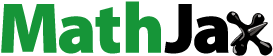
Abstract
There is growing recognition that a sizable fraction of COPD patients with forced expiratory volume in one second (FEV1)/forced vital capacity ratio below the lower limit of normal but preserved FEV1 reports out-of-proportion dyspnea relative to the severity of airflow limitation. Most physicians, however, assume that patients’ breathlessness is unlikely to reflect the negative physiological consequences of COPD vis-à-vis FEV1 normalcy. This concise review integrates the findings of recent studies which uncovered the key pathophysiological features shared by these patients: poor pulmonary gas exchange efficiency (increased “wasted” ventilation) and gas trapping. These abnormalities are associated with two well-known causes of exertional dyspnea: heightened ventilation relative to metabolic demand and critically low inspiratory reserves, respectively. From a clinical standpoint, a low diffusion capacity associated with increased residual volume (RV) and/or RV/total lung capacity ratio might uncover these disturbances, identifying the subset of patients in whom exertional dyspnea is causally related to “mild” COPD.
Introduction
There is well-established evidence that dyspnea, either induced or worsened by exertion, is the main determinant of disablement and reduced quality-adjusted-life-years in patients with chronic obstructive pulmonary disease (COPD) [Citation1, Citation2]. Owing to COPD’s insidious nature [Citation3], and the frequent coexistence of demographic (senescence) [Citation4], anthropometric (obesity) [Citation5–7] and behavioral (sedentarism) [Citation8, Citation9] features that may also cause exertional dyspnea, an etiological role for the disease in eliciting the symptom is usually confined to those with moderate-to-severe dysfunction at rest. However, there is growing recognition that a sizable fraction of patients with only “mild” disease (i.e. forced expiratory volume in one second (FEV1)/forced vital capacity (FVC) ratio below the lower limit of normal but preserved FEV1) [Citation3] reports out-of-proportion dyspnea relative to the severity of airflow limitation [Citation10, Citation11]. These patients are more likely to present with poor health-related quality of life [Citation12], being heavy consumers of health care resources - particularly if the disease is not properly treated [Citation13]. Unfortunately, most physicians assume that any shortness of breath on exertion in these patients is unlikely to reflect the negative physiological consequences of COPD vis-à-vis FEV1 normalcy. Under-recognition that mild COPD might be mechanistically related to patients’ dyspnea has relevant undesirable consequences, including delay on smoking cessation, progression of disease to poorly reversible stages, and, crucially, lack of proper treatment (including over-treatment in whom dyspnea is not etiologically linked to COPD) [Citation14, Citation15]. This concise review integrates the findings of recent studies which uncovered the key pathophysiological features (poor gas exchange efficiency and gas trapping) shared by a subset of patients with mild COPD who are particularly prone to report limiting dyspnea on exertion. We highlight the commonest abnormalities on routine pulmonary function tests, beyond FEV1, which can help the pulmonologist to causally link mild COPD to exertional dyspnea in individual patients.
Recent advances in qualification and quantification of exertional dyspnea
Dynamic exercise triggers varying levels of discomfort associated with breathing. Subjects free of cardiorespiratory disease frequently describe their respiratory sensations as “my breathing requires more effort” and/or “my breathing is heavy”; thus, a heightened awareness of breathing seems to underlie their perceptions [Citation16, Citation17]. In contrast, dyspnea in patients with cardiopulmonary disease is thought to arise from an imbalance between inspiratory neural drive to breathe, which is inherently elevated on exertion, and the capacity of the respiratory system to respond appropriately [Citation18]. In fact, exertional dyspnea ratings in COPD have been closely associated with a simultaneous increase in several physiologic ratios (inspiratory neural drive from bulbo-pontine and cortical respiratory control centers, ventilation and muscular effort, all relative to their respective maxima) which ultimately reflect demand-capacity imbalance of the respiratory system () [Citation19]. Interestingly, patients with COPD presenting with mechanical abnormalities which restrain their ability to expand tidal volume (Vt) (see Demand-Capacity Imbalance: The Role of Critical Inspiratory Constraints in Mild COPD) frequently select descriptors of insufficient inspiration, such as “my breath does not go in all the way” and/or “I feel a need for more air” [Citation20]. Thus, the report of unsatisfied inspiration may have an adjunct role in exposing the mechanical abnormalities typical of COPD [Citation18].
Figure 1. Proposed neurophysiological model of perceived respiratory discomfort (dyspnea) during exercise. Briefly, the somatosensory cortex calibrates and interprets the appropriateness of the mechanical/muscular response of the respiratory system to the prevailing level of central respiratory motor drive. When the mechanical/muscular response of the respiratory system is constrained, by disease, below the level dictated or pre-programmed by central respiratory motor drive then the intensity of “unsatisfied inspiration” increases in direct proportion to the widening disparity between drive and mechanics (i.e. neuromechanical uncoupling). Increased activation of central limbic structures as a result of neuromechanical uncoupling are also likely components of “respiratory distress”.
Symbols and Abbreviations: O2: oxygen uptake;
CO2: carbon dioxide output; Type III and IV mechano- and metabosensitive afferents in the peripheral locomotor (and respiratory) muscles and their vasculature; PSRs: pulmonary stretch receptors; C-fibers: bronchopulmonary C-fibers; J-receptors: juxtapulmonary capillary receptors; GTOs: Golgi tendon organs; PCO2: partial pressure of carbon dioxide; [H+]: hydrogen ion concentration; [La−]: lactate ion concentration; PaO2: arterial partial pressure of oxygen; SaO2: arterial oxygen saturation.
Reproduced from Respir Physiol Neurobiol. O’Donnell DE, Ora J, Webb KA, et al. Mechanisms of activity-related dyspnea in pulmonary diseases.167(1):116–32, , page 127, Copyright (2009), with permission of Elsevier [Citation18].
