rates of S100A9−CD163+ monocytes (Gate 4) were significantly decreased in smokers with normal lung functions and COPD patients, when compared to nonsmoker volunteers, although CD163+ cells (Gate 2 + 4) did not show any differences among the three groups. The ratios of S100A9+CD163−/S100A9−CD163+ monocytes (Gate 1/Gate 4) did not show a significant difference among the three groups, although those in COPD patients were significantly increased when compared in two groups with each of nonsmokers or smokers with normal lung functions (nonsmoker vs. COPD, p < 0.001; smokers with normal lung functions vs. COPD, p < 0.01).
Figure 2. Rates of S100A9+ and CD163+ circulating classical monocytes in three groups. (A). Rates of S100A9+ monocytes (Gate 1 + 2). (B). Rates of S100A9+CD163− monocytes (Gate 1). (C). Rates of S100A9+CD163+ monocytes (Gate 2). (D). Rates of CD163+ monocytes (Gate 2 + 4). (E). Rates of S100A9−CD163+ monocytes (Gate 4). (F). Ratios of S100A9+/CD163+ monocytes (Gate 1/Gate 4). LFT: lung function tests. ∗ p < 0.05, ∗∗ p < 0.01, and ∗∗∗ p < 0.001.
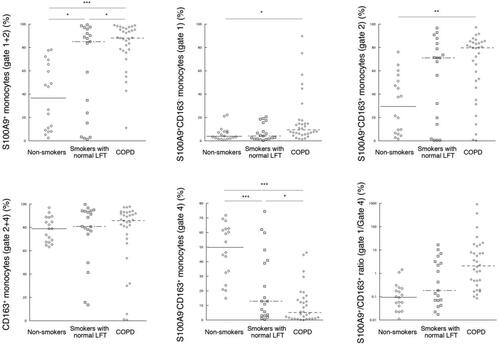
Figure 3. Receiver operating characteristic (ROC) curve analysis to assess the diagnostic validity of the ratio of S100A9+CD163−/S100A9−CD163+ cells in circulating classical monocytes in discriminating COPD patients from current smokers with normal lung functions. The area under the curve (AUC) was 0.719 (p < 0.01, 95% confidence interval [CI] = 0.567–0.871) for the diagnoses of COPD.
![Figure 3. Receiver operating characteristic (ROC) curve analysis to assess the diagnostic validity of the ratio of S100A9+CD163−/S100A9−CD163+ cells in circulating classical monocytes in discriminating COPD patients from current smokers with normal lung functions. The area under the curve (AUC) was 0.719 (p < 0.01, 95% confidence interval [CI] = 0.567–0.871) for the diagnoses of COPD.](/cms/asset/6caefc7b-fa3e-46e1-9898-e6415e0ee9d3/icop_a_1793925_f0003_b.jpg)
Comparison of S100A9+ and CD163+ cells in subjects over 65 years old
We explored whether each parameter of S100A9+ and CD163+ cells in circulating classical monocytes showed any differences among the three groups over 65 years old subjects, because these subjects showed a significant increase in S100A9+CD163− cells (Gate 1), and COPD patients were older than healthy volunteers. The rates of S100A9+ cells (Gate 1 + 2) showed a significant increase in COPD relative to nonsmoker healthy volunteers and/or smokers with normal lung functions (Supplementary Material Figure S2). In contrast, the rates of S100A9−CD163+ monocytes (Gate 4) were significantly decreased in COPD relative to nonsmoker healthy volunteers and/or smokers with normal lung functions (Supplementary Material Figure S2).
