Abstract
The open reading frames of 17 connexins from Syrian hamster (using tissues) and 16 connexins from the Chinese hamster cell line V79, were fully (Cx30, Cx31, Cx37, Cx43 and Cx45) or partially sequenced. We have also detected, and partially sequenced, seven rat connexins that previously were unavailable. The expression of connexin genes was examined in some hamster organs and cultured hamster cells, and compared with wild-type mouse and the cancer-prone Min mouse. Although the expression patterns were similar for most organs and connexins in hamster and mouse, there were also some prominent differences (Cx29 and 30.3 in testis; Cx31.1 and 32 in eye; Cx46 in brain, kidney and testis; Cx47 in kidney). This suggests that some connexins have species-specific expression profiles. In contrast, there were minimal differences in expression profiles between wild type and Min mice. Species-specific expression profiles should be considered in attempts to make animal models of human connexin-associated diseases.
INTRODUCTION
Gap junction channels are found in nearly all tissues of the vertebrate body. Even white blood cells perform gap junctional intercellular communication (GJIC) with other cells (Citation1, Citation2). The chordate gap junction channel proteins are called connexins. The connexin family is presently considered to consist of 20 functional members and three pseudogenes in humans and 19 functional members in mouse (Citation3, Citation4), excluding the potential member, connexin23 (Cx23) (Citation5). Their expression varies between tissues, and one cell may express several connexins. Generally, the gap junction channels allow the diffusion of small (<1000 Da), water-soluble molecules directly from the cytosol of one cell into the cytosol of the adjacent cell. However, there is some permselectivity among the channels depending on the connexin isoform constituting them (Citation6, Citation7).
Connexins and GJIC have been implicated in fundamental cellular and physiological processes like regulation of growth and cell division, differentiation, and embryonic development (Citation8, Citation9). Connexin proteins are targets for several signal transduction pathways, and have thus been found to be direct substrates for an increasing number of kinases (Citation10) and phosphatases (Citation11). This might be one reason why the regulation of connexin functions is often aberrant in major human health problems like cancer and heart and coronary diseases (Citation8, Citation12, Citation13). As the sequence of orthologue connexin proteins vary more or less between species (Citation14), the knowledge of connexin sequences in different species is a valuable part in the dissection of pathways regulating GJIC and connexins. When this work started, the connexin sequences of hamster were unexplored, and even for rat, no information was available for several connexins. We therefore aimed at detecting and sequencing (partially or fully) as many connexins as possible from two hamster species, and the remaining unsequenced connexins in rat.
Considering the role of GJIC and connexins in cellular physiology, it is not surprising that a number of human inheritable diseases are caused by mutations in connexin genes, and some of them are mimicked by connexin knockout mice (Citation15, Citation16). For experimental animals to function as model systems of connexin-associated diseases, one important criterion would be that the connexin in question has similar expression profiles among the organs and tissues in different species. Thus, another aim of the present work was to compare the expression profiles of as many connexins as possible between two species, and we have here selected Syrian hamster and mouse. Furthermore, with the proposed role of GJIC and connexins in cancer development (Citation8, Citation12), it was of great interest to investigate whether predisposition to cancer would cause major changes in connexin expression. For these studies, we selected the cancer-prone Min (multiple intestinal neoplasia) mouse (Citation17), (Citation18), (Citation19), which harbors a heterozygote deletion mutant of the Apc (adenomatous polyposis coli) allele. This allele is strongly implicated in human colon cancer. Moreover, the Apc gene product participates in Wnt signaling (Citation20, Citation21), a pathway that can regulate the expression of Cx43 (Citation22, Citation23).
It is therefore of considerable interest to identify the connexins expressed in any tissue using simple methods available in most laboratories. With the lack of readily available and high specificity antibodies for many of the connexin proteins, RT-PCR is a sensitive and relatively rapid method for first-line screening of expressed mRNAs. Many groups have used PCR to detect the expression of connexins, but they have usually been aimed at the detection of previously sequenced connexins from a specific species, or using strongly degenerated primers to pick up virtually any novel connexin expressed. Our approach was to design primers specific for each connexin isoform, which then could be used in species where the relevant connexin had not been sequenced before, and the detected connexins were partially or fully sequenced. Human, mouse and rat cells or organs were initially used as positive controls for the functionality of the primers. Subsequently, we investigated the expression of all detectable connexins in some organs of hamster, and compared this to wild-type mouse and the cancer-prone Min mouse.
MATERIALS AND METHODS
Animals and Preparation of Tissues
Handling of animals followed national and institutional guidelines. All animals had free access to water and food. Young males of Syrian hamsters were purchased from the Norwegian Institute of Public Health (Oslo, Norway). Male Rowett (nude) rats were from an in-house colony (courtesy of Department of Tumor Biology, The Norwegian Radium Hospital, Oslo, Norway) (Citation24). The animals were killed by CO2, and the organs to be studied were rapidly removed, cut in small pieces (except the eyes), and dropped into liquid nitrogen. The tissue pieces were kept at −70°C until isolation of RNA.
The mice were bred at the Norwegian Institute of Public Health from inbred mice originally purchased from the Jackson Laboratory (Bar Harbor, ME). The Min/+ pedigree was maintained by mating C57BL/6J +/+ (wild-type) females with C57BL/6J Min/+ males, and procedures to secure inbreeding were followed. The Min/+ mice were identified by allele specific PCR on DNA isolated from blood as described previously (Citation25). The mice were killed by cervical dislocation. Tissues were prepared as described above.
Cell Cultures
The V79 Chinese hamster lung fibroblast cell line and early passage Syrian hamster embryo cells were cultured as described previously (Citation26). HeLa cells transfected with a rat Cx43 construct and human kidney K7 cells were made available to us by Dr. E. Rivedal. Cells were rinsed in cold PBS, and scraped into lysis buffer (from a Sigma kit, see below). The lysates were kept at −70°C until isolation of RNA.
Isolation of RNA and Generation of cDNA
The tissue pieces were homogenized in lysis buffer with the help of micropestles (Eppendorf, Hamburg, Germany), except the heart, which was powderized in liquid nitrogen by a porcelain mortar and pestle. All RNAs (cells and tissue pieces) were extracted using the GenElute Mammalian Total RNA Kit (Sigma, St. Louis, MO) or the Strata Prep Total RNA Miniprep Kit (Stratagene; La Jolla, CA) according to the suppliers' recommendations. The RNA preparations were cleared of genomic DNA contamination with DNase I (DNA-free kit; Ambion, Huntingdon, Cambridgeshire, UK). One to two μ g RNA were reverse transcribed in the presence of anchored oligo(dT) using Superscript II (Invitrogen, Paisley, UK). Polymerase chain reaction was thereafter run on the cDNAs (35–75 ng of cDNA per reaction tube) using Taq or Pfu DNA polymerase. The products were then visualized on acrylamide gels after staining with SYBR green (Molecular Probes, Leiden, The Netherlands). A ϕX174 Hae III digest was used as size marker (Sigma).