![Figure 1. Proposed neurophysiological model of perceived respiratory discomfort (dyspnea) during exercise. Briefly, the somatosensory cortex calibrates and interprets the appropriateness of the mechanical/muscular response of the respiratory system to the prevailing level of central respiratory motor drive. When the mechanical/muscular response of the respiratory system is constrained, by disease, below the level dictated or pre-programmed by central respiratory motor drive then the intensity of “unsatisfied inspiration” increases in direct proportion to the widening disparity between drive and mechanics (i.e. neuromechanical uncoupling). Increased activation of central limbic structures as a result of neuromechanical uncoupling are also likely components of “respiratory distress”.Symbols and Abbreviations: V̇O2: oxygen uptake; V̇CO2: carbon dioxide output; Type III and IV mechano- and metabosensitive afferents in the peripheral locomotor (and respiratory) muscles and their vasculature; PSRs: pulmonary stretch receptors; C-fibers: bronchopulmonary C-fibers; J-receptors: juxtapulmonary capillary receptors; GTOs: Golgi tendon organs; PCO2: partial pressure of carbon dioxide; [H+]: hydrogen ion concentration; [La−]: lactate ion concentration; PaO2: arterial partial pressure of oxygen; SaO2: arterial oxygen saturation.Reproduced from Respir Physiol Neurobiol. O’Donnell DE, Ora J, Webb KA, et al. Mechanisms of activity-related dyspnea in pulmonary diseases.167(1):116–32, Figure 8, page 127, Copyright (2009), with permission of Elsevier [Citation18].](/cms/asset/cf97efb6-0611-4977-99ec-355002ed2083/icop_a_1913110_f0001_c.jpg)
The intensity of respiratory discomfort on exertion is known to be modulated by age, sex, body dimensions, past experiences, and psychological traits (e.g. degree of stoicism), among others [Citation21]. It is not surprising, therefore, that the ratings of exertional dyspnea according to numerical scales (e.g. the 0-10 Borg category-ratio scale) vary markedly at a given exercise intensity across age groups in both sexes [Citation22]. In this context, a frame of reference to grade exertional dyspnea within ranges of severity (“mild”, “moderate”, “severe” and “very severe” based on percentiles distribution) at standardized work rates and ventilation in men and women aged 20-85 years has been recently published () [Citation23]. Of note, dyspnea scores typically fell in the higher ranges of severity in subjects under investigation for chronic dyspnea (some with “mild” COPD) who showed physiological abnormalities known to elicit the symptom. Such reference values provide a new benchmark to judge whether the intensity of dyspnea is (or not) above the expected range relative to healthy sedentary subjects of same sex and age [Citation23].
Figure 2. Reference values for submaximal dyspnea-work rate scores as a function of age in men and women in the “reference” sample. Dyspnea burden categorized as “mild” (5th–25th percentile), “moderate” (25th–50th percentile), “severe” (50th–75th percentile) and “very severe” (75th–95th percentile). Generalized lines model analysis indicated higher dyspnea scores in women in each age group with progressively higher values as age increased in both sexes (p < 0.01). Similar results were observed regarding dyspnea-ventilation relationship.
Reproduced from Eur Respir J. Neder JA, Berton DC, Nery LE, et al. A frame of reference for assessing the intensity of exertional dyspnea during incremental cycle ergometry. 56(4):2000191, , Page 5, Copyright (2020), with permission of European Respiratory Society Publications [Citation23].
![Figure 2. Reference values for submaximal dyspnea-work rate scores as a function of age in men and women in the “reference” sample. Dyspnea burden categorized as “mild” (5th–25th percentile), “moderate” (25th–50th percentile), “severe” (50th–75th percentile) and “very severe” (75th–95th percentile). Generalized lines model analysis indicated higher dyspnea scores in women in each age group with progressively higher values as age increased in both sexes (p < 0.01). Similar results were observed regarding dyspnea-ventilation relationship.Reproduced from Eur Respir J. Neder JA, Berton DC, Nery LE, et al. A frame of reference for assessing the intensity of exertional dyspnea during incremental cycle ergometry. 56(4):2000191, Figure 1, Page 5, Copyright (2020), with permission of European Respiratory Society Publications [Citation23].](/cms/asset/04d00b3e-d2ef-4f53-943f-eaae5042e8c8/icop_a_1913110_f0002_b.jpg)
Demand-capacity imbalance: the role of increased exertional ventilation in mild COPD
In pathological conditions, the precise matching between ventilation and metabolic demands brought by exertion [Citation24] might be disturbed by increased afferent stimuli from multiple sources [Citation25]. In patients with COPD, a major determinant of the increased afferent stimulation is the amount of ventilation “wasted” in the physiological dead space* [Citation26–28]. Although the precise mechanisms by which the respiratory controller is informed about the magnitude of the “wasted” ventilation remains controversial [Citation29–31], lower rate of CO2 unloading from the mixed venous blood to the alveoli may lead to large intra- and inter-breath fluctuations in the PCO2 of the arterial blood leaving the lungs [Citation32, Citation33] The consequence is a heightened stimulation of the peripheral and, secondarily, the central chemoreceptors () [Citation34], leading to an increased ventilation-CO2 production (E/
CO2) relationship [Citation22]. Tobacco-related injury of the lung parenchyma, small airways, and microvasculature in mild COPD leads to heterogeneous ventilation-perfusion mismatch and significant abnormalities in the efficiency of the lungs as gas exchangers [Citation35–37]. In fact, a high
E/
CO2 has been consistently observed in dyspneic patients with mild COPD () [Citation27, Citation28, Citation38–47]. The importance of microvascular dysfunction (rather than destruction) [Citation48] in increasing
E/
CO2 might have been underestimated in dyspneic patients with mild COPD: for instance, a pulmonary vasodilator (inhaled nitric oxide) concomitantly decreased
E/
CO2 and exertional dyspnea in patients with preserved FEV1 (89 ± 11% predicted) [Citation49]. Conversely, transition from upright to supine position to increase central blood volume was not associated with normalization of lung diffusing capacity for carbon monoxide (DLCO) and capillary volumes on exertion, suggesting some degree of irreversible pulmonary microvascular destruction [Citation50]. In any circumstance, if the inspiratory neural drive can be appropriately translated into VT displacement and higher ventilation (i.e. there is no mechanical limitation): a) patients are unlikely to report “unsatisfied inspiration” and b) dyspnea ratings at a given work rate are expected to increase, remaining within the lower ranges of severity when expressed relative to the heightened ventilation () (see Recent Advances in Qualification and Quantification of Exertional Dyspnea).
