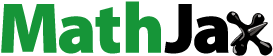
ABSTRACT
A large amount of chemicals, water and energy are used during the bleaching of raw cotton fabrics. For this reason, it is extremely important for economic and ecological aspect to develop eco-friendlier bleaching technology that eliminates the need for heat energy, process water, and wetting agent in the bleaching of these fabrics. Here, we report on green approach for industrial-scale bleaching with sodium chlorite (NaClO2) in presence of phosphonates (HEDP), which is used as both wetting agent and pH regulator and contributes to the high whiteness of fabrics. In bleaching, the process water was used in ambiant temperature as flote without any heating process, thus reducing the energy requirement significantly. In the measurements, it was observed that the whiteness value (W.I. = 72.6) obtained after 8.0 h bleaching at 20°C which was very close to the whiteness value (W.I. = 74.2) of reference W-1 fabric. Also, it has been confirmed that cotton fabrics bleached with NaClO2 have higher strength values, less damage, and residue on the fabric compared to reference fabrics, as well. This study showed that cotton fabrics can generate a small amount of wastewater and can be successfully bleached in the presence of NaClO2 without the need for any heating energy.
摘要
原棉织物漂白过程中需要大量的化学物质、水和能量. 因此, 从经济和生态方面来说, 开发更环保的漂白技术是极其重要的, 该技术消除了这些织物漂白过程中对热能、工艺水和润湿剂的需求. 在这里, 我们报道了在膦酸盐 (HEDP) 存在下用亚氯酸钠 (NaClO2) 进行工业规模漂白的绿色方法, 该方法既用作润湿剂又用作pH调节剂, 有助于织物的高白度. 在漂白过程中, 工艺水在环境温度下作为浮石使用, 无需任何加热过程, 从而显著降低了能量需求. 在测量中, 观察到在20°C下漂白8.0小时后获得的白度值 (W.I. = 72.6) 非常接近参考W-1织物的白度 (W.I = 74.2). 此外, 已经证实, 与参考织物相比, 用NaClO2漂白的棉织物具有更高的强度值、更少的损伤和织物上的残留物. 这项研究表明, 棉织物可以产生少量废水, 并且可以在NaClO2存在下成功漂白, 而不需要任何热能.
Introduction
The world’s population is expected to increase by around 30% by 2050. This population growth will lead to a significant increase in textile production and consumption, which will significantly increase the textile industry’s energy and water use and emissions of carbon dioxide and other harmful emissions to the environment (CO2) (Atav et al. Citation2021; Hasanbeigi and Price Citation2015). Due to the increasing demand for textile products, reducing energy use and CO2 emissions requires innovation in addition to existing technologies.
Cotton fabric, which is the most popular of the cellulosic textile materials, constitutes about half of the fabric sector in recent years (Liu, Liu, and Fang Citation2022; Peng et al. Citation2018). The main reasons why cotton fabrics are so preferred are their sustainability, low environmental concerns, and biocompatibility (Andra et al. Citation2021; Kim et al. Citation2017). While 90% of cotton fabrics consist of cellulose, 10% consists of impurities such as protein, pectin, minerals, and wax (Bogard et al. Citation2021; Farrell, De Boskey, and Ankeny Citation2016). Even though pectin and wax have positive effects on cotton, it significantly reduces the wettability of cotton by preventing water adsorption (Xia et al. Citation2021). Considering these results, dyeing fabrics without removing impurities present in cotton fabric is difficult for the dyeing industry. In commercial bleaching processes, bleaching processes are usually carried out at high alkalinity at high temperatures to achieve the required whiteness of the fabrics. This can cause significant damage to cotton fibers as well as significant energy consumption (Wang et al. Citation2020). Bleaching of cellulose material with hydrogen peroxide is generally carried out under alkaline conditions at extreme temperatures, resulting in greater energy consumption and fiber damage (Liu, Zhang, and Yan Citation2018). The processes to remove these impurities in cotton fabrics are very important in order not to damage the cotton structure and to ensure its whiteness. The hydrogen peroxide bleaching process, which is the most used to remove the natural pigments from cotton and cotton blends, requires high water consumption and energy input, as well as an alkaline environment, stabilizer, and long incubation time at high temperatures. This process results in damage to the cotton fibers and decreases product quality, as well. For this reason, enzyme-mediated systems are considered better alternatives, and there are remarkable studies on the bleaching of cotton fabric by enzymatic methods in the literature. Tülek et al. worked with MmLac cloned and heterologously expressed in P. pastoris for the removal of specific synthetic dyes and in the treatment of textile effluent (Tülek et al. Citation2021). Subsequently, they investigated their performance in bleaching cotton fabrics by using the materials MmLac, silica@MmLac/ZIF-8 and silica@Glu-MmLac/ZIF-8 produced by them. As a result of these studies, silica@MmLac/ZIF-8 and silica@Glu MmLac/ZIF-8 concluded that laccase-based biocatalysts are promising for biotechnology (Tülek et al. Citation2021). In the Mojsov study, treatment of cotton terry fabrics with alkaline pectinase enzyme and properties such as whiteness and weight loss after abrasion were investigated. It was determined that cotton fabrics treated with enzymatic treatment were less damaged and needed less energy and water (Mojsov Citation2018). These processes consist of two pre-treatment processes, namely scrubbing and bleaching (Wang et al. Citation2020). However, natural fibers and fabrics are still yellowish and brownish in color even after scrubbing. The reason for these colors may be various environmental factors such as climate, soil, drought, and frost (Amutha et al. Citation2022; Vörösmarty et al. Citation2010). The purpose of the bleaching step is to remove the coloring matter via bleaching chemicals and as a result, to obtain white fabrics.
