Abstract
Dye-doped liquid crystals (LCs) have attracted much attention as promising materials for all-optical smart windows and optical limiters. However, more efficient nonlinear optical (NLO) molecular reorientation is expected to be applied to the materials and devices. This review introduces recent efforts to enhance optical nonlinearity using oligothiophene dye. First, the mechanism of NLO response based on oligothiophene-doped LCs is described. Second, our efforts focusing on nonlinearity enhancement in oligothiophene-doped LCs are introduced. Finally, the optical applications of the oligothiophene dye-doped LCs are presented. This review contributes to the material design for all-optical devices utilizing the nonlinear response of dye-doped LCs.
1. Introduction
Since the discovery of laser technology in 1960, optics and electro-optics have rapidly evolved. Immediately after that, many researchers have increasingly studied a nonlinear optical (NLO) effect that changes optical properties under an intense optical-electric field. The most well-known NLO processes are, for instance, harmonic generation [Citation1,Citation2], multiple-photon absorption [Citation3,Citation4], and self-phase modulation [Citation5,Citation6]. The NLO effect has been reported in various materials, such as crystals [Citation7], polymers [Citation1,Citation8], and liquid crystals (LCs) [Citation9].
Among all NLO materials, LCs exhibit large optical nonlinearities due to their high optical anisotropy in coincidence with molecular reorientation. Thus, LCs have been extensively studied over the last decades. In 1980, three groups almost simultaneously discovered the giant optical nonlinearity in nematic LCs (nonlinear refractive index n2 = 10−5 cm2/W), nine orders of magnitude larger than the previous NLO materials [Citation10–12]. Subsequently, two groups demonstrated that the threshold intensity of molecular reorientation decreased to an unobservable level in a hybrid-aligned LC whose director was changed continuously from out-of-plane to in-plane direction [Citation13–15]. Furthermore, Tabiryan et al. [Citation16] published a more than 100-page review of the NLO effects of nematic LCs. The threshold intensity to induce the NLO effect was 100 W/cm2, much lower than other materials in previous reports. However, taking advantage of the NLO effects of LCs still required high-power and focused continuous-wave (CW) laser sources. With high expectations of developing optical devices for future NLO applications, further enhancement of the light sensitivity of LC was desired.
An excellent approach to enhance the sensitivity of LCs was proposed by doping the LCs with a small amount of certain dichroic organic dyes. The dyes are classified into two types: (1) photoisomerizable dyes and (2) non-photoisomerizable dyes. One of the well-known photoisomerizable dyes is azobenzene derivatives, which show high sensitivity due to the molecular reorientation of LCs triggered by axial-selective trans-cis photoisomerization (photochemical reaction) [Citation17–23]. Upon irradiation of azobenzene-doped LCs with a linearly polarized beam, azobenzene molecules cause trans-cis-trans photoisomerization, resulting in a unidirectional orientation perpendicular to the incident polarization direction (or along the direction of the beam propagation). Azobenzene-doped LCs have attracted a great deal of attention due to their high sensitivity and fast response, which are suitable for various photonic applications, such as optical switching [Citation24], smart window [Citation25], flat lens [Citation26], and mechanical actuation [Citation27]. However, azobenzene-based systems require two light sources with another wavelength for reversible reorientation.
On the other hand, non-photoisomerizable dyes show a more direct response, which is called the Jánossy effect. In 1990, Jánossy et al. [Citation28] reported that doping a small amount (below 1 mol%) of anthraquinone dyes to LCs lowered the threshold intensity of molecular reorientation by two orders of magnitude than undoped LC systems. The rod-shaped dyes excited by light increase the interaction with the LC molecules, achieving high sensitivity. Molecules in this system tend to align parallel to the incident polarization direction. Such non-photoisomerizable dye-doped LCs have unique advantages compared to the photoisomerizable dye-doped LCs as follows:
Photoinduced molecular reorientation only occurs when light above a well-defined threshold intensity is incident on the LCs. This gives a certain advantage in optical applications, such as optical limiters that protect human eyes and optical sensors from irradiation with lasers.
The molecular reorientation process is an order-to-order process, which allows for more precise control of LC alignment.
Non-photoisomerizable dye-doped LCs can be more stable than azobenzene-doped LCs because the chemical conformation of the non-photoisomerizable dye-doped LC system remains unchanged.
Although there are such attractive benefits, non-photoisomerizable-doped LC systems are less noted than azobenzene systems, primarily due to the relatively high threshold intensity of molecular reorientation.
In the past decades, we have focused on exploring advanced non-photoisomerizing dyes that achieve relatively low thresholds of molecular reorientation. This work reviews recent progress in nonlinear molecular reorientation using non-photoisomerizable oligothiophene dyes, which are effective π-conjugated systems. First, Section 2 describes the mechanism of molecular reorientation in LCs induced by irradiation with linearly polarized light. Section 3 introduces the oligothiophene dye-doped LCs. Section 4 focuses on nonlinearity enhancement in oligothiophene-doped LCs. Finally, Section 5 presents the possible applications utilizing oligothiophene-doped LCs.