Figure 3. Ventilatory and derived gas exchange parameters during incremental cycle exercise in dyspneic patients with mild chronic obstructive pulmonary disease (COPD) and age-matched healthy control subjects. Higher ventilation (E) (A) and
E/CO2 output (
CO2) ratio (B) was associated with high dead space/tidal volume ratio (physiological dead space) (C), largely due to a high VD dead space (D). Increased wasted ventilation implied in a higher
E to reach a certain level of alveolar ventilation (
A) in patients (E) compared to healthy controls (F).
Values represent means ± SEM. *P < 0.05, patients with mild COPD versus healthy control subjects at rest, at standardized work rates, or at peak exercise; †P < 0.05, difference between E and
A in patients with COPD versus healthy control subjects at rest, at a standardized work rate, or at peak exercise.
Reproduced from Am J Respir Crit Care Med. Elbehairy AF, Ciavaglia CE, Webb KA, et al. Pulmonary gas exchange abnormalities in mild chronic obstructive pulmonary disease. implications for dyspnea and exercise intolerance. 15;191(12):1384–94, , page 1389, Copyright (2015), with permission of American Thoracic Society Publications [Citation28].
![Figure 3. Ventilatory and derived gas exchange parameters during incremental cycle exercise in dyspneic patients with mild chronic obstructive pulmonary disease (COPD) and age-matched healthy control subjects. Higher ventilation (V̇E) (A) and V̇E/CO2 output (V̇CO2) ratio (B) was associated with high dead space/tidal volume ratio (physiological dead space) (C), largely due to a high VD dead space (D). Increased wasted ventilation implied in a higher V̇E to reach a certain level of alveolar ventilation (V̇A) in patients (E) compared to healthy controls (F).Values represent means ± SEM. *P < 0.05, patients with mild COPD versus healthy control subjects at rest, at standardized work rates, or at peak exercise; †P < 0.05, difference between V̇E and V̇A in patients with COPD versus healthy control subjects at rest, at a standardized work rate, or at peak exercise.Reproduced from Am J Respir Crit Care Med. Elbehairy AF, Ciavaglia CE, Webb KA, et al. Pulmonary gas exchange abnormalities in mild chronic obstructive pulmonary disease. implications for dyspnea and exercise intolerance. 15;191(12):1384–94, Figure 2, page 1389, Copyright (2015), with permission of American Thoracic Society Publications [Citation28].](/cms/asset/1451b41a-821d-4b69-92bf-3dc8aec9f885/icop_a_1913110_f0003_b.jpg)
Figure 4. Representative patient with COPD who presented with poor ventilatory efficiency (high ventilation (E)/carbon dioxide output (
CO2)) (C) but did not reach critical inspiratory constraints to tidal volumes expansion (CIC), i.e. tidal volume (VT)/dynamic inspiratory capacity (ICdyn) ≤0.7 (D). Note the lack of upward inflection in dyspnea-
E (B), indicating that the high dyspnea burden (dyspnea-work rate in the “very severe” range) (A) was secondary to increased ventilatory demands rather than mechanical constraints. The dotted line represents the sensory and physiological responses found in a healthy subject of same sex and age.
Reproduced from Eur Respir J. Neder JA, Berton DC, Nery LE, et al. A frame of reference for assessing the intensity of exertional dyspnea during incremental cycle ergometry. 56(4):2000191, , Page 9, Copyright (2020), with permission of European Respiratory Society Publications [Citation23].
![Figure 4. Representative patient with COPD who presented with poor ventilatory efficiency (high ventilation (V̇E)/carbon dioxide output (V̇CO2)) (C) but did not reach critical inspiratory constraints to tidal volumes expansion (CIC), i.e. tidal volume (VT)/dynamic inspiratory capacity (ICdyn) ≤0.7 (D). Note the lack of upward inflection in dyspnea-V̇E (B), indicating that the high dyspnea burden (dyspnea-work rate in the “very severe” range) (A) was secondary to increased ventilatory demands rather than mechanical constraints. The dotted line represents the sensory and physiological responses found in a healthy subject of same sex and age.Reproduced from Eur Respir J. Neder JA, Berton DC, Nery LE, et al. A frame of reference for assessing the intensity of exertional dyspnea during incremental cycle ergometry. 56(4):2000191, Figure 4, Page 9, Copyright (2020), with permission of European Respiratory Society Publications [Citation23].](/cms/asset/be3e8957-6c65-4ae6-b018-2be13f0b8085/icop_a_1913110_f0004_c.jpg)
* A high E/
CO2 usually represents poor pulmonary gas exchange efficiency (high ventilation-to- perfusion ratio) and/or alveolar hyperventilation. “Ventilatory inefficiency”, therefore, is a widely used misnomer.
Linking gas exchange inefficiency to increased exertional ventilation in mild COPD
As mentioned, the larger the fraction of VT “wasted” in areas with excessively high ventilation-to perfusion ratio the higher the ventilatory demand at a given metabolic load, i.e. the higher the E/
CO2 (26) [Citation27, Citation28] In other words, regional reductions in pulmonary blood flow relative to alveolar ventilation will result in a high and relatively fixed physiological dead space during exercise, which requires compensatory increases in ventilation to maintain arterial blood gas and acid-base homeostasis [Citation51]. Amongst noninvasive routine pulmonary function tests, DLCO is the physiological measurement that best reflects the efficiency of the lungs as gas exchangers. Thus, a reduced alveolar surface area for gas exchange, a low blood volume in the alveolar-capillary bed, and a poor distribution of alveolar ventilation are all factors known to impair gas exchange efficiency and DLCO [Citation52]. In line with these premises, significant cross-associations between low DLCO, high
E/
CO2, and more intense dyspnea at a given exercise intensity has been consistently found in symptomatic patients with mild COPD [Citation43, Citation45, Citation53]. Of note, dyspnea intensity was not increased at iso-ventilation in those with a low DLCO, (), (45) lending support to the notion that if VT is not mechanically constrained, there is a proportional relationship between the heightened ventilatory output and its sensory consequences in mild COPD () [Citation11].