Diagnostic value of S100A9+ and CD163+ cells in circulating classical monocytes for COPD
We examined whether S100A9+ and/or CD163+ classical monocytes are independently associated with COPD in comparison with all healthy volunteers. Multivariate logistic regression analyses revealed the higher rate of S100A9+ cells as a diagnostic factor for COPD, independent of age and sex (odds ratio 1.0603, 95% confidence interval 1.0213–1.1008, p value < 0.01) (Supplementary Material Table S2). In addition, we explored whether S100A9+ and/or CD163+ classical monocytes are independently associated with COPD in comparison with smokers with normal lung functions. Multivariate logistic regression analyses revealed the higher rate of S100A9+ cells (gate 1 + 2) as an independent diagnostic factor for COPD (odds ratio 1.0366, 95% confidence interval 1.0063–1.0617, p value < 0.05) (). Furthermore, we determined the discriminative value of S100A9+ and CD163− cells in circulating classical monocytes between COPD and smokers with normal lung functions. In ROC-AUC analyses, the ratios of S100A9+CD163− cells/S100A9−CD163+ cells (Gate 1/Gate 4) showed the best diagnostic value among each parameter (ROC-AUC 0.719, p < 0.01) (, ).
Table 3. Univariate and multivariate logistic regression analyses for COPD diagnoses discriminating from smokers with normal lung functions.
Table 4. Specificity, sensitivity, and threshold values of each parameter with area under the receiver operating characteristic.
Association of S100A9+ and CD163+ cells in circulating classical monocytes with serum CRP levels, pulmonary function tests, and exacerbation of COPD
We compared each parameter of S100A9+ and/or CD163+ classical monocytes between stage I/II and III/IV COPD patients. No parameter showed any difference between the two groups (Supplementary Material Figure S3). In addition, we examined whether S100A9+ and/or CD163+ classical monocytes are associated with serum CRP levels obtained from COPD patients (Supplementary Material Table S3). Serum CRP levels were not correlated with any parameter of classical monocytes. Further, the parameters of S100A9+ and CD163+ cells in classical monocytes showed no correlation with pulmonary function tests (Supplementary Material Table S4). Moreover, we explored whether S100A9+ and/or CD163+ classical monocytes are associated with exacerbation of COPD. We divided patients with COPD into two groups, patients with (n = 14) or without (n = 18) exacerbation within the current one year. No parameter in classical monocytes showed differences between the two groups (Supplementary Material Figure S4).
Discussion
In this study, we confirmed that S100A9 and CD163 could be detected on circulating classical monocytes at varying levels, which could be divided into four groups, including S100A9+CD163−, S100A9+CD163+, S100A9−CD163−, and S100A9−CD163+ cells. In particular, S100A9+CD163+ monocytes were increased in patients with COPD. The rates of S100A9+CD163− and S100A9−CD163+ cells were characteristically sensitive to pulmonary conditions, in addition to host background factors such as age and smoking. Higher age, smoking, and COPD were all associated with inflammatory characteristics of monocytes. Furthermore, multivariate analyses revealed that the higher rates of S100A9+ cells in classical monocytes were associated with COPD, independently from age and smoking. ROC analyses showed a significant diagnostic value of S100A9+CD163−/S100A9−CD163+ ratios between COPD patients and smokers with normal lung functions. Therefore, our findings might provide new insights for the pathogenic roles of circulating monocytes in general health and pulmonary conditions, and facilitate further investigations exploring detective biomarkers for the diagnoses of COPD.
Limited information is available regarding the polarization of circulating monocytes in COPD. Cornwell and coworkers compared the expression levels of CD163 and CD206, M2-related cell surface markers, on circulating monocytes among four patient groups, including nonsmoker healthy volunteers, smoker with normal lung functions, and patients with mild and severe COPD, and they found a decreased percentage of CD163-positive monocytes in nonsmoker healthy volunteers relative to the others, despite the lower percentages of CD206-positive monocytes in smoker healthy volunteers and patients with mild and severe COPD [Citation28]. On the other hand, Bazzan and coworkers immunohistochemically stained alveolar macrophages in COPD using primary antibodies against iNOS, an M1 marker, and CD206 and found a significant increase in M1 and M2 double-positive macrophages [Citation42]. In the present study, the rates of S100A9+CD163+ double-positive monocytes were significantly increased in COPD. Although the rates of all CD163+ cells did not differ across the four groups, the rates of S100A9−CD163+ monocytes were significantly decreased in smokers with normal lung functions and patients with COPD compared to nonsmoker volunteers. Our results suggested that the estimation of pro-inflammation-single positive and anti-inflammation-single positive cells should be divided from pro-inflammation/anti-inflammation-double-positive cells when estimating cell phenotypes of monocyte lineage cells.