2. Mechanism for photoinduced molecular reorientation in LCs and non-photoisomerizable dye-doped LCs
Rod-shaped nematic LCs with optical anisotropy, such as refractive index and dielectric constant, tend to align their long-axis directions parallel together. The average collective direction of the long axis is defined as the director n. In an LC cell composed of two substrates whose surfaces were treated, the director of LC molecules is usually aligned perpendicular to the substrates (homeotropic alignment) or parallel to the substrates (homogeneous alignment). Irradiation with linearly polarized light to nematic LC molecules with positive dielectric anisotropy causes the molecular reorientation parallel to the polarization direction [Citation29]. The physical mechanism is shown in . The optical field E creates an electric torque τem that pulls the direction n toward the electric field direction. At the same time, the director rotation is opposed by surface anchoring sanc and bulk elasticity sela. This torque balance determines the final reorientation of the molecular director n at the steady state, which leads to the refractive index change. As mentioned in Section 1, nematic LCs exhibit much larger optical nonlinearity than other NLO materials due to their unique properties in coincidence with molecular reorientation.
Figure 1. Schematic images of the mechanism of photoinduced molecular reorientation by linearly polarized light for (a) LCs and (b) dye-doped LCs. The red and green arrows in are the molecular reorientational torques as a result of the interaction between dye and LC molecule. The torque balance generates a dye torque τdye, which pulls the director n toward the excited dye molecules.
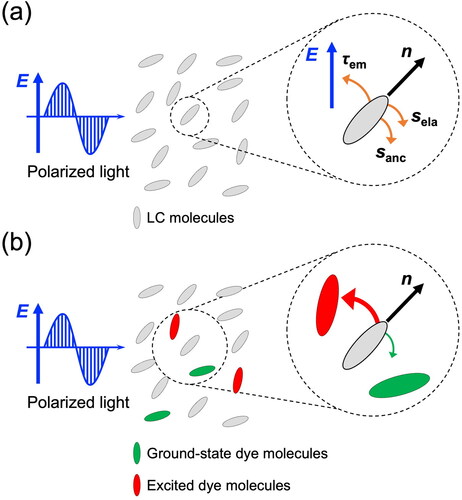
As described in Section 1, Jánossy et al. [Citation28] reported unexpected observation of significant nonlinear effects in nematic LCs doped with small amounts (below 1%) of anthraquinone dyes. Accountable models were proposed by Jánossy [Citation30] and Marrucci and Paparo [Citation29,Citation31] (). For dye-doped LC, the dye molecules are selectively excited by polarized light because the probability of light absorption is given by p ∝ cos2α, where α is the angle between the optical electric field E and the molecule transition dipole. Then, the excited dye molecules attract the director’s orientation, which is more potent than the ground-state dye molecules. This bias generates the additional dye torque τdye. The dye torque contributes to an increase in total optical torque τo (τo = τem + τdye), which makes reorientation of director n easier. Although the orientational distribution of the overall dye population is not affected by the photoinduced excitation, the dye molecules are aligned parallel to the polarization direction due to the reorientation of LC director.
3. Oligothiophene-doped LCs
As described earlier, azobenzene and anthraquinone derivatives effectively enhance the optical nonlinearity of dye-doped LCs. Much work has been done to find novel effective dyes that further increase optical nonlinearity [Citation32–41]. In those processes, it has been suggested that the guest-host interaction in the excited state of the dye based on the LC and dye structure is the critical factor in enhancing optical nonlinearity. It has not been yet clear which types of intermolecular interaction are involved. However, since van der Waals interactions are the main force between dye and LC molecules in most guest-host systems, a large photoinduced change in dipole moment or polarizability of the dye molecules is expected to be effective.
Our group assumed that conjugated oligomers and polymers would be good candidates for improving optical nonlinearity due to their high polarizability derived from electronic delocalization. In addition, when they are photoexcited, their polarizability is even higher at the ground state [Citation42]. In 2000, we designed a thiophene-based oligomer, 5-5′′-bis-(5-butyl-2-tienylethynyl)-2,2′:5′,2′′-terthiophene (TR5), which contains five thiophene group () [Citation43]. This dye molecule was designed based on the following concepts:
Figure 2. Reaction scheme for the synthesis and structure of TR5. Reprinted with permission from Zhang et al. [Citation43].
![Figure 2. Reaction scheme for the synthesis and structure of TR5. Reprinted with permission from Zhang et al. [Citation43].](/cms/asset/9e1ed969-75d1-428e-941f-9801d35bdeb9/gmcl_a_2360220_f0002_b.jpg)
Oligomers are more compatible with LCs than polymers.
Conjugated thiophenes exhibit higher environmental stability than oligomers with a vinylene spacer.
The effective conjugation length of oligothiophene is much longer than that of oligophenylenes, which shows better electron delocalization and absorption in the visible region [Citation44].
The acetylene group acting as a weak electron acceptor shows a good conjugative character with donor systems and exhibits different conjugative characteristics in the excited and ground states. Thus it might enhance the photoinduced change in the electronic properties of TR5 [Citation45].
To investigate the effect of TR5 on nonlinear molecular reorientation, two dye-doped LCs were prepared by doping 0.22 mol% of TR5 into host LCs. A polar LC, 5CB (4-cyano-4′-pentyl biphenyl) and an apolar LC, MBBA (N-4-methoxybenzylidene)-4-butylaniline) were used as host LCs to observe how TR5 affects the different polar LCs. Then, the mixtures were injected into 100-µm-thick glass cells, whose inner surfaces had been treated with lecithin for homeotropic alignment. TR5-doped 5CB and MBBA cells showed similar absorbance values of 0.27 and 0.26 at 488 nm, respectively.