Figure 5. Reduced lung diffusing capacity for carbon monoxide (DLCO) was associated with poorer ventilatory efficiency (higher ventilation (E)/carbon dioxide output (
CO2)) ratio) (A) and higher dyspnea ratings as a function of exercise intensity (C) in a group of COPD patients with largely preserved FEV1. As the inspiratory reserve volume (IRV) did not reach critically-low values indicating significant mechanical constraints (shadowed area in (B)), dyspnea was proportional to the higher ventilation, i.e. there were no significant differences in dyspnea at a given
E in patients with low versus preserved DLCO (D).
Reproduced from J Appl Physiol. Elbehairy AF, O’Donnell CD, Abd Elhameed A, et al. Low resting diffusion capacity, dyspnea, and exercise intolerance in chronic obstructive pulmonary disease. 1;127(4):1107–16, , Pages 1112-1113, Copyright 2019, with permission of American Physiological Society Publications [Citation66].
![Figure 5. Reduced lung diffusing capacity for carbon monoxide (DLCO) was associated with poorer ventilatory efficiency (higher ventilation (V̇E)/carbon dioxide output (V̇CO2)) ratio) (A) and higher dyspnea ratings as a function of exercise intensity (C) in a group of COPD patients with largely preserved FEV1. As the inspiratory reserve volume (IRV) did not reach critically-low values indicating significant mechanical constraints (shadowed area in (B)), dyspnea was proportional to the higher ventilation, i.e. there were no significant differences in dyspnea at a given V̇E in patients with low versus preserved DLCO (D).Reproduced from J Appl Physiol. Elbehairy AF, O’Donnell CD, Abd Elhameed A, et al. Low resting diffusion capacity, dyspnea, and exercise intolerance in chronic obstructive pulmonary disease. 1;127(4):1107–16, Figures 1-3, Pages 1112-1113, Copyright 2019, with permission of American Physiological Society Publications [Citation66].](/cms/asset/2cdc6820-3daa-4e02-9fcc-4d4ce3b6b4ff/icop_a_1913110_f0005_b.jpg)
Demand-capacity imbalance: the role of critical inspiratory constraints in mild COPD
Dynamic gas trapping and mechanical constraints on VT expansion may also contribute to exertional dyspnea and reduced exercise tolerance in patients with mild COPD [Citation11]. Exercise-induced gas trapping occurs through a combination of tachypnea and expiratory flow limitation [Citation54]: end-expiratory lung volume increases and inspiratory capacity decreases, which has been reflected in a steeper dyspnea slope in dyspneic patients with mild COPD compared with healthy controls [Citation37]. For instance, external dead space loading was associated with an inability to further increase end-inspiratory lung volume, VT, and ventilation at the peak of exercise in these patients, i.e. neuromechanical dissociation (). These data indicate that the respiratory system had reached its physiological limits, being associated with an earlier onset of intolerable dyspnea in mild COPD but not in healthy controls () [Citation28]. Of note, heightened ventilation due to gas exchange inefficiency hastened dynamic gas trapping whereas a lower VT increased the physiological dead space in a vicious circle in dyspneic patients with mild COPD () [Citation41]. Thus, when the patients reach critically-low inspiratory reserves the stimuli directed to the respiratory muscles (i.e. the inspiratory neural drive) cannot be appropriately translated into VT displacement and higher ventilation [Citation55]. It follows that patients are more likely to report “unsatisfied inspiration” onwards, and dyspnea ratings as a function of both work rate and ventilation are expected to shift upwards to the higher ranges of severity () (see Recent Advances in Qualification and Quantification of Exertional Dyspnea) [Citation56]. Interestingly, elderly women reached VT constraints at a lower work rate and ventilation than age-matched men, likely reflecting their naturally reduced ventilatory reserve [Citation57].
Figure 6. External dead space (DS) was associated with early tidal volume plateau, higher respiratory frequency (Fb) relative to tidal volume, lower inspiratory reserve volume (IRV) and lower inspiratory capacity in dyspneic patients with mild COPD (left) but not in healthy controls (CTRL) (right). The shaded area represents the minimal IRV reached in all tests These data that, when challenged with increased “wasted” ventilation, patients reached their mechanical limit at a lower peak ventilation and power output compared with healthy age-matched participants. In other words, excessive ventilation and critically-low inspiratory reserves conflate to increase dyspnea at a given exercise intensity in symptomatic patients with preserved FEV1.
Values are means ± SEM. *P < 0.05 DS versus CTRL at a given work rate or at end-exercise.
Reproduced from Am J Respir Crit Care Med. Chin RC, Guenette JA, Cheng S, et al. Does the respiratory system limit exercise in mild chronic obstructive pulmonary disease? 187(12):1315–23, , page 1320, Copyright (2013), with permission of American Thoracic Society Publications [Citation39].