Primer Design
Two approaches were used for designing primers. We first used a primer construction program (Oligo, MedProbe, Oslo, Norway). We here aligned the reading frame of all isoforms of connexins by ClustalW (http://www.ebi.ac.uk/clustalw/), and designed primers taking into account standard primer restrictions and being isoform specific, i.e., no false priming in other isoforms. The success rate for these primers was considerably less than 50% (i.e., less than half the primers recognized the relevant unsequenced connexins). We therefore proceeded to a manual design of primers. The known sequences of a specific connexin isoform from as many mammals as possible (usually mouse, rat, and human, occasionally dog, pig, cow, and other species) were aligned. The most conserved areas were identified and manually analyzed for the suitability as templates for primers (GC content, lack of potential hairpin structures, etc.). Up to 3–4 degenerations were allowed. Candidates (usually 20–25 bases (b) long) were then submitted to nucleotide BLAST for short nearly exact matches (http://www.ncbi.nlm.nih.gov/BLAST/). If more than 75% of the nucleotides were aligned with nonrelevant mammalian sequences, including other connexins, the sequence was usually rejected from primer synthesis. To obtain the longest possible sequence of the connexins, we aimed at designing some of the primers close to the 5′- and 3′-ends of the reading frames. After obtaining partial sequences of the connexins (see below), more species-specific primers could be designed when needed. More than 75% of the manually designed primers worked successfully, although irrelevant bands were occasionally detected. The primers were synthesized by Eurogentec S.A. (Ougrée, Belgium). In total, approximately 300 primers were synthesized, and their sequences can be obtained from the authors.
RACE
To obtain full-length sequences, rapid amplification of cDNA ends (5′-RACE and 3′-RACE) was performed with the FirstChoice RLM-RACE kit (Ambion) according to the supplier's recommendations.
Sequencing
Using ExoSAP-IT (USB; obtained through Amersham, Buckinghamshire, UK), the PCR and RACE products that were of suitable quality were cleaned from residual single-stranded primers, any extraneous single-stranded DNA and remaining dNTPs that could interfere with the sequencing reaction. The sequencing reactions were performed with the Dyenamic ET Dye Terminator Cycle Sequencing Kit (Amersham) as described by the supplier. The samples were finally separated by capillary electrophoresis and analyzed using a MegaBACE1000 sequencing instrument (Amersham). Both strands were sequenced at least two times from independent PCR or RACE products with consistent results.
Naming Conventions
We follow here the “size” nomenclature, and mention the “Greek” nomenclature only once. When referring to the gene or the mRNA, the names are in italics. To avoid the confusion between different species, we add a two-letter Latin abbreviation in front of the connexin name where needed (Homo sapiens, Hs; Mus musculus, Mm; Rattus norvegicus, Rn; Mesocricetus auratus, Ma; Cricetulus griseus, Cg).
RESULTS
We used connexin sequences found in GenBank or the EMBL databases as a starting point. We have added new sequences when published in the databases, and have ended up with the following set, using mouse nomenclature and with the recently suggested naming revisions (Citation16) in bold: Cx26 [β2], 29 [ε1, δ1], 30 [β6], 30.2 [χ1, α11, δ3], 30.3 [β4], 31 [β3], 31.1 [β5], 32 [β1], 33 [α6], 36 [α9, δ2], 37 [α4], 39 [α4], 40 [α5], 43 [α1], 45 [α7, c1], 46 [α3], 47 [α12, c2], 50 [α8], and 57 [α10]. Please note that a number of inconsistencies can be found in the literature and databases, especially for the “Greek” nomenclature. Very recently, sequences encoding a candidate Cx23 [δ5] were predicted in mouse and humans by sequence database searches (Citation5), but it has not been included in the present work.
At the time we started this work, there were no available sequences from rat (Rattus norvegicus) Cx26, 29, 30.2, 39, 45, 47, 50, and 57 (some of them were published during this study, see below), and we attempted to detect and sequence also these connexins. When available, we aligned the human, mouse and rat orthologues, and occasionally also other species, by the ClustalW program. When in doubt, the closest related sequence in other species was identified by BLAST, limiting the search to the species of interest. In the case of MmCx29, we found the closest human homolog to be Cx31.3, similar to the information given for this connexin in the GenBank/EMBL databases (AF503615). HsCx30.2, which is also suggested as the human orthologue of MmCx29 (Citation3, Citation27), is identical to HsCx31.3 (as determined by the amino acid sequence (Citation27)), but is terminated around 60 b too early. For the time being, we therefore consider HsCx30.2 as an artefactually truncated version of HsCx31.3. HsCx25 (β7; AJ414563) and HsCx58 (α9; AF271261; also called Cx59) were not considered in this work, as database searches revealed no close relatives in rat or mouse, although they can be found in other mammals (Citation4). A novel HsCx gene in AL138688 (complementary strand of bases 67514–68821) was considered identical to HsCx46.
Rat Connexins
Among the rat connexins that had not been sequenced when this work was started, we detected Cx26, 29, 39, 45, 47, 50, and 57, and the products were sequenced ().
TABLE 1 Overview of Rattus norvegicus connexins detected and sequenced, and their accession numbers in GenBank
Cx26: RnCx26 was one of the first connexins sequenced (Citation28), but only the protein sequence (P21994) was available in the databases. On the other hand, the Rattus rattus Cx26 DNA sequence (X51615) was available. The obtained partial open reading frame (ORF) was identical to the published Cx26 from Rattus rattus. It is possible that X51615 is erroneously registered, and may therefore in reality be Rattus norvegicus.
Cx29: The sequence obtained corresponded to positions 142-384 in MmCx29 (AJ297318). Relative to MmCx29, RnCx29 lacked a triplet (positions 325–327 of MmCx29, counting from the starting codon). Also HsCx31.3 lacks a triplet in the same area. Our partial sequence was identical in the overlapping area to the complete RnCx29 sequence (XM_221997) that was published during the course of the present work.
Cx39: The obtained 634 b long sequence was identical to the recently published sequence for RnCx39 (XM_344617) in the overlapping area. RnCx39 lacked the triplets corresponding to amino acid positions 108 and 202 in the mouse sequence.
Cx45: The obtained sequence started at position-1 relative to the starting codon, and lacked 42 b in the 3′-end to complete the reading frame. As judged from the previously published Cx45 sequences from other species, Cx45 is among the most conserved connexins. The present sequence was 90 (dog) to 96% (mouse) identical to other published mammalian sequences.
Cx47: Within our 1228 b sequence, there were differences at five positions compared with the recently published RnCx47 (XM_220511), but none of these caused changes in the amino acid sequence of the protein. These differences might be single nucleotide polymorphisms (SNPs). For unknown reasons, XM_220511 has more recently changed name to “similar to guanylate cyclase,” and the 5′-end totally changed sequence, but from the middle of the second extracellular loop it remained identical to our sequence.
Cx50: A 300 b sequence was obtained for RnCx50. The sequence was identical to the recently published complete ORF of RnCx50 (AB078344), and corresponded to positions 521–820 in this sequence.