Several reports have demonstrated cell polarization of circulating monocytes under systemic pathological conditions and physiological background factors [Citation43,Citation44]. Using primary antibodies against CD80 (another M1 marker) and CD163, Costantini et al. reported that the rates of CD80−CD163+ M2-polarized cells in circulating classical monocytes were significantly decreased in older healthy controls [Citation45]. Reactive oxygen species (ROS) promote M1 polarization of monocytes/macrophages, and aging and smoking are related to ROS production [Citation43,Citation46,Citation47]. Prolonged cigarette exposure has been shown to cause phenotypic changes of monocytes in an experimental mouse model [Citation48]. Thus, our results support the notion that inflammatory phenotypes of circulating monocytes are induced by long-term exposure to low intensity stress without disease development.
Many studies have reported M1- and M2-polarization of alveolar macrophages in patients with COPD and/or experimental mouse models [Citation29–31]. Using immunohistochemical methods, Eapen et al. reported the elevated ratio of M1/M2 macrophages in airway walls of patients with COPD [Citation29]. Kunz and coworkers found a decreased number of CD163+ macrophages in BAL and sputum obtained from patients with COPD, and an increase number after the cessation of smoking [Citation30]. In morphometric analyses of experimental mouse models using elastase, the number of iNOS+ macrophages was increased, whereas Arg-1+ M2-macrophages were decreased [Citation31]. These reports have supported M1 polarization of macrophages located in the airway and alveolar spaces in COPD. Notably, circulating monocytes from patients with COPD were also characterized as inflammatory phenotypes, similarly to tissue macrophages in previous studies. It would be interesting to determine whether an increase in circulating classical monocytes with inflammatory phenotypes is a consequence of systemic inflammation in COPD or facilitates the progression of COPD.
An increasing body of information is available regarding biomarkers associated with COPD. In particular, CRP and surfactant protein D have been reported as promising biomarkers of COPD in clinical settings [Citation3,Citation7–9,Citation49]. Higher levels of ROC-AUC for the distinction of COPD from smokers with normal lung functions have been demonstrated, in addition to predictive values for the prognoses of COPD [Citation50]. However, it remains to be fully elucidated whether these biomarkers are useful in the detection of early onset of COPD. In the present study, the ratio of S100A9+CD163−/S100A9−CD163+ cells showed statistically significant values of ROC-AUC for discrimination between smokers with normal lung functions and COPD patients. In addition, the rates of S100A9−CD163+ cells showed significant differences between nonsmoker healthy volunteers and smokers with normal lung functions. Our results indicate the potential usefulness of S100A9 and CD163 expression in circulating classical monocytes as biomarkers for detecting harmful effects of cigarette smoking and early onset COPD.
This study has some limitations. First, the ratio of female nonsmoker healthy volunteers was significantly higher than the other group. However, no parameters showed significant differences between males and females in healthy volunteers. Second, healthy volunteers were younger than patients with COPD. However, multivariate analyses revealed the independent association of S100A9 expression in classical monocytes with COPD. Third, we used only S100A9 and CD163 in circulating monocytes as inflammatory and ant-inflammatory biomarkers, respectively, and found the inflammatory phenotypes in classical monocytes in smokers with normal lung functions and COPD patients. However, it remains to be elucidated whether other M1 and M2 surface markers can confirm the inflammatory phenotypes of classical monocytes determined by S100A9 and CD163. Fourth, the study population was relatively small. Therefore, further studies on a larger cohort of patients are needed to validate cellular phenotypes of circulating classical monocytes in COPD found in this study and to determine its detective values for early COPD.
Author contributions
Conceptualization: M.Y. Data curation: M.Y. and Y.U. Formal analysis: M.Y. and Y.U. Funding acquisition: M.Y. Investigation: M.Y. and Y.U. Supervision: K.Y. Writing—original draft: M.Y. Writing—review and editing: M.Y., Y.U., and K.Y.
Supplemental Material
Download PDF (984.3 KB)Disclosure statement
The authors do not have any conflicts of interest.
