Abstract
Several observational studies report decreased incidence of mortality and of exacerbations with aspirin use in patients with chronic obstructive pulmonary disease (COPD), with calls for a large randomized trial. Aspirin does have local and systemic pulmonary mechanisms of action that could make this drug beneficial in the treatment of COPD. However, the potential for biases in the observational studies has not been examined. We searched the literature for all observational studies reporting on the effect of aspirin in COPD patients on exacerbation and mortality. We reviewed the studies for the presence of time-related and other biases. We identified eight observational studies reporting an overall reduction in all-cause mortality or exacerbation with aspirin use of 21% (pooled rate ratio (RR) 0.79; 95% CI 0.71–0.86). We found two studies affected by immortal time bias (pooled RR 0.81; 95% CI 0.74–0.89), three studies affected by collider-stratification bias (pooled RR 0.66; 95% CI 0.55–0.79) and three that involved some exposure misclassification (pooled RR 0.85; 95% CI 0.78–0.92). Moreover, while adjusting for cardiovascular factors, six of the eight studies did not adjust for important markers of COPD severity and thus remain susceptible to confounding bias. In conclusion, all observational studies reporting on the effectiveness of aspirin on major outcomes of COPD are affected by biases known to exaggerate the effectiveness of a drug. As these studies cannot be used to support a beneficial effect for aspirin in COPD, it would be premature to consider a randomized trial to investigate this question until methodologically rigorous studies are available.
Introduction
Chronic obstructive pulmonary disease (COPD) is a progressive lung disease characterized by chronic bronchitis and impaired lung function. It is a major cause of disability and has become the third leading cause of death globally [Citation1]. Cardiovascular disease is a major co-morbidity in COPD patients and is a predictor of all-cause mortality [Citation2].
Several studies have investigated the potential benefits of cardiovascular drugs, including statins and beta-blockers, reporting important reductions in COPD exacerbations and mortality with these drugs [Citation3,Citation4]. Subsequent large randomized trials could not confirm these findings [Citation5,Citation6]. It was noted that some observational studies were affected by time-related biases that tend to erroneously introduce or exaggerate benefits of drugs [Citation7,Citation8].
Aspirin has also been the subject of several observational studies that reported decreased incidence of death and exacerbations with aspirin use in patients with COPD, with calls for a large randomized trial [Citation9]. Indeed, a meta-analysis of five observational studies reported a relative risk of all-cause mortality of 0.81 (95% CI: 0.75–1.13) with aspirin/antiplatelet use vs. nonuse in patients with COPD, concluding that “antiplatelet therapy might significantly contribute to reduce all-cause mortality in COPD patients” [Citation10]. However, while the authors of the meta-analysis employed various quantitative standards and checklists to appraise the studies, the potential for time-related and other biases in the observational studies were not investigated.
In this article, we perform a methodological review of the observational studies reporting on the effectiveness of aspirin on the major outcomes of mortality and exacerbation in patients with COPD. In particular, we examine the different study designs and data analyses for potential biases, including time-related biases.
Methods
MEDLINE and EMBASE were searched by the first author from their inception to July 2021 for observational studies of the effects of aspirin on mortality or exacerbations in patients with COPD. Keywords and derivations for “COPD,” “Obstructive Lung Disease” and “aspirin,” “acetylsalicylic acid,” “anti-platelet therapy” were used. Duplicate studies were removed, and the titles and abstracts of studies were screened to identify eligible studies. Full-texts of potentially eligible studies were reviewed by both authors and the references of all eligible studies were examined. We only included studies that provided information on the effects of aspirin or antiplatelet therapy on mortality or on COPD exacerbations.
The methods section of each publication was reviewed for time-related biases, such as immortal time bias, and for other sources of biases. Rate ratios and hazard ratios were extracted, as well as the confidence intervals and sample sizes, in order to create the forest plots. The study-specific results were stratified according to the type of bias and pooled using a random effects model due to the likelihood of substantial heterogeneity between studies [Citation11]. The heterogeneity assumption was due to differences in the treatment effect arising from different study populations, different definitions of aspirin exposure, or different length of follow-up, among other factors. All statistics and analyses were carried out on R version 1.2.5033 (R Core Team, 2020), the meta (v4.18-2), metaphor (v 3.0-2) and the forestplot packages (v.10.1) [Citation12–14].