Sodium chlorite is one of the most important bleaching chemicals, as it ensures that the textile material it is treated with exhibits low loss of strength and a high degree of whiteness (Forte-Tavčer Citation2010; Kramar, Ivanovska, and Kostić Citation2021). In addition, sodium chlorite is easily obtained and is relatively stable in storage. In addition, sodium chlorite is a stable free radical that acts as a one-electron oxidant in reactions with reducing substrates such as amines, sulfites, and phenols (Jangam and Richardson Citation2010; Kawata et al. Citation2021). However, sodium chlorite, like other bleaching agents, is either relatively environmentally harmful or shows high energy use during bleaching (Hasanbeigi and Price Citation2015). For instance, when bleaching cotton fabric with the aid of sodium chlorite, the higher the ambient temperature, the higher the rate of decomposition of sodium chlorite as a strong oxidizer (Toshikj et al. Citation2019). However, this results in relatively high energy use in the bleaching process.
In this study, an approach that can minimize energy consumption by performing the bleaching process of cotton fabrics at process water temperature (20 ± 5°C) without the need for additional heat is presented. In addition, the chemical consumption in the bleaching process was tried to be reduced by using HEDP chemical as both pH regulator and wetting agent. It was observed that the whiteness value obtained as a result of bleaching using sodium chlorite at 20 ± 5°C was close to the whiteness value obtained using hydrogen peroxide in a high temperature environment. The study is also remarkable in terms of obtaining high quality and tenacity cotton fabrics with sufficient whiteness value.
Experimental
Equipment and materials
Orbital shaker of LBX Orb-Pro was used for bleaching. The orbital shaker descriptions were as follow: timer function (1 min–19 h 59 min), The speed is adjustable between 100 and 500 rpm, an orbital diameter of 10 mm and a load capacity of 7.5 kg. The whiteness of the bleached samples was measured as reflectance by the Konica Minolta Spectrophotometer, CM-3600d. Mettler Toledo S220-K table top pH meter was used for pH measurements. Cotton samples were examined using a scanning electron microscope (SEM, Hitachi S-4700). A Shimadzu brand analytical balance (AUX320 Analytical Balance, 320 g/0.1 mg) was used to calculate the weight loss of fabrics as a result of bleaching. Cotton fabric was coated with a sputter machine (cressington 108 auto sputter coater). Fourier transform infrared spectroscopy (FT-IR, VERTEX-70 Bruker) was used to determine the chemical bonds of the fabrics and whether the impurities contained in the fabrics were successfully removed by bleaching. Measurements were made at a resolution of 4 cm−1 in the range of 4000–400 cm−1. The 100% cotton fabric used in the studies was supplied by NUR Textile Co., Turkey. The fabric has 150 g/m2 weight, 30 yarn/cm weft, and 36 yarn/cm warp properties.
Chemicals
Phosphoric acid (H3PO4, 85% pure), citric acid (C₆H₈O₇, 99% pure), acetic acid (C2H4O2, 99% pure), and formic acid (CH₂O₂ reagent grade, ≥96%) used for pH adjustment were obtained from Merck Chemical Company. Potassium iodide (KI, reagent grade, ≥99%), sulfuric acid (H2SO4, 96%), sodium thiosulfate (Na2S2O3, reagent grade, ≥99%), and hydrogen peroxide solution (H2O2, 30%) were obtained from Sigma Aldrich Chemical Company. Sodium chlorite was supplied as a 31% solution in water by Turoksi Chemical Co. 1-hydroxy ethylidene-1,1-diphosphonic acid (HEDP) used for wetting agent and pH adjustment and Nonylphenol (NP-10) used for wetting agent were supplied by the Turkish domestic market as technical grade.