The nonlinear molecular reorientation of those two dye-doped LCs was investigated by self-diffraction ring measurement, a classic approach often used to evaluate the NLO response of LC systems. shows the mechanism of self-diffraction ring generations. The diffraction ring is formed by the spatial distribution of the refractive index due to photoinduced molecular reorientation. Irradiation of a homeotropic LC cell with a low-intensity Gaussian beam maintains its initial molecular orientation (). On the other hand, when the light intensity exceeding the threshold is incident on the LCs to trigger the molecular reorientation, the diffraction rings are generated due to self-focusing and self-phase modulation, which are NLO effects () [Citation12]. The number of rings (N) depends on the photoinduced refractive index change (Δn′) of the samples as well as the wavelength (λ) and cell thickness (L) through |Δn′| = NλL−1 [Citation46]. Rod-shaped LC molecules have an anisotropic refractive index in the short and long axes of the molecules. When the photoinduced molecular reorientation from perpendicular to parallel to the polarization direction occurs, the refractive index change Δn′ becomes large, increasing the number of rings N. Here, the threshold intensity of self-diffraction measurement is defined as the light intensity at which the first diffraction ring appears.
Figure 3. Principle of self-diffraction ring formation: (a) below and (b) above the threshold light intensity.
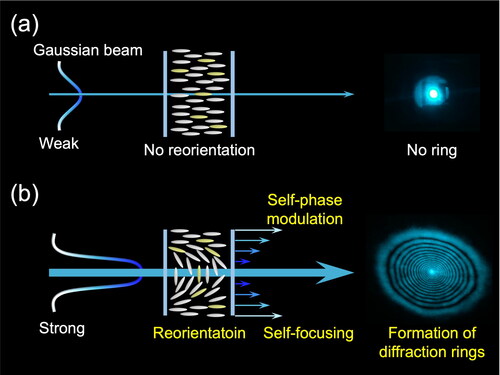
displays the typical diffraction-ring pattern of TR5-doped 5CB. The number of rings as a function of incident light intensity is shown in for undoped 5CB and MBBA and for TR5-doped 5CB and MBBA. The threshold light intensity for undoped 5CB and MBBA is 840 and 830 W/cm2, while those for TR5-doped 5CB and MBBA are highly reduced to 6.2 and 4.8 W/cm2, respectively. Only 0.22 mol% of TR5 can decrease the threshold intensity about 135 times for 5CB and 173 times for MBBA. These results indicate that doping of TR5 can significantly enhance optical nonlinearity. Based on the mechanism proposed by Jánossy and Marrucci [Citation29–31], the change in the interaction between the TR5 and host LC at the ground and excited state enhances the NLO response. Despite the unclear detailed mechanism, the efficiency of TR5, which is a non-polar molecule, in both 5CB and MBBA supports the idea that changes in dispersive interaction between TR5 and host LC molecules are a key factor for the enhancement. Assuming the same reorientation mechanism for TR5-doped 5CB and MBBA, the photoinduced change of polarizability plays an essential role in the reorientation process.
Figure 4. Typical diffraction rings observed in a TR5-doped 5CB system. Reprinted with permission from Zhang et al. [Citation43].
![Figure 4. Typical diffraction rings observed in a TR5-doped 5CB system. Reprinted with permission from Zhang et al. [Citation43].](/cms/asset/61b5a344-4ecc-4d55-8710-168f3dba53cd/gmcl_a_2360220_f0004_c.jpg)
Figure 5. The number of rings as a function of light intensity observed in (a) undoped LC hosts and (b) 0.22 mol% TR5-doped systems. Reprinted with permission from Zhang et al. [Citation43].
![Figure 5. The number of rings as a function of light intensity observed in (a) undoped LC hosts and (b) 0.22 mol% TR5-doped systems. Reprinted with permission from Zhang et al. [Citation43].](/cms/asset/b923dd98-66eb-4eae-a00e-81ff50afee11/gmcl_a_2360220_f0005_b.jpg)
To investigate the effect of the polarizability of dye molecules, Yaegashi et al. [Citation47] newly synthesized two oligomer-type thiophene derivatives with ester moieties as the guest dye (). The molecular design includes the following points. First, an ester group is well-known as a weak electron acceptor group, which could enhance the absorption and optical nonlinearity. Second, an alkyl chain that increases solubility can be substituted through the ester moiety unlike cyano and nitro groups. Third, a carbonyl group is a polar group that might enhance the dipole-dipole interaction between dye and LC molecules with the cyano group. Then, 5CB with the cyano group was used as a host LC. The nonlinear molecular reorientation was evaluated by the self-diffraction measurement. As a result, only TD1, which has an ester moiety directly connected to the terminal thiophene, successfully reduced the threshold intensity for molecular reorientation to 1/3 of that using TR5. This result indicates that the direct introduction of the ester moiety into thiophene could expand the intramolecular delocalization of π electrons along the molecular long axis, and enhance the molecular polarizability of the excited state compared to the other two dye molecules.