Results
We identified 2,206 records from the database search (). After screening, 14 articles met the inclusion criteria, of which four were excluded because they did not provide results for exacerbation or mortality [Citation15–18], one was a review [Citation19], and one because it was a meta-analysis [Citation10]. Thus, we reviewed the 8 studies that reported on aspirin effectiveness in COPD for the outcomes of interest, namely exacerbation or mortality [Citation20–27].
All studies reported on mortality except one for which the outcome was moderate or severe exacerbation () [Citation26]. The overall rate ratio of mortality or exacerbation across the eight studies was 0.79 (95% CI 0.71–0.86). displays these according to the nature of the bias, with two studies affected by immortal time bias (pooled rate ratio 0.81; 95% CI 0.74–0.89), three studies by collider-stratification bias (pooled rate ratio 0.66; 95% CI 0.55–0.79) and three involved exposure misclassification (pooled rate ratio 0.85; 95% CI 0.78–0.92), described below.
Figure 1. Forest plot of reported hazard and rate ratios of mortality associated with aspirin use in COPD from the eight observational studies from . Pooled estimates by a random effects approach grouped according to immortal time bias, collider-stratification bias and exposure misclassification are illustrated. Abbreviations: RR: rate ratio; CI: confidence interval.
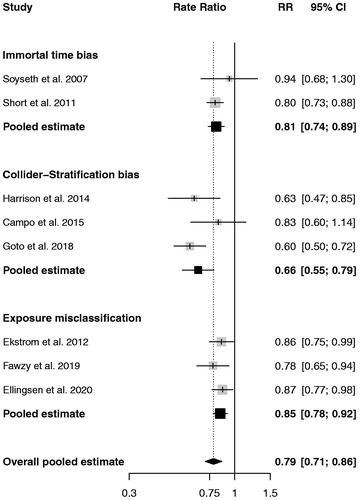
Table 1. Observational studies reporting on the effects of aspirin in COPD on the risk of death or exacerbation.
Immortal time bias
Two of the eight studies were affected by immortal time bias [Citation20,Citation21]. Immortal time is a period of time during the observation or follow-up of a cohort in which the outcome under study cannot occur [Citation28]. The bias is introduced when the exposure status is established after the start of follow-up, so that a patient classified as exposed must have remained alive and event-free, and thus “immortal,” until the exposure criterion is met.
Immortal time bias arises when there is either a misclassification or exclusion of this “immortal” time [Citation29]. It inflates the rate of outcome under study in the unexposed group and underestimates the rate in the treated group, so that the treatment under study appears to have a protective effect in preventing the outcome under study [Citation28,Citation29]. Immortal time bias has been shown to affect several observational studies of medication effects in COPD, including inhaled corticosteroids, statins and beta-blockers [Citation7,Citation8,Citation29–31].
An example of immortal time bias is the retrospective cohort study carried out by Soyseth et al. which evaluated the effects of various cardiovascular drugs on COPD patients with and without cardiovascular disease [Citation20]. The study included 854 patients hospitalized for a COPD exacerbation in a Norwegian hospital from 1 January 2000 to 31 December 2003. Patients were followed from hospital discharge until death or end of observation. The exposure status to the cardiovascular drugs was done through a retrospective search of medical records, so that aspirin exposure could have also included new aspirin use after hospital discharge. In this case, the time period between hospital discharge and the first prescription for aspirin in the medical record is “immortal” because the patient must have survived until the first prescription. Since follow-up for patients classified as “exposed” to aspirin began immediately after discharge, these exposed patients will have an artificial survival advantage () [Citation28]. This survival advantage in aspirin users falsely increases the rate of outcome among aspirin nonusers and creates the inaccurate result that aspirin exposure is “protective” and effective in reducing the outcome under study.
Figure 2. Illustration of immortal time bias in typical exposed and unexposed subjects from cohort studies. Drug exposure is defined after cohort entry. Therefore, the time between cohort entry (time 0) and the first drug exposure is immortal, because the patient had to survive this period to be classified as exposed. Abbreviation: COPD: chronic obstructive pulmonary disease.