![Figure 6. External dead space (DS) was associated with early tidal volume plateau, higher respiratory frequency (Fb) relative to tidal volume, lower inspiratory reserve volume (IRV) and lower inspiratory capacity in dyspneic patients with mild COPD (left) but not in healthy controls (CTRL) (right). The shaded area represents the minimal IRV reached in all tests These data that, when challenged with increased “wasted” ventilation, patients reached their mechanical limit at a lower peak ventilation and power output compared with healthy age-matched participants. In other words, excessive ventilation and critically-low inspiratory reserves conflate to increase dyspnea at a given exercise intensity in symptomatic patients with preserved FEV1.Values are means ± SEM. *P < 0.05 DS versus CTRL at a given work rate or at end-exercise.Reproduced from Am J Respir Crit Care Med. Chin RC, Guenette JA, Cheng S, et al. Does the respiratory system limit exercise in mild chronic obstructive pulmonary disease? 187(12):1315–23, Figure 3, page 1320, Copyright (2013), with permission of American Thoracic Society Publications [Citation39].](/cms/asset/9249b86a-e4b7-4dc8-8f4d-a91b1e976af6/icop_a_1913110_f0006_b.jpg)
Figure 7. Metabolic and ventilatory responses to exercise in a group of dyspneic patients with mild COPD and age- and sex-matched non-trained controls. Despite preserved O2 uptake (O2)-work rate (WR) relationship (A), patients showed consistently higher ventilation (
E)/carbon dioxide output (
CO2)) ratio (B) and higher
E at a given WR (C). Tidal volume (VT) plateau (D) (marked by triangles) with a consequent increase in breathing frequency (Fb) (E) resulted from earlier attainment of critically low inspiratory reserves (F). These data illustrate the concept that excessive
E brought by gas exchange inefficiency (increased “wasted”
E in the physiological dead space) may accelerate the rate of dynamic gas trapping, an effect that is particularly deleterious when the patient started exercising at higher “static” lung volumes (note higher end-expiratory lung volume (EELV) at rest).
Symbols and Abbreviations: TLC = total lung capacity; EILV = end-inspiratory lung volume. Data are presented as mean ± SEM. *: p < 0.05.
Reproduced from Eur Respir J. Guenette JA, Chin RC, Cheng S, et al. Mechanisms of exercise intolerance in global initiative for chronic obstructive lung disease grade 1 COPD. 44(5):1177–87, , Page 1182, Copyright (2014), with permission of European Respiratory Society Publications [Citation41].
![Figure 7. Metabolic and ventilatory responses to exercise in a group of dyspneic patients with mild COPD and age- and sex-matched non-trained controls. Despite preserved O2 uptake (V̇O2)-work rate (WR) relationship (A), patients showed consistently higher ventilation (V̇E)/carbon dioxide output (V̇CO2)) ratio (B) and higher V̇E at a given WR (C). Tidal volume (VT) plateau (D) (marked by triangles) with a consequent increase in breathing frequency (Fb) (E) resulted from earlier attainment of critically low inspiratory reserves (F). These data illustrate the concept that excessive V̇E brought by gas exchange inefficiency (increased “wasted” V̇E in the physiological dead space) may accelerate the rate of dynamic gas trapping, an effect that is particularly deleterious when the patient started exercising at higher “static” lung volumes (note higher end-expiratory lung volume (EELV) at rest).Symbols and Abbreviations: TLC = total lung capacity; EILV = end-inspiratory lung volume. Data are presented as mean ± SEM. *: p < 0.05.Reproduced from Eur Respir J. Guenette JA, Chin RC, Cheng S, et al. Mechanisms of exercise intolerance in global initiative for chronic obstructive lung disease grade 1 COPD. 44(5):1177–87, Figure 1, Page 1182, Copyright (2014), with permission of European Respiratory Society Publications [Citation41].](/cms/asset/fff020f6-398e-4d31-9d3a-f0807aceb64d/icop_a_1913110_f0007_c.jpg)
Figure 8. Representative patient with COPD who presented with a sudden upward inflection in dyspnea-work rate (A) and dyspnea-ventilation (E) (B). Note the simultaneous attainment of critical inspiratory constraints (CIC) (tidal volume (VT)/dynamic inspiratory capacity (ICdyn) >0.7) (D) without an increase in
E as a function of carbon dioxide output (
CO2) i.e.
E/
CO2 below the upper limit of normal (C). Thus, dyspnea increased out or proportion to
E as the CIC precluded further increases in
E The dotted line represents the sensory and physiological responses found in a healthy subject of same sex and age.
Reproduced from Eur Respir J. Neder JA, Berton DC, Nery LE, et al. A frame of reference for assessing the intensity of exertional dyspnea during incremental cycle ergometry. 56(4):2000191, , Page 10, Copyright (2020), with permission of European Respiratory Society Publications [Citation23].
![Figure 8. Representative patient with COPD who presented with a sudden upward inflection in dyspnea-work rate (A) and dyspnea-ventilation (V̇E) (B). Note the simultaneous attainment of critical inspiratory constraints (CIC) (tidal volume (VT)/dynamic inspiratory capacity (ICdyn) >0.7) (D) without an increase in V̇ E as a function of carbon dioxide output (V̇CO2) i.e. V̇E/V̇CO2 below the upper limit of normal (C). Thus, dyspnea increased out or proportion to V̇E as the CIC precluded further increases in V̇E The dotted line represents the sensory and physiological responses found in a healthy subject of same sex and age.Reproduced from Eur Respir J. Neder JA, Berton DC, Nery LE, et al. A frame of reference for assessing the intensity of exertional dyspnea during incremental cycle ergometry. 56(4):2000191, Figure 5, Page 10, Copyright (2020), with permission of European Respiratory Society Publications [Citation23].](/cms/asset/7a1c0e19-82be-4db2-a11e-66aff314cfdd/icop_a_1913110_f0008_c.jpg)
Linking gas trapping to critical inspiratory constraints on exertion in mild COPD
The small airways in the lung periphery are the initial locus of inflammation in COPD [Citation58]. Loss of small airways may precede the development of centrilobular emphysema, and mucus hypersecretion due to chronic bronchitis can result in extensive peripheral airway obliteration [Citation59, Citation60]. From a physiological perspective, small airways disease is typically associated with increased pulmonary gas trapping due to expiratory flow limitation and early airway closure [Citation61]. These abnormalities are reflected on a high residual volume (RV) and/or RV/total lung capacity (TLC) ratio despite largely preserved FEV1 [Citation62]. Importantly, expiratory flow limitation may worsen during the hyperpnea of exercise leading to dynamic gas trapping [Citation63]. There is convincing evidence that tidal expiratory flow limitation prompts an increase in end-expiratory lung volume (EELV), an effect that might be linked to a reflex mechanism that terminates expiration prematurely, thus increasing EELV [Citation64, Citation65]. Although EELV is the key lung volume to determine the limits for VT expansion [Citation63], patients with mild COPD showing increased RV and/or RV/TLC also presented with high operating lung volumes on exertion, leading to dyspnea at low work rates () [Citation41, Citation45]. In line with these premises, increased RV/TLC ratio was associated with increased respiratory symptoms in both never- and ever-smokers with mild COPD in a large population based study [Citation43].
