Abstract
Forced expiratory volume in 1 s (FEV1) is a standard physiological index of chronic obstructive pulmonary disease (COPD), but reflects emphysema and vascular abnormalities less sensitively than diffusion capacity for carbon monoxide (DLCO). This study tested whether a disproportionally impaired DLCO relative to FEV1 (FEV1 z-score>-3 and DLCO z-score≤-3) is a common functional COPD phenotype associated with distinct clinical and structural features and the prognosis of two cohorts. The cross-sectional analyses of the Korea COPD Subgroup Study (KOCOSS) cohort (multicenter study in Korea) included 743 males with COPD whose DLCO was available. The cross-sectional and longitudinal analyses of the Kyoto University Cohort (single-center study in Japan) included 195 males with COPD who were prospectively followed for 10 years. A disproportionally impaired DLCO relative to FEV1 was observed in 29% and 31% of patients in the KOCOSS and Kyoto University cohorts, respectively. In the multivariable analysis, the disproportionally impaired DLCO was associated with worse symptoms, shorter 6-minute walking distance, paraseptal and centrilobular emphysema on computed tomography, and reduced arterial oxygen and carbon dioxide pressures compared to the reference (FEV1 z-score>-3 and DLCO z-score>-3). In the multivariable Cox proportional hazard model, a higher long-term mortality was observed in the disproportionally impaired DLCO group than in the reference group (hazard ratio [95% confidence interval] = 3.09 [1.52–6.29]) and similar to the DLCO z-score≤-3 and FEV1 z-score≤-3 group. The disproportionally impaired DLCO relative to FEV1 is common and associated with increased symptoms, emphysema, arterial blood gas abnormalities, and increased long-term mortality in patients with COPD.
Introduction
Chronic obstructive pulmonary disease (COPD) is a leading cause of death worldwide [Citation1]. While the diagnosis of COPD is simply based on airflow limitation on spirometry [Citation2], spirometry is insufficient to capture the heterogeneous structural alterations underlying the clinical manifestations, including airway disease, emphysema, and vascular abnormalities [Citation3].
The single-breath lung diffusion capacity for carbon monoxide (DLCO) is a noninvasive, repeatable physiological measure of the capacity of gas exchange in the alveolar space of the lungs.[Citation4,Citation5]. DLCO is closely associated with emphysema measured on histology[Citation6,Citation7] and computed tomography (CT) [Citation8], as well as vascular abnormalities on CT [Citation9–11]. Moreover, a lower DLCO is associated with a lower arterial partial pressure of oxygen (PaO2), exercise capacity, and poor prognosis in patients with COPD [Citation12–14]. Even in smokers with normal spirometry, DLCO may be decreased, and the decreased DLCO is associated with more severe symptoms and impaired exercise capacity [Citation15] and predicts the future development of COPD [Citation16]. Furthermore, Balasubramanian et al. [Citation17] recently proposed the categorization of patients with COPD based on a combination of forced expiratory volume in 1 s (FEV1) on spirometry and DLCO, and showed that an impaired DLCO (≤50% of predicted) has negative effects on symptoms, exercise capacity, and exacerbation frequency, even in patients without a substantial reduction in FEV1 (>50% of predicted). These findings suggest that functional phenotyping based on FEV1 and DLCO may improve clinical COPD management. However, the detailed structure–function relationships and even long-term prognosis in relation to this phenotyping remain to be explored.
A disproportionally impaired DLCO relative to FEV1 was hypothesized to be a common functional phenotype associated with the distinct clinical manifestations, structural changes, and prognosis of COPD. This study aimed to identify patients with COPD presenting a disproportionally impaired DLCO relative to FEV1 in two observational cohorts: the Kyoto University Cohort (single-center study in Japan) [Citation8,Citation18] and the Korea COPD Subgroup Study (KOCOSS) Cohort (multicenter study in Korea) [Citation19,Citation20]. Furthermore, this study tested whether this functional phenotype was associated with impairments in patient-reported outcomes and exercise capacity in the KOCOSS Cohort, and with a greater severity of emphysema on CT, abnormal arterial oxygen and carbon dioxide pressures, and increased long-term mortality in the Kyoto University Cohort.