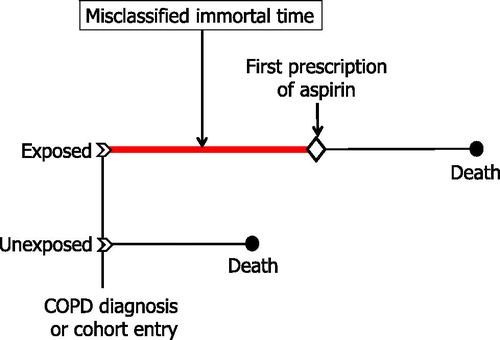
Immortal time bias also impacted similarly the study by Short et al. [Citation21] Indeed, the retrospective cohort study of 5,977 patients discharged from hospital after a COPD diagnosis used prescription data for aspirin at any time during follow-up. The solution for these studies is to fully account for all the misclassified immortal time at the design and analysis stage using a time-dependent analysis. This approach has been described in detail previously [Citation28].
Collider-stratification bias
Three of the eight studies were potentially affected by collider-stratification bias [Citation23–25]. Collider-stratification bias, or collider bias, is a form of selection bias that has been shown to affect some observational studies as a result of the process by which individuals are selected into the study [Citation32,Citation33]. Selection bias due to collider-stratification is introduced when the study cohort is defined by a common effect of the exposure and outcome, called a collider [Citation33]. Collider-stratification bias can introduce an association between the exposure and outcome, where one does not exist [Citation33,Citation34]. It can also reverse the direction of an association, making a harmful exposure appear protective and create a paradoxical relationship [Citation34].
We use two examples to explain this bias, first is the example of the “obesity paradox” where obesity, which is associated with higher mortality, appears to confer a survival advantage when the study is conducted among patients with CVD/diabetes [Citation35]. In this situation, obese people are more likely to develop CVD and diabetes, with obesity present before the onset of CVD/diabetes. Thus, studies conducted among patients with CVD/diabetes necessarily used exposure to obesity measured pre-CVD/diabetes, which makes CVD/diabetes a collider and causes collider-stratification bias. This bias would be avoided in a study conducted among patients with CVD/diabetes if it included patients with no prior obesity and compared mortality between those who develop obesity during follow-up with those who do not, with proper attention given to timing. Another relevant example to COPD is inhaled corticosteroids (ICS) that are associated with an increased incidence of pneumonia and pneumonia-related mortality [Citation36]. However, in studies conducted among patients hospitalized for pneumonia (the collider), prior ICS use appears to decrease mortality [Citation37]. However, this “paradoxical” effect of ICS was shown to be affected by collider-stratification bias [Citation38]. Here again, a proper study design that avoids this bias for the question of ICS and mortality among patients hospitalized for pneumonia would include patients with no prior ICS use and compare mortality between those who initiate ICS during follow-up with those who do not.
The three studies that we identified with this bias were based on cohorts of patients defined by a hospitalization for COPD exacerbation, a potential collider in the association between aspirin use and mortality. Indeed, these studies report aspirin as protective against incidence of major outcomes associated with COPD hospitalization, such as death [Citation23–25]. However, it has been well-established that severe COPD exacerbations requiring hospitalizations worsen the course of the disease, increase risk of subsequent exacerbations and increase the risk of death [Citation39–42]. Moreover, aspirin use is potentially associated with a decrease in COPD hospitalization, as hypothesized in all studies. Thus, the COPD hospitalization that defines the cohort is a potential collider.
presents the directed acyclic graph that depicts the collider-stratification due to the hypothesized relations between aspirin use, COPD hospitalizations, and mortality, with aspirin use and unmeasured shared risk factors colliding at COPD hospitalization. Since the studies report a protective effect with aspirin on COPD hospitalizations and on mortality, and previous literature has established hospitalizations for COPD as a predictor for mortality, conditioning/stratifying on COPD hospitalizations biases the results of the study through introducing a noncausal association between aspirin use and unmeasured shared risk factors, such as genetic and lifestyle factors [Citation33]. This noncausal association creates a pathway that affects the aspirin–mortality relationship and can result in an apparent protective effect of aspirin on COPD hospitalizations and mortality, reported in the three studies.