Cx57: Our 1273 b sequence differed from the recently published XM_232859 by two bases. These differences caused Q145 (in XP_232859) → H, and A382 → V. It is not known whether these differences are SNPs or sequencing errors. Note that the counting of lacking nucleotides in the 3′-end of the Cx57 ORFs (, and ) is based on the sequence found in Ref. 29. A recent publication indicated that the 3′-end of MmCx57 might possess an intron causing a change in sequence and length of the ORF (Citation30), and similar sequences are found in other mammals (Citation4).
TABLE 2 Overview of Mesocricetus auratus connexins and accession numbers in GenBank
TABLE 3 Overview of Cricetulus griseus connexins and their accession numbers in GenBank
As large scale sequencing of the rat genome is taking place (Citation31), we made no further attempts to obtain the full ORFs of the mentioned rat connexins. The RnCx30.2 sequence was recently published (XM_343965). Thereby, the rat orthologues of all known members in the mouse connexin family have been found except for the potential candidate, Cx23.
Syrian and Chinese Hamster Connexins
No PCR products recognized as a connexin were obtained for Cx33 or Cx36 in either hamster species. These connexins will not be further mentioned below. The overviews of sequenced connexins from Syrian hamster (Mesocricetus auratus) and Chinese hamster (Cricetulus griseus) V79 cells are found in and , respectively.
Cx26: Partial coding sequences were obtained for both species, but for Syrian hamster a part of the 5′-UTR was also included. There were 18 nucleotide differences between the two hamster species in the 354 b overlapping sequence (i.e., 95% identity), but none of them caused changes in the predicted amino acid sequence.
Cx29: A 371 b sequence was obtained from Syrian hamster tissues. Relative to the mouse sequence, a 9b deletion has occurred (between positions 105 and 106) in the present MaCx29 sequence. PCR products were achieved from genomic DNA of V79 cells at the correct sizes, but for unknown reasons, we were not able to sequence these products.
Cx30: The complete ORFs of 786 b were obtained from both species. There were 21 nucleotide differences between the two ORFs (i.e., 97% identity), but none of them caused any changes in the amino acid sequence. Both hamster Cx30 ORFs were 92–93% identical to the mouse ORF, but there were only two amino acid differences (both of them conservative) between the mouse and hamster proteins.
Cx30.2: Partial sequences were obtained from both systems. There were six nucleotide differences between the two species within the 304 b common sequence (98% identity), and one of the differences caused a (conservative) change in the predicted amino acid sequence.
Cx30.3: Long 5′-UTR sequences were obtained from both Syrian hamster tissues and from V79 cells, in addition to the major parts of the ORFs. In the 622 b overlapping within the two ORFs, there were 28 nucleotide differences (95% identity), causing three (conservative) differences in the predicted amino acid sequences.
Cx31: The full-length ORF of 813 b was obtained from Syrian hamster tissues, while the sequence from V79 cells was formally lacking the initiation codon to achieve complete ORF because the upstream primer included the initiation codon. A possible SNP was detected at position 492 (C or A) of the V79 sequence, but both resulted in alanine. MaCx31 had a C in the corresponding position. There was a 6-base deletion in CgCx31, corresponding to positions 763–768 in the ORF of MaCx31. Otherwise, there were 45 nucleotide differences between the two hamster species (94% identity). The Syrian hamster ORF was 91% identical to rat, 90% identical to mouse, and 84% identical to human Cx31. The predicted amino acid sequences from the two hamster species had 14 differences plus the two amino acid gap, bringing the identities of the hamster Cx31 proteins down to 94%.
Cx31.1: The major parts of the ORFs were obtained from both systems. There were 34 nucleotide differences (95% identity) in the ORF sequences resulting in 13 predicted amino acid differences (i.e., 95% identity within the overlapping 248 amino acid long sequence). In position 595 of the V79 sequence, we always obtained peaks both for G (usually the highest peak, and therefore the reported base) and A. Thus, this was likely a SNP. The position corresponded to first position in codon 222 of the mouse sequence. G results in alanine, while A results in threonine. There was a C in the corresponding position of MaCx31.1, resulting in proline in the protein. The surrounding area is one of the most variable in the Cx31.1 protein when comparing hamster, mouse and human sequences.
Cx32: The major part of the ORF was obtained from V79 cells, while a shorter sequence was achieved from Syrian hamster tissues. In the overlapping 527 nucleotides, there were 15 differences (97% identity), causing one (conservative) amino acid difference (within the 175 amino acid overlapping sequence, i.e., 99% identity).
Cx37: The complete ORF was obtained from V79 cells, while the Syrian hamster sequence formally lacked the initiation codon to complete the ORF, again because it was included in the upstream primer. The MaCx37 and CgCx37 ORFs had 51 nucleotide differences (95% identity), and the MaCx37 ORF was 92, 91, and 86% identical to those of mouse, rat, and human, respectively. There were four amino acid differences in the predicted protein sequences of Cx37 from the two hamster species, giving 99% identity.
A partial MaCx37 sequence (AF508035) was published during the course of this work. This sequence is fully contained within the present sequence, and they are identical except for a lacking base in the fifth last position of AF508035.
Cx39: Partial ORF sequences were obtained from both Syrian hamster tissues and V79 cells. The sequences lacked the triplets corresponding to MmCx39 amino acid positions 108, 201, and 202. The rat is also lacking the former and the latter, but not the middle triplet. In the 640 overlapping bases of the hamster sequences, there were 37 differences, i.e., 94% identity. For the corresponding 213 amino acids, there were 15 differences, giving 93% identity.
Cx40: The major parts of the Syrian and Chinese hamster ORFs were obtained. There were 44 nucleotide differences in the overlapping 936 b sequences, giving 95% identity. For the predicted 311 overlapping amino acids, there were 15 differences, also giving 95% identity.
A recently published 611 b long partial ORF of MaCx40 (AF508036) is entirely contained within the area we have covered. There were two differences between the sequences (437C → T and 629T → C), but none of them caused changes in the predicted amino acid sequence. The differences may be due to SNPs.
Cx43: Cx43 is one of the most commonly expressed connexin proteins in tissues and in cell cultures. We obtained the full-length coding sequences from both hamster species. There were 42 nucleotide differences between the ORFs of MaCx43 and CgCx43 (i.e., 96% identity). The predicted Cx43 proteins of 382 amino acids differed in two positions (one conservative change), giving 99.5% identity. The ORF of MaCx43 was 92% identical to those of mouse and rat, and 88% identical to human Cx43.
Partial MaCx43 (AF508037; the entry is called Cx47) and CgCx43 (AY049064) sequences were recently published. AF508037 is identical to our MaCx43 sequence in the overlapping area, while two of the last five bases of AY049064 differ from our CgCx43 sequence, causing two amino acid changes (277L → P and 278S → T). We note that the predicted amino acid sequences in this area are identical in mouse, rat, human and Syrian hamster Cx43 (271-SSPTAP LSPMSPP-283). We consider it probable that also Chinese hamster will have an 14 identical amino acid sequence in this area. We therefore believe it is likely that the two mentioned bases of AY049064 are incorrect.