Bleaching
Process conditions
An average of 10 g was cut from a roll of raw cotton fabric for each bleaching experiment. The cut fabric was soaked in a liquor solution. Liquids consist of sodium chlorite, a wetting agent (optional), and different acids. The fabric/liquor ratio specified in the recipes is 1/10. 5.25 g/L NaClO2, (0–1 g/L) wetting agent was used in the experiments. NP-10 was used as a wetting agent. The pH of the liquids was adjusted (2.5–11.0) by adding phosphoric acid (PA), citric acid (CA), acetic acid (AA), formic acid (FA), and 1-hydroxy ethylidene-1,1-diphosphonic acid (HEDP) acids. HEDP is used as both a pH regulator and a wetting agent. The resulting solution and cotton fabric were transferred into a 200 mL beaker. Beakers were fixed on the orbital shaker. Then, the orbital shaker rotation speed of 100 rpm was adjusted and fabric bleaching was carried out at different times (). At the end of the period, the fabrics taken from the beaker were rinsed with tap water. Then, the fabric was left to dry at ambient temperature. Further research has been carried out with bleached fabrics and their process waste.
Preparation of reference fabrics
In this study, experimental fabrics bleached with NaClO2 were compared with reference fabrics bleached with H2O2 under two different conditions. With the approach of the textile industry, Witness-1 (W-1) and Witness-2 (W-2) reference cotton fabrics were given, respectively, for the reference of bleached fabric before dyeing and the reference of fabric showing exact whiteness. 6 g/L H2O2 was used for bleaching the W-1 fabrics. NaOH was used to adjust the pH in bleaching performed at pH = 10.5. Non-ionic NP-10 was used as a wetting agent and bleaching duration was determined as 2 h. In the bleaching of the W-2 fabrics, unlike in the bleaching process of the W-1 fabric, 4 g/L H2O2 was used and the bleaching time was determined as 1 h.
Measurements and analysis
Sodium chlorite (NaCIO2) analysis
A sample of 0.25 g was taken into the erlenmeyer with a pipette for sodium chlorite analysis. 50 mL of distilled water was added to the substance. Then, 20 mL of KI (100 g/L) and 10 mL of H2SO4 (50 g/L) were added, respectively. The Erlenmeyer containing the sample to be analyzed was kept in the dark for 10 min. Titration was performed with 0.1 M sodium thiosulfate after adding a few drops of starch indicator. The point at which the solution turned a light blue-black color was recorded as a turning point. C8 is the sodium chlorite (NaClO2) content, as a percentage by mass given by the formula:
where V1 is the spent sodium thiosulfate (mL), c is the concentration of sodium thiosulfate solution (M) and m is the test sample weight (g).
Weight loss
The weight loss of the fabric after the bleaching process was calculated on a dry weight basis using the following formula:
where W1 and W2 are the dry weights of the cotton fabric before and after bleaching treatment, respectively.
Degree of whiteness
In the whiteness measurements, the whiteness degrees of the reference samples (W-1 and W-2) bleached with H2O2 and the samples bleached with NaClO2 using different combinations in this study were investigated. The whiteness indexes (W.I.) of bleached cotton fabrics were measured using a Konica Minolta spectrophotometer (CM-3600d). Whiteness indexes were calculated in terms of CIE Y (green) and (blue) reflectance components using the ASTM Method E31373 equation.
where Y and Z are the readings of the device.
Tensile strength (RT)
Tensile strength of cotton samples was determined by the strip method according to ASTM procedure D 2256-66T.
SEM analysis of cotton samples
Scanning electron microscopy (SEM, Hitachi S-4700) was used to obtain information about the microstructures of the raw cotton fabric, the reference (W-1) sample bleached with H2O2 and the cotton fabric with the highest whiteness index bleached with NaClO2, and to observe the differences before and after bleaching. Before SEM analysis, the samples were coated with a Cressington 108 automatic spray coater device. The diameter and size distribution of cotton fibers were determined using Image J software.
Results and discussion
Since it is known that NaClO2 shows better bleaching properties under acidic conditions, the effect of acid species on whiteness was first investigated at different pH values (pH = 2.5, 3.0, 3.5, 4.0 and 4.5). The acid types used are phosphoric acid (PA), citric acid (CA), acetic acid (AA), formic acid (FA), and 1-hydroxy ethylidene-1,1-diphosphonic acid (HEDP). The results of these examinations are given in .
Figure 2. (a) The effect of different pH values and different acid types on the whiteness of cotton fabric, and (b) Comparison of the whiteness index of the bleaching processes using HEDP at different pH values with the reference (W-1 and W-2) fabrics.