Methods
Study design
This study consisted of the following datasets from two independent cohorts: the cross-sectional data from the KOCOSS Cohort and the cross-sectional and longitudinal data from the Kyoto University Cohort. The KOCOSS Cohort was obtained from a multicenter prospective observational study conducted at 48 tertiary referral hospitals in the Republic of Korea beginning in 2011 [Citation19,Citation20]. The study protocol was approved by the Institutional Review Board of Konkuk University Medical Center (Institutional Review Board No. 177 KHH1010338), and all the hospitals obtained approval from the Institutional Review Board committee. The Kyoto University Cohort is a single-center prospective observational study that has been conducted at the Kyoto University Hospital in Japan since 2006 using a single CT scanner with the fixed scanning conditions described below [Citation8,Citation18,Citation21]. The study was performed in accordance with the Declaration of Helsinki and was approved by the Ethics Committee of Kyoto University (approval Nos. E182 and R1660-1). All participants in both the Kyoto University and KOCOSS cohorts provided written informed consent. The collaborative analysis of the two cohorts was further approved by the Ethics Committee of Kyoto University (approval No. R2033).
The inclusion criteria of this study as follows: (1) age 40–85 years with a smoking history of at least 10 pack-years, (2) a physician’s diagnosis of COPD based on patient-reported respiratory symptoms and the presence of airflow limitation confirmed by a postbronchodilator FEV1/forced vital capacity (FVC) ratio below the lower limit of normal (LLN), and (3) availability of postbronchodilator spirometry and DLCO. DLCO was adjusted by the blood hemoglobin level according to a previous report [Citation22]. The LLN of FEV1/FVC and z-scores and reference values of FEV1 and FVC were obtained based on the “other” ethnic group data provided by the Global Lung Function Initiative (GLI) 2012 [Citation23]. The z-scores and reference values of DLCO were also calculated using the GLI calculation system [Citation4]. Patients with a history of lung resection surgery or other lung diseases, such as interstitial lung disease and those with alpha-1 antitrypsin deficiency, were excluded. Because the majority (> 90%) of patients enrolled in the two cohorts were male, female patients were also excluded.
In the KCOSS Cohort, patient-reported outcomes, including the mMRC dyspnea scale, COPD assessment test (CAT), and St. George's Respiratory Questionnaire (SGRQ) [Citation24, Citation25], and exercise capacity as assessed by the 6-minute walking distance (6MWD) were cross-sectionally evaluated.
In the Kyoto University Cohort, the residual volume (RV), RV to total lung capacity (TLC) ratio (RV/TLC), mMRC, emphysema and airway diseases on inspiratory CT and arterial blood gases measured in room air, including PaO2 and partial pressure of carbon dioxide (PaCO2) at baseline, were cross-sectionally evaluated. The CO transfer coefficient (Kco) that corresponds to DLCO divided by alveolar volume (VA) was also measured. Furthermore, longitudinal follow-up survival data available as of October 2019 from the Kyoto University Cohort were evaluated.
Chest CT
All subjects in the Kyoto University Cohort underwent full-inspiratory CT with a peak kilovoltage of 120, a 0.5-second exposure time, and autoexposure control using an Aquilion 64 scanner (Cannon Medical, Tokyo, Japan). Images with a 0.5-mm slice thickness were reconstructed with a high-spatial frequency algorithm (FC56). Using a SYNAPSE VINCENT volume analyzer (FUJIFILM Medical, Tokyo, Japan), the percentage of low attenuation regions less than −950 HU to the total lung regions (LAV%) was calculated to evaluate emphysema [Citation26–28]. The wall area percentage (WA%), which was defined as the percentage of the wall area relative to the sum of the wall and lumen areas, was measured for the right apical and posterior basal segmental bronchus and averaged to evaluate airway disease [Citation29–31]. Mild and substantial paraseptal emphysema (PSE), and mild and substantial (moderate to advanced) centrilobular emphysema (CLE) were visually identified based on the Fleischner Society classification system [Citation32]. The inter-rater variability of two pulmonologists (NT and HS) was excellent (kappa = 0.80 and 0.76 for the PSE and CLE evaluations). Substantial PSE and CLE were considered to indicate the presence of PSE and CLE in this study. In addition, the ratio of the pulmonary artery diameter to the aorta diameter (PA/Ao) was obtained by manually measuring the pulmonary and aorta diameters [Citation33].
Statistics
The data are reported as means ± SD, unless indicated otherwise. Statistical analyses were performed with the R program [Citation34]. A p-value less than .05 was considered statistically significant. Based on the z-scores of FEV1 and DLCO [Citation35], the patients were categorized into the following four groups: (1) FEV1 z-score > −3 and DLCO z-score > −3 (reference), (2) FEV1 z-score > −3 and DLCO z-score ≤ −3 (disproportionally impaired DLCO), (3) FEV1 z-score ≤ −3 and DLCO z-score > −3 (disproportionally impaired FEV1), and (4) FEV1 z-score ≤ −3 and DLCO z-score ≤ −3 (mixed-impaired). Tukey’s method was used to compare the variables among the four groups. Multivariable linear regression and Cox proportional hazard models were constructed and adjusted for age, height, weight, and smoking pack-years to examine the effects of the disproportionally impaired DLCO, disproportionally impaired FEV1, and mixed-impaired groups on the clinical measures and long-term outcome in comparison with the reference group. Furthermore, similar analyses were performed by defining the four groups using a cutoff of 50% for the % of predicted FEV1 and DLCO.