Figure 3. Classic directed acyclic graph representing selection bias due to collider-stratification as a result of defining the study cohort by COPD hospitalization. COPD hospitalization and death share several unmeasured risk factors. Studies reporting a decrease in COPD hospitalization and death with aspirin use, imply an association between aspirin and hospitalization for COPD, making COPD hospitalization a collider (indicated by the box). Hence, conditioning on COPD hospitalization through adjustment, stratification or restriction will induce collider-stratification bias. Abbreviation: COPD: chronic obstructive pulmonary disease.
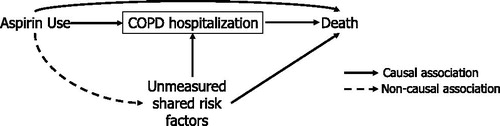
An example is the cohort study of 206,686 patients hospitalized for an acute exacerbation of COPD, identified from the State Inpatient Databases (SID) of seven US states [Citation25]. The study cohort was defined by a hospitalization for an AECOPD, with aspirin exposure measured prior to the hospitalization defining cohort entry, a design potentially subject to collider-stratification bias (). Indeed, 13,826 patients used aspirin prior to the COPD hospitalization, resulting in an adjusted odds ratio of in-hospital mortality of 0.60 (95% CI 0.50–0.72) with prior aspirin use compared with nonuse. The data analysis was adjusted for age, sex, race/ethnicity, household income, comorbidity, hospitalization year and region. Nevertheless, such adjustment is insufficient to bypass collider-stratification bias [Citation33].
Exposure misclassification
The remaining three studies used designs that were subject to some form of bias from exposure misclassification [Citation22,Citation26,Citation27]. For example, the cohort study of patients starting long-term oxygen therapy for COPD in Sweden from 2005 to 2009 assessed the effect of various cardiovascular drugs on mortality [Citation22]. In particular, antiplatelet drugs, mainly aspirin, were found to improve survival in these patients (HR 0.86; 95% CI: 0.75–0.99). This study avoided immortal time bias by using a time-dependent definition for drug exposures, though the approach was subject to exposure misclassification. Indeed, using 91-day intervals as the time unit, patients were considered exposed for outcome events occurring during a given interval if they received a prescription for aspirin in the previous 91-day interval. The change of status from exposed to unexposed periods was defined as “at least the two full 91-day periods preceding event or censoring had zero exposure,” which can lead to some misclassification of exposure.
describes this bias by depicting six hypothetical patients from the study cohort over five 91-day periods. Patient 1 is unexposed throughout all periods, so that the outcome event in period 5 is classified as unexposed. In contrast, patient 2 only had one full 91-day period of non-exposure, so that it is unclear how this event in the second interval is classified according to the definitions. Patient 3 received a prescription in the final days of the first interval and then had two successive periods of no prescription outtakes, so that the event in period 4 is classified as unexposed. In contrast, patient 4 received a prescription early on in the first interval followed by one period of no prescriptions, resulting in the event in period 3 classified as exposed. Paradoxically, the time between the prescription and the event in the “unexposed patient” (patient 3) is shorter than that for the exposed subject (patient 4), which can contribute to exposure misclassification. Patient 5 is another example of a patient classified as exposed while patient 6 is classified as unexposed despite the fact that they received a prescription within the same 91-day period they had the event, adding to exposure misclassification.
Figure 4. Illustration of exposure misclassification in the exposure definition in the cohort study by Ekstrom et al. [Citation22] Patients classified as exposed may have in-fact had minimal exposure to aspirin.