Conclusions
A COPD phenotype is “a single or combination of disease attribute(s) that describe differences between individuals with the disease as they relate to clinically meaningful outcomes”. Increased exertional ventilation due to gas exchange inefficiency and/or critical inspiratory constraints secondary to high operating lung volumes characterize a sub-set of patients with preserved FEV1 who reports out-of-proportion dyspnea. Those abnormalities reflect, at closer inspection, demand-capacity imbalance: whereas in the former scenario ventilation is a large fraction of maximum breathing capacity, the latter situation implies a limited room for VT expansion. The corollary is that the results of pulmonary function tests which jointly reflect the underlying mechanisms (low DLCO exposing poor gas exchange efficiency and high RV and/or RV/TLC revealing gas trapping) are poised to characterize a specific physiological phenotype associated with exertional dyspnea in patients with mild COPD.
Declaration of interest
The authors report no conflict of interest
Additional information
Funding
References
- GBD Chronic Respiratory Disease Collaborators. Prevalence and attributable health burden of chronic respiratory diseases, 1990-2017: a systematic analysis for the Global Burden of Disease Study 2017. Lancet Respir Med. 2020; 8(6):585–596.
- Verberkt CA, van den Beuken-van Everdingen MHJ, et al. Healthcare and Societal Costs in Patients with COPD and Breathlessness after Completion of a Comprehensive Rehabilitation Program. COPD. 2021;:1–24. DOI:10.1080/15412555.2020.1868420. Online ahead of print.
- GOLD Executive Committee. Global strategy for the diagnosis, management and prevention of chronic obstructive pulmonary disease: 2021 report. [Internet]. Available from: www.goldcopd.org. Accessed on March 22nd 2021.
- Allen B, Aboussouan LS. Diagnostic and therapeutic challenges of chronic obstructive pulmonary disease in the elderly. Curr Opin Pulm Med. 2021;27(2):113–119. DOI:10.1097/MCP.0000000000000750
- Enright PL, Brusasco V. Obesity and risks of misclassification and over-treatment in COPD. COPD. 2015;12(1):3–4. DOI:10.3109/15412555.2015.1003461
- O'Donnell DE, Ciavaglia CE, Neder JA. When obesity and chronic obstructive pulmonary disease collide. Physiological and clinical consequences. Ann Am Thorac Soc. 2014;11(4):635–644. DOI:10.1513/AnnalsATS.201312-438FR
- Zewari S, Vos P, van den Elshout F, et al. Obesity in COPD: Revealed and Unrevealed Issues. COPD. 2017;14(6):663–673. DOI:10.1080/15412555.2017.1383978
- Mihaltan F, Adir Y, Antczak A, et al. Importance of the relationship between symptoms and self-reported physical activity level in stable COPD based on the results from the SPACE study. Respir Res. 2019;20(1):89. DOI:10.1186/s12931-019-1053-7
- O’Donnell DE, Gebke KB. Activity restriction in mild COPD: a challenging clinical problem. Int J Chron Obstruct Pulmon Dis. 2014;9:577–588.
- Gupta N, Pinto L, Benedetti A, et al. The COPD Assessment Test: Can It Discriminate Across COPD Subpopulations? Chest. 2016;150(5):1069–1079. DOI:10.1016/j.chest.2016.06.016
- James MD, Milne KM, Phillips DB, et al. Dyspnea and Exercise Limitation in Mild COPD: The Value of CPET. Front Med (Lausanne). 2020;7:442. DOI:10.3389/fmed.2020.00442
- Lee H, Jhun BW, Cho J, et al. Different impacts of respiratory symptoms and comorbidities on COPD-specific health-related quality of life by COPD severity. COPD. 2017;Volume 12:3301–3310. DOI:10.2147/COPD.S145910
- Gershon AS, Thiruchelvam D, Chapman KR, et al. Health Services Burden of Undiagnosed and Overdiagnosed COPD. Chest. 2018;153(6):1336–1346. DOI:10.1016/j.chest.2018.01.038
- Elbehairy AF, Webb KA, Neder JA, et al. Should mild COPD be treated? Evidence for early pharmacological intervention. Drugs. 2013;73(18):1991–2001. DOI:10.1007/s40265-013-0145-9
- O'Donnell DE, Gebke KB. Examining the role of activity, exercise, and pharmacology in mild COPD. Postgrad Med. 2014;126(5):135–145. DOI:10.3810/pgm.2014.09.2808
- Mahler DA. Evaluation of Dyspnea in the Elderly. Clin Geriatr Med. 2017;33(4):503–521. DOI:10.1016/j.cger.2017.06.004
- Mahler DA, O'Donnell DE. Recent advances in dyspnea. Chest. 2015;147(1):232–241. DOI:10.1378/chest.14-0800
- O'Donnell DE, Ora J, Webb KA, et al. Mechanisms of activity-related dyspnea in pulmonary diseases. Respir Physiol Neurobiol. 2009;167(1):116–132. DOI:10.1016/j.resp.2009.01.010
- O'Donnell DE, Elbehairy AF, Faisal A, et al. Exertional dyspnoea in COPD: the clinical utility of cardiopulmonary exercise testing. Eur Respir Rev. 2016;25(141):333–347. DOI:10.1183/16000617.0054-2016
- Laveneziana P, Webb KA, Ora J, et al. Evolution of dyspnea during exercise in chronic obstructive pulmonary disease: impact of critical volume constraints. Am J Respir Crit Care Med. 2011;184(12):1367–1373. DOI:10.1164/rccm.201106-1128OC
- Mahler DA, Horowitz MB. Clinical evaluation of exertional dyspnea. Clin Chest Med. 1994;15(2):259–269.