Additional information
Funding
References
- Nagai A , West WW , Paul JL , et al. The National Institutes of Health Intermittent Positive-Pressure Breathing trial: pathology studies. I. Interrelationship between morphologic lesions. Am Rev Respir Dis. 1985;132(5):937–945. DOI:10.1164/arrd.1985.132.5.937
- McDonough JE , Yuan R , Suzuki M , et al. Small-airway obstruction and emphysema in chronic obstructive pulmonary disease. N Engl J Med. 2011;365(17):1567–1575. DOI:10.1056/NEJMoa1106955
- Gan WQ , Man SF , Senthilselvan A , et al. Association between chronic obstructive pulmonary disease and systemic inflammation: a systematic review and a meta-analysis. Thorax. 2004;59(7):574–580. DOI:10.1136/thx.2003.019588
- Mannino DM , Ford ES , Redd SC . Obstructive and restrictive lung disease and markers of inflammation: data from the Third National Health and Nutrition Examination. Am J Med. 2003;114(9):758–762. DOI:10.1016/S0002-9343(03)00185-2
- Seymour JM , Spruit MA , Hopkinson NS , et al. The prevalence of quadriceps weakness in COPD and the relationship with disease severity. Eur Respir J. 2010;36(1):81–88. DOI:10.1183/09031936.00104909
- Kunik ME , Roundy K , Veazey C , et al. Surprisingly high prevalence of anxiety and depression in chronic breathing disorders. Chest. 2005;127(4):1205–1211. DOI:10.1378/chest.127.4.1205
- Dahl M , Vestbo J , Lange P , et al. C-reactive protein as a predictor of prognosis in chronic obstructive pulmonary disease. Am J Respir Crit Care Med. 2007;175(3):250–255. DOI:10.1164/rccm.200605-713OC
- Kony S , Zureik M , Driss F , et al. Association of bronchial hyperresponsiveness and lung function with C-reactive protein (CRP): a population based study. Thorax. 2004;59(10):892–896. DOI:10.1136/thx.2003.015768
- Celli BR , Locantore N , Yates J , et al. Inflammatory biomarkers improve clinical prediction of mortality in chronic obstructive pulmonary disease. Am J Respir Crit Care Med. 2012;185(10):1065–1072. DOI:10.1164/rccm.201110-1792OC
- Lozano R , Naghavi M , Foreman K , et al. A systematic analysis for the Global Burden of Disease Study 2010. Lancet. 2012;380(9859):2095–2128. DOI:10.1016/S0140-6736(12)61728-0
- Burney P , Jarvis D , Perez-Padilla R . The global burden of chronic respiratory disease in adults. Int J Tuberc Lung Dis. 2015;19(1):10–20. DOI:10.5588/ijtld.14.0446
- Cazzola M , Molimard M . The scientific rationale for combining long-acting beta2-agonists and muscarinic antagonists in COPD. Pulm Pharmacol Ther. 2010;23(4):257–267. DOI:10.1016/j.pupt.2010.03.003
- Mahler DA , Decramer M , D’Urzo A , et al. Dual bronchodilation with QVA149 reduces patient-reported dyspnoea in COPD: the BLAZE study. Eur Respir J. 2014;43(6):1599–1609. DOI:10.1183/09031936.00124013
- Mahler DA , Kerwin E , Ayers T , et al. FLIGHT1 and FLIGHT2: efficacy and safety of QVA149 (indacaterol/glycopyrrolate) versus its monocomponents and placebo in patients with chronic obstructive pulmonary disease. Am J Respir Crit Care Med. 2015;192(9):1068–1079. DOI:10.1164/rccm.201505-1048OC