Results
shows patient flowcharts for the two cohorts. In the KOCOSS Cohort, 743 male patients whose hemoglobin-adjusted DLCO was available and FEV1/FVC was below the LNN were included in the cross-sectional analysis. In the Kyoto University Cohort, of the 253 stable patients with COPD enrolled from January to December 2012, 195 male patients with an FEV1/FVC below the LNN were included in the cross-sectional and longitudinal analyses. shows the basic clinical data of the two cohorts.
Figure 1. Patient flowcharts.
A. The KOCOSS Cohort was cross-sectionally analyzed. B. The Kyoto University Cohort was cross-sectionally and longitudinally analyzed.
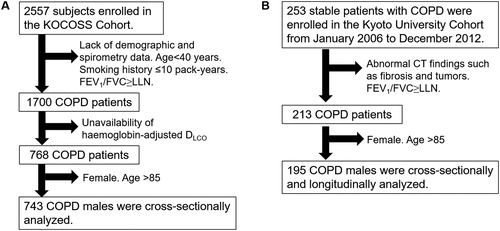
Table 1. Demographics of patients in the two cohorts.
As shown in , approximately 47%, 30%, 5%–10%, and 16% of the patients were categorized into the reference, disproportionally impaired DLCO, disproportionally impaired FEV1, and mixed-impaired groups in both cohorts, respectively (n = 351/212/62/118 in the KOCOSS Cohort, and n = 89/62/10/34 in the Kyoto University Cohort).
Figure 2. Distributions of FEV1 and DLCO in the two cohorts.
A. KOCOSS Cohort. B. Kyoto University Cohort. Patients were categorized into four groups: (1) FEV1 z-score > –3 and DLCO z-score > –3 (reference, red), (2) FEV1 z-score > –3 and DLCO z-score ≤ –3 (disproportionally impaired DLCO, green), (3) FEV1 z-score ≤ –3 and DLCO z-score > –3 (disproportionally impaired FEV1, blue), and (4) FEV1 z-score ≤ –3 and DLCO z-score ≤ –3 (mixed-impaired, purple).

The cross-sectional analysis of the KOCOSS Cohort showed that age, smoking pack-years, mMRC ≥ 2, CAT, and the SGRQ scores were higher, while the BMI and 6-minute walking distance were lower in the disproportionally impaired DLCO group, as shown in . In the multivariable analysis shown in , compared to the reference group, the disproportionally impaired DLCO was significantly associated with higher mMRC, CAT, and SGRQ scores and a lower 6MWD.
Figure 3. Associations of DLCO, FEV1, and both impairments with patient-reported outcomes and exercise capacity in a multivariable analysis of the KOCOSS cohort.
Patients (n = 743) were categorized into four groups: (1) FEV1 z-score > –3 and DLCO z-score > –3 (reference, n = 351), (2) FEV1 z-score > –3 and DLCO z-score ≤ –3 (disproportionally impaired DLCO, n = 212), (3) FEV1 z-score ≤ –3 and DLCO z-score > –3 (disproportionally impaired FEV1, n = 62), and (4) FEV1 z-score ≤ –3 and DLCO z-score ≤ –3 (mixed-impaired, n = 118). A dot with an error bar indicates the least-square mean (LS mean) with the 95% CI. * p<.05 compared to the reference group in the multivariable models. Each model was adjusted for age, pack-years of smoking, height and weight. 6MWD = six-minute walking distance. CAT = COPD assessment test. SGRQ = St. George's Respiratory Questionnaire. 6MWD, CAT, and SGRQ data were available for 641, 717, and 395 patients, respectively.
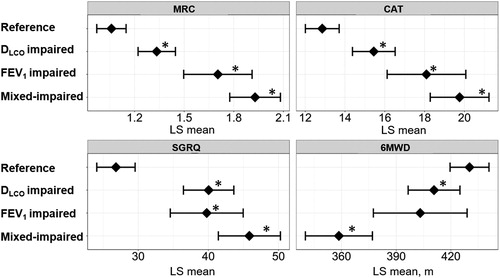
Table 2. Clinical characteristics of the four groups in the KOCOSS Cohort.