Cx45: The full ORFs (1191 b) and parts of the 5′- and 3′-UTRs were obtained both from Syrian hamster tissues and V79 cells. There were 24 differences between the ORFs of the two hamster species (i.e., 98% identity). None of the nucleotide differences caused changes in the predicted protein (i.e., the amino acid sequences were 100% identical). The recently published 396 b long partial ORF of MaCx45 (AF508038) is entirely contained within the area we have covered, and the sequences are fully identical. The ORF of MaCx45 was 96 and 92% identical to those of mouse and humans, respectively. Additionally, we were able to obtain products that extended the sequence of MaCx45 into both the 5′-UTR (240 b) and the 3′-UTR (107 b). The 240 b 5′-UTR is partly corresponding to the 5′-UTR of MmCx45, in that the sequence 142–213 lines up with mouse exon 2 (Citation32). However, positions 1–111 lined up with a mouse genomic sequence approximately 16.6 kb upstream of mouse exon 1. Whether this means that there is an unidentified mouse exon in this area, or the hamster gene is differently organized, is not known.
Cx46: The major parts of the ORFs were obtained from both Syrian hamster tissues and V79 cells. There were 47 nucleotide differences in the overlapping area (96% identity), causing 13 amino acid differences (within 375 amino acids) in the predicted protein sequences (96% identity).
Cx47: The major parts of the coding sequences were obtained from both systems. There were 48 differences in the overlapping nucleotides (96% identity), causing four amino acid differences in the predicted 378 amino acid long overlap (99% identity).
Cx50: The major parts of the ORFs were obtained from both systems. Aligning the two hamster sequences showed that both lacked two triplets relative to the mouse or rat sequences, but the triplets were located at different places in the part of the sequences that encode for the C-terminal tails of the proteins. Relative to the mouse sequence, MaCx50 lacked the triplets corresponding to amino acids 358 and 392, and CgCx50 lacked the triplets corresponding to amino acids 351–352. Otherwise, there were 65 differences in the 1169 b long overlapping sequences from the two hamster species (94% identity), causing 19 amino acid differences and two lacking amino acids in the corresponding 389 amino acid long sequences (95% identity).
Cx57: The major part of the ORF was obtained from V79 cells, while a shorter sequence was achieved from Syrian hamster tissues. Relative to the V79 sequence, the Syrian hamster lacked a nucleotide triplet between positions 721–722. Otherwise, there were 37 nucleotide differences in the overlapping area (96% identity), causing 11 amino acid differences and one gap in the overlapping 300 amino acids (96% identity).
Expression of Connexin mRNAs in Hamster Cells and Organs, and a Comparison with Wild-Type Mouse
The expression of mRNA may be evaluated by several methods, each having advantages and drawbacks. We wanted to achieve a rough impression of the expression of the different connexins in different organs and cells. Preferably, the method should be easy to apply to other species with only minor modifications. Microarray systems are expensive and aimed at high throughput, rather than studying a selected number of gene products. Northern blots require the generation of labeled probes for each connexin. RT-PCR is relatively rapid and sensitive, but requires RNA that is free of genomic contamination (because of the intronless reading frame in most connexin genes). We settled for RT-PCR in these experiments, using selected primers () from the sequencing work described above. The results are summarized in (hamster) and 6 (wild-type mouse in left half of table). Note that the expression in the heart was only examined in hamster, and not in mouse.
TABLE 4 Primer-combinations for screening of connexin expression in Syrian hamster (Mesocricetus auratus) organs and cells, V79 cells (Chinese hamster, Cricetulus griseus) and mouse (Mus musculus, Mm) organs
TABLE 5 Expression of connexins in some Syrian hamster organs, SHE cells and V79 cells as detected by RT-PCR
Cx26 was widely expressed in many tissues in hamster and mouse, as could be expected from previous observations (reviewed in 33). It was not expressed in SHE or V79 cells.
Cx29 has previously been indicated as a rather neurospecific connexin (Citation27, Citation34), and we found it expressed in the brain in both species (). However, it was surprising to find a relatively prominent expression in hamster testis, but not in mouse testis (). Cx29 may also be expressed in low or trace amounts in the hamster eye, liver, and heart and in SHE cells, while in the mouse it was present in eye and liver. The presence of Cx29 in the eyes may partly be explained by contaminating neurons. Interestingly, the human orthologue, HsCx31.3, was also found expressed in liver and heart (Citation14). Cx29 is also expressed at low level in the mouse intestine (Citation35) and in a colon epithelial cell line (Citation36).
Figure 1 Similarities and differences in connexin expression in hamster and mouse. Expression of (A) Cx29, (B) Cx39, (C) Cx46, and (D) Cx57 in some hamster organs and cells (left panels) and in some mouse organs (right panels). RNA was isolated from several Syrian hamster and mouse organs, Syrian hamster embryo (SHE) cells, and Chinese hamster V79 cells. The RNA was DNase treated. After removal of DNase, the samples were divided in two. One part was reverse transcribed, the other part was handled similarly but without reverse transcriptase. PCR was then performed on both samples. The upper panels show samples that were reverse transcribed, and the lower panels show the corresponding samples that were not reverse transcribed, indicating the lack of genomic contamination. The positive controls (pos.) are samples that have not been cleaned from genomic contamination. The positions of size markers are indicated to the left.
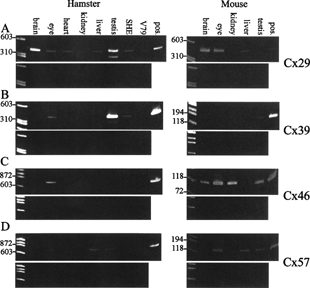
Cx30 was found expressed in high amounts in brain in both species, consistent with previous results (Citation37). The presence in the eye may be caused by the presence of (contaminating) neurons. There was also a relatively evident presence of Cx30 in the hamster and mouse testis and in mouse kidney, but not in SHE or V79 cells.
Cx30.2 was clearly expressed in hamster brain, SHE cells, and V79 cells. For the other hamster organs, it was difficult to obtain samples free of genomic contamination in spite of DNase treatment. In mouse, we detected its expression in all examined tissues. The human orthologue, HsCx31.9, was also detected in several organs, but was most strongly expressed in the heart and pancreas (Citation38).
Cx30.3 had its highest expression in the hamster eye, followed by kidney and testis. It was expressed in both SHE and V79 cells. In the mouse, it was highly expressed in the eye and kidney. It was not found expressed in the mouse testis. The presence of Cx30.3 in kidney and skin has previously been indicated (Citation39, Citation40).
Cx31 was expressed in the hamster and mouse eye, kidney and testis, although the relative amount differed somewhat between the two species. It was also expressed in the hamster brain and in SHE and V79 cells. Among the present organs, Cx31 was previously found expressed only in the rat eye (Citation41).
Cx31.1 may be expressed in trace amounts in hamster kidney, but was clearly expressed in SHE and V79 cells. In the mouse, Cx31.1 was previously found mainly expressed in skin (Citation39), but also in kidney (Citation40). Here we found the expression of Cx31.1 in mouse eye and kidney.