![Figure 4. Illustration of exposure misclassification in the exposure definition in the cohort study by Ekstrom et al. [Citation22] Patients classified as exposed may have in-fact had minimal exposure to aspirin.](/cms/asset/45ac942f-2883-4923-91cb-4f8f71d1dc0b/icop_a_1963696_f0004_b.jpg)
The Subpopulations and Intermediate Outcome Measures in COPD Study (SPIROMICS) cohort followed for patients with COPD for 36 months and identified acute exacerbations of COPD by telephone questionnaires [Citation26]. Aspirin users at baseline were matched one-to-one with nonusers using propensity scores, resulting in a reduced incidence rate of exacerbation (IRR 0.78; 95% CI 0.65–0.94) with aspirin use. However, aspirin exposure was not updated during follow-up, thus, lacking analyses of adherence or duration of aspirin use. But since the study is investigating mortality 3 years after entry into cohort, it is unclear to what extent aspirin use was continued throughout the duration of the study or could have been initiated during this follow-up, resulting in exposure misclassification.
Finally, the cohort study of 17,745 patients with COPD, identified using 76 primary healthcare centers across Sweden, recruited on the day of the first COPD diagnosis and followed until death [Citation27]. The use of ASA was associated with a decreased risk of death per defined daily dose (HR 0.87; 95% CI: 0.77–0.98). This study avoided immortal time bias using a time-dependent definition for ASA exposure. However, ASA use and cumulative defined daily dose was updated on a yearly basis, which may be too coarse to avoid bias from exposure misclassification.
Confounding bias
The studies adjusted for various cardiovascular and respiratory factors (). However, six of the eight studies were potentially affected by significant confounding bias from the absence of control for two important markers of COPD severity, prior exacerbations and hospitalizations for COPD, when comparing aspirin users with nonusers () [Citation20,Citation22–26]. For example, the SID cohort study adjusted for a wide range of co-morbidity measures of cardiovascular prognostic factors, but not COPD severity [Citation25]. Indeed, one of the most significant predictors of severe exacerbations and mortality is prior exacerbations and hospitalizations for COPD [Citation39,Citation42]. Thus, in the absence of this information, the results are subject to bias from confounding by COPD severity if the users and nonusers of aspirin differ in COPD severity. Another example is the Swedish cohort study that adjusted for PaO2 and PaCO2 as proxies for severity [Citation22]. However, there was no adjustment for prior exacerbations and the covariates were not updated after baseline, so that residual confounding cannot be ruled out.
Table 2. Presence of adjustment for COPD-specific confounders in the observational studies reporting on the effects of aspirin in COPD on mortality or exacerbation.
Discussion
Observational studies are now used extensively as real world evidence to identify new indications for drugs approved and prescribed in other indications [Citation43]. Our methodological review of the studies of aspirin as a potential treatment for COPD found that all eight studies which reported on the outcomes of exacerbations or mortality were affected by three major biases. Two of these, immortal time bias and collider-stratification bias tend to overestimate the benefit of a drug, while information bias from exposure misclassification may or may not. We also found that several studies did not control for imbalances in COPD severity between users and nonusers of aspirin, so that significant confounding bias could not be ruled out.
COPD and cardiovascular disease (CVD) are leading causes of mortality that share a number of risk factors such as age and smoking. CVD is an established predictor of mortality and exacerbations in COPD, and thus, an important factor in the clinical management of patients with COPD [Citation44]. Hence, there is interest in examining the potential benefits of cardiovascular drugs, such as aspirin, to improve respiratory-related outcomes in patients with COPD. Indeed, aspirin has local and systemic pulmonary mechanisms of action that may be beneficial in COPD. It suppresses circulating inflammatory markers IL-6 and C-reactive protein, both of which when constantly elevated have been reported in a subgroup of patients with COPD [Citation45,Citation46]. It was, thus, judicious to initially conduct observational studies to investigate the potential effectiveness of aspirin in COPD [Citation9]. However, our methodological review of the eight published studies to date concluded that the biases were too important to use the results as a basis for the conduct of a randomized trial.