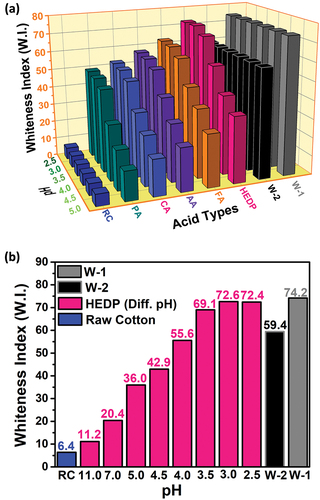
Among these acid types, HEDP acid gave the best results, and the rest of the study was carried out on this acid. After determining the acid to be used, bleaching studies were carried out at different pH values (2.5, 3.0, 3.5, 4.0, 4.5, 5.0, 7.0, and 11.0) to determine the optimum pH and the results were given in the . It was determined that the pH value giving the best whiteness value (W.I. = 72.6) was 3.0. Used during the bleaching process; Information such as the amount of bleach, the name of the pH regulator, the liquor ratio, and the temperature value was given in in detail. One of the most important advantages of this study is that no wetting agent is used in bleaching, and HEDP acts as both an acid regulator and a wetting agent.
Table 1. Determination of optimum pH in cotton bleaching.
While there was an increase in the whiteness value as the pH value decreased, this acceleration deviated only at pH = 2.5. The lower the pH value in bleaching processes, the higher the bleaching success (Hubbell and Ragauskas Citation2010). Chloric acid (HClO2) used in bleaching is an unstable acid. Therefore, it decomposes into chlorine dioxide (ClO2), which is the active species during bleaching. However, when a pH below 3.0 is used, it causes a decrease in the whiteness value as it will cause a decrease in oxygen formation in the chlorite, which plays an active role in bleaching. This explains the decrease in whiteness value at pH = 2.5. In order to better understand the effect of pH on bleaching, changes in sodium chlorite concentration in the presence of different pH values were investigated using a UV-visible spectrophotometer (). The absorption peaks at 260 nm and 360 nm in the figure belong to NaClO2 and ClO2, respectively (Yang et al. Citation2017). It is observed that as the pH value decreases, the concentration of NaClO2 decreases and the formation of ClO2 increases. This can be explained by the higher oxidation performance of the system with the increase of H+ in the bleaching process. However, as the pH decreases, NaClO2 can turn into toxic and corrosive chlorine dioxide gas. For this reason, it was desired to determine the optimum pH value that gives the appropriate whiteness value at different pH values (Hao et al. Citation2017). When the pH value drops to 2.5 and below, it turns into the above-mentioned undesirable gas. In addition, low pH values can damage cotton fabric. Considering these results, it was decided not to drop below pH = 3 in the bleaching process, and supports this situation. In this figure, the remaining NaClO2 content in the medium after bleaching at different pH values is given. It was determined that as the pH value decreased, the amount of NaClO2 decreased in direct proportion. While there was no significant change in NaClO2 concentration at high pH values, it was observed that NaClO2 decreased as the pH value decreased, that is, as the system became acidic (Gong and Li Citation2019).
Figure 3. Investigation of (a) the effect of pH change on sodium chlorite concentration by means of UV-visible spectrophotometer, and (b) the change in sodium chlorite content at different pH values in the presence of HEDP.
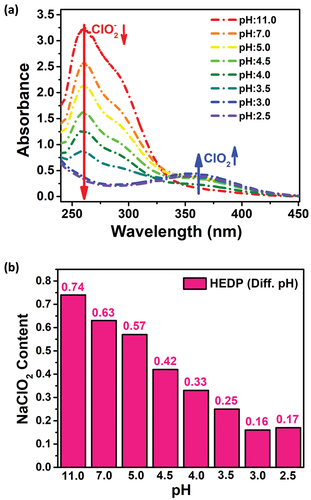
After the optimum pH value for bleaching was determined as 3.0, bleaching studies were carried out to determine the optimum duration. For this, bleaching studies were carried out at different times (0.5, 1.0, 2.0, 4.0, 8.0, 12.0, and 24.0 h) while keeping other conditions such as pH = 3, temperature = 20 ± 5°C constant ().
Figure 4. (a) Effect of different durations of bleaching on whiteness, and (b) Comparison of the weight loss of the fabric as a result of different bleaching times with the weight loss of the W-1 fabrics.