Cx32 was expressed in all tissues except heart in hamster, while in the mouse, Cx32 was not found in eye and testis. It was not expressed in SHE or V79 cells.
Cx33 was not detected in hamster, and its expression could therefore be examined in mouse only. It was previously detected in testis (Citation42) and brain (Citation43) similar to the present results, but we also found a low expression in the kidney, and with potential trace amounts in the eye, perhaps due to contaminating neuronal tissue.
Cx36 was previously detected in brain (Citation44), eye (Citation45) and pancreas (Citation46). We here confirm its expression in the mouse brain and eye.
Cx37 was widely expressed in all examined organs and cells. It has previously been found expressed in a range of tissues (Citation47).
Cx39 was expressed in the testis of both species, with potential trace amounts in the hamster eye () and (in one sample) kidney (not shown). It was weakly expressed in SHE cells. In contrast, Söhl et al. (Citation14) did not find MmCx39 in any of the eight tissues examined, which did not include testis or eye, while the human orthologue, HsCx40.1, was detected in several tissues.
Cx40 was widely expressed in all the examined hamster and mouse organs, and it was also found in V79 cells. It was previously found expressed in a number of tissues (Citation48, Citation49).
Cx43 is one of the most commonly expressed connexins, and was found in all organs examined, and in both cell systems.
Cx45 was expressed in all organs examined, consistent with a relatively broad expression range (Citation33). It was also expressed in SHE cells, but not in V79 cells.
Cx46 was expressed in hamster and mouse eye (). In the mouse, Cx46 was also found in brain, kidney and testis, while it was absent from these organs in hamster (). It was previously detected in the rat lens, heart, and kidney (Citation50).
Cx47 has previously been detected in the brain of the mouse (Citation51), which is confirmed here. Its expression in the hamster brain is more uncertain. On the other hand, Cx47 was expressed in the hamster, but not mouse, kidney.
Cx50 was previously detected in the mouse eye, but not in other tissues (Citation52). We found it expressed in the hamster and mouse eye and testis, and at low or potential trace amounts in brain. There was also a low expression in the mouse kidney. It was expressed in V79 cells, but not in SHE cells.
Cx57 was previously detected in several tissues in the mouse (Citation29), but in more recent work the expression was strictly limited to the horizontal cells of mouse retina (Citation30). Using RT-PCR, we found it expressed in hamster and mouse eye, but also in liver and testis of both species (). The human orthologue, HsCx62, has a relatively restricted expression pattern, and was found only weakly expressed in the heart and (curiously) strongly expressed in skeletal muscle (Citation14).
Expression of Connexin mRNAs in Min Mouse
Only minor differences in expression were found between wild-type mouse and Min mouse (, Min mouse in right half of table). As we have not used quantitative PCR methods, it is not possible to consider such differences as significant. Thus, in the presently studied organs the differences in connexin expression profiles between Min and wild-type mice must be regarded as small. Data for different parts of the intestine are presented elsewhere (Citation35).
TABLE 6 Connexin expression profiles in wild-type and Min mouse as detected by RT-PCR
DISCUSSION
This work had three main aims. First, the detection of as many connexin genes/cDNAs as possible. Second, the partial or complete sequencing of the previously unsequenced connexins, focusing on Syrian hamster tissues, the Chinese hamster lung fibroblast cell line V79, and the unsequenced connexins of rat. Third, the study of expression of connexins in some organs from hamster and hamster cell cultures, and the comparison of the expression profiles of hamster to those of wild type and Min mouse.
To achieve the first and second aims, we designed some of the primers to be located as close as possible to the 5′- and 3′-ends of the reading frames, taking the advantage of previously sequenced connexins from other species. The 5′-ends are much conserved among the different isoforms of the connexins, while the 3′-ends often display a considerable diversity. Thus, a primer close to the 5′-end will often recognize several connexin genes/cDNAs, while a primer close to the 3′-end often will not recognize the relevant connexin in another species. Even in relatively closely related species like Syrian and Chinese hamster, we found some primers that worked well in one species, but not in the other. If we later were able to sequence through the area covered by the primer, we often found a single base difference between the two hamster species.
In spite of using only one cell line from Chinese hamster, the V79 lung fibroblasts, we were able to detect 17 connexins, but sequences were obtained from only 16 (Cx29 being unobtainable). Among these, the full length coding sequences were obtained for 5—Cx30, Cx31 (formally lacking the starting codon), Cx37, Cx43, Cx45—and the major parts of the coding sequences (fewer than 100 bases lacking to complete the ORF) were obtained for four connexins (Cx30.3, Cx31.1, Cx32, Cx47). This compares favorably with tissues of Syrian hamster where we detected 17 connexins, and obtained five full length coding sequences—Cx30, Cx31, Cx37 (formally lacking the starting codon), Cx43, Cx45—and the major parts of the coding sequences (less than 103 bases lacking to complete the ORF) were obtained for four connexins (Cx31.1, Cx40, Cx46, Cx50). A partial Cx29 sequence was obtained from Syrian hamster tissues only. We were not able to detect Cx33 and Cx36 in either system. The latter is among the conserved connexins, and it is highly unlikely that hamsters are devoid of it. It is also likely that Cx33 should be present. Until recently Cx33 was identified in mouse and rat only. It now appears that Cx33 in fact has an orthologue in other mammals (Citation4), and it may be a functional gene in the dog, but a pseudogene in man, chimpanzee and cow.
Cx30.2 presented particular problems due to its very high GC content (70.3% in mouse), making any genetic work, like PCR, more difficult. There are few areas in Cx30.2 where reasonable primer sequences can be put, but still, by carefully titrating the amount of free Mg2+ for the PCR reaction (by the addition of EDTA) sequenceable products were obtained. In fact, it was easier to achieve good Cx30.2 PCR products from the genomic DNA (contaminated samples) than from highly purified RNA, making it more difficult to study the expression in cells and tissues. In spite of our problems to get an expression profile of MaCx30.2, it appears to be one of the most commonly expressed connexins at the mRNA level in mice and humans (present results; 38, 53), but it has been little studied. HsCx31.9 has already been shown to possess unique functional properties among the connexin proteins (Citation38, Citation53), but whether this extends to the rodent orthologue is presently unknown. Further molecular, physiological and electrophysiological studies of this connexin seem warranted.
It was unexpected to find that the immortal cell line V79 expresses eight connexins (Cx30.2, Cx30.3, Cx31, Cx31.1, Cx37, Cx40, Cx43, Cx50) as mRNA in easily detectable amounts. The early passage SHE cells, which have a considerable heterogeneity, express only six connexins at relatively abundant levels (Cx30.2, Cx30.3, Cx31, Cx31.1, Cx43, Cx45), while another three are expressed in more limited amounts (Cx37, Cx39, Cx40). Although the expression at protein level has only been investigated for Cx43 in the two cell systems (Citation26, Citation54), it is likely that some of the other connexin mRNAs are translated, giving the possibility of extensive interactions between connexin proteins.
The connexin expression profiles revealed some evident differences between mouse and hamster organs:
Cx29 and Cx30.3 are present in hamster testis, but not in mouse testis.
