Abstract
Muscle dysfunction is one of the main features in individuals with chronic obstructive pulmonary disease (COPD). Handgrip strength (HS) has been used as a representation of general muscle strength in various populations, and a few studies found correlation between HS and other measures of upper and lower limbs’ muscle strength in the general population, although this was not yet studied in depth in COPD. The aims of this study were to verify if HS is cross-sectionally well correlated with upper and lower limbs’ muscle strength in individuals with COPD, and to identify a new cutoff for handgrip weakness in this population. HS was assessed by a dynamometer, whereas other muscle strength assessments comprised maximal voluntary contraction (MVC) of the quadriceps femoris and 1-repetition maximum (1RM) of biceps and triceps brachii, pectoralis major, latissimus dorsi and quadriceps femoris. Additional assessments included pulmonary function and volumes, body composition and exercise capacity. Fifty individuals with COPD were studied (65 ± 7 years; FEV1 51 ± 14%pred). HS showed moderate-to-strong correlations with all 1-RM assessments (0.62<r < 0.75) and especially with MVC of the quadriceps femoris (r = 0.83) (p < 0.05 for all). Correlations were stronger for male than female individuals, and the assessment performed on right, left or dominant hand did not present significant differences. A cutoff of 0.3892 for HS/weight yielded an AUC = 0.90 to identify weakness. In conclusion, HS is a good reflection of upper and lower limbs’ muscle strength in individuals with COPD. Its usefulness as a surrogate for more complex assessments must be based on the settings and conditions.
Introduction
Peripheral muscle dysfunction is a common feature of individuals with chronic obstructive pulmonary disease (COPD) and presents negative and important clinical implications such as reduction in exercise tolerance, quality of life and survival [Citation1]. Assessment of peripheral muscle strength in individuals with COPD was formally recommended by the American Thoracic Society (ATS) and European Respiratory Society (ERS) at least since 1999 [Citation2], and this recommendation was strongly reinforced in 2014 [Citation3]. The assessment of peripheral muscle strength can be performed through different approaches, including complex systems as computerized dynamometers, simple procedures as manual muscle testing, weight-based tests (i.e. multi-gym or dumb bells), and portable devices such as hand-held dynamometers and handgrip dynamometers [Citation4].
Handgrip strength (HS) is easy and quick to measure, both in the research and clinical settings [Citation4]. Although a few studies have considered HS as an indicator of peripheral muscle strength [Citation5–7], this assumption was not done based on solid evidence from the literature. While some studies suggested that HS is not a surrogate for lower limbs muscle strength since they concern different body segments [Citation8, Citation9], others suggest that this assessment should be considered mainly as the evaluation of hand function [Citation4].
In adults and healthy elderly, some evidence of moderate-to-strong correlations were found between HS and strength of knee extensor and other muscle groups from the lower limbs [Citation10–12]. Moreover, in individuals with COPD, a previous study found strong correlations of HS with some muscle groups of the upper limbs [Citation13]. However, in that study, measurements were focused on function rather than strength per se, i.e. through movements aimed to achieve the action of more than one muscle, thus preventing interpretations for each muscle group separately. Furthermore, the sample of that study was relatively small (n = 26), hence driving the need for a more representative sample in order to corroborate or oppose its conclusions. Lastly, to the best of the authors’ knowledge, no study so far has analyzed the influence of hand dominance/non-dominance in the assessment of HS in individuals with COPD, as well as no cutoff for identifying low values of HS was yet determined in this population when relating HS with strength of the lower limbs.
Therefore, aiming to circumvent these gaps, the objectives of this study were to verify if HS assessment with a simple hydraulic dynamometer is a good reflection of upper and lower limbs muscle strength in this population, and to identify a cutoff indicative of handgrip weakness which corresponds to a cutoff for weakness of the quadriceps femoris. Based on the previous literature in healthy individuals, the authors’ hypothesis was that at least moderate correlations are found between HS and muscle strength of upper and lower limbs. Secondarily, for methodological standardization purposes, the study aimed to verify whether there are differences in the assessment of HS concerning the assessment variations (right, left or dominant hand and the highest value of these options).