- Wedzicha JA , Decramer M , Ficker JH , et al. Analysis of chronic obstructive pulmonary disease exacerbations with the dual bronchodilator QVA149 compared with glycopyrronium and tiotropium (SPARK): a randomised, double-blind, parallel-group study. Lancet Respir Med. 2013;1(3):199–209. DOI:10.1016/S2213-2600(13)70052-3
- Auffray C , Sieweke MH , Geissmann F . Blood monocytes: development, heterogeneity, and relationship with dendritic cells. Annu Rev Immunol. 2009; 27:669–692. DOI:10.1146/annurev.immunol.021908.132557
- Ziegler-Heitbrock L , Ancuta P , Crowe S , et al. Nomenclature of monocytes and dendritic cells in blood. Blood. 2010;116(16):e74–e80. DOI:10.1182/blood-2010-02-258558
- Geissmann F , Jung S , Littman DR . Blood monocytes consist of two principal subsets with distinct migratory properties. Immunity. 2003;19(1):71–82. DOI:10.1016/S1074-7613(03)00174-2
- Murray PJ , Wynn TA . Protective and pathogenic functions of macrophage subsets. Nat Rev Immunol. 2011;11(11):723–737. DOI:10.1038/nri3073
- Mantovani A , Sica A , Sozzani S , et al. The chemokine system in diverse forms of macrophage activation and polarization. Trends Immunol. 2004;25(12):677–686. DOI:10.1016/j.it.2004.09.015
- MacMicking J , Xie QW , Nathan C . Nitric oxide and macrophage function. Annu Rev Immunol. 1997; 15:323–350. DOI:10.1146/annurev.immunol.15.1.323
- Mills CD , Kincaid K , Alt JM , et al. M-1/M-2 macrophages and the Th1/Th2 paradigm. J Immunol. 2000;164(12):6166–6173. DOI:10.4049/jimmunol.164.12.6166
- Mills CD . Anatomy of a discovery: m1 and m2 macrophages. Front Immunol. 2015; 6:212. DOI:10.3389/fimmu.2015.00212
- Stein M , Keshav S , Harris N , et al. Interleukin 4 potently enhances murine macrophage mannose receptor activity: a marker of alternative immunologic macrophage activation. J Exp Med. 1992;176(1):287–292. DOI:10.1084/jem.176.1.287
- Doyle AG , Herbein G , Montaner LJ , et al. Interleukin-13 alters the activation state of murine macrophages in vitro: comparison with interleukin-4 and interferon-gamma. Eur J Immunol. 1994;24(6):1441–1445. DOI:10.1002/eji.1830240630
- Wynn TA . Fibrotic disease and the T(H)1/T(H)2 paradigm. Nat Rev Immunol. 2004;4(8):583–594. DOI:10.1038/nri1412
- Auffray C , Fogg D , Garfa M , et al. Monitoring of blood vessels and tissues by a population of monocytes with patrolling behavior. Science. 2007;317(5838):666–670. DOI:10.1126/science.1142883
- Cornwell WD , Kim V , Fan X , et al. Activation and polarization of circulating monocytes in severe chronic obstructive pulmonary disease. BMC Pulm Med. 2018;18(1):101 DOI:10.1186/s12890-018-0664-y
- Eapen MS , Hansbro PM , McAlinden K , et al. Abnormal M1/M2 macrophage phenotype profiles in the small airway wall and lumen in smokers and chronic obstructive pulmonary disease (COPD). Sci Rep. 2017;7:13392.
- Kunz LI , Lapperre TS , Snoeck-Stroband JB , et al. Smoking status and anti-inflammatory macrophages in bronchoalveolar lavage and induced sputum in COPD. Respir Res. 2011;12:34.