Cx31.1 is present in mouse eye, but not in hamster eye. As Cx31.1 is not found in brain in any of the two species, it is unlikely that this difference is caused by contaminating neurons.
In contrast to Cx31.1, Cx32 is found in the hamster eye, but not in mouse eye. As Cx32 is expressed in the brain of both species, we cannot fully exclude the possibility of contaminating neurons for the hamster eye, but we would then have expected a relatively weak expression in the hamster eye, and clearly weaker than that of the brain. On the contrary, the expression in the eye was as strong as in the brain. In contrast, the weak expression of Cx32 in the eye of the Min mouse could be due to neuronal contamination.
Cx46 is absent from hamster brain, kidney, and testis, but present in the corresponding organs of mouse.
Cx47 is present in hamster kidney, but absent from mouse kidney.
It might be a coincidence, but we note that in most cases the “loss” of one or two connexins is compensated by the appearance of another connexin in the same organ. Thus, the “loss” of Cx32 in the mouse eye is compensated by the appearance of Cx31.1, the “loss” of Cx47 in mouse kidney is compensated by the appearance of Cx46, and the “loss” of Cx29 and Cx30.3 in mouse testis is compensated by the appearance of Cx46. As yet, we do not have any explanation for these deviating expression profiles. Söhl et al. (Citation14) found that MmCx29/HsCx31.3, MmCx39/HsCx40.1, and MmCx57/HsCx62 have different expression profiles in humans and mice. These connexins are among the less conserved, with 66%, 56%, and 78% identities at amino acid level in human-mouse comparisons (Citation14). Also Cx31.1 and Cx46 belong to the less conserved group of connexins with 78% and 77% identities at the amino acid level for the mouse and human orthologues, while Cx47 is moderately conserved with 85% identity, and Cx32 is strongly conserved with 99% identity (Citation14). Thus, species-specific differences in expression profiles may be more common among the less conserved connexins. The potential discrepancies in connexin expression may be more firmly settled in a larger comparative study using several species and several methods for the detection of expression.
In contrast to the prominent, but few, differences in connexin expression between hamster and mouse as described above, the differences between wild-type mouse and Min mouse were small. This suggests that prominent differences between hamster and wild-type mouse are not likely to be caused by accidental experimental variations. This further indicates that the Min genotype does not strongly affect connexin gene expression pattern, and that one wild-type Apc allele is able to produce sufficient amounts of Apc protein to maintain the normal regulation of Cx43, at least at the scale of the organs. This does not exclude the possibility of aberrant regulation in specific tissues or cells, and certainly not in tumors that have lost the remaining functional Apc allele.
The species-specific expression of connexins in certain organs has several implications, of which the most important concerns human diseases. There are a number of human pathological conditions that have been causally associated with mutations in connexin genes (Citation15), the last published being Pelizaeus-Merzbacher-like disease linked with mutations in HsCx47 (Citation55). Some of the connexin-linked pathological conditions are mimicked by mouse connexin knockouts (Citation16). This may partly also be the case for the hypomyelinating Pelizaeus-Merzbacher-like disease, as Cx47-deficient mice display myelination abnormalities in the central nervous system, but otherwise did not show any obvious morphological or behavioral defects (Citation51), in contrast to the strong motoric deficiencies in humans (Citation55). The varying degree of identities among the mouse-human orthologues (Citation14) and the different expression profiles described above, could suggest that the more conserved connexins are under a stricter selection pressure by their physiological tasks than are the less conserved connexins. Thus, there might be major difficulties in establishing animal models for some human connexin-associated diseases. First, the parameters expression profile, physiological tasks, or gating properties may be significantly different between humans and experimental animals for certain connexin proteins. Second, the property of heterooligomerization may cause a mutated connexin to affect the functions of another (nonmutated) connexin, e.g., during the heterooligomerization of a connexin showing species-specific expression profile with another, more conserved, connexin. This property may be of particular importance in those cases where a disease-causing mutation generates a dominant-negative connexin version (Citation56, Citation57). In both cases, an animal knockout model should not be expected to mimic the human symptoms.
In summary, the present results confirm that there are organ- and cell-specific expression patterns with some connexins very commonly expressed (Cx26, Cx37, Cx40, Cx43, Cx45), and that other connexins show more restricted expression profiles (Cx31.1, Cx39, Cx47). The results clearly indicate that some connexins show species-specific expression patterns in certain organs. This will be important to consider in attempts of generating animal models for human connexin-associated diseases.
ACKNOWLEDGMENTS
Dr. B. Smith-Sørensen is gratefully thanked for advice on PCR. Dr. E. Rivedal is thanked for making three pairs of primers and two human cell culture systems available to us. Prof. T. Sanner is thanked for support and valuable comments. V.C. was partly supported by the Research Council of Norway, and is now financed by the Norwegian Cancer Society. This work was supported by the Norwegian Cancer Society and The Family Blix' Fund.
REFERENCES
- Jara P I, Boric M P, Sáez J C. Leukocytes express connexin 43 after activation with lipopolysaccharide and appear to form gap junctions with endothelial cells after ischemia-reperfusion. Proc Natl Acad Sci USA 1995; 92: 7011–7015, [PUBMED], [INFOTRIEVE], [CSA]