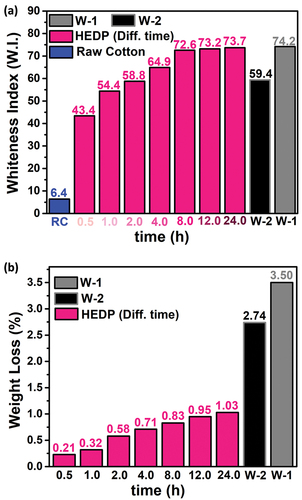
For this, bleaching studies were carried out at different times while keeping other conditions constant. It has been observed that fabrics produced after 0.5-h bleaching exhibit a low whiteness value (W.I. = 43.4). It was determined that there was a significant increase in the whiteness value of the fabric in bleaching from 1.0 h to 4.0 h. However, it was determined that there was no significant change in the whiteness value of the cotton fabric as a result of 8.0-h bleaching and 24.0-h bleaching time. The reaction of sodium chlorite with yellowish and brownish colored groups caused by different physical, chemical, and environmental factors in cotton fabric is similar to chemical reactions. For this reason, the bleaching process is almost completed within 8 h. Considering the measured whiteness values, it was decided that 8.0-h of bleaching time would be sufficient for bleaching cotton fabrics. The whiteness value (W.I. = 71.7) obtained after 8.0 h of bleaching is very close to the reference value (W.I. = 74.2) of the fabric used as a W-1 after bleaching with H2O2 at 90°C. In addition, the whiteness value of the cotton fabric bleached for 1.0 and 2.0 h is close to the reference value of the W-2 fabric bleached with H2O2 at 70°C, and it can be used easily in under dye fabrics. The parameters used to determine the optimum time in the bleaching process and the whiteness values at different times are given in .
Table 2. Determination of optimum duration in cotton bleaching.
One of the points to be considered in the bleaching process is the weight loss of the fabric after bleaching. For this reason, the weight losses that occur as a result of the bleaching processes carried out at different times are calculated and given in . As seen from the figure, it is seen that the weight loss of the fabric increases as the bleaching time increases. This can be explained by the removal of unwanted parts such as waxes, pectins, proteins, pigments, hemicelluloses, and other organic and inorganic impurities from the fabric as the bleaching process continues. In addition, the weight loss in the cotton fabric as a result of bleaching in the presence of NaClO2 is 1/3 of the weight loss in the presence of it. It is noteworthy that the weight loss when the whiteness values are close to each other is considerably lower than the weight loss with H2O2. The bleaching process performed with NaClO2 also provides an advantage with less weight loss on the fabric.
In , FE-SEM and high resolution FE-SEM images of unbleached raw cotton fabric and cotton fabrics bleached with H2O2 and NaClO2 were given. The FE-SEM image in belongs to the raw cotton fabric, and it is seen from the image that the fibers have homogeneous diameters. As a result of the measurements made from 40 different points on the FE-SEM image with the ImageJ program, the average diameter of the fibers was calculated to be 18 ± 6 µm. The size distribution diagram of the fibers was given in as an inset in figure. A high resolution FE-SEM image of the same fabric was given in . When looking at the image, it is observed that there are residues that can consist of waxes, pectin, proteins, pigments, hemicelluloses, and other organic and inorganic impurities, which are also indicated by pink arrows among the cotton fibers. shows the FE-SEM image of cotton fabric bleached with H2O2 to be used as a reference sample. After bleaching, the cotton fabric appears to be damaged, and there are some tears in the fibers (indicated by pink circles in the image). In addition, as a result of the measurement performed on the FE-SEM image, it was determined that the average fiber diameters were 13 ± 5 µm (the size distribution diagram of the fibers is given as an inside picture in ). This decrease in fiber diameters can be explained by the removal of organic and inorganic impurities in cotton fibers as a result of bleaching. High resolution FE-SEM image of the reference cotton fabric sample was given in . Looking at the image, it is observed that there are residues in the cotton fibers, which are also indicated by the pink arrows. It is observed that the residues cannot be removed to a large extent after bleaching with H2O2. In , the FE-SEM image of the experimental cotton fabric bleached with NaClO2 was given. Looking at the image, it is seen that the fibers have homogeneous diameters. As a result of the measurements made on the image, the average diameter of the fibers was found to be 16 ± 5 µm and the size distribution diagram was given in as an inside graph. While this value is smaller than the average diameter of raw cotton fibers, it is larger than the diameter of cotton fabric fibers bleached with H2O2. A slight reduction in fiber diameters may mean that the cotton fibers may not have suffered any damage as a result of bleaching. The high-resolution FE-SEM image in also supports this situation. In this figure, it is seen that the surface of the fibers is smoother than the reference fiber. In addition, it was observed that there was much less residue in the fabric bleached with NaClO2 compared to the FE-SEM images of raw and H2O2-bleached cotton fabrics. The results from all of the FE-SEM images match the results in the weight loss graph in .
Figure 5. FE-SEM images of samples of (a) raw fabric, (b) W-1 fabric bleached with H2O2, (c) experimental fabric bleached with NaClO2 (fiber size distribution histograms were given as inset in each figure) and high-magnification FE-SEM images of samples (d) raw fabric, (e) W-1 fabric bleached with H2O2, and (f) experimental fabric bleached with NaClO2.
