Abstract
Recent studies have revealed a second class of gap-junction-forming proteins in vertebrates. These genes are termed pannexins, and it has been suggested that they perform similar functions as connexins. Pannexin1 is expressed in diverse tissues including the central nervous system and seems to form gap junction channels in the Xenopus oocyte expression system. Since protein interacting partners have frequently been described for connexins, the most prominent family of gap junction forming proteins, we thus started to search for candidate genes of pannexin interacting partners. Kvβ3, a protein belonging to the family of regulatory β-subunits of the voltage-dependent potassium channels, was identified as a binding partner of pannexin1 in an E. coli two-hybrid system. This result was verified by confocal laser scanning microscopy using double transfected Neuro2A cells. The colocalization of both proteins at the plasma membrane is suggestive of functional interaction.
INTRODUCTION
Recently, a novel group of potential gap junction proteins termed pannexins was identified. They were discovered because of their predicted structural homology to the innexins, the major group of gap junction forming proteins in invertebrates. In contrast to innexins, pannexins occur in both phyla (Citation1). Pannexin1 (Panx1) is abundantly expressed in different parts of the central nervous system (CNS) and in various nonneural tissues (Citation2, Citation3, Citation4).
Bruzzone et al. (Citation3, Citation5) were able to demonstrate the formation of homomeric Panx1 hemichannels and homotypic intercellular channels in the Xenopus oocyte expression system. Furthermore, the formation of heteromeric hemichannels and intercellular channels in combination with Pannexin2 (Panx2) was shown (Citation3). Based on these findings, Panx1 has been suggested to account for an additional neuronal gap junction forming protein (Citation3). This assumption has been strengthened by a comparative study of expression levels of neuronal connexins and Panx1 in the hippocampus and the inferior olive which reported high expression levels for Panx1 in both brain areas (Citation6). At present the issue of whether pannexins play a substantial role as gap junction forming proteins has not been settled. The discovery that Panx1 hemichannels are mechanosensitive and ATP permeant (Citation7) suggested additional roles for these channel-forming proteins which involve functional regulation by interacting partners.
We, therefore, focused on a two-hybrid system originally developed by Joung and coworkers (Citation8) (). A stringent two-step selection procedure was used to identify putative binding proteins. The strategy led to the identification of Kvβ3, a protein belonging to the family of regulatory β-subunits of the voltage-dependent potassium channels. Confocal laser scanning microscopy of Panx1 and Kvβ3 transfected Neuro2A-cells confirmed colocalization of both proteins in the plasma membrane, supporting functional interaction.
Figure 1. Cartoon of the E. coli two-hybrid system. The DNA of the reporter gene construct is represented through the black line. λ cI is the repressor protein of the bacteriophage λ, and RNAP the N-terminal domain of the α -subunit of the bacterial RNA-polymerase. The promoter (PlacZ) is a modified form of the lac promoter, which is not inducible by IPTG. “bait” is the protein of interest (in this case the C-terminus of Panx1), while the potential interacting partner is called “target.” The expression of the two reporter genes HIS3 and aadA is induced through the depicted interaction of the two hybrid structures (adapted from Stratagene).

METHODS
E. coli Two-hybrid System
The BacterioMatch® II two-hybrid system including plasmids pBT, pTRG and the positive control plasmids pBT-LGF2 and pTRG-Gal11P, the BacterioMatch® II Rat Brain Library, and BacterioMatch® II Reporter Cells were purchased from Stratagene (La Jolla, CA, USA). (All media and procedures are described in detail at http://www.stratagene.com)
A sequence corresponding to the C-terminal amino acids 297–426 of mouse Panx1 (gi:18043025) was cloned into the pBT-vector, giving rise to plasmid pBT-Panx1-CT. This vector expresses the full-length bacteriophage λ repressor protein fused to the inserted bait. A rat brain cDNA library was fused to the N-terminal domain of the α -subunit of the prokaryotic RNA polymerase. The screening transformation, selection steps, and isolation of the target plasmids were performed according to the manufacturer's recommendation. The inserts of positive clones were sequenced and analyzed using the BLASTX-algorithm (Citation9).
Expression Analysis and Confocal Imaging
The full-length mouse Panx1 cDNA (gi:18043025; nt 373-1650) excluding the stop codon was cloned into the plasmid pEYFP-N1 (BD Biosciences). The full-length mouse Kvβ3 cDNA (gi:17028407; nt 351-1562) excluding the stop codon was cloned into the plasmid pECFP-N1 (BD Biosciences). The expression of Panx1 and Kvβ3 expression vector constructs was confirmed by Western blot analysis using transient transfected Neuro2A cells (Citation10). Neuro2A cells were obtained from the ATCC collection (Manassas, VA, USA), cultured and transfected as described (Citation10).