Methods
This is a retrospective analysis of baseline data from a convenience sample recruited at the outpatient clinics of Respiratory Physiotherapy and Pulmonology of State University of Londrina (UEL, Brazil) for inclusion in an exercise training program. The data collection scheme included in this study is represented in . Data were collected between 02/2016 and 02/2020 at the Laboratory of Research in Respiratory Physiotherapy of UEL. Inclusion criteria for the exercise training program were clinical diagnosis of COPD [Citation14]; clinical stability without exacerbations in the previous month; no severe/unstable cardiac disease; and no orthopedic, neurologic or muscular impairment that could hinder the assessments. Further, participants must have had no missing data regarding the assessments of peripheral muscle strength, pulmonary function, body composition, exercise capacity, demographics and anthropometric data, including self-reported right-handed or left-handed dominance, as described below. The study protocol was approved by the institutional Committee of Ethics in Research (n° 1.730.247), and all participants signed an informed consent form at inclusion.
HS was assessed in both hands with a validated hydraulic dynamometer (Saehan Corporation, Korea) [Citation15]. Participants performed three encouraged maximal attempts of each hand with three seconds of contraction while seated with arms along the body, elbow at 90° of flexion and wrist in a neutral position, and 30 s of rest were given between each attempt [Citation1, Citation4]. The highest value achieved in each hand was used for the analysis. Dominance as right-handed or left-handed was determined according to the individual’s report.
Other assessments of muscle strength were performed by the 1-repetition maximum (1-RM) test and maximal voluntary contraction (MVC). 1-RM was performed in a multi-gym (CRW 1000, Brazil) aiming to determine the maximal amount of weight that could be lifted up in one single movement of the tested muscles in their full range of motion [Citation1, Citation16]. The main muscles assessed were quadriceps femoris, biceps and triceps brachii, pectoralis major and latissimus dorsi. A more detailed description of the 1-RM assessment can be found in the supplementary material.
Isometric MVC of quadriceps femoris was assessed with a strain gauge dynamometer 200 kgf and a signal acquisition system (EMG System, Brazil). The dynamometer was fixed at the multi-gym and its extremity was fixed at the individual’s ankle in a seated position with back support, fastening straps on the trunk and hip and knee in a position of 90° [Citation17, Citation18]. A minimum of four and a maximum of 15 maneuvers were performed in an isometric MVC of each leg with duration of six seconds each. The two highest values should not present more than 5% of difference between them, otherwise the test was continued. Strong standardized encouragement was given during each maneuver.
Pulmonary function and volumes were assessed by spirometry and whole-body plethysmography, respectively, according to the ATS standardizations [Citation19, Citation20]. Assessments were based on post-bronchodilator values of forced expiratory volume on first second (FEV1), forced vital capacity (FVC) and their FEV1/FVC ratio, total lung capacity and functional residual capacity. Population-specific reference values were used for pulmonary function [Citation21] and for plethysmography [Citation22]. Body composition was assessed by bioelectrical impedance (Biodynamics, United States of America). Values of fat-free mass (FFM) were recorded and calculated specifically for individuals with chronic respiratory failure [Citation23]. FFM index and body mass index (BMI) were calculated by dividing FFM weight and body weight, respectively, by squared height. Exercise capacity was assessed with the 6-minute walking test (6MWT), according to international guidelines [Citation24] and with population-specific reference values [Citation25].
Statistical analysis
The Statistical Package of Social Sciences (SPSS) version 22.0 (SPSS Inc., USA) was used for statistical analysis. Considering the study by Marino et al. [Citation13] with r = 0.76, bilateral-α = 0.05 and β = 0.20 according to the table proposed by Hulley et al. [Citation26], a minimum of 13 individuals would be necessary. Based on Shapiro Wilk’s test, data normally distributed were described as mean ± standard deviation and data non-normally distributed as median (interquartile range 25–75%). Correlations between HS and other muscle groups and correlations of all muscle groups with each other were analyzed by the coefficients of Pearson or Spearman, according to normality in data distribution. Correlation coefficients were classified as weak (r < 0.50), moderate (0.50 ≤ r < 0.70) or strong (r ≥ 0.70).