- Krenacs T, Van Dartel M, Lindhout E, Rosendaal M. Direct cell/cell communication in the lymphoid germinal center: Connexin43 gap junctions functionally couple follicular dendritic cells to each other and to B lymphocytes. Eur J Immunol 1997; 27: 1489–1497, [PUBMED], [INFOTRIEVE], [CSA]
- Willecke K, Eiberger J, Degen J, Eckardt D, Romualdi A, Güldenagel M, Deutsch U, Söhl G. Structural and functional diversity of connexin genes in the mouse and human genome. Biol Chem 2002; 383: 725–737, [PUBMED], [INFOTRIEVE], [CSA], [CROSSREF]
- Cruciani V, Mikalsen S -O. The connexin gene family in mammals. Biol Chem 2005; 386: 325–332, [PUBMED], [INFOTRIEVE], [CSA], [CROSSREF]
- Söhl G, Willecke K. Gap junctions and the connexin protein family. Cardiovasc Res 2004; 62: 228–232, [CSA], [CROSSREF]
- Veenstra R D. Size and selectivity of gap junction channels formed from different connexins. J Bioenerg Biomembr 1996; 28: 327–337, [PUBMED], [INFOTRIEVE], [CSA], [CROSSREF]
- Goldberg G S, Moreno A P, Lampe P D. Gap junctions between cells expressing connexin 43 or 32 show inverse permselectivity to adenosine and ATP. J Biol Chem 2002; 277: 36725–36730, [PUBMED], [INFOTRIEVE], [CSA], [CROSSREF]
- Loewenstein W R. Junctional intercellular communication and the control of growth. Biochim Biophys Acta 1979; 560: 1–65, [PUBMED], [INFOTRIEVE], [CSA]
- Lo C W. The role of gap junction membrane channels in development. J Bioenerg Biomembr 1996; 28: 379–385, [PUBMED], [INFOTRIEVE], [CSA], [CROSSREF]
- Cruciani V, Mikalsen S -O. Connexins, gap junctional intercellular communication and kinases. Biol Cell 2002; 94: 433–443, [PUBMED], [INFOTRIEVE], [CSA], [CROSSREF]
- Hervé J C, Sarrouilhe D. Modulation of junctional communication by phosphorylation: Protein phosphatases, the missing link in the chain. Biol Cell 2002; 94: 423–432, [CSA], [CROSSREF]
- Mesnil M. Connexins and cancer. Biol Cell 2002; 94: 493–500, [PUBMED], [INFOTRIEVE], [CSA], [CROSSREF]
- Severs N J. Gap junction remodeling in heart failure. J Card Fail 2002; 8: S293–S299, [PUBMED], [INFOTRIEVE], [CSA], [CROSSREF]
- Söhl G, Nielsen P A, Eiberger J, Willecke K. Expression profiles of the novel human connexin genes hCx30.2, hCx40.1, and hCx62 differ from their putative mouse orthologues. Cell Commun Adhes 2003; 10: 27–36, [CSA], [CROSSREF]
- Kelsell D P, Dunlop J, Hodgins M B. Human diseases: Clues to cracking the connexin code?. Trends Cell Biol 2001; 11: 2–6, [PUBMED], [INFOTRIEVE], [CSA], [CROSSREF]
- Söhl G, Willecke K. An update on connexin genes and their nomenclature in mouse and man. Cell Commun Adhes 2003; 10: 173–180, [CSA]
- Moser A R, Dove W F, Roth K A, Gordon J I. The Min (multiple intestinal neoplasia) mutation: Its effect on gut epithelial cell differentiation and interaction with a modifier system. J Cell Biol 1992; 116: 1517–1526, [PUBMED], [INFOTRIEVE], [CSA], [CROSSREF]
- Luongo C, Moser A R, Gledhill S, Dove W F. Loss of Apc+ in intestinal adenomas from Min mice. Cancer Res 1994; 54: 5947–5952, [PUBMED], [INFOTRIEVE], [CSA]
- Shoemaker A R, Gould K A, Luongo C, Moser A R, Dove W F. Studies of neoplasia in the Min mouse. Biochim Biophys Acta 1997; 1332: F25–F48, [PUBMED], [INFOTRIEVE], [CSA]
- Giles R H, van Es J H, Clevers H. Caught up in a Wnt storm: Wnt signaling in cancer. Biochim Biophys Acta 2003; 1653: 1–24, [PUBMED], [INFOTRIEVE], [CSA]
- Fodde R. The APC gene in colorectal cancer. Eur J Cancer 2002; 38: 867–871, [PUBMED], [INFOTRIEVE], [CSA], [CROSSREF]
- Van der Heyden M AG, Rook M B, Hermans M MP, Rijksen G, Boonstra J, Defize L HK, Destrée O HJ. Identification of connexin43 as a functional target for Wnt signalling. J Cell Sci 1998; 111: 1741–1749, [PUBMED], [INFOTRIEVE], [CSA]
- Ai Z W, Fischer A, Spray D C, Brown A MC, Fishman G I. Wnt-1 regulation of connexin43 in cardiac myocytes. J Clin Invest 2000; 105: 161–171, [PUBMED], [INFOTRIEVE], [CSA]
- Engebraaten O, Fodstad Ø. Site-specific experimental metastasis patterns of two human breast cancer cell lines in nude rats. Int J Cancer 1999; 82: 219–225, [PUBMED], [INFOTRIEVE], [CSA], [CROSSREF]
- Steffensen I -L, Paulsen J E, Eide T J, Alexander J. 2-Amino-1-methyl-6-phenylimidazo[4,5-b]pyridine increases the numbers of tumors, cystic crypts and aberrant crypt foci in multiple intestinal neoplasia mice. Carcinogenesis 1997; 18: 1049–1054, [PUBMED], [INFOTRIEVE], [CSA], [CROSSREF]
- Cruciani V, Mikalsen S -O, Vasseur P, Sanner T. Effects of peroxisome proliferators and 12-O-tetradecanoyl phorbol-13-acetate on intercellular communication and connexin43 in two hamster fibroblast systems. Int J Cancer 1997; 73: 240–248, [PUBMED], [INFOTRIEVE], [CSA], [CROSSREF]
- Söhl G, Eiberger J, Jung Y T, Kozak C A, Willecke K. The mouse gap junction gene connexin29 is highly expressed in sciatic nerve and regulated during brain development. Biol Chem 2001; 382: 973–978, [CSA], [CROSSREF]
- Zhang J -T, Nicholson B J. Sequence and tissue distribution of a second protein of hepatic gap junctions, Cx26, as deduced from its cDNA. J Cell Biol 1989; 109: 3391–3401, [PUBMED], [INFOTRIEVE], [CSA], [CROSSREF]
- Manthey D, Bukauskas F, Lee C G, Kozak C A, Willecke K. Molecular cloning and functional expression of the mouse gap junction gene connexin-57 in human HeLa cells. J Biol Chem 1999; 274: 14716–14723, [PUBMED], [INFOTRIEVE], [CSA], [CROSSREF]
- Hombach S, Janssen-Bienhold U, Söhl G, Schubert T, Büssow H, Ott T, Weiler R, Willecke K. Functional expression of connexin57 in horizontal cells of the mouse retina. Eur J Neurosci 2004; 19: 2633–2640, [PUBMED], [INFOTRIEVE], [CSA], [CROSSREF]
- Rat Genome Sequencing Project Consortium. Genome sequence of the Brown Norway rat yields insights into mammalian evolution. Nature 2004; 428: 493–521, [CSA], [CROSSREF]
- Jacob A, Beyer E C. Mouse connexin 45: Genomic cloning and exon usage. DNA Cell Biol 2001; 20: 11–19, [PUBMED], [INFOTRIEVE], [CSA], [CROSSREF]
- Bruzzone R, White T W, Paul D L. Connections with connexins: The molecular basis of direct intercellular signaling. Eur J Biochem 1996; 238: 1–27, [PUBMED], [INFOTRIEVE], [CSA], [CROSSREF]