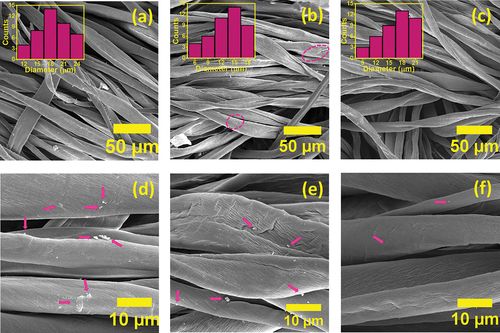
The FTIR spectra of cotton fabric raw and bleached with NaClO2 at 20 ± 5°C are given in . The peaks at 1594 and 1592 cm−1 in the spectra of both samples can be attributed to the stretching vibration of the conjugated carbonyl group. Likewise, the peaks at 1325 and 1321 cm−1 in both samples express the bending vibrations of the C-H groups. The peaks of raw cotton fabric at 1027 and 885 cm−1 and bleached cotton fabric at 1024 and 882 cm−1 are related to the ether bonds of cellulose (Bulut Citation2016; Zhao et al. Citation2010). The peak corresponds to the C=O stretching vibration of the aryl group in pectin. However, this peak is not in the spectrum of cotton fabric bleached with NaClO2. This can be explained by the removal of pectin from the fabric during bleaching. A similar situation can be said for the peak at 1155 cm−1 of the raw cotton sample. The fact that the peak at this frequency is not observed in the bleached sample can be explained by the deformation of the anti-symmetric C-O-C bond as a result of the bleaching process (Sangeetha, Varghese, and Nayak Citation2019).
The mechanical properties of fabrics are very important for their purposes and areas of use. For this reason, it is desirable that the bleached fabrics show sufficient strength. Considering this situation, the strengths of pure, H2O2 and NaClO2 bleached fabrics were measured and it is given in . In , how pH and time parameters affect the tenacity of the fabric is examined. As seen from the figure, it was observed that tenacity increased with the increase in pH, but decreased as the bleaching time increased. The sample with the highest tenacity was the unbleached raw cotton fabric sample, while the sample with the lowest tenacity was the W-1 sample, which was bleached with H2O2. It was determined that the tenacity value of the fabric sample exhibiting optimum bleaching with NaClO2 was significantly higher than the tenacity value of the W-1 sample. For a better understanding of the above, in , the relationship between whiteness value and tenacity is discussed in the graph. When the graph was examined, it was observed that there was an inverse proportion between the whiteness value and tenacity, and the tenacity decreased as the whiteness increased. The results of the measurements made to determine the physical properties of raw, reference and experimental fabrics bleached at different pH values (tenacity and percentage elongation at break) are given in . As seen from the table, fabrics that were bleached at low pH values and exhibited high whiteness gave relatively better percentage elongation results. It is seen that all these results obtained in the determination of physical properties coincide with the results of FE-SEM and FT-IR. It is an expected result that components such as cellulose and pectin in raw fabric fibers are removed from the fiber by the bleaching process and cause a decrease in fiber diameters, resulting in a decrease in tenacity and a relatively increase in percent elongation (Bulut Citation2016).
Figure 7. (a) The effect of different pH values and duration on tenacity of cotton fabric, and (b) Whiteness and tenacity values of fabrics bleached at different pH values via HEDP and NaClO2.
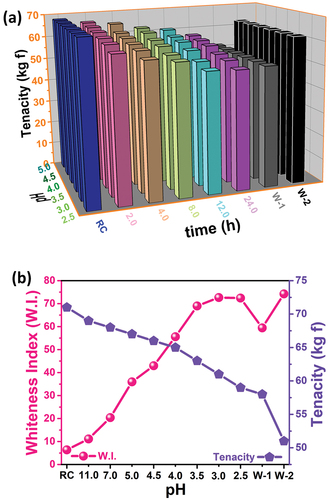
Table 3. Effect of pH on the physical properties and whiteness index of the bleached cotton fabric.
Conclusion
In this study, the effects of parameters such as pH regulator, pH, time, and bleach concentration on the bleaching of raw cotton fabric were investigated. HEDP gave the best whiteness value among the acids, and the rest of the study was carried out on this acid. HEDP acts as a wetting agent as well as being used as a pH regulator. After determining the acid to be used, bleaching studies were carried out at different pH values to determine the optimum pH, and the best whiteness value was obtained at pH = 3.0. In addition, the effect of pH on NaClO2 was investigated, and it was determined that as the pH value decreased, the percentage of NaClO2 decomposed by bleaching increased. Also, bleaching was done at different times to determine the optimum bleaching time. In the bleaching experiments carried out up to 8.0 h, it has been determined that the whitening speed of cotton fabrics is high, and the bleaching rate decreases significantly as the time gets longer, i.e., 24 h. Considering the whiteness values of the bleached fabrics, it was decided that 8.0 h of bleaching time would be sufficient for bleaching the cotton fabrics. The whiteness value (W.I. = 72.6) obtained at this bleaching time was found to be close to the whiteness value (W.I. = 74.2) of the reference W-1 fabric. In addition, it has been calculated that the weight loss of the fabric as a result of bleaching with NaClO2 is only 1/3 of that of the fabric bleached with H2O2. From the FE-SEM images, it was seen that the experimental fabric bleached with NaClO2 had larger fiber diameters, less residue, and caused less damage to cotton fibers than the reference fabric bleached with H2O2. In addition, the mechanical properties of the fabrics were examined, and it was determined that the strength of the fabric bleached with NaClO2 was better than the reference fabrics bleached with H2O2. In future studies, it is considered that biocomposite catalysts will be produced and used in the bleaching of cotton fabrics. In this way, it is aimed to obtain fabrics that are less damaged, have a higher whiteness value, and are more durable.