The cross-sectional analysis of the baseline data from the Kyoto University Cohort presented in showed that age, an mMRC ≥ 2, the prevalence of visual CT findings of CLE and PSE, and LAV% were higher, while the PaO2 and PaCO2 were lower in the disproportionally impaired group than in the reference group. WA% and PA/Ao on CT did not significantly differ among the groups. In the multivariable analysis shown in , the rates of both PSE and CLE were higher and PaO2 and PaCO2 were lower in the disproportionally impaired DLCO group than in the reference group. In contrast, the rates of PSE and PaCO2 in the disproportionally impaired FEV1 and mixed-impaired groups did not significantly differ from those in the reference group.
Figure 4. Associations of DLCO, FEV1, and both impairments with emphysema subtypes and arterial blood gases in a multivariable analysis of the Kyoto University Cohort.
Patients (n = 195) were categorized into four groups: (1) FEV1 z-score > –3 and DLCO z-score > –3 (reference, n = 89), (2) FEV1 z-score > –3 and DLCO z-score ≤ –3 (disproportionally impaired DLCO, n = 62), (3) FEV1 z-score ≤ –3 and DLCO z-score > –3 (disproportionally impaired FEV1, n = 10), and (4) FEV1 z-score ≤ –3 and DLCO z-score ≤ –3 (mixed-impaired, n = 34). (A) Odds ratio for the presence of paraseptal emphysema and centrilobular emphysema on CT. A dot with an error bar indicates the regression coefficient with the 95% CI. (B) Least-square mean (LS mean) with the 95% CI for the partial pressure of oxygen (PaO2) and partial pressure of carbon dioxide (PaCO2). * p<.05 compared to the reference group in the multivariable models. Each model was adjusted for age, pack-years of smoking, height and weight. PaO2 and PaCO2 data were available for 184 patients.
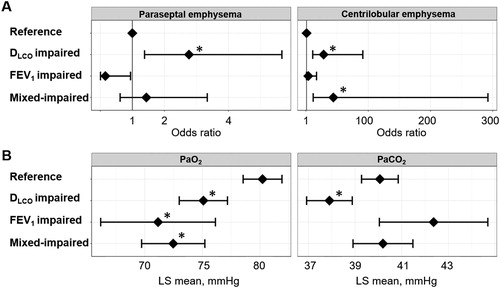
Table 3. Clinical characteristics of the four groups in the Kyoto University Cohort.
Of the 195 male patients enrolled in the Kyoto University Cohort from 2006 to 2012, 52 had died as of October 2019. As shown in , the survival rate differed among the four groups. In , the percentages of respiratory disease-related deaths were 29%, 36%, 0%, and 67% in the reference, disproportionally impaired DLCO, disproportionally impaired FEV1, and mixed-impaired groups, respectively. In the multivariable Cox proportional hazard model shown in , the disproportionally impaired DLCO and mixed-impaired groups had similar effects on all-cause mortality (HR [95% confidence interval (CI)] = 3.09 [1.52–6.29] and 3.53[1.56–8.03], respectively), whereas the effect of the disproportionally impaired FEV1 on all-cause mortality was not significant (HR [95% CI] = 0.91 [0.19–4.19]). The prognostic effect of the disproportionally impaired DLCO was detected even after adjusting for LAV% (HR [95% CI] = 2.55 [1.21–5.34]).
Figure 5. Long-term survival of patients with COPD in the Kyoto University Cohort.
(A) Kaplan–Meier curves of survival for the four groups: (1) FEV1 z-score > –3 and DLCO z-score > –3 (reference, n = 89), (2) FEV1 z-score > –3 and DLCO z-score ≤ –3 (disproportionally impaired DLCO, n = 62), (3) FEV1 z-score ≤ –3 and DLCO z-score > –3 (disproportionally impaired FEV1, n = 10), and (4) FEV1 z-score ≤ –3 and DLCO z-score ≤ –3 (mixed-impaired, n = 34). (B) Causes of death. (C) Multivariable Cox proportional hazard models. A dot with an error bar indicates the hazard ratio with 95% CI. * p<.05 compared to the reference group in the multivariable models. The model used for the upper panel included the group, age, pack-years of smoking, height, and weight as independent variables, and the model used for the lower panel included the group, age, pack-years of smoking, height, weight, and LAV% (a CT index of emphysema severity) as independent variables.