- Antunes MA , Abreu SC , Cruz FF , et al. Effects of different mesenchymal stromal cell sources and delivery routes in experimental emphysema. Respir Res. 2014;15:118. DOI:10.1186/s12931-014-0118-x
- He Z , Riva M , Björk P , et al. CD14 is a co-receptor for TLR4 in the S100A9-induced pro-inflammatory response in monocytes. PLoS One. 2016;11(5):e0156377. DOI:10.1371/journal.pone.0156377
- Manferdini C , Paolella F , Gabusi E , et al . Adipose stromal cells mediated switching of the pro-inflammatory profile of M1-like macrophages is facilitated by PGE2: in vitro evaluation. Osteoarthr Cartil. 2017;25(7):1161–1171. DOI:10.1016/j.joca.2017.01.011
- Kristiansen M , Graversen JH , Jacobsen C , et al. Identification of the haemoglobin scavenger receptor. Nature. 2001;409(6817):198–201. DOI:10.1038/35051594
- Verreck FA , de Boer T , Langenberg DM , et al. Phenotypic and functional profiling of human proinflammatory type-1 and anti-inflammatory type-2 macrophages in response to microbial antigens and IFN-gamma- and CD40L-mediated costimulation. J Leukoc Biol. 2006;79(2):285–293. DOI:10.1189/jlb.0105015
- Ehrchen JM , Sunderkötter C , Foell D , et al. The endogenous toll-like receptor 4 agonist S100A8/S100A9 (Calprotectin) as innate amplifier of infection autoimmunity, and cancer. J Leuko Biol. 2009;86(3):557–566. DOI:10.1189/jlb.1008647
- Bjork P , Bjork A , Vogl T , et al. Identification of human S100A9 as a novel target for treatment of autoimmune disease via binding to quinoline-3-carboxamides. PLoS Biol. 2009;7(4):e1000097. DOI:10.1371/journal.pbio.1000097
- Chakraborty P , Bjork P , Källberg E , et al. Vesicular location and transport of S100A8 and S100A9 proteins in monocytoid cells. PLoS One. 2015;10(12):e0145217. DOI:10.1371/journal.pone.0145217
- Wang S , Song R , Wang Z , et al. S100A8/A9 in inflammation. Front Immunol. 2018;9:1298. DOI:10.3389/fimmu.2018.01298
- Pouwels SD , Nawijn MC , Bathoorn E , et al. Increased serum levels of LL37, HMGB1 and S100A9 during exacerbation in COPD patients. Eur Respir J. 2015;45(5):1482–1485. DOI:10.1183/09031936.00158414
- Kubota M , Kobayashi H , Quanjer PH , et al. Reference values for spirometry, including vital capacity, in Japanese adults calculated with the LMS method and compared with previous values. Respir Investig. 2014;52(4):242–250. DOI:10.1016/j.resinv.2014.03.003
- Bazzan E , Turato G , Tinè M , et al. Dual polarization of human alveolar macrophages progressively increases with smoking and COPD severity. Respir Res. 2017; 18:40.
- Rendra E , Riabov V , Mossel DM , et al. Reactive oxygen species (ROS) in macrophage activation and function in diabetes. Immunobiology. 2019;224(2):242–253. DOI:10.1016/j.imbio.2018.11.010
- Min D , Brooks B , Wong J , et al. Monocyte CD163 is altered in association with diabetic complications: possible protective role. J Leukoc Biol. 2016;100(6):1375–1383. DOI:10.1189/jlb.3A1015-461RR
- Costantini A , Viola N , Berretta A , et al. Age-related M1/M2 phenotype changes in circulating monocytes from healthy/unhealthy individuals. Aging. 2018;10(6):1268–1280. DOI:10.18632/aging.101465
- Kim J , Kim HY , Song SY , et al. Synergistic oxygen generation and reactive oxygen species scavenging by manganese ferrite/ceria co-decorated nanoparticles for rheumatoid arthritis treatment. ACS Nano. 2019;13(3):3206–3217. DOI:10.1021/acsnano.8b08785
- Yuan Y , Chen Y , Peng T , et al. Mitochondrial ROS-induced lysosomal dysfunction impairs autophagic flux and contributes to M1 macrophage polarization in a diabetic condition. Clin Sci (Lond). 2019;133(15):1759–1777. DOI:10.1042/CS20190672
- Oliveira da Silva C , Monte-Alto-Costa A , et al. Time course of the phenotype of blood and bone marrow monocytes and macrophages in the lung after cigarette smoke exposure in vivo. Int J Mol Sci. 2017;18:1940.
- Sin DD , Leung R , Gan WQ , et al. Circulating surfactant protein D as a potential lung-specific biomarker of health outcomes in COPD: a pilot study. BMC Pulm Med. 2007;7:13. DOI:10.1186/1471-2466-7-13
- Ambade VN , Sontakke AN , Barthwal MS , et al. Diagnostic utility of biomarkers in COPD. Respir Care. 2015;60(12):1729–1742. DOI:10.4187/respcare.03753