- Altevogt B M, Kleopa K A, Postma F R, Scherer S S, Paul D L. Connexin29 is uniquely distributed within myelinating glial cells of the central and peripheral nervous systems. J Neurosci 2002; 22: 6458–6470, [PUBMED], [INFOTRIEVE], [CSA]
- Husøy T, Knutsen H K, Cruciani V, Ølstørn H B, Mikalsen S O, Løberg E M, Alexander J. Connexin43 is overexpressed in ApcMin/+-mice adenomas and colocalises with COX-2 in myofibroblasts. Int J Cancer 2005; 116: 325–332, [CSA]
- Husøy T, Cruciani V, Knutsen H K, Mikalsen S -O, Ølstørn H B, Alexander J. Cells heterozygous for the ApcMin-mutation have decreased gap junctional intercellular communication and connexin43 level, and reduced microtubule polymerization. Carcinogenesis 2003; 24: 643–650, [CSA], [CROSSREF]
- Dahl E, Manthey D, Chen Y, Schwarz H -J, Chang Y S, Lalley P A, Nicholson B J, Willecke K. Molecular cloning and functional expression of mouse connexin-30, a gap junction gene highly expressed in adult brain and skin. J Biol Chem 1996; 271: 17903–17910, [PUBMED], [INFOTRIEVE], [CSA], [CROSSREF]
- Nielsen P A, Beahm D L, Giepmans B NG, Baruch A, Hall J E, Kumar N M. Molecular cloning, functional expression, and tissue distribution of a novel human gap junction forming protein, connexin-31.9. J Biol Chem 2002; 277: 38272–38283, [PUBMED], [INFOTRIEVE], [CSA], [CROSSREF]
- Hennemann H, Dahl E, White J B, Schwarz H -J, Lalley P A, Chang S, Nicholson B J, Willecke K. Two gap junction genes, connexin 31.1 and 30.3, are closely linked on mouse chromosome 4 and preferentially expressed in skin. J Biol Chem 1992; 267: 17225–17233, [PUBMED], [INFOTRIEVE], [CSA]
- Tucker M A, Barajas L. Rat connexins 30.3 and 31 are expressed in the kidney. Exp Cell Res 1994; 213: 224–230, [PUBMED], [INFOTRIEVE], [CSA], [CROSSREF]
- Hoh J H, John S A, Revel J -P. Molecular cloning and characterization of a new member of the gap junction gene family, connexin-31. J Biol Chem 1991; 266: 6524–6531, [PUBMED], [INFOTRIEVE], [CSA]
- Haefliger J -A, Bruzzone R, Jenkins N A, Gilbert D J, Copeland N G, Paul D L. Four novel members of the connexin family of gap junction proteins. Molecular cloning, expression, and chromosome mapping. J Biol Chem 1992; 267: 2057–2064, [PUBMED], [INFOTRIEVE], [CSA]
- Rozental R, Morales M, Mehler M F, Urban M, Kremer M, Dermietzel R, Kessler J A, Spray D C. Changes in the properties of gap junctions during neuronal differentiation of hippocampal progenitor cells. J Neurosci 1998; 18: 1753–1762, [PUBMED], [INFOTRIEVE], [CSA]
- Söhl G, Degen J, Teubner B, Willecke K. The murine gap junction gene connexin36 is highly expressed in mouse retina and regulated during brain development. FEBS Lett 1998; 428: 27–31, [CSA], [CROSSREF]
- Al-Ubaidi M R, White T W, Ripps H, Poras I, Avner P, Gomès D, Bruzzone R. Functional properties, developmental regulation, and chromosomal localization of murine connexin36, a gap-junctional protein expressed preferentially in retina and brain. J Neurosci Res 2000; 59: 813–826, [PUBMED], [INFOTRIEVE], [CSA], [CROSSREF]
- Serre-Beinier V, Le Gurun S, Belluardo N, Trovato-Salinaro A, Charollais A, Haefliger J -A, Condorelli D F, Meda P. Cx36 preferentially connects β-cells within pancreatic islets. Diabetes 2000; 49: 727–734, [PUBMED], [INFOTRIEVE], [CSA]
- Willecke K, Heynkes R, Dahl E, Stutenkemper R, Hennemann H, Jungbluth S, Suchyna T, Nicholson B J. Mouse connexin37: Cloning and functional expression of a gap junction gene highly expressed in lung. J Cell Biol 1991; 114: 1049–1057, [PUBMED], [INFOTRIEVE], [CSA], [CROSSREF]
- Beyer E C, Reed K E, Westphale E M, Kanter H L, Larson D M. Molecular cloning and expression of rat connexin40, a gap junction protein expressed in vascular smooth muscle. J Membr Biol 1992; 127: 69–76, [PUBMED], [INFOTRIEVE], [CSA]
- Hennemann H, Suchyna T, Lichtenberg-Fraté H, Jungbluth S, Dahl E, Schwarz J, Nicholson B J, Willecke K. Molecular cloning and functional expression of mouse connexin40, a second gap junction gene preferentially expressed in lung. J Cell Biol 1992; 117: 1299–1310, [PUBMED], [INFOTRIEVE], [CSA], [CROSSREF]
- Paul D L, Ebihara L, Takemoto L J, Swenson K I, Goodenough D A. Connexin46, a novel lens gap junction protein, induces voltage-gated currents in nonjunctional plasma membrane of Xenopus oocytes. J Cell Biol 1991; 115: 1077–1089, [PUBMED], [INFOTRIEVE], [CSA], [CROSSREF]
- Odermatt B, Wellershaus K, Wallraff A, Seifert G, Degen J, Euwens C, Fuss B, Bussow H, Schilling K, Steinhauser C, Willecke K. Connexin 47 (Cx47)-deficient mice with enhanced green fluorescent protein reporter gene reveal predominant oligodendrocytic expression of Cx47 and display vacuolized myelin in the CNS. J Neurosci 2003; 23: 4549–4559, [PUBMED], [INFOTRIEVE], [CSA]
- White T W, Bruzzone R, Goodenough D A, Paul D L. Mouse Cx50, a functional member of the connexin family of gap junction proteins, is the lens fiber protein MP70. Mol Biol Cell 1992; 3: 711–720, [PUBMED], [INFOTRIEVE], [CSA]
- White T W, Srinivas M, Ripps H, Trovato-Salinaro A, Condorelli D F, Bruzzone R. Virtual cloning, functional expression, and gating analysis of human connexin31.9. Am J Physiol 2002; 283: C960–C970, [CSA]
- Husøy T, Mikalsen S -O, Sanner T. Phosphatase inhibitors, gap junctional intercellular communication and [125I]-EGF binding in hamster fibroblasts. Carcinogenesis 1993; 14: 2257–2265, [CSA]
- Uhlenberg B, Schuelke M, Ruschendorf F, Ruf N, Kaindl A M, Henneke M, Thiele H, Stoltenburg-Didinger G, Aksu F, Topaloglu H, Nurnberg P, Hubner C, Weschke B, Gartner J. Mutations in the gene encoding gap junction protein α12 (connexin 46.6) cause Pelizaeus-Merzbacher-like disease. Am J Hum Genet 2004; 75: 251–260, [PUBMED], [INFOTRIEVE], [CSA], [CROSSREF]
- Omori Y, Mesnil M, Yamasaki H. Connexin 32 mutations from X-linked Charcot-Marie-Tooth disease patients: functional defects and dominant negative effects. Mol Biol Cell 1996; 7: 907–916, [PUBMED], [INFOTRIEVE], [CSA]
- Rouan F, White T W, Brown N, Taylor A M, Lucke T W, Paul D L, Munro C S, Uitto J, Hodgins M B, Richard G. Trans-dominant inhibition of connexin-43 by mutant connexin-26: Implications for dominant connexin disorders affecting epidermal differentiation. J Cell Sci 2001; 114: 2105–2113, [PUBMED], [INFOTRIEVE], [CSA]