There are other examples of medications used to treat cardiovascular diseases that have been studied for the treatment of COPD. Statins were the object of several observational studies that reported significant reductions in mortality and exacerbations among patients with COPD [Citation3,Citation20,Citation47]. However, these studies were shown to be affected by major time-related biases, including immortal and immeasurable time biases that tend to exaggerate the benefit of a drug [Citation7]. This potential benefit was subsequently refuted in the large STATCOPE randomized trial that evaluated the potential effectiveness of simvastatin in reducing the incidence of exacerbations in COPD [Citation5]. Beta-blockers were also associated, in several observational studies, with significant reductions in mortality and exacerbations among patients with COPD [Citation4]. However, these studies were shown to be affected by two sources of bias, namely confounding by contraindication and immortal time bias [Citation8]. The subsequent randomized βLOCK-COPD trial found no benefit with the beta-blocker metoprolol in reducing the incidence of exacerbations in COPD [Citation6].
In conclusion, observational studies are important to assess the effects of approved medications and in particular to investigate new indications for these drugs. However, their proper design and analysis are essential to avoid bias. Immortal time bias, collider-stratification bias and bias from exposure misclassification that we found in the observational studies of aspirin in COPD can be avoided. In addition, the absence of any control for imbalances in COPD severity measures in several studies, which introduces confounding bias, was surprising. At this time, new observational studies that use rigorous methods to avoid such time-related and confounding biases are needed to address this uncertainty regarding the potentially beneficial role of aspirin in COPD. In the meantime, the biases present in the eight observational studies are too important to contemplate the conduct of a large-scale randomized trial of aspirin effectiveness on reducing exacerbations and mortality in patients with COPD.
Declaration of interest statement
Dr. Suissa attended scientific advisory committee meetings for Atara, Merck, Pfizer and Seqirus, and received speaking fees from Boehringer-Ingelheim and Novartis. Mr. Bakshi has no conflict of interest.
References
- Murray CJ, Atkinson C, Bhalla K, et al. The state of US health, 1990-2010: burden of diseases, injuries, and risk factors. JAMA. 2013;310:591–608. DOI:10.1001/jama.2013.13805
- Huiart L, Ernst P, Suissa S. Cardiovascular morbidity and mortality in COPD. Chest. 2005;128(4):2640–2646. DOI:10.1378/chest.128.4.2640
- Mancini GB, Etminan M, Zhang B, et al. Reduction of morbidity and mortality by statins, angiotensin-converting enzyme inhibitors, and angiotensin receptor blockers in patients with chronic obstructive pulmonary disease. J Am Coll Cardiol. 2006;47(12):2554–2560. DOI:10.1016/j.jacc.2006.04.039
- Etminan M, Jafari S, Carleton B, et al. Beta-blocker use and COPD mortality: a systematic review and meta-analysis. BMC Pulm Med. 2012;12:48. DOI:10.1186/1471-2466-12-48
- Criner GJ, Connett JE, Aaron SD, et al. Simvastatin for the prevention of exacerbations in moderate-to-Severe COPD. N Engl J Med. 2014;370(23):2201–2210. DOI:10.1056/NEJMoa1403086
- Dransfield MT, Voelker H, Bhatt SP, et al. Metoprolol for the prevention of acute exacerbations of COPD. N Engl J Med. 2019;381(24):2304–2314. DOI:10.1056/NEJMoa1908142
- Suissa S. Co-morbidity in COPD: the effects of cardiovascular drug therapies. Respiration. 2010;80(1):3–7. DOI:10.1159/000315387
- Suissa S, Ernst P. Beta-Blockers in COPD: a methodological review of the observational studies. COPD. 2018;15(5):520–525. DOI:10.1080/15412555.2018.1554637
- Sin DD. The devastating power of platelets in COPD exacerbations: can aspirin save lives in COPD? Thorax. 2014;69(7):603–604. DOI:10.1136/thoraxjnl-2014-205329
- Pavasini R, Biscaglia S, d’Ascenzo F, et al. Antiplatelet treatment reduces all-cause mortality in COPD patients: a systematic review and meta-analysis. COPD. 2016;13(4):509–514. DOI:10.3109/15412555.2015.1099620
- Riley RD, Higgins JPT, Deeks JJ. Interpretation of random effects Meta-analyses. BMJ. 2011;342:d549. DOI:10.1136/bmj.d549
- Balduzzi S, Rücker G, Schwarzer G. How to perform a Meta-analysis with R: a practical tutorial. Evid Based Ment Health. 2019;22(4):153–160. DOI:10.1136/ebmental-2019-300117
- Viechtbauer W. Conducting meta-analyses in R with the metafor package. J Stat Soft. 2010;36(3):1–48.