One-way ANOVA or Kruskal Wallis test was used to analyze differences concerning the assessment variations of HS and MVC of quadriceps strength (right, left, dominant and the highest value of these options), according to normality in data distribution. The intraclass correlation coefficient (ICC) and 95% confidence interval (CI) between each two assessment options (right vs. left, and so on) was used to investigate the agreement among all options.
A Receiver Operational Characteristic (ROC) curve was performed to identify a cutoff indicative of handgrip weakness which corresponds to a known cutoff for weakness of the quadriceps femoris (i.e. quadriceps MVC lower than 120% of BMI [Citation27]), considering the clinical importance of the association of quadriceps weakness with functional impairment and mortality [Citation1, Citation27]. In order to correct for the individual’s body weight and due to the strong correlation found between HS and weight (r = 0.70; p < 0.001), the cutoff was based on the ratio of HS and weight (HS/weight). We also run ROC curves separately by sex. Student t or Mann Whitney tests were used for comparisons of muscle strength of all muscle groups assessed between male and female and between subjects with values of HS/weight below and above the identified cutoff, according to normality in data distribution. In order to show the relevance of the cutoff, Chi-square test was performed to verify the association between being weak based on the new cutoff and according to the quadriceps femoris cutoff [Citation27] and the 10th percentile of HS reference values as proposed by Burtin et al. [Citation28] and Spruit et al. [Citation29], since both classifications of weakness were associated with mortality, a very important outcome. The odds ratio (OR) of quadriceps femoris weakness according to HS weakness identified by the new cutoff was also calculated. For all analyses a P-value <0.05 was adopted as statistically significant.
Results
Fifty subjects were analyzed. shows that the sample had moderate-to-very severe airflow obstruction, BMI relatively high and exercise capacity relatively preserved. Forty-seven subjects (94% of the sample) reported their right hand as dominant and all others reported their left hand as dominant.
Table 1. Sample characteristics (n = 50).
Assessment variations of HS and quadriceps femoris MVC (i.e. right, left, dominant and the highest value) did not yield any statistically significant differences () (p > 0.05 for all). Also, there were high values of ICC between each pair of measures, both for HS and quadriceps femoris MVC, as described in . As HS values were statistically similar regardless of the choice, for further analysis we have focused on the values of the dominant hand as usual in the literature. Furthermore, male participants were stronger than female for all muscle strength assessments ( and , supplementary material).
Table 2. Values of muscle strength concerning the assessment variations of handgrip strength and maximal voluntary contraction of knee extensors.
Table 3. Agreement concerning the assessment variations of handgrip strength (HS) and maximal voluntary contraction (MVC) of knee extensors.
Table 4. Values of muscle strength of the total sample, male and female subjects.
HS showed moderate-to-strong significant correlations with all 1-RM assessments of upper and lower limbs, and especially with quadriceps femoris MVC (r = 0.83) (). Furthermore, in an additional analysis according to sex, correlations of HS with all assessed muscle groups of upper and lower limb muscles were stronger in male individuals ( and ). Correlations among all muscle strength assessments were generally moderate-to-strong (, supplementary material).
Figure 2. Correlations according to sex between handgrip strength and strength of upper limb muscle groups (A: biceps brachii; B: triceps brachii; C: pectoralis major; D: latissimus dorsi).
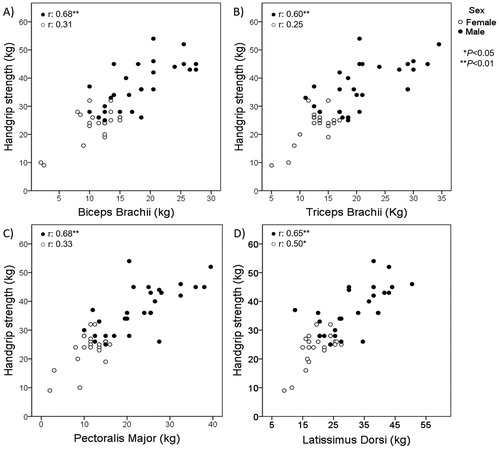
Figure 3. Correlations according to sex between handgrip strength and quadriceps femoris assessed by (A) maximum voluntary contraction and (B) 1-repetition maximum test. 1-RM: one-repetition maximum; MVC: maximum voluntary contraction.