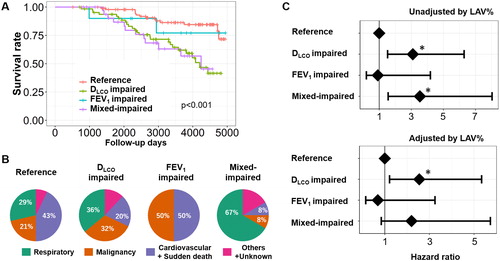
Furthermore, additional analyses were performed using the % predicted FEV1 and DLCO to categorize patients into the four groups (see the online Supplemental Figures S1 and S2). While the percentage of subjects with the disproportionally impaired DLCO, defined using the z-scores of FEV1 and DLCO, was 29% and 31% in the KOCOSS and Kyoto University cohorts, the use of the % predicted value-based definition of this subtype (% of predicted FEV1 > 50% and % of predicted DLCO ≤ 50%) changed the percentages to 18% and 21% in the KOCOSS and Kyoto University cohorts, respectively. Nonetheless, the disproportionally impaired DLCO relative to FEV1 based on the % predicted value was significantly associated with an increase in MRC, CAT, and SGRQ scores in the KOCOSS cohort, and with increased odds ratio of the presence of PSE and CLE, lower PaO2 and PaCO2, and higher mortality in the Kyoto University Cohort.
Discussion
This study shows that a disproportionally impaired DLCO relative to FEV1 was common (approximately 30%) in patients with COPD in two cohorts from different countries. This functional subgroup presented an increased severity of symptoms, impaired quality of life and exercise capacity, greater PSE and CLE, and lower PaO2 and PaCO2 than the reference group. Furthermore, the longitudinal data collected over 10 years from the Kyoto University Cohort show that this group exhibited a higher risk of long-term mortality. These findings highlight the clinical relevance of identifying a disproportionally impaired DLCO relative to FEV1 in COPD management.
DLCO reflects emphysema more strongly than FEV1 and predicts future emphysema progression and mortality [Citation12,Citation36]. Nonetheless, FEV1 on spirometry has been exclusively used in clinical practice and research fields until Balasubramanian et al. [Citation17] recently showed the utility of categorizing patients with COPD based on a combination of FEV1 and DLCO. The present data confirm and extend those previous findings by showing that the disproportionally impaired DLCO is associated with worse patient-reported outcomes, an abnormal gas exchange, higher rates of PSE and CLE, and increased mortality rates in patients with COPD. In particular, the finding that the hazard ratio of mortality did not differ between the disproportionally impaired DLCO and mixed-impaired groups is important, as it improves our ability to estimate the prognosis of patients with COPD.
The rates of both PSE and CLE were higher in the disproportionally impaired DLCO group, while the rate of CLE, but not PSE, was higher in the disproportionally impaired FEV1 and mixed-impaired groups than in the reference group. This result is consistent with a previous finding that a reduced in FEV1 is associated with CLE, but not PSE [Citation37–39]. A recent micro-CT study showed relatively milder small airway disease in PSE than CLE regions in explanted lungs from patients with COPD [Citation40]. Collectively, the disproportionally impaired DLCO might reflect more severe emphysema, particularly PSE, with relatively less damage to the airways in patients with COPD.
The disproportionally impaired DLCO group showed a higher mortality than the reference group, even after adjusting for LAV%. An impaired diffusion capacity is associated with emphysema, pulmonary vascular abnormalities [Citation6,Citation9–11], and dysfunction of pulmonary microvascular perfusion [Citation41], even in patients with mild COPD. Therefore, the disproportionally impaired DLCO might reflect pulmonary vascular dysfunction and might be associated with increased mortality independent of the emphysema severity.
The present data, showing associations between the disproportionally impaired DLCO and lower PaO2 and PaCO2, confirm a previous finding that the diffusion capacity is correlated with PaO2 [Citation12]. Additionally, the data are the first to show that a lower DLCO is associated with a lower PaCO2 in patients with a relatively preserved FEV1. This result is also consistent with a previous finding that differences in alveolar-arterial oxygen levels characterized by decreases in both PaCO2 and PaO2 precede chronic respiratory failure in patients with COPD [Citation13]. Therefore, PaCO2 may be decreased in the early stage of emphysema development and DLCO impairment, and then become increased in the late stage of the disease to eventually cause chronic hypercapnic respiratory failure.
The use of two cohorts from Japan and Korea is an advantage of this study. The two cohorts consistently showed similar frequencies in the four groups, suggesting that the disproportionally impaired DLCO relative to FEV1 is commonly identified in patients with COPD. Interestingly, the percentage of this functional phenotype was higher than the value documented in a previous report from the COPDGene study [Citation17]. The discrepancy might be due to the different severity between the studies as % of predicted FEV1 in the previous study (70%) was higher than in the present two cohorts.