Confocal laser scanning microscopy was performed 48h after transient transfection on samples fixed with 4% paraformaldehyde. Samples were mounted using ProLong® Antifade Kit (Invitrogen, Karlsruhe, Germany), coverslipped, and analyzed with a Zeiss LSM 510-Meta inverted confocal microscope (Zeiss, Göttingen, Germany). Data were collected as Z-stacks using the “emission fingerprinting mode” scanning module and analyzed using the Zeiss LSM Image Browser software. Colocalization was calculated from TIFF files using the RG2B plugin program implemented into the ImageJ software (http://rsb.info.nih.gov/ij/) with default settings.
RESULTS
Interacting partners of the protein Panx1 were identified by a library screen using a rat brain cDNA library as target and the carboxy-terminus of Panx1 as bait (). Self-activation of the reporters was excluded by several cotransformations. The known interaction of the dimerization domain of the yeast transcriptional activator Gal4 with a domain derived from a mutant form of Gal11 protein (Gal11P) was used to verify that the experimental conditions applied were permissive for protein interaction in E. coli cells on selective medium (Citation11). Pilot experiments demonstrated that one screening transformation produces sufficient double transformants to cover the whole rat brain library containing 2.1× 106 primary clones. In the following screening transformation more than 3000 double transformants were obtained on selective medium lacking histidine and containing the inhibitor 5 mM 3-aminotriazol (3-AT), increasing the necessary amount of HIS3 gene product. Thereafter, 169 fast growing colonies were chosen for testing the activation of the second reporter gene aadA. This selection step led to a further reduction to 82 clones. The pTRG-cDNA plasmid of each colony was isolated and subjected to a second round of transformation including pBT-Panx1-CT (positive control) and pBT (negative control). Finally, 46 plasmids were chosen for sequencing. All sequences were compared using the basic local alignment search tools (BLASTX 2.2.9) (Citation9). A total of 25 different peptide sequences were obtained. Out of these, four sequences were identified which led to the synthesis of annotated fusion proteins with the α -subunit of the RNA-polymerase. Among the four positive sequences was the protein Kvβ3. The cDNA clone recovered had 100% identity with the last 58 amino acids of the Kvβ3 protein.
For further verification of the interaction between Kvβ3 and Panx1, we decided to perform colocalization studies on double transfected Neuro2A cells using pEYFP-Panx1 and pECFP-Kvβ3 expression vector constructs. Neuro2A cells were transiently transfected and the expression of the two vector constructs verified by Western blot analysis as shown in . This experiment demonstrated the expression of the fusion protein products, since immunoreactive bands were detected close to the expected sizes (Panx1-EYFP, 75 kDa, , lane 5; Kvβ3-ECFP, , lane 6, 71 kDa). Furthermore, coexpression was confirmed in double transfected cells (, lane 7).
Figure 2. Western-blot analyses of transfected Neuro2A cells. Neuro2A cells were transfected with 400ng DNA and lysed 48 h later. 12 μg protein was loaded in case of double-transfected cells and 8 μg in case of single-transfected cells. Immunodetection was carried out using BD-Living Colors A.v. Peptide antibody (BD Biosciences).
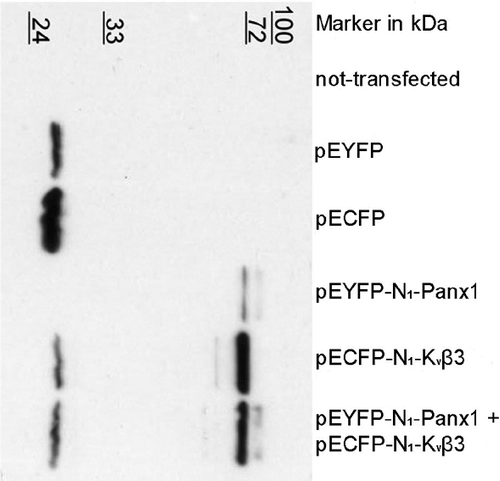
Next, the intracellular distribution of the two fusion proteins was analyzed in single and double transfected cells by confocal laser scanning microscopy making use of the distinct expression spectra of the ECFP and EYFP fusion proteins. The single transfected cells were used to record reference spectra. Confocal pictures showed that Panx1-EYFP was mainly located in the plasma membrane while Kvβ3-ECFP was found in the cytoplasm (data not shown).