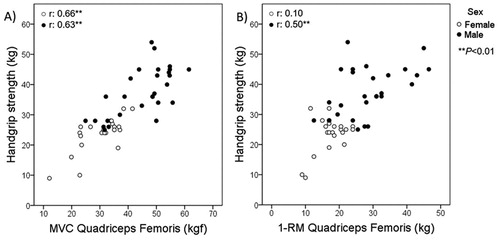
Table 5. Correlation of handgrip strength with upper and lower limbs’ muscle strength.
By using the quadriceps femoris MVC cutoff for weakness as reference, the ROC curve of the total sample identified the cutoff for HS/weight as 0.3892 with an AUC of 0.90, sensitivity 0.89 and specificity 0.87 (). Twenty-two individuals (40%) had HS/weight below or equal the cutoff, and they had significantly lower values of strength in all muscles assessed in comparison to those above the cutoff (p < 0.05 for all). Specifically, for males the cutoff identified for HS/weight was 0.3848 (AUC of 0.896, sensitivity 0.80 and specificity 0.91) and for females 0.4019 (AUC of 0.848; sensitivity 0.93 and specificity 0.75). Since the cutoff is corrected for body weight, additional analyses revealed strong correlation between HS/weight and quadriceps MVC in percentage of BMI and weak correlation between HS/weight and 1-RM quadriceps femoris ().
Figure 5. General correlations and correlations according to sex between handgrip/body weight and (A) quadriceps femoris MVC in % of BMI and (B) 1-RM quadriceps femoris. BMI: Body mass index; MVC: Maximal voluntary contraction; BMI: 1-RM: one-maximal repetition.
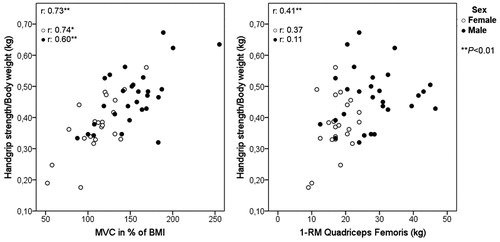
All three cutoffs classified the same individuals as weak, although only four individuals were classified as having reduced HS according to the cutoff based on 10th percentile of reference values [Citation28, Citation29]. Out of the 19 individuals identified with weakness of the quadriceps femoris, 17 (89.5%) were also identified as presenting HS weakness based on the new cutoff, in comparison to 2 individuals (10.5%) identified as having preserved HS (p < 0.001). The OR was 0.02 (95% CI: 0.00–0.13); therefore, the likelihood of having weakness of the quadriceps femoris was 98% higher for individuals with HS/weight below the new cutoff. There was also significant association between being classified as weak by the proposed cutoff identified in this study and by reference values [Citation28, Citation29] (p = 0.03). Cutoffs for HS in absolute value (i.e. not divided by weight) and using the 10th percentile of reference values [Citation28, Citation29] were attempted, however yielded results considerably inferior to the HS/weight cutoff or even not statistically significant.
Discussion
The main findings of this study comprise moderate-to-strong correlations between HS and strength of all muscle groups assessed from the upper and lower limbs, especially in male individuals. This suggests that despite the heterogeneity of muscle dysfunction in COPD [Citation1], HS can be considered as a good reflection of general muscle strength. Furthermore, a cutoff for HS/weight aimed at identifying HS weakness presented very good values of AUC, sensitivity and specificity. Finally, no significant differences were found among all variations of HS assessment.
Correlations found in this study agree with the previous literature in healthy individuals, which have shown moderate-to-strong associations between HS and muscle strength of trunk, upper and lower limbs [Citation6, Citation10, Citation12]. Similarly, our results also agree with those from Marino and colleagues in individuals with COPD, which showed strong correlations between HS and strength of knee extension, upper front pulley and supine sitting strength assessed by 1-RM [Citation13]. Of note, the present study brings additional and novel information in comparison to the study by Marino and colleagues [Citation13], including more than one assessment method (i.e. quadriceps femoris MVC), assessment of a larger number of specific muscle groups (rather than “grouped” movements) and a twice-as-large sample size.