FEV1/FVC decreases with age and may cause an overdiagnosis of COPD in elderly subjects [Citation35,Citation42,Citation43]. Therefore, this study defined the airflow limitation based on FEV1/FVC < LNN, but not FEV1/FVC < 0.7 (the Global Initiative for Chronic Obstructive Lung Disease [GOLD] criteria [Citation2]). Indeed, as shown in Supplemental Figure S3, of 798 males with FEV1/FVC <0.7 in the KOCOSS cohort, 55 males showed FEV1/FVC ≥ LLN, and age was higher in those with FEV1/FVC ≥ LNN than those with FEV1/FVC < LLN.
This study has some limitations. First, although cardiac dysfunction and pulmonary hypertension may affect DLCO, this study did not examine the possible effects of these abnormalities using echocardiography and heart catheterization. However, PA/Ao, which is a good marker for pulmonary hypertension [Citation44], did not differ significantly between the four groups in this study. Second, this study analyzed the data from male patients. Further studies are needed to confirm whether the findings from this study are generalizable to female subjects.
Conclusion
In this study, the data obtained from the Korean and Japanese cohorts show that a disproportionally impaired DLCO relative to FEV1 is a common functional phenotype in patients with COPD. The identification of this phenotype may improve our understanding of the various clinical manifestations of each individual and help noninvasively estimate the long-term prognosis of patients with COPD in daily practice.
Supplemental Material
Download PDF (535.4 KB)Disclosure statement
The authors report no conflicts of interest in this work.
Additional information
Funding
References
- Adeloye D, Chua S, Lee C, Global Health Epidemiology Reference Group (GHERG), et al. Global and regional estimates of COPD prevalence: systematic review and meta-analysis. J Glob Health. 2015;5(2):020415. DOI:10.7189/jogh.05-020415
- Vogelmeier CF, Criner GJ, Martinez FJ, et al. Global Strategy for the Diagnosis, Management, and Prevention of Chronic Obstructive Lung Disease 2017 Report: GOLD Executive Summary. Eur Respir J. 2017;49(3):1700214. DOI:10.1183/13993003.00214-2017
- Hogg JC. Pathophysiology of airflow limitation in chronic obstructive pulmonary disease. Lancet. 2004;364(9435):709–721. DOI:10.1016/S0140-6736(04)16900-6
- Stanojevic S, Graham BL, Cooper BG, et al. Official ERS technical standards: Global Lung Function Initiative reference values for the carbon monoxide transfer factor for Caucasians. Eur Respir J. 2017;50(3):1700010. DOI:10.1183/13993003.00010-2017
- Radovanovic D, Contoli M, Marco FD, et al. Clinical and functional characteristics of COPD Patients across GOLD Classifications: results of a multicenter observational study. COPD. 2019;16(3-4):215–226. DOI:10.1080/15412555.2019.1659760
- Gould GA, MacNee W, McLean A, et al. CT measurements of lung density in life can quantitate distal airspace enlargement-an essential defining feature of human emphysema. Am Rev Respir Dis. 1988;137(2):380–392. DOI:10.1164/ajrccm/137.2.380
- Morrison NJ, Abboud RT, Muller NL, et al. Pulmonary capillary blood volume in emphysema. Am Rev Respir Dis. 1990;141(1):53–61. DOI:10.1164/ajrccm/141.1.53
- Tanabe N, Muro S, Hirai T, et al. Impact of exacerbations on emphysema progression in chronic obstructive pulmonary disease. Am J Respir Crit Care Med. 2011;183(12):1653–1659. DOI:10.1164/rccm.201009-1535OC
- Cho YH, Lee SM, Seo JB, et al. Quantitative assessment of pulmonary vascular alterations in chronic obstructive lung disease: Associations with pulmonary function test and survival in the KOLD cohort. Eur J Radiol. 2018;108:276–282. DOI:10.1016/j.ejrad.2018.09.013
- Estepar RS, Kinney GL, Black-Shinn JL, for the COPDGene Study, et al. Computed tomographic measures of pulmonary vascular morphology in smokers and their clinical implications. Am J Respir Crit Care Med. 2013;188(2):231–239. doi: 10.1164/rccm.201301-0162OC.
- Matsuoka S, Washko GR, Yamashiro T, et al. Pulmonary hypertension and computed tomography measurement of small pulmonary vessels in severe emphysema. Am J Respir Crit Care Med. 2010;181(3):218–225. doi: 10.1164/rccm.200908-1189OC.
- Boutou AK, Shrikrishna D, Tanner RJ, et al. Lung function indices for predicting mortality in COPD. Eur Respir J. 2013;42(3):616–625. doi: 10.1183/09031936.00146012. PubMed PMID: 23349449; PubMed Central PMCID: PMCPMC3759303.