Highlights
The bleaching process used in the study is economical and ecological.
Since no heating process is applied, minimum energy is consumed.
Less chemicals are used thanks to functional phosphonate (HEDP).
Bleached fabrics exhibit high tenacity, whiteness and low residue formation.
Acknowledgements
The authors extend their appreciation to the TUROKSI Chemical Co. Ltd. for providing the cotton fabric and some chemicals.
Disclosure statement
No potential conflict of interest was reported by the author(s).
Additional information
Funding
References
- Amutha, K., S. G. Annapoorani, P. Sakthivel, and N. Sudhapriya. 2022. Ecofriendly dyeing of textiles with natural dyes extracted from commercial food processing waste materials. Journal of Natural Fibers 1–14. doi:10.1080/15440478.2021.1993506.
- Andra, S., J. Jeevanandam, M. Muthalagu, and M. K. Danquah. 2021. Surface cationization of cellulose to enhance durable antibacterial finish in phytosynthesized silver nanoparticle treated cotton fabric. Cellulose 28 (9):5895–910. doi:10.1007/s10570-021-03846-2.
- Atav, R., M. F. Yüksel, S. Özkaya, and B. Buğdaycı. 2021. The use of ozone technology for color stripping and obtaining vintage effect on reactive dyed cotton fabrics. Journal of Natural Fibers 1–11. doi:10.1080/15440478.2021.1982442.
- Bogard, F., T. Bach, B. Abbes, C. Bliard, C. Maalouf, V. Bogard, F. Beaumont, and G. Polidori. 2021. A comparative review of Nettle and Ramie fiber and their use in biocomposites, particularly with a PLA matrix. Journal of Natural Fibers 1–25. doi:10.1080/15440478.2021.1961341.
- Bulut, M. O. 2016. Low temperature bleaching for reactive dyeing and top white knitted cotton fabric. Journal of Cleaner Production 137:461–74. doi:10.1016/j.jclepro.2016.07.124.
- Farrell, M. J., M. J. De Boskey, and M. A. Ankeny. 2016. Improving the wettability of enzyme-bleached cotton fabric with inclusion of sodium surfactin. ACS Sustainable Chemistry & Engineering 4 (3):1569–72. doi:10.1021/acssuschemeng.5b01486.
- Forte-Tavčer, P. 2010. Impregnation and exhaustion bleaching of cotton with peracetic acid. Textile Research Journal 80 (1):3–11. doi:10.1177/0040517509104542.
- Gong, P., and X. Li. 2019. Promoting effect of H+ and other factors on NO removal by using acidic NaClO2 solution. Energies 12 (15):2966. doi:10.3390/en12152966.
- Hao, R., X. Wang, Y. Liang, Y. Lu, Y. Cai, X. Mao, B. Yuan, and Y. Zhao. 2017. Reactivity of NaClO2 and HA-Na in air pollutants removal: Active species identification and cooperative effect revelation. Chemical Engineering Journal 330:1279–88. doi:10.1016/j.cej.2017.08.085.
- Hasanbeigi, A., and L. Price. 2015. A technical review of emerging technologies for energy and water efficiency and pollution reduction in the textile industry. Journal of Cleaner Production 95:30–44. doi:10.1016/j.jclepro.2015.02.079.
- Hubbell, C. A., and A. J. Ragauskas. 2010. Effect of acid-chlorite delignification on cellulose degree of polymerization. Bioresource Technology 101 (19):7410–15. doi:10.1016/j.biortech.2010.04.029.
- Jangam, A., and D. E. Richardson. 2010. Epoxidation by sodium chlorite with aldehyde-promoted chlorine dioxide formation. Tetrahedron letters 51 (50):6481–84. doi:10.1016/j.tetlet.2010.09.102.