It should be noted that even though HS was shown to reflect general muscle strength, the decision to use it as a surrogate of complex assessments must be based on certain contexts. One may argue that for prescription of exercise training in rehabilitation settings, more complex and specific tools are required for the assessment of severely weakened individuals or in studies requiring a more “in-depth” assessment of peripheral muscle strength. On the other hand, the applicability brought by these results is that HS assessment could be used in fact as an indicator of overall muscle strength in individuals with COPD in a context of screening or follow up investigations (e.g. hospitalized/critical individuals, primary care or multicenter studies involving large populations), or even in places where few resources are available.
HS has been used in studies involving a variety of populations [Citation6, Citation30–34], and was also used either as a component of indexes or for diagnostic purposes, such as for sarcopenia and frailty [Citation34, Citation35]. Some studies bring the assessment of “peripheral muscle strength” or “overall muscle strength” through HS testing [Citation7, Citation11, Citation36]. Moreover, despite being considerably cheaper than more complex methods, HS was also shown to be associated with prognosis and mortality in the general population [Citation7, Citation11, Citation36] and individuals with COPD [Citation28, Citation37]. This is therefore a measure of muscle strength that can be understood as a comprehensive and useful assessment method in individuals with COPD in certain contexts.
Regarding the stronger correlations found in male than in female participants for almost all muscle groups, we hypothesize that this may be explained by the larger variation in values of muscle strength in male than female (clearly seen in and , and also in the interquartile ranges presented in ), accompanied by a smaller variation of age in the male group than in the female group (). The less pronounced variation of strength in the female group may be explained by the statistically significant lower values of FFM index and also lower values and less variation in FFM weight found in women compared to men (). This finding was already expected, since it is known that women have less muscle mass than men [Citation3].
In this study, a cutoff for HS/weight (0.3892) with good AUC, sensitivity and specificity was identified. The differences between groups below and above this cutoff reinforce the representation of general muscle strength by HS, since participants below the cutoff showed worse values of strength in all muscle groups assessed. We have applied a ROC curve based on a cutoff of quadriceps femoris strength because in this population, quadriceps strength is the most assessed muscle in subjects with COPD and is known to be associated with mortality [Citation27], as well as HS [Citation28], and also by the fact that quadriceps MVC presented the best correlation with HS in our results.
This new cutoff may be useful to classify individuals with moderate-to-very severe COPD as having abnormal HS or not, reflecting whether these individuals present or not abnormal quadriceps strength, since individuals with reduced HS/weight presented a 98% higher likelihood of also having reduced quadriceps muscle strength. This novel cutoff may prove itself clinically relevant since it is based on a cutoff for weakness of the quadriceps femoris known to be associated with mortality [Citation27]. Although we found an association between being weak according to this new cutoff and the cutoff based on the 10th percentile of reference values [Citation28, Citation29], the later identified fewer individuals as weak possibly because it is more strict and identifies essentially individuals with very marked impairment. Future longitudinal studies may validate this new cutoff in a larger and more diverse cohort and investigate its capacity to predict death.
The absence of statistical significance among values of HS when different assessment choices were compared (i.e. right, left, dominant and highest value) makes comparison between studies more feasible, since apparently any option among those four may be used. A previous study corroborates this finding, describing close values when comparing HS of dominant and non-dominant hands in individuals from different continents [Citation38].
Limitations of the study
The retrospective design and the absence of a validation sample to test the proposed cutoff are two of the main limitations of this study. Another limitation was the lack of representation of individuals in GOLD stage I, which does not allow us at this moment to generalize these findings to individuals with mild COPD. These may be topics for future investigations.
Conclusion
In conclusion, HS assessed with a simple hydraulic dynamometer is a good reflection of upper and lower limbs’ muscle strength in individuals with COPD, especially in male individuals. Its usefulness as a surrogate for more complex assessments of peripheral muscle strength must be based on the context of use, aims, target groups and resources. Besides, we identified a new cutoff involving HS/weight which showed association with weakness of quadriceps femoris and may be the subject of further validation.