- Uemasu K, Sato S, Muro S, et al. Annual decline in arterial blood oxygen predicts development of chronic respiratory failure in COPD with mild hypoxaemia: a 6-year follow-up study. Respirology. 2019;24(3):262–269. DOI:10.1111/resp.13402
- Santus P, Radovanovic D, Balzano G, et al. Improvements in lung diffusion capacity following pulmonary rehabilitation in COPD with and without ventilation inhomogeneity. Respiration. 2016;92(5):295–307. DOI:10.1159/000448847
- Kirby M, Owrangi A, Svenningsen S, et al. On the role of abnormal DL(CO) in ex-smokers without airflow limitation: symptoms, exercise capacity and hyperpolarised helium-3 MRI. Thorax. 2013;68(8):752–759. DOI:10.1136/thoraxjnl-2012-203108
- Harvey BG, Strulovici-Barel Y, Kaner RJ, et al. Risk of COPD with obstruction in active smokers with normal spirometry and reduced diffusion capacity. Eur Respir J. 2015;46(6):1589–1597. DOI:10.1183/13993003.02377-2014 PubMed PMID: 26541521; PubMed Central PMCID: PMCPMC4752006.
- Balasubramanian A, MacIntyre NR, Henderson RJ, et al. Diffusing capacity of carbon monoxide in assessment of COPD. Chest. 2019;156(6):1111–1119. DOI:10.1016/j.chest.2019.06.035
- Tanimura K, Sato S, Fuseya Y, et al. Quantitative assessment of erector spinae muscles in patients with chronic obstructive pulmonary disease. Annals ATS. 2016;13(3):334–341. DOI:10.1513/AnnalsATS.201507-446OC
- Lee JY, Chon GR, Rhee CK, et al. Characteristics of patients with chronic obstructive pulmonary disease at the first visit to a Pulmonary Medical Center in Korea: The KOrea COpd Subgroup Study Team Cohort. J Korean Med Sci. 2016;31(4):553–560. doi: 10.3346/jkms.2016.31.4.553. PubMed PMID: 27051239; PubMed Central PMCID: PMCPMC4810338.
- Rhee CK, Kim JW, Hwang YI, et al. Discrepancies between modified Medical Research Council dyspnea score and COPD assessment test score in patients with COPD. Int J Chron Obstruct Pulmon Dis. 2015;10:1623–1631. DOI:10.2147/COPD.S87147 PubMed PMID: 26316736; PubMed Central PMCID: PMCPMC4541543.
- Tanabe N, Sato S, Tanimura K, et al. Associations of CT evaluations of antigravity muscles, emphysema and airway disease with longitudinal outcomes in patients with COPD. Thorax. 2020. 10.1136/thoraxjnl-2020-215085
- Macintyre N, Crapo RO, Viegi G, et al. Standardisation of the single-breath determination of carbon monoxide uptake in the lung. Eur Respir J. 2005;26(4):720–735. DOI:10.1183/09031936.05.00034905
- Quanjer PH, Stanojevic S, Cole TJ, ERS Global Lung Function Initiative, et al. Multi-ethnic reference values for spirometry for the 3-95-yr age range: the global lung function 2012 equations . Eur Respir J. 2012;40(6):1324–1343. DOI:10.1183/09031936.00080312
- Jones PW, Quirk FH, Baveystock CM, et al. A self-complete measure of health status for chronic airflow limitation. The St. George's Respiratory Questionnaire. Am Rev Respir Dis. 1992;145(6):1321–1327. DOI:10.1164/ajrccm/145.6.1321
- Choi JY, Yoon HK, Shin KC, et al. CAT score and SGRQ definitions of chronic bronchitis as an alternative to the classical definition. Int J Chron Obstruct Pulmon Dis. 2019;14:3043–3052. DOI:10.2147/COPD.S228307 PubMed PMID: 31920301; PubMed Central PMCID: PMCPMC6941605.
- Gevenois PA, De Vuyst P, de Maertelaer V, et al. Comparison of computed density and microscopic morphometry in pulmonary emphysema. Am J Respir Crit Care Med. 1996;154(1):187–192. DOI:10.1164/ajrccm.154.1.8680679
- Shimizu K, Tanabe N, Tho NV, et al. Per cent low attenuation volume and fractal dimension of low attenuation clusters on CT predict different long-term outcomes in COPD. Thorax. 2020;75(2):116–122. DOI:10.1136/thoraxjnl-2019-213525
- Shima H, Tanabe N, Sato S, et al. Lobar distribution of non-emphysematous gas trapping and lung hyperinflation in chronic obstructive pulmonary disease. Respir Investig. 2020;58(4):246–254. DOI:10.1016/j.resinv.2020.01.001
- Oguma T, Hirai T, Niimi A, et al. Limitations of airway dimension measurement on images obtained using multi-detector row computed tomography. PLoS One. 2013;8(10):e76381. doi: 10.1371/journal.pone.0076381. PubMed PMID: 24116105; PubMed Central PMCID: PMCPMC3792973.