Figure 6. Chemical structures of the dyes used in the articles of Yaegashi et al. [Citation47] and Yaegashi et al. [Citation57], and their abbreviations. Reprinted with permission from Yaegashi et al. [Citation47]. Copyright 2005, American Chemical Society.
![Figure 6. Chemical structures of the dyes used in the articles of Yaegashi et al. [Citation47] and Yaegashi et al. [Citation57], and their abbreviations. Reprinted with permission from Yaegashi et al. [Citation47]. Copyright 2005, American Chemical Society.](/cms/asset/c95b3274-d55e-4612-a562-af8df5e1312f/gmcl_a_2360220_f0006_b.jpg)
4. Sensitizing methods of molecular reorientation in dye-doped LCs
As described above, we show that oligothiophene dye is a candidate for improving the optical nonlinearity of the LC system. However, the threshold intensity required molecular reorientation is still in the W/cm2 range. Thus, various approaches to enhance the sensitivity of molecular reorientation have been conducted regarding materials design, surface treatment, assistance of electric field, and light source.
Polymer-stabilized LCs (PSLCs) that contain a small amount of polymer can significantly enhance the sensitivity of molecular reorientation in dye-doped LCs. Aihara et al. [Citation48] reported that polymer stabilization not only lowered the threshold intensity of the molecular reorientation in oligothiophene-doped NLCs by a factor of six than that of a low-molecular LC system but also increased the induced refractive change by 56%. Furthermore, to investigate the effect of polymer in dye-doped LCs, Wang et al. [Citation49] prepared PSLCs with different polymer concentrations (0, 2, 4, 6, 7, 8, 9, 10, 11, and 13 mol%). The lowest threshold light intensity of 3.4 W/cm2 was obtained at 10 mol%. The mechanism of high sensitization can be explained as follows. First, the polymer disordered the molecular alignment, which increases the light absorbance and, hence, the optical torque for molecular reorientation. Second, polymers reduce the surface anchoring that arises from the interface between the LCs and the substrate.
Mejia et al. [Citation50] reported that incorporating 5 wt% of polymer-grafted ZnO nanorods in oligothiophene-doped host LCs were able to significantly reduce the threshold intensity of molecular reorientation by 39% compared to pure oligothiophene-doped systems. The reason for this is interpreted as the change in the order parameter of LCs. In fact, the order parameter of LCs decreased by 21% only by incorporating 1 wt% of polymer-grafted ZnO nanorods in the oligothiophene-doped host LCs. Incorporating polymer-grafted ZnO nanorods caused a slight disordering of LC and TR5 molecules, weakening the forces from the surface anchoring in the LC cells.
Yokota et al. [Citation51] investigated the effect of host LC structures on molecular reorientation using fluorinated LCs (). Host LCs were prepared by adding fluorinated LCs (F-LCx: x = 1–4) to 5CB with 25 mol% concentration. The threshold light intensity for molecular reorientation in mixtures using trifluorinated LCs was 42% lower than in LCs without fluorine substituents. Furthermore, there was a clear relationship between the threshold intensity and the K33 elastic constant of LCs, which is related to the elastic torque (). This result indicates that the decreased elastic torque contributes to the higher sensitivity.
Figure 7. Chemical structures of the components as host LCs used in the articles of Yokota et al. [Citation51] and Yokota et al. [Citation59].
![Figure 7. Chemical structures of the components as host LCs used in the articles of Yokota et al. [Citation51] and Yokota et al. [Citation59].](/cms/asset/b2e97618-4fd5-4461-9f3d-3e3f7bc33663/gmcl_a_2360220_f0007_b.jpg)
Table 1. The K33 elastic constant and threshold intensity of TR5/5CB, TR5/(5CB F-LC1), TR5/(5CB F-LC2), TR5/(5CB F-LC3), and TR5/(5CB F-LC4).
From the surface treatment viewpoint, our group reduced light intensity. Wang et al. [Citation52] reported a molecular reorientation induced with a 1 mW handheld laser pointer in hybrid-aligned TR5-doped PSLC. The key to the achievement is using hybrid molecular alignment instead of the commonly used homeotropic or homogeneous LC alignment. The schematic images of hybrid-alignment and homeotropic cells are shown in and b. Hybrid alignment refers to homeotropic (out-of-plane) alignment at one substrate of the LC cell. In contrast, homogeneous (in-plane) alignment occurs at the other substrate. Both cells were transparent and of high optical quality (), and their initial molecular alignment were confirmed by polarized optical microscopy (POM) (). The threshold intensity in the hybrid-aligned cell was 0.4 W/cm2, which was markedly reduced by a factor of 8.5 compared with that of homeotropic-aligned PSLC. The surface anchoring direction in the homeotropic-aligned cell is perpendicular to the final molecular alignment, indicating that torque must induce molecular reorientation. On the other hand, the surface anchoring direction of the homogeneous side of the hybrid-aligned cell is parallel to the light polarization; the optical torque required to trigger the molecular reorientation could be significantly reduced. Hence, such a low threshold intensity was achieved.
Figure 8. (a) Schematic images of a hybrid-aligned cell. (b) Schematic images of a homeotropic-aligned cell. (c) Photograph of a hybrid-aligned cell. (d) Photograph of a homeotropic-aligned cell. (e) Polarized optical micrograph of a hybrid-aligned cell on a rotatable stage. (f) Polarized optical micrograph of a homeotropic-aligned cell on a rotatable stage. Optically transparent film with hybrid alignment is confirmed from photograph and conoscopic images with a polarized optical microscope. Reprinted with permission from Wang et al. [Citation52]. Copyright 2015. The authors licensed under CC BY 4.0.