Author contributions
JF and FP had full access to all of the data and take responsibility as guarantors for the integrity of the data and the accuracy of the analysis and conclusions. JF, FVCM, LCS and KCF made substantial contributions to the conception or design of the work. All authors contributed substantially to the acquisition, analysis or interpretation of data for the work. Further, all authors also contributed by critically revising the article and providing the final approval of the version to be submitted.
Ethical approval
The study protocol was approved by the Institutional Committee of Ethics in Research (no. 1.730.247).
Acknowledgments
The authors would like to thank the colleagues from the Laboratory of Research in Respiratory Physiotherapy of UEL for their support.
Declaration of interest
The authors report no conflicts of interest in this work.
References
- Nyberg A, Saey D, Maltais F. Why and how limb muscle mass and function should be measured in patients with chronic obstructive pulmonary disease. Annals ATS. 2015;12(9):1269–1277.
- Casaburi R, Gosselink R, Decramer M, et al. Skeletal muscle dysfunction in chronic obstructive pulmonary disease. A statement of the American Thoracic Society and European Respiratory Society. Am J Respir Crit Care Med. 1999;159:S1–S40.
- Maltais F, Decramer M, Casaburi R, et al. An official American Thoracic Society/European Respiratory Society statement: update on limb muscle dysfunction in chronic obstructive pulmonary disease. Am J Respir Crit Care Med. 2014;189(9):e15–e62.
- Robles PG, Mathur S, Janaudis-Fereira T, et al. Measurement of peripheral muscle strength in individuals with chronic obstructive pulmonary disease: a systematic review. J Cardiopulm Rehabil Prev. 2011;31(1):11–24.
- Beenakker KG, Ling CH, Meskers CG, et al. Patterns of muscle strength loss with age in the general population and patients with a chronic inflammatory state. Ageing Res Rev. 2010;9(4):431–436.
- Rantanen T, Era P, Kauppinen M, et al. Maximal isometric muscle strength and socioeconomic status, health, and physical activity in 75-year-old persons. J Aging Phys Act. 1994;2(3):206–220.
- Sasaki H, Kasagi F, Yamada M, et al. Grip strength predicts cause-specific mortality in middle-aged and elderly persons. Am J Med. 2007;120(4):337–342.
- Bohannon RW. Dynamometer measurements of grip and knee extension strength: are they indicative of overall limb and trunk muscle strength? Percept Mot Skills. 2009;108(2):339–342.
- Kuh D, Bassey J, Butterworth S, et al. Grip strength, postural control, and functional leg power in a representative cohort of British men and women: associations with physical activity, health status, and socioeconomic conditions. J Gerontol Med Sci. 2005;60(2):224–231.
- Cawthon PM, Fullman RL, Marshall L, et al. Physical performance and risk of hip fractures in older men. J Bone Miner Res. 2008;23(7):1037–1044.
- Fragala MS, Alley DE, Shardell MD, et al. Comparison of handgrip and leg extension strength in predicting slow gait speed in older adults. J Am Geriatr Soc. 2016;64(1):144–150.
- Lauretani F, Russo CR, Bandinelli S, et al. Age-associated changes in skeletal muscles and their effect on mobility: an operational diagnosis of sarcopenia. J Appl Physiol. 2003;95(5):1851–1860.
- Marino DM, Marrara KT, Ike D, et al. Study of peripheral muscle strength and severity indexes in individuals with chronic obstructive pulmonary disease. Physiother Res Int. 2010;15(3):135–143.
- Global strategy for the diagnosis, management, and prevention of chronic obstructive pulmonary disease. 2018. Report. Global Initiative for Chronic Obstructive Disease. 2018:1-123 [assessed 2019 September 16]. https://goldcopd.org/wp-content/uploads/2017/11/GOLD-2018-v6.0-FINAL-revised-20-Nov_WMS.pdf.
- Reis MM, Arantes PMM. Assessment of hand grip strength – Validity and reliability of the Saehan Dynamometer. Fisioter Pesqui. 2011;18(2):176–181.
- Brown LE, Weir JP. ASEP Procedures recommendation I: accurate assessment of muscular strength and power. J Exerc Physiol. 2001;4(3):1–21.
- Edwards RHT, Young A, Hosking GP, et al. Human skeletal muscle function - description of tests and normal values. Clin Sci Mol Med. 1977;52(3):283–290.