- Killian KJ, Summers E, Jones NL, et al. Dyspnea and leg effort during incremental cycle ergometry. Am Rev Respir Dis. 1992;145(6):1339–1345. JunDOI:10.1164/ajrccm/145.6.1339
- Neder JA, Berton DC, Nery LE, et al. A Frame of Reference for Assessing the Intensity of Exertional Dyspnoea During Incremental Cycle Ergometry. Eur Respir J. 2020;56(4):2000191.
- Whipp BJ. The hyperpnea of dynamic muscular exercise. Exerc Sport Sci Rev. 1977;5:295–311. DOI:10.1249/00003677-197700050-00010
- Dempsey JA, Smith CA. Pathophysiology of human ventilatory control. Eur Respir J. 2014;44(2):495–512. AugDOI:10.1183/09031936.00048514
- Neder JA, Berton DC, Müller P de T, Canadian Respiratory Research Network, et al. Ventilatory Inefficiency and Exertional Dyspnea in Early Chronic Obstructive Pulmonary Disease. Ann Am Thorac Soc. 2017;14(Supplement_1):S22–S9. JulDOI:10.1513/AnnalsATS.201612-1033FR
- Neder JA, Arbex FF, Alencar MCN, et al. Exercise ventilatory inefficiency in mild to end-stage COPD. Eur Respir J. 2015;45(2):377–387. DOI:10.1183/09031936.00135514
- Elbehairy AF, Ciavaglia CE, Webb KA, et al. Pulmonary Gas Exchange Abnormalities in Mild Chronic Obstructive Pulmonary Disease. Implications for Dyspnea and Exercise Intolerance. Am J Respir Crit Care Med. 2015;191(12):1384–1394. DOI:10.1164/rccm.201501-0157OC
- Whipp BJ. Control of the exercise hyperpnea: the unanswered question. Adv Exp Med Biol. 2008;605:16–21. DOI:10.1007/978-0-387-73693-8_3
- Poon C-S, Tin C, Song G. Submissive hypercapnia: Why COPD patients are more prone to CO2 retention than heart failure patients. Respir Physiol Neurobiol. 2015;216:86–93. DOI:10.1016/j.resp.2015.03.001
- Ward SA. Commentary on “Mechanism of augmented exercise hyperpnea in chronic heart failure and dead space loading” by Poon and Tin. Respir Physiol Neurobiol. 2013;189(1):203–210. DOI:10.1016/j.resp.2013.07.004
- Allen CJ, Jones NL, Killian KJ. Alveolar gas exchange during exercise: a single-breath analysis. J Appl Physiol Respir Environ Exerc Physiol. 1984;57(6):1704–1709. DOI:10.1152/jappl.1984.57.6.1704
- Forster HV, Haouzi P, Dempsey JA. Control of breathing during exercise. Compr Physiol. 2012;2(1):743–777. DOI:10.1002/cphy.c100045
- Dempsey JA, Smith CA. Update on Chemoreception: Influence on Cardiorespiratory Regulation and Pathophysiology. Clin Chest Med. 2019;40(2):269–283. JunDOI:10.1016/j.ccm.2019.02.001
- Barbera JA, Ramirez J, Roca J, et al. Lung structure and gas exchange in mild chronic obstructive pulmonary disease. Am Rev Respir Dis. 1990;141(4 Pt 1):895–901. DOI:10.1164/ajrccm/141.4_Pt_1.895
- Barbera JA, Roca J, Ramirez J, et al. Gas exchange during exercise in mild chronic obstructive pulmonary disease. Correlation with lung structure. Am Rev Respir Dis. 1991;144(3 Pt 1):520–525. DOI:10.1164/ajrccm/144.3_Pt_1.520
- O'Donnell DE, Neder JA, Elbehairy AF. Physiological impairment in mild COPD. Respirology. 2016;21(2):211–223. DOI:10.1111/resp.12619
- Ofir D, Laveneziana P, Webb KA, et al. Mechanisms of dyspnea during cycle exercise in symptomatic patients with GOLD stage I chronic obstructive pulmonary disease. Am J Respir Crit Care Med. 2008;177(6):622–629. DOI:10.1164/rccm.200707-1064OC
- Chin RC, Guenette JA, Cheng S, et al. Does the respiratory system limit exercise in mild chronic obstructive pulmonary disease? Am J Respir Crit Care Med. 2013;187(12):1315–1323. DOI:10.1164/rccm.201211-1970OC
- O’Donnell DE, Maltais F, Porszasz J, et al. The continuum of physiological impairment during treadmill walking in patients with mild-to-moderate COPD: patient characterization phase of a randomized clinical trial. PLoS One. 2014;9(5):e96574. DOI:10.1371/journal.pone.0096574
- Guenette JA, Chin RC, Cheng S, et al. Mechanisms of exercise intolerance in global initiative for chronic obstructive lung disease grade 1 COPD. Eur Respir J. 2014;44(5):1177–1187. DOI:10.1183/09031936.00034714
- Neder JA, O’Donnell CDJ, Cory J, et al. Ventilation Distribution Heterogeneity at Rest as a Marker of Exercise Impairment in Mild-to-Advanced COPD. COPD. 2015;12(3):249–256.