Colocalization was investigated in double transfected cells. Recorded spectra of Z-stacks were unmixed using the Panx1-EYFP and Kvβ3-ECFP reference spectra to identify the intracellular localization of both proteins. summarizes a typical distribution of Kvβ3 () and Panx1 () fluorescence. Panx1 appeared to colocalize with Kvβ3 almost exclusively in plasma membrane regions in case of double transfected cells as shown in the merged image (). Finally, colocalization information was extracted from raw data using the ImageJ software. This analysis () demonstrated Panx1 and Kvβ3 colocalization in membranous structures. In summary, our results provide evidence that an interaction between the two proteins may exist in both bacteria and eukaryotic cells, making the Kvβ3 protein the first candidate for a Panx1 interacting partner.
DISCUSSION
We gained first evidence for an interacting partner of Panx1 by applying an E. coli two-hybrid system. Two stringent selection steps using two different reporter genes yielded four annotated clones. Furthermore, 21 clones were obtained generating fusion proteins of yet unknown identity. This result supports our experimental strategy to select the C-terminal domain of Panx1 as bait consistent with previous studies on connexins demonstrating that the C-terminus is a prominent candidate region for various protein interactions (Citation12, Citation13, Citation14, Citation15). One of the four clones expressed part of the C-terminal domain of the Kvβ3 protein. Kvβ3 belongs to the family of β-subunits of voltage-dependent potassium channels (Citation16). The β-subunits are expressed in the central nervous system and are known to be oxoreductase enzymes with a NADPH-binding site as well as a binding site for an unknown substrate (Citation17). Some of these proteins convey rapid inactivation to the otherwise very slowly inactivating delayed rectifier channels. The velocity of the inactivation is dependent on the intracellular redox potential (Citation16, Citation18).
With respect to our in vitro findings, we regarded an interaction of Panx1 with Kvβ3 feasible. We therefore employed confocal microscopy to analyze the localization of these two proteins in transfected Neuro2A cells. Confocal laser imaging is a well established technique to verify the interaction of connexins with cytoplasmic binding proteins (Citation12, Citation14). Colocalization of the two fusion proteins was confirmed which occurred preferentially in the plasma membrane. This result suggests that a physical interaction between the two proteins is likely in eukaryotic cells. However, the present data are preliminary and additional experiments need to prove this interaction beyond doubt. Technically, coimmunoprecipitations using antibodies directed against tagged fusion proteins are capable of proving an interaction with high confidence. Since the large EYFP-/ECFP-tags used in the present study potentially could interfere with the binding of Kvβ3 and Panx1, it is necessary to introduce small tags at the amino terminus of the fusion proteins. These experiments are underway and first results indicate that this interaction does exist in transfected cells (data not shown).
It has been speculated that the interaction of some β-subunits with delayed rectifier channels resembles the ball and chain model known for alpha subunits of the A-type potassium channels (Citation16, Citation18). A similar ball and chain model involving the C-terminus has been proposed in connexin functions (Citation19, Citation20). We thus suggest that Kvβ3 binds to the carboxy-terminus of Panx1 and is likely to be involved in controlling inactivation mechanisms of Panx1 hemichannels or intercellular channels, possibly in response to changes of the intracellular redox potential.
ACKNOWLEDGEMENTS
We thank Helga Schulze for excellent technical assistance. This project is supported by grants of the Graduiertenkolleg GRK 736 “Development and Plasticity of the Nervous System: Molecular, synaptic and cellular mechanisms” to S.B., and A.H., and the DFG (292/11-4) to R.D. and G.Z.
REFERENCES
- Panchin Y, Kelmanson I, Matz M, Lukyanov K, Usman N, Lukyanov S. A ubiquitous family of putative gap junction molecules. Curr Biol 2000; 10: R473–R474, [PUBMED], [INFOTRIEVE], [CSA]