![Figure 8. (a) Schematic images of a hybrid-aligned cell. (b) Schematic images of a homeotropic-aligned cell. (c) Photograph of a hybrid-aligned cell. (d) Photograph of a homeotropic-aligned cell. (e) Polarized optical micrograph of a hybrid-aligned cell on a rotatable stage. (f) Polarized optical micrograph of a homeotropic-aligned cell on a rotatable stage. Optically transparent film with hybrid alignment is confirmed from photograph and conoscopic images with a polarized optical microscope. Reprinted with permission from Wang et al. [Citation52]. Copyright 2015. The authors licensed under CC BY 4.0.](/cms/asset/e8f09299-3b68-4549-8ca2-40a7638ff336/gmcl_a_2360220_f0008_c.jpg)
Usui et al. [Citation53] demonstrated that threshold light intensity for molecular reorientation decreased by modifying a substrate surface in PSLCs. The surface of the glass substrate was treated with various concentrations of a silane coupler, which induces the initial homeotropic orientation of LCs. The cells treated with a 0.003 wt% silane coupler solution showed the lowest threshold intensity (4.9 W cm2), 30% lower than that treated with a 0.15 wt% silane coupler solution. This means that the threshold intensity increased with the concentration of the silane coupler, which can increase surface anchoring. This indicates that a reduced threshold intensity is caused by a reduction in surface anchoring that controls the initial alignment of PSLC molecules.
A series of our research indicates that an increase in optical torque and decreases in elastic torque and surface anchoring are effective ways to lower the threshold. Considering that the LC is dielectric, the optical torque is enhanced by an electric field. Usui et al. [Citation54] designed a susceptible LC system with the assistance of an electric field. The PSLC cell was prepared by replacing one glass substrate with a glass with a comb-shaped indium-tin oxide electrode. An alternating current (AC) electric field with a 100 Hz frequency was applied to the PSLC cell to generate an electric field in the in-plane direction parallel to the reorientation direction. The threshold values decreased with increasing the applied AC voltage. An electric field application of 6.5 V to the PSLC cell resulted in the lowest threshold intensity of 170 mW/cm2. This value is the lowest threshold previously reported in oligothiophene-doped LC systems. The possible mechanisms for lowering the threshold are the following: the application of external voltage generates the electric-induced torque that rotates the directors parallel to the direction of the electric field and thus decreases the photoinduced torque required to reorient molecules, which decreases the light intensity for exceeding threshold intensity.
Various approaches have been reported to develop new LC materials showing a low threshold intensity for nonlinear molecular reorientation. Although the threshold intensity has been successfully reduced, the nonlinear molecular reorientation was explored only with a focused linearly polarized laser beam. It is supposed that various light sources can induce the NLO response of oligothiophene dye-doped LCs. In that case, we can provide a powerful pathway for all-optical devices. Matsumoto et al. [Citation55] reported that a depolarized laser beam composed of polarization patterns could induce nonlinear molecular reorientation. A linearly polarized beam was modulated by a depolarizer, in which a spatial grating retardation pattern periodically changes the polarization direction of the depolarized beam. The depolarized laser beam was split into two beams at the focal point. Two diffraction rings appeared on the screen, and the number of rings increased with light intensity. The threshold intensities of the focused depolarized laser beams were approximately two times higher than that of the linearly polarized laser beam. This may be due to the randomized polarization of the focused depolarized laser beams at the focal point. Such polarization direction induces the random optical-electric torque direction, leading to the increase in threshold intensity.
Furthermore, Matsumoto et al. [Citation56] reported that the irradiation of oligothiophene-doped PSLCs with an unfocused laser beam (collimate beam, diameter: 700 µm ()) also caused nonlinear molecular reorientation. The threshold intensity irradiated with the collimated beam was lower than that of a conventional focused laser beam whose diameter is 50 µm. A decrease in the bulk elasticity might reduce the threshold intensity, resulting in a sensitive NLO effect of dye-doped PSLCs by applying the collimated beam.
Figure 9. Beam profiles of collimated and focused beams. Blue and gray lines are the collimated and focused beams, respectively. Reprinted with permission from Matsumoto et al. [Citation56].
![Figure 9. Beam profiles of collimated and focused beams. Blue and gray lines are the collimated and focused beams, respectively. Reprinted with permission from Matsumoto et al. [Citation56].](/cms/asset/41a97cae-d4ee-4b4d-a6a0-33367980ffc2/gmcl_a_2360220_f0009_c.jpg)
5. Optical function of oligothiophene-doped LCs
LC is a soft functional material with optical anisotropy such as refractive index and absorbance. Thus, our group has demonstrated the application of optical devices that utilize birefringence modulation and absorption change in oligothiophene-doped LCs.