- Kawata, H., M. Kohno, K. Nukina, I. Horiuchi, H. Goda, T. Kuwahara, K. Yoshimori, A. Miyaji, T. Kamachi, and T. Yoshikawa. 2021. Identifying the chloroperoxyl radical in acidified sodium chlorite solution. Plos One 16 (5):e0252079. doi:10.1371/journal.pone.0252079.
- Kim, Y., L. T. McCoy, E. Lee, H. Lee, R. Saremi, C. Feit, I. R. Hardin, S. Sharma, S. Mani, and S. Minko. 2017. Environmentally sound textile dyeing technology with nanofibrillated cellulose. Green Chemistry 19 (17):4031–35. doi:10.1039/C7GC01662J.
- Kramar, A., A. Ivanovska, and M. Kostić. 2021. Regenerated cellulose fiber functionalization by two-step oxidation using sodium periodate and sodium chlorite-impact on the structure and sorption properties. Fibers and Polymers 22 (8):2177–86. doi:10.1007/s12221-021-0996-8.
- Liu, X., H. Liu, and Y. Fang. 2022. High-efficiency and durable flame retardant cotton fabric with good physical and mechanical properties by layer-by-layer assembly polyethylenimine/phytic acid coating. Journal of Natural Fibers 1–12. doi:10.1080/15440478.2022.2044428.
- Liu, K., X. Zhang, and K. Yan. 2018. Development of o-phthalic anhydride as a low-temperature activator in H2O2 bleaching system for cotton fabric. Cellulose 25 (1):859–67. doi:10.1007/s10570-017-1578-1.
- Mojsov, K. 2018. Enzymatic scouring and bleaching of cotton terry fabrics–opportunity of the improvement on some physicochemical and mechanical properties of the fabrics. Journal of Natural Fibers 15 (5):740–51. doi:10.1080/15440478.2017.1361884.
- Peng, M., S. Wu, J. Du, C. Sun, C. Zhou, C. Xu, and X. Hu. 2018. Establishing a rapid pad-steam process for bleaching of cotton fabric with an activated peroxide system. ACS Sustainable Chemistry & Engineering 6 (7):8599–603. doi:10.1021/acssuschemeng.8b00912.
- Sangeetha, V., T. Varghese, and S. K. Nayak. 2019. Isolation and characterisation of nanofibrillated cellulose from waste cotton: Effects on thermo-mechanical properties of polylactic acid/ma-g-SEBS blends. Iranian Polymer Journal 28 (8):673–83. doi:10.1007/s13726-019-00733-3.
- Toshikj, E., A. Tarbuk, K. Grgić, B. Mangovska, and I. Jordanov. 2019. Influence of different oxidizing systems on cellulose oxidation level: Introduced groups versus degradation model. Cellulose 26 (2):777–94. doi:10.1007/s10570-018-2133-4.
- Tülek, A., D. Yıldırım, D. Aydın, and B. Binay. 2021. Highly-stable Madurella mycetomatis laccase immobilized in silica-coated ZIF-8 nanocomposites for environmentally friendly cotton bleaching process. Colloids and Surfaces B: Biointerfaces 202:111672. doi:10.1016/j.colsurfb.2021.111672.
- Vörösmarty, C. J., P. B. McIntyre, M. O. Gessner, D. Dudgeon, A. Prusevich, P. Green, S. Glidden, S. E. Bunn, C. A. Sullivan, and C. R. Liermann. 2010. Global threats to human water security and river biodiversity. Nature 467 (7315):555–61. doi:10.1038/nature09440.
- Wang, N., P. Tang, C. Zhao, Z. Zhang, and G. Sun. 2020. An environmentally friendly bleaching process for cotton fabrics: Mechanism and application of UV/H2O2 system. Cellulose 27 (2):1071–83. doi:10.1007/s10570-019-02812-3.
- Xia, L., A. Wang, Y. Wang, C. Zhang, Y. Wang, S. Zhou, Z. Fu, H. Zhao, C. Ding, and W. Xu. 2021. Eco-friendly dyeing of raw cotton fibres in an ethanol–water mixture without scouring and bleaching pretreatments. Green Chemistry 23 (2):796–807. doi:10.1039/D0GC02839H.
- Yang, S., X. Pan, Z. Han, D. Zheng, J. Yu, P. Xia, B. Liu, and Z. Yan. 2017. Nitrogen oxide removal from simulated flue gas by UV-irradiated sodium chlorite solution in a bench-scale scrubbing reactor. Industrial & Engineering Chemistry Research 56 (13):3671–78. doi:10.1021/acs.iecr.6b04463.
- Zhao, Q., J. Pu, S. Mao, and G. Qi. 2010. Process optimization of tetra acetyl ethylene diamine activated hydrogen peroxide bleaching of Populus nigra CTMP. BioResources 5 (1):276–90. doi:10.15376/BIORES.5.1.276-290.