- Nakano Y, Muro S, Sakai H, et al. Computed tomographic measurements of airway dimensions and emphysema in smokers. Correlation with lung function. Am J Respir Crit Care Med. 2000;162(3 Pt 1):1102–1108. DOI:10.1164/ajrccm.162.3.9907120
- Tanabe N, Oguma T, Sato S, et al. Quantitative measurement of airway dimensions using ultra-high resolution computed tomography. Respir Investig. 2018;56(6):489–496. DOI:10.1016/j.resinv.2018.07.008
- Lynch DA, Austin JH, Hogg JC, et al. CT-definable subtypes of chronic obstructive pulmonary disease: a statement of the Fleischner Society. Radiology. 2015;277(1):192–205. DOI:10.1148/radiol.2015141579 PubMed PMID: 25961632; PubMed Central PMCID: PMCPMC4613878.
- Wells JM, Washko GR, Han MK, et al. Pulmonary arterial enlargement and acute exacerbations of COPD. N Engl J Med. 2012;367(10):913–921. doi: 10.1056/NEJMoa1203830. PubMed PMID: 22938715; PubMed Central PMCID: PMCPMC3690810.
- R Core Team. R: A Language and Environment for Statistical Computing. http://www.R-project.org/.2015
- Quanjer PH, Pretto JJ, Brazzale DJ, et al. Grading the severity of airways obstruction: new wine in new bottles. Eur Respir J. 2014;43(2):505–512. DOI:10.1183/09031936.00086313
- Mohamed Hoesein FA, Zanen P, van Ginneken B, et al. Association of the transfer coefficient of the lung for carbon monoxide with emphysema progression in male smokers. Eur Respir J. 2011;38(5):1012–1018. DOI:10.1183/09031936.00050711
- Smith BM, Austin JH, Newell JD, Jr, et al. Pulmonary emphysema subtypes on computed tomography: the MESA COPD study. Am J Med. 2014;127(1):94 e7. DOI:10.1016/j.amjmed.2013.09.020PubMed PMID: 24384106; PubMed Central PMCID: PMC3882898.
- Araki T, Nishino M, Zazueta OE, et al. Paraseptal emphysema: prevalence and distribution on CT and association with interstitial lung abnormalities. Eur J Radiol. 2015;84(7):1413–1418. doi: 10.1016/j.ejrad.2015.03.010. PubMed PMID: 25868675; PubMed Central PMCID: PMCPMC4450117.
- Park J, Hobbs BD, Crapo JD, et al. Subtyping COPD by using visual and quantitative CT imaging features. Chest. 2020;157(1):47–60. doi: 10.1016/j.chest.2019.06.015. PubMed PMID: 31283919; PubMed Central PMCID: PMCPMC6965698.
- Tanabe N, Vasilescu DM, Hague CJ, et al. Pathological comparisons of paraseptal and centrilobular emphysema in chronic obstructive pulmonary disease. Am J Respir Crit Care Med. 2020;202(6):803–811. DOI:10.1164/rccm.201912-2327OC
- Hueper K, Vogel-Claussen J, Parikh MA, et al. Pulmonary microvascular blood flow in mild chronic obstructive pulmonary disease and emphysema. The MESA COPD Study. Am J Respir Crit Care Med. 2015;192(5):570–580. doi: 10.1164/rccm.201411-2120OC. PubMed PMID: 26067761; PubMed Central PMCID: PMCPMC4595687.
- Quanjer PH, Brazzale DJ, Boros PW, et al. Implications of adopting the Global Lungs Initiative 2012 all-age reference equations for spirometry. Eur Respir J. 2013;42(4):1046–1054. DOI:10.1183/09031936.00195512
- Kubota M, Kobayashi H, Quanjer PH, Clinical Pulmonary Functions Committee of the Japanese Respiratory Society, et al. Reference values for spirometry, including vital capacity, in Japanese adults calculated with the LMS method and compared with previous values. Respir Investig. 2014;52(4):242–250. DOI:10.1016/j.resinv.2014.03.003
- Devaraj A, Wells AU, Meister MG, et al. Detection of pulmonary hypertension with multidetector CT and echocardiography alone and in combination. Radiology. 2010;254(2):609–616. DOI:10.1148/radiol.09090548