Yaegashi et al. [Citation57] fabricated the microlens array with desired patterns and polarization selectivity only by irradiation with polarized light. Samples were prepared by adding a small amount of photoinitiator with absorption at 488 nm to an LC-LC monomer mixture. The mixture doped with a small amount of oligothiophene dye (TD1) was irradiated with a linearly polarized beam with a wavelength of 488 nm. In this method, the molecular reorientation of LC and the photopolymerization could be brought about simultaneously with a single beam, which fabricated microlens arrays. shows the POM images and polarization properties of the prepared microlens arrays. The fabricated microlens arrays became dark when rotated by 45° under POM observation (). The vivid black spot based on the reflection of microlens arrays disappeared when the parallel polarizers are perpendicular to the incident polarization direction (). In addition, the focusing ability was lost when light with perpendicular polarization was incident (). Furthermore, the adjustment of incident light intensity allowed for the control of the microlens’ focal length. These results clearly indicate that these microlens arrays were polarization-selective, reflecting the LC molecules’ alignment. Mejia et al. [Citation58] fabricated a microlens array with a tunable surface topography and focal length under heat treatment. The fabricated microlens array had no polarization-selectivity because the molecular reorientation was due to thermal distortion, i.e., order-to-disorder reorientation. The top views of the surface topographical structure of the microlens array are shown in . The surface topographical structure of the microlens array became smaller and more uniform in shape when the temperature was increased above the TNI of the host 5CB (TNI = 35.3 °C) and recovered when cooled down to room temperature. This indicates that the topographical change in the microlens array was due to the nematic-to-isotropic phase transition of 5CB and the thermal expansion of the polymer network. Furthermore, the focal length of the microlens array was thermally tuned reversibly.
Figure 10. POM images and polarization properties of the prepared microlens arrays. (a, b) POM images of the prepared microlens array. Crossed polarizers (white arrows) were rotated by 45° (a) and 0° (b) to the polarization direction of the 488 nm beam (blue arrow). (c, d) POM images of the prepared microlens array observed with polarized light parallel (c) and perpendicular (d) to the polarization direction of the 488 nm beam (blue arrow). An analyzer was set parallel to the polarizer. (e, f) Focusing properties of the prepared microlens array observed with polarized light parallel (e) and perpendicular (f) to the polarization direction of the 488 nm beam (blue arrow). An analyzer was set perpendicular to the polarizer. Reprinted with permission from Yaegashi et al. [Citation57].
![Figure 10. POM images and polarization properties of the prepared microlens arrays. (a, b) POM images of the prepared microlens array. Crossed polarizers (white arrows) were rotated by 45° (a) and 0° (b) to the polarization direction of the 488 nm beam (blue arrow). (c, d) POM images of the prepared microlens array observed with polarized light parallel (c) and perpendicular (d) to the polarization direction of the 488 nm beam (blue arrow). An analyzer was set parallel to the polarizer. (e, f) Focusing properties of the prepared microlens array observed with polarized light parallel (e) and perpendicular (f) to the polarization direction of the 488 nm beam (blue arrow). An analyzer was set perpendicular to the polarizer. Reprinted with permission from Yaegashi et al. [Citation57].](/cms/asset/fc21c3e2-1e93-443f-99ea-ce3fc80ca7f2/gmcl_a_2360220_f0010_c.jpg)
Figure 11. Top-view micrographs of the microlens array with topographical changes at various temperatures. The scale bar is 50 µm. Reprinted with permission from Mejia et al. [Citation58]. Copyright 2022. The authors licensed under CC BY 4.0.
![Figure 11. Top-view micrographs of the microlens array with topographical changes at various temperatures. The scale bar is 50 µm. Reprinted with permission from Mejia et al. [Citation58]. Copyright 2022. The authors licensed under CC BY 4.0.](/cms/asset/59e39beb-bf6e-4788-a1a6-d08a5d33b8b6/gmcl_a_2360220_f0011_c.jpg)
Usui et al. [Citation54] reported that the nonlinear molecular reorientation of oligothiophene-doped PSLC systems could act as optical limiters. When the linearly polarized light was normally incident to the LC cells, the transmittance stayed the same in the low-intensity region. However, the irradiation with a high-intensity beam above the threshold significantly decreased the transmittance. Our group revealed that this nonlinear transmittance change is induced by molecular reorientation. The reoriented TR5 molecules parallel to the polarization direction of the incident laser beam enhanced the light absorption due to their dichroism, and subsequently, the transmittance decreased nonlinearly. Furthermore, the oligothiophene-doped LC system can act as a deformable optical limiter by replacing the glass substrates with a highly transparent cycloolefin polymer film (COP). shows a photograph of a largely bent optical limiter with PSLCs sandwiched with flexible COP films. Conoscopic POM images confirmed that the original homeotropic orientation in the PSLC film was maintained during the bending procedure. Furthermore, the diffraction rings due to the nonlinear molecular reorientation were observed even in a bent state. These indicate that the fluidity of LCs prevents the destruction of the optical functional layer under applied stress. Yokota et al. [Citation59] reported that the host LC structures can control the threshold intensity of the optical limiting behavior. The molecular structures of host LCs and the mixing ratio of the compounds were the same as in the previous report (). The threshold intensity of the LC systems that contain the trifluoro-substituted LCs was lower than that of TR5/5CB. The reduction of the threshold intensity can be explained by the changes in the elastic torque related to K33 elastic constants.