- Tan WC, Sin DD, Bourbeau J, et al. Characteristics of COPD in never-smokers and ever-smokers in the general population: results from the CanCOLD study. Thorax. 2015;70(9):822–829. DOI:10.1136/thoraxjnl-2015-206938
- Hirai DM, Jones JH, Zelt JT, et al. Oral N-acetylcysteine and exercise tolerance in mild chronic obstructive pulmonary disease. J Appl Physiol (1985). 2017;122(5):1351–1361. May 1DOI:10.1152/japplphysiol.00990.2016
- Elbehairy AF, Faisal A, Guenette JA, et al. Resting Physiological Correlates of Reduced Exercise Capacity in Smokers with Mild Airway Obstruction. COPD. 2017;14(3):267–275. DOI:10.1080/15412555.2017.1281901
- Neder JA, Berton DC, Marillier M, et al. Inspiratory Constraints and Ventilatory Inefficiency Are Superior to Breathing Reserve in the Assessment of Exertional Dyspnea in COPD. COPD. 2019;16(2):174–181. DOI:10.1080/15412555.2019.1631776
- Phillips DB, Domnik NJ, Elbehairy AF, et al. Elevated exercise ventilation in mild COPD is not linked to enhanced central chemosensitivity. Respir Physiol Neurobiol. 2021;284:103571. DOI:10.1016/j.resp.2020.103571
- Hueper K, Vogel-Claussen J, Parikh MA, et al. Pulmonary Microvascular Blood Flow in Mild Chronic Obstructive Pulmonary Disease and Emphysema. The MESA COPD Study. Am J Respir Crit Care Med. 2015;192(5):570–580. DOI:10.1164/rccm.201411-2120OC
- Phillips DB, Brotto AR, Ross BA, et al. Inhaled nitric oxide improves ventilatory efficiency and exercise capacity in patients with mild COPD: A randomized-control cross-over trial. J Physiol. 2021;599(5):1665–1683. DOI:10.1113/JP280913
- Ross BA, Brotto AR, Fuhr DP, et al. The supine position improves but does not normalize the blunted pulmonary capillary blood volume response to exercise in mild COPD. J Appl Physiol (1985). 2020;128(4):925–933. DOI:10.1152/japplphysiol.00890.2019
- Neder JA, Di Paolo M, O’Donnell DE, et al. On the complexities of measuring exercise “ventilatory efficiency” in obstructive lung diseases. Pediatr Pulmonol. 2020;55(2):280–282. DOI:10.1002/ppul.24556
- Neder JA, Berton DC, Muller PT, et al. Incorporating Lung Diffusing Capacity for Carbon Monoxide in Clinical Decision Making in Chest Medicine. Clin Chest Med. 2019;40(2):285–305. DOI:10.1016/j.ccm.2019.02.005
- Jones JH, Zelt JT, Hirai DM, et al. Emphysema on Thoracic CT and Exercise Ventilatory Inefficiency in Mild-to-Moderate COPD. 2017;14(2):210–218.
- Dominelli PB, Foster GE, Guenette JA, et al. Quantifying the shape of the maximal expiratory flow-volume curve in mild COPD. Respir Physiol Neurobiol. 2015;219:30–35. DecDOI:10.1016/j.resp.2015.08.002
- Faisal A, Alghamdi BJ, Ciavaglia CE, et al. Common Mechanisms of Dyspnea in Chronic Interstitial and Obstructive Lung Disorders. Am J Respir Crit Care Med. 2016;193(3):299–309. DOI:10.1164/rccm.201504-0841OC
- O'Donnell DE, James MD, Milne KM, et al. The Pathophysiology of Dyspnea and Exercise Intolerance in Chronic Obstructive Pulmonary Disease. Clin Chest Med. 2019;40(2):343–366. JunDOI:10.1016/j.ccm.2019.02.007
- Guenette JA, Jensen D, Webb KA, et al. Sex differences in exertional dyspnea in patients with mild COPD: physiological mechanisms. Respir Physiol Neurobiol. 2011;177(3):218–227. DOI:10.1016/j.resp.2011.04.011
- Hogg JC. Pathophysiology of airflow limitation in chronic obstructive pulmonary disease. Lancet. 2004;364(9435):709–721. DOI:10.1016/S0140-6736(04)16900-6
- Hogg JC, Paré PD, Hackett T-L. The Contribution of Small Airway Obstruction to the Pathogenesis of Chronic Obstructive Pulmonary Disease. Physiol Rev. 2017;97(2):529–552. DOI:10.1152/physrev.00025.2015
- Kirby M, Tanabe N, Tan WC, et al. Total Airway Count on Computed Tomography and the Risk of Chronic Obstructive Pulmonary Disease Progression. Findings from a Population-based Study. Am J Respir Crit Care Med. 2018;197(1):56–65. DOI:10.1164/rccm.201704-0692OC
- Macklem PT. The physiology of small airways. Am J Respir Crit Care Med. 1998;157(5 Pt 2):S181–S183. DOI:10.1164/ajrccm.157.5.rsaa-2
- Macklem PT. The pathophysiology of chronic bronchitis and emphysema. Med Clin North Am. 1973;57(3):669–670. DOI:10.1016/s0025-7125(16)32266-0
- Langer D, Ciavaglia CE, Neder JA, et al. Lung hyperinflation in chronic obstructive pulmonary disease: mechanisms, clinical implications and treatment. Expert Rev Respir Med. 2014;8(6):731–749.
- Pellegrino R, Brusasco V, Rodarte JR, et al. Expiratory flow limitation and regulation of end-expiratory lung volume during exercise. J Appl Physiol. 1993;74(5):2552–2558. DOI:10.1152/jappl.1993.74.5.2552
- Pellegrino R, Villosio C, Milanese U, et al. Breathing during exercise in subjects with mild-to-moderate airflow obstruction: effects of physical training. J Appl Physiol (1985). 1999;87(5):1697–1704. DOI:10.1152/jappl.1999.87.5.1697
- Elbehairy AF, O’Donnell CD, Abd Elhameed A, et al. Low resting diffusion capacity, dyspnea, and exercise intolerance in chronic obstructive pulmonary disease. J Appl Physiol. 2019;127(4):1107–1116. DOI:10.1152/japplphysiol.00341.2019