- Gordon M, Lumley T. Forestplot: advanced forest plot using ‘grid’ graphics. R package version 1.10.1. 2020. https://cran.r-project.org/web/packages/forestplot/forestplot.pdf
- Aaron CP, Schwartz JE, Hoffman EA, et al. A longitudinal cohort study of aspirin use and progression of emphysema-like lung characteristics on CT imaging: the MESA lung study. Chest. 2018;154(1):41–50. DOI:10.1016/j.chest.2017.11.031
- McKeever TM, Lewis SA, Smit HA, et al. The association of acetaminophen, aspirin, and ibuprofen with respiratory disease and lung function. Am J Respir Crit Care Med. 2005;171(9):966–971. DOI:10.1164/rccm.200409-1269OC
- Kunadian V, Wilson N, Stocken DD, et al. Antiplatelet therapy in the primary prevention of cardiovascular disease in patients with chronic obstructive pulmonary disease: a randomised controlled proof-of-concept trial. ERJ Open Res. 2019;5(3):00110–2019. DOI:10.1183/23120541.00110-2019
- Yayan J. No significant detectable anti-infection effects of aspirin and statins in chronic obstructive pulmonary disease. Int J Med Sci. 2015;12(3):280–287. DOI:10.7150/ijms.11054
- Mallah H, Ball S, Sekhon J, et al. Platelets in chronic obstructive pulmonary disease: an update on pathophysiology and implications for antiplatelet therapy. Respir Med. 2020;171:106098. DOI:10.1016/j.rmed.2020.106098
- Soyseth V, Brekke PH, Smith P, et al. Statin use is associated with reduced mortality in COPD. Eur Respir J. 2007;29(2):279–283. DOI:10.1183/09031936.00106406
- Short PM, Lipworth SI, Elder DH, et al. Effect of beta blockers in treatment of chronic obstructive pulmonary disease: a retrospective cohort study. BMJ. 2011;342:d2549. DOI:10.1136/bmj.d2549
- Ekstrom MP, Hermansson AB, Strom KE. Effects of cardiovascular drugs on mortality in severe chronic obstructive pulmonary disease. Am J Respir Crit Care Med. 2013;187(7):715–720. DOI:10.1164/rccm.201208-1565OC
- Harrison MT, Short P, Williamson PA, et al. Thrombocytosis is associated with increased short and long term mortality after exacerbation of chronic obstructive pulmonary disease: a role for antiplatelet therapy? Thorax. 2014;69(7):609–615. DOI:10.1136/thoraxjnl-2013-203996
- Campo G, Pavasini R, Malagu M, et al. Relationship between troponin elevation, cardiovascular history and adverse events in patients with acute exacerbation of COPD. COPD. 2015;12(5):560–567. DOI:10.3109/15412555.2014.995293
- Goto T, Faridi MK, Camargo CA, et al. The association of aspirin use with severity of acute exacerbation of chronic obstructive pulmonary disease: a retrospective cohort study. NPJ Prim Care Respir Med. 2018;28(7). DOI:10.1038/s41533-018-0074-x
- Fawzy A, Putcha N, Aaron CP, et al. Aspirin use and respiratory morbidity in COPD: a propensity score-matched analysis in subpopulations and intermediate outcome measures in COPD study. Chest. 2019;155(3):519–527. DOI:10.1016/j.chest.2018.11.028
- Ellingsen J, Johansson G, Larsson K, et al. Impact of comorbidities and commonly used drugs on mortality in COPD - Real-World data from a primary care setting. Int J Chron Obstruct Pulmon Dis. 2020;15:235–245. DOI:10.2147/COPD.S231296
- Suissa S. Immortal time bias in pharmaco-epidemiology. Am J Epidemiol. 2008;167(4):492–499. DOI:10.1093/aje/kwm324
- Suissa S. Effectiveness of inhaled corticosteroids in chronic obstructive pulmonary disease: immortal time bias in observational studies. Am J Respir Crit Care Med. 2003;168(1):49–53. DOI:10.1164/rccm.200210-1231OC
- Samet JM. Measuring the effectiveness of inhaled corticosteroids for COPD is not easy! Am J Respir Crit Care Med. 2003;168(1):1–2. DOI:10.1164/rccm.2304004
- Suissa S. Immortal time bias in observational studies of drug effects. Pharmacoepidemiol Drug Saf. 2007;16(3):241–249. DOI:10.1002/pds.1357
- Greenland S. Quantifying biases in causal models: classical confounding vs collider-stratification bias. Epidemiology. 2003;14(3):300–306.