Figure 12. Molecular reorientation behavior of the deformable TR5/PSLC optical limiter in the largely bent state. (a) Image of the deformable optical limiter based on TR5/PSLC sandwiched by COP substrates. (b) Formation of diffraction rings through the bent TR5/PSLC film that added an applied strain of 40% by the jig. Reprinted with permission from Usui et al. [Citation54]. Copyright 2015. The authors licensed under CC BY-NC-ND 4.0.
![Figure 12. Molecular reorientation behavior of the deformable TR5/PSLC optical limiter in the largely bent state. (a) Image of the deformable optical limiter based on TR5/PSLC sandwiched by COP substrates. (b) Formation of diffraction rings through the bent TR5/PSLC film that added an applied strain of 40% by the jig. Reprinted with permission from Usui et al. [Citation54]. Copyright 2015. The authors licensed under CC BY-NC-ND 4.0.](/cms/asset/e33835f1-1017-481d-959b-60fbfb463a8e/gmcl_a_2360220_f0012_c.jpg)
6. Conclusion
Our group has contributed to the recent development of advanced dye-doped LC materials with low threshold intensity for NLO response. We designed an oligothiophene dye molecule with an effective π-conjugated system. This led to a two-orders-magnitude lower threshold intensity in TR5-doped LC than in undoped LC. The nonlinear opticality was enhanced in terms of material design, substrate surface modification, and electric field assistance. Our series of such studies has successfully demonstrated that nonlinear molecular reorientation of dye-doped LCs can be induced with light intensity in the order of mW/cm2. In addition, the nonlinear response was induced by a depolarized and collimated beam. Furthermore, we demonstrated that oligothiophene-doped LC systems could be applied to microlens array and light dimming. Our guest-host system is a promising NLO material applied to all optical devices.
Disclosure statement
No potential conflict of interest was reported by the author(s).
Additional information
Funding
References
- L. R. Dalton, P. A. Sullivan, and D. H. Bale, Chem. Rev. 110 (1), 25 (2010). DOI: 10.1021/cr9000429.
- R. A. Kumar, J. Chem. 2013, 1 (2013). DOI: 10.1155/2013/154862.
- M. Pawlicki et al., Angew. Chem. Int. Ed. 48 (18), 3244 (2009). DOI: 10.1002/anie.200805257.
- S. H. Park, D. Yang, and K. Lee, Laser Photon. Rev. 3 (1–2), 1 (2009). DOI: 10.1002/lpor.200810027.
- M. Peccianti, and G. Assanto, Phys. Rep. 516 (4–5), 147 (2012). DOI: 10.1016/j.physrep.2012.02.004.
- J. M. Dudley, G. Genty, and S. Coen, Rev. Mod. Phys. 78 (4), 1135 (2006). DOI: 10.1103/RevModPhys.78.1135.
- T. Sasaki et al., Mater. Sci. Eng. R 30 (1–2), 1 (2000). DOI: 10.1016/S0927-796X(00)00025-5.
- S. K. Yesodha, C. K. S. Pillai, and N. Tsutsumi, Prog. Polym. Sci 29 (1), 45 (2004). DOI: 10.1016/j.progpolymsci.2003.07.002.
- I. C. Khoo, Phys. Rep. 471 (5–6), 221 (2009). DOI: 10.1016/j.physrep.2009.01.001.
- A. S. Zolot’ko et al., JETP Lett 32 (2), 158 (1980). DOI: 10.1007/978-94-011-3180-3_10.
- B. Y. Zel’dovich et al., JETP Lett 31 (5), 263 (1980). https://link.springer.com/journal/11448
- S. D. Durbin, S. M. Arakelian, and Y. R. Shen, Phys. Rev. Lett. 47 (19), 1411 (1981). DOI: 10.1103/PhysRevLett.47.1411.
- G. Barbero, and F. Simoni, Appl. Phys. Lett. 41 (6), 504 (1982). DOI: 10.1063/1.93587.
- G. Barbero, F. Simoni, and P. Aiello, J. Appl. Phys. 55 (2), 304 (1984). DOI: 10.1063/1.333098
- F. Simoni, and R. Bartolino, Opt. Commun. 53 (3), 210 (1985). DOI: 10.1016/0030-4018(85)90333-5.
- N. V. Tabiryan, A. V. Sukhov, and B. Y. Zel’dovich, Mol. Cryst. Liq. Cryst. 136 (1), 1 (1986). DOI: 10.1080/00268948608074569.
- L. Lucchetti et al., Opt. Commun. 233 (4–6), 417 (2004). DOI: 10.1016/j.optcom.2004.01.057.
- I. C. Khoo et al., Opt. Lett. 23 (4), 253 (1998). DOI: 10.1364/OL.23.000253.
- I. C. Khoo et al., Opt. Express. 4 (11), 432 (1999). DOI: 10.1364/OE.4.000432.
- W. M. Gibbons et al., Nature 351 (6321), 49 (1991). DOI: 10.1038/351049a0.
- H. He, and B. Xu, Bull. Chem. Soc. Jpn. 91 (6), 900 (2018). DOI: 10.1246/bcsj.20180076.
- I. C. Khoo, H. Li, and Y. Liang, IEEE J. Quantum Electron. 29 (5), 1444 (1993). DOI: 10.1109/3.236160.
- P. Wu et al., Appl. Opt. 42 (22), 4560 (2003). DOI: 10.1364/AO.42.004560.