- Hopkinson NS, Tennant RC, Dayer MJ, et al. A prospective study of decline in fat free mass and skeletal muscle strength in chronic obstructive pulmonary disease. Respir Res. 2007;8(1):25.
- Miller MR, Crapo R, Hankinson J, et al. General considerations for lung function testing. Eur Respir J. 2005;26(1):153–161.
- Wanger J, Clausen JL, Coates A, et al. Standardisation of the measurement of lung volumes. Eur Respir J. 2005;26(3):511–522.
- Pereira CAC, Sato T, Rodrigues SC. New reference values for forced spirometry in white adults in Brazil. J Bras Pneumol. 2007;33(4):397–406.
- Neder JA, Andreoni S, Castelo-Filho A, et al. Reference values for lung function tests. I. Static volumes. Braz J Med Biol Res. 1999;32(6):703–717.
- Kyle UG, Pichard C, Rochat T, et al. New bioelectrical impedance formula for patients with respiratory insufficiency: comparison to dual-energy X-ray absorptiometry. Eur Respir J. 1998;12(4):960–966.
- Holland AE, Spruit MA, Troosters T, et al. An official European Respiratory Society/American Thoracic Society technical standard: field walking tests in chronic respiratory disease. Eur Respir J. 2014;44(6):1428–1446.
- Britto RR, Probst VS, de Andrade AF, et al. Reference equations for six-minute walk distance based on a Brazilian multicenter study. Braz J Phys Ther. 2013;17(6):556–563.
- Hulley SB, Cummings SR, Browner WS, et al. Delineando a pesquisa clínica. Uma abordagem epidemiológica. 4th ed. Porto Alegre: Artmed; 2015.
- Swallow EB, Reyes D, Hopkinson NS, et al. Quadriceps strength predicts mortality in patients with moderate to severe chronic obstructive pulmonary disease. Thorax. 2007;62(2):115–120.
- Burtin C, Ter Riet G, Puhan MA, et al. Handgrip weakness and mortality risk in COPD: a multicentre analysis. Thorax. 2016;71(1):86–87.
- Spruit MA, Sillen MJH, Groenen MTJ, et al. New normative values for handgrip strength: results from the UK Biobank. J Am Med Dir Assoc. 2013;14(10):775.e5–775.e11.
- McNicholl T, Curtis L, Dubin JA, et al. Handgrip strength predicts length of stay and quality of life in and out of hospital. Clin Nutr. 2019;39(8):2501–2509.
- Riviati N, Setiati S, Laksmi PW, et al. Factors related with handgrip strength in elderly patients. Acta Med Indones - Indones J Intern Med. 2017; 49(3):215–219.
- Turusheva A, Frolova E, Degryse J-M. Age-related normative values for handgrip strength and grip strengths usefulness as a predictor of mortality and both cognitive and physical decline in older adults in northwest Russia. J Musculoskelet Neuronal Interact. 2017;17(1):417–432.
- Volaklis KA, Halle M, Thorand B, et al. Handgrip strength is inversely and independently associated with multimorbidity among older women: results from the KORA-Age study. Eur J Intern Med. 2016;31:35–40.
- Bahat G, Tufan A, Tufan F, et al. Cut-off points to identify sarcopenia according to European Working Group on Sarcopenia in Older People (EWGSOP) definition. Clin Nutr. 2016;35(6):1557–1563.
- Fried LP, Tangen CM, Walston J, et al. Frailty in older adults evidence for a phenotype. J Gerontol. 2001;56(3):M146–M56.
- Leong DP, Teo KK, Rangarajan S, et al. Prognostic value of grip strength: findings from the Prospective Urban Rural Epidemiology (PURE) study. The Lancet. 2015;386(9990):266–273.
- Martinez CH, Diaz AA, Meldrum CA, et al. Handgrip strength in chronic obstructive pulmonary disease. Annals ATS. 2017;14(11):1638–1645.
- Leong DP, Teo KK, Rangarajan S, et al. Reference ranges of handgrip strength from 125,462 healthy adults in 21 countries: a prospective urban rural epidemiologic (PURE) study. J Cachexia Sarcopenia Muscle. 2016;7(5):535–546.