- Cole SR, Platt RW, Schisterman EF, et al. Illustrating bias due to conditioning on a collider. Int J Epidemiol. 2010;39(2):417–420. DOI:10.1093/ije/dyp334
- Hernández-Díaz S, Schisterman EF, Hernán MA. The birth weight "paradox" uncovered? Am J Epidemiol. 2006;164(11):1115–1120. DOI:10.1093/aje/kwj275
- Banack HR, Kaufman JS. Does selection bias explain the obesity paradox among individuals with cardiovascular disease? Ann Epidemiol. 2015;25(5):342–349. DOI:10.1016/j.annepidem.2015.02.008
- Ernst P, Saad N, Suissa S. Inhaled corticosteroids in COPD: the clinical evidence. Eur Respir J. 2015;45(2):525–537. DOI:10.1183/09031936.00128914
- Festic E, Bansal V, Gupta E, et al. Association of inhaled corticosteroids with incident pneumonia and mortality in COPD patients; systematic review and meta-analysis. COPD. 2016;13(3):312–326. DOI:10.3109/15412555.2015.1081162
- Suissa S. Inhaled corticosteroids preventing pneumonia mortality: paradox or selection bias? Eur Respir J. 2019;53(2):1802112. DOI:10.1183/13993003.02112-2018
- Soler-Cataluña JJ, Martínez-García MA, Román Sánchez P, et al. Severe acute exacerbations and mortality in patients with chronic obstructive pulmonary disease. Thorax. 2005;60(11):925–931. DOI:10.1136/thx.2005.040527
- Bustamante-Fermosel A, De Miguel-Yanes JM, Duffort-Falcó M, et al. Mortality-related factors after hospitalization for acute exacerbation of chronic obstructive pulmonary disease: the burden of clinical features. Am J Emerg Med. 2007;25(5):515–522. DOI:10.1016/j.ajem.2006.09.014
- Hoogendoorn M, Hoogenveen RT, Rutten-van Mölken MP, et al. Case fatality of COPD exacerbations: a meta-analysis and statistical modelling approach. Eur Respir J. 2011;37(3):508–515. DOI:10.1183/09031936.00043710
- Suissa S, Dell’Aniello S, Ernst P. Long-term natural history of chronic obstructive pulmonary disease: severe exacerbations and mortality. Thorax. 2012;67(11):957–963. DOI:10.1136/thoraxjnl-2011-201518
- Sherman RE, Anderson SA, Dal Pan GJ, et al. Real-world evidence - What is it and what can it tell us? N Engl J Med. 2016;375(23):2293–2297. DOI:10.1056/NEJMsb1609216
- Rabe KF, Hurst JR, Suissa S. Cardiovascular disease and COPD: dangerous liaisons? Eur Respir Rev. 2018;27(149):180057. DOI:10.1183/16000617.0057-2018
- Ikonomidis I, Andreotti F, Economou E, et al. Increased proinflammatory cytokines in patients with chronic stable angina and their reduction by aspirin. Circulation. 1999;100(8):793–798. DOI:10.1161/01.CIR.100.8.793
- Agustí A, Edwards LD, Rennard SI, et al. Persistent systemic inflammation is associated with poor clinical outcomes in COPD: a novel phenotype. PLoS One. 2012;7(5):e37483. DOI:10.1371/journal.pone.0037483
- Frost FJ, Petersen H, Tollestrup K, et al. Influenza and COPD mortality protection as pleiotropic, dose-dependent effects of statins. Chest. 2007;131(4):1006–1012. DOI:10.1378/chest.06-1997