- C. Y. Wang et al., Sci. Rep. 6 (1), 30873 (2016). DOI: 10.1038/srep30873.
- S. W. Oh, S. H. Kim, and T. H. Yoon, Sol. Energy Mater. Sol. C 183, 146 (2018). DOI: 10.1016/j.solmat.2018.04.019.
- L. C. Lin et al., Opt. Express. 15 (6), 2900 (2007). DOI: 10.1364/OE.15.002900.
- H. Zeng et al., Adv. Mater. 29 (30), 1701814 (2017). DOI: 10.1002/adma.201701814.
- I. Jánossy, A. D. Lloyd, and B. S. Wherrett, Mol. Cryst. Liq. Cryst. 179 (1), 1 (1990). DOI: 10.1080/00268949008055354.
- L. Marrucci, and D. Paparo, Phys. Rev. E 56 (2), 1765 (1997). DOI: 10.1103/PhysRevE.56.1765.
- I. Jánossy, Phys. Rev. E 49 (4), 2957 (1994). DOI: 10.1103/PhysRevE.49.2957.
- L. Marrucci, Liq. Cryst. Today 11 (3), 6 (2002). DOI: 10.1080/13583140260230264.
- I. Jánossy, and A. D. Lloyd, Mol. Cryst. Liq. Cryst. 203 (1), 77 (1991). DOI: 10.1080/00268949108046047.
- R. Muenster et al., Phys. Rev. Lett. 78 (1), 42 (1997). DOI: 10.1103/PhysRevLett.78.42.
- A. A. Rodríguez-Rosales et al., Opt. Mater. 31 (2), 350 (2008). DOI: 10.1016/j.optmat.2008.05.006.
- I. Jánossy, and T. Kósa, Opt. Lett. 17 (17), 1183 (1992). DOI: 10.1364/OL.17.001183.
- S. Residori, and A. Petrossian, Mol. Cryst. Liq. Cryst. 398 (1), 137 (2003). DOI: 10.1080/15421400390221574.
- A. Jafari, H. Tajalli, and A. Ghanadzadeh, Laser Phys. 16 (8), 1213 (2006). DOI: 10.1134/S1054660X06080093.
- A. Miniewicz et al., Adv. Mater. Opt. Electron. 10 (2), 55 (2000). DOI: 10.1002/1099-0712(200003/04)10:2<55::AID-AMO398>3.0.CO;2-Y
- M. Kreuzer et al., Phys. Rev. Lett. 88 (1), 013902 (2001). DOI: 10.1103/PhysRevLett.88.013902
- L. Marrucci et al., J. Chem. Phys. 107 (23), 9783 (1997). DOI: 10.1063/1.475276
- L. Marrucci et al., J. Chem. Phys. 113 (22), 10361 (2000). DOI: 10.1063/1.1322624
- W. Liptay et al., Naturforsch 26 (12), 2020 (1971). DOI: 10.1515/zna-1971-1211.
- H. Zhang et al., Adv. Mater. 12 (18), 1336 (2000). DOI: 10.1002/1521-4095(200009)12:18<1336::AID-ADMA1336>3.0.CO;2-A
- H. Meier, U. Stalmach, and H. Kolshorn, Acta Polym. 48 (9), 379 (1997). DOI: 10.1002/actp.1997.010480905
- D. R. Maulding, and B. G. Roberts, J. Org. Chem. 34 (6), 1734 (1969). DOI: 10.1021/jo01258a045.
- S. D. Durbin, S. M. Arakelian, and Y. R. Shen, Opt. Lett. 6 (9), 411 (1981). DOI: 10.1364/OL.6.000411.
- M. Yaegashi et al., Chem. Mater. 17 (17), 4304 (2005). DOI: 10.1021/cm0503580.
- Y. Aihara et al., Adv. Opt. Mater 1 (11), 787 (2013). DOI: 10.1002/adom.201300326.
- J. Wang et al., Opt. Mater. Express 5 (3), 538 (2015). DOI: 10.1364/OME.5.000538.
- J. C. Mejia et al., Mater. Adv. 3 (20), 7531 (2022). DOI: 10.1039/D2MA00774F.
- J. Yokota et al., Materials 15 (12), 4125 (2022). DOI: 10.3390/ma15124125.
- J. Wang et al., Sci. Rep. 5 (1), 9890 (2015). DOI: 10.1038/srep09890
- K. Usui et al., Polym. J. 49 (1), 209 (2017). DOI: 10.1038/pj.2016.102
- K. Usui et al., ACS Appl. Mater. Interfaces 13 (19), 23049 (2021). DOI: 10.1021/acsami.1c06951
- K. Matsumoto et al., J. Photopolym. Sci. Technol. 33 (1), 77 (2020). DOI: 10.2494/photopolymer.33.77
- K. Matsumoto et al., Mol. Cryst. Liq. Cryst. 713 (1), 46 (2020). DOI: 10.1080/15421406.2020.1856532
- M. Yaegashi et al., Adv. Mater. 19 (6), 801 (2007). DOI: 10.1002/adma.200601329
- J. C. Mejia et al., Appl. Sci. 12 (15), 7901 (2022). DOI: 10.3390/app12157901
- J. Yokota et al., Proc. SPIE 12658, 1265805 (2023). DOI: 10.1117/12.2674372.