- Baranova A, Ivanov D, Petrash N, Pestova A, Skoblov M, Kelmanson I, Shagin D, Nazarenko S, Geraymovych E, Litvin O, Tiunova A, Born T L, Usman N, Staroverov D, Lukyanov S, Panchin Y. The mammalian pannexin family is homologous to the invertebrate innexin gap junction proteins. Genomics 2004; 83: 706–716, [PUBMED], [INFOTRIEVE], [CSA], [CROSSREF]
- Bruzzone R, Hormuzdi S G, Barbe M T, Herb A, Monyer H. Pannexins, a family of gap junction proteins expressed in brain. Proc Natl Acad Sci USA 2003; 100: 13644–13649, [PUBMED], [INFOTRIEVE], [CSA], [CROSSREF]
- Ray A, Zoidl G, Weickert S, Wahle P, Dermietzel R. Site-specific and developmental expression of pannexin1 in the mouse nervous system. Eur J Neurosci 2005; 21: 3277–3290, [PUBMED], [INFOTRIEVE], [CSA], [CROSSREF]
- Bruzzone R, Barbe M T, Jakob N J, Monyer H. Pharmacological properties of homomeric and heteromeric pannexin hemichannels expressed in Xenopus oocytes. J Neurochem 2005; 92: 1033–1043, [PUBMED], [INFOTRIEVE], [CSA], [CROSSREF]
- Weickert S, Ray A, Zoidl G, Dermietzel R. Expression of neural connexins and pannexin1 in the hippocampus and inferior olive: A quantitative approach. Brain Res Mol Brain Res 2005; 133: 102–109, [PUBMED], [INFOTRIEVE], [CSA], [CROSSREF]
- Bao L, Locovei S, Dahl G. Pannexin membrane channels are mechanosensitive conduits for ATP. FEBS Lett 2004; 572: 65–68, [PUBMED], [INFOTRIEVE], [CSA], [CROSSREF]
- Joung J K, Ramm E I, Pabo C O. A bacterial two-hybrid selection system for studying protein-DNA and protein-protein interactions. Proc Natl Acad Sci USA 2000; 97: 7382–7387, [PUBMED], [INFOTRIEVE], [CSA], [CROSSREF]
- Gish W, States D J. Identification of protein coding regions by database similarity search. Nat Genet 1993; 3: 266–272, [PUBMED], [INFOTRIEVE], [CSA], [CROSSREF]
- Zoidl G, Meier C, Petrasch-Parwez E, Zoidl C, Habbes H W, Kremer M, Srinivas M, Spray D C, Dermietzel R. Evidence for a role of the N-terminal domain in subcellular localization of the neuronal connexin36 (Cx36). J Neurosci Res 2002; 69: 448–465, [PUBMED], [INFOTRIEVE], [CSA], [CROSSREF]
- Dove S L, Hochschild A. Conversion of the omega subunit of Escherichia coli RNA polymerase into a transcriptional activator or an activation target. Genes Dev 1998; 12: 745–754, [PUBMED], [INFOTRIEVE], [CSA]
- Giepmans B N, Moolenaar W H. The gap junction protein connexin43 interacts with the second PDZ domain of the zona occludens-1 protein. Curr Biol 1998; 8: 931–934, [PUBMED], [INFOTRIEVE], [CSA], [CROSSREF]
- Kausalya P J, Reichert M, Hunziker W. Connexin45 directly binds to ZO-1 and localizes to the tight junction region in epithelial MDCK cells. FEBS Lett 2001; 505: 92–96, [PUBMED], [INFOTRIEVE], [CSA], [CROSSREF]
- Li X, Olson C, Lu S, Kamasawa N, Yasumura T, Rash J E, Nagy J I. Neuronal connexin36 association with zonula occludens-1 protein (ZO-1) in mouse brain and interaction with the first PDZ domain of ZO-1. Eur J Neurosci 2004; 19: 2132–2146, [PUBMED], [INFOTRIEVE], [CSA], [CROSSREF]
- Warn-Cramer B J, Lampe P D, Kurata W E, Kanemitsu M Y, Loo L W, Eckhart W, Lau A F. Characterization of the mitogen-activated protein kinase phosphorylation sites on the connexin-43 gap junction protein. J Biol Chem 1996; 271: 3779–3786, [PUBMED], [INFOTRIEVE], [CSA], [CROSSREF]
- Heinemann S H, Rettig J, Wunder F, Pongs O. Molecular and functional characterization of a rat brain Kv beta 3 potassium channel subunit. FEBS Lett 1995; 377: 383–389, [PUBMED], [INFOTRIEVE], [CSA], [CROSSREF]
- Gulbis J M, Mann S, MacKinnon R. Structure of a voltage-dependent K+ channel beta subunit. Cell 1999; 97: 943–952, [PUBMED], [INFOTRIEVE], [CSA], [CROSSREF]
- Rettig J, Heinemann S H, Wunder F, Lorra C, Parcej D N, Dolly J O, Pongs O. Inactivation properties of voltage-gated K+ channels altered by presence of beta-subunit. Nature 1994; 369: 289–294, [PUBMED], [INFOTRIEVE], [CSA], [CROSSREF]
- Anumonwo J M, Taffet S M, Gu H, Chanson M, Moreno A P, Delmar M. The carboxyl terminal domain regulates the unitary conductance and voltage dependence of connexin40 gap junction channels. Circ Res 2001; 88: 666–673, [PUBMED], [INFOTRIEVE], [CSA]
- Moreno A P, Chanson M, Elenes S, Anumonwo J, Scerri I, Gu H, Taffet S M, Delmar M. Role of the carboxyl terminal of connexin43 in transjunctional fast voltage gating. Circ Res 2002; 90: 450–457, [PUBMED], [INFOTRIEVE], [CSA], [CROSSREF]