ABSTRACT
In this study, the Enset fibers were equally divided lengthwise from bottom to top into four portions explicitly 0–300 mm (EV-I),300–600 mm (EV-II), 600–900 mm (EV-III), and 900–1200 mm (EV-IV), and the physio-mechanical properties were investigated at three different GL (Gauge length). The distribution of the physical properties of the mass, cross-sectional area, linear density, and diameter was decreased along the fibers portion from bottom to top at three different tests GL. In terms of mechanical property distributions, the Enset fiber’s tensile strength grew considerably at around the height of over 25% of the portion along the length (EV-300) from the bottom and reduced marginally at its top places in each test GL. Regarding the significance of tensile properties, the overall ANOVA has no significant difference while the interaction between the locations along the length of enset fiber has a significant difference, and this variation is also confirmed by the Weibull distribution. The current study looks into the physical and mechanical properties of Enset fibers, which are controlled by the position of the leave sheath, which is used as an input to optimize its variation for unidirectional composite applications.
摘要
在这项研究中,Enset纤维从下到上沿纵向均匀地分为四部分,分别为0-300 mm (EV-I)、300-600 mm (EV-II)、600-900 mm (EV-III) 和900-1200 mm (EV-IV),并在三种不同的GL (标距长度) 下研究了物理力学性能. 在三种不同的试验GL中,质量、横截面积、线密度和直径的物理性质分布沿着纤维部分从下到上减小. 就机械性能分布而言,Enset纤维的抗拉强度在从底部开始沿长度方向 (EV-300) 超过25%的高度处显著增加,在每个GL试验中,其顶部略有降低. 关于拉伸性能的显著性,总体ANOVA没有显著差异,而沿enset纤维长度的位置之间的相互作用有显著差异,这种差异也通过威布尔分布得到证实. 目前的研究着眼于恩塞特纤维的物理和机械性能,这些性能受叶鞘位置的控制,叶鞘位置被用作优化单向复合材料应用中其变化的输入.
Introduction
Human-made fibers pollute the environment when they are dumped in landfills, causing air and water pollution. To overcome this tough situation, environmental concerns have rekindled interest in natural materials, and issues like recyclability and environmental safety have become increasingly relevant in the development of novel materials and products. Natural fiber-reinforced composite engineering has got a remarkable and outstanding interest in the automobile industries and buildings owing to its eco-friendly nature, low-cost availability, high flexural and tensile strength, good abrasion, and acoustic resistance (Bledzki, Mamun, and Faruk Citation2007). The major advantages of natural fibers composite are the vast number of natural fibers available around the world, reduced environmental impact and it is generated from renewable resources (Baley et al. Citation2020). Natural fibers, on the other hand, have a greater range of mechanical properties and are associated with worries regarding their long-term durability in moist environments due to their capacity to absorb moisture (Virk Citation2010).
The good opportunity of natural fibers, their surface is often easily modified to induce the will properties. Some of the primally natural fibers category are, namely, bast fibers, leaf fibers, and seed-hair fibers, which are grouped consistent with their morphological structure (Godara Citation2019). Remarkably, the suitable plant with a great potential for the production of natural fibers under the category of leaf fibers, enset plant, is a vital multipurpose indigenous crop for Ethiopia (Ayele and Sahu Citation2014; Yemataw et al. Citation2018; Zafeiropoulos and Baillie Citation2007; Zewdie Citation2012). Enset plant called Enset (Enset Ventricosum) may be a large banana-like perennial herbaceous monocarpous plant) could be a perennial plant that has leaves, an outsized underground corm, pseudostem, and one among the most food plants grown for traditional diet in Ethiopia. As a by-product enset fiber could be a natural fiber obtained from the pseudostem (leaf) a part of enset plant, andthe strong enset bundle fibers are currently solely employed for the local rope industry (Blomme et al. Citation2018). As a result, processing this agricultural product and using it as an input for businesses would be a significant contribution to the economic sector’s success. Therefore, this green fabricating motto is well met by the enset fiber-based products. However, the wide variation of their physical and mechanical properties makes the enset fibers difficult to use for structural parts when high reliability is required. This argument has been supported by (Amroune et al. Citation2021).The fiber qualities are determined by the growing conditions, harvest maturity, fiber processing technique, and the moisture content of the fiber. Sequentially, these aspects may influence the microstructure and therefore the result influences its mechanical properties (Virk Citation2010). Furthermore, because of the variability, estimating the composite qualities accurately is challenging. For natural fibers to emerge as a viable alternative to synthetic fiber reinforcements for structural composites, a thorough study of the natural variation of their physical and mechanical properties is required. Selecting fibers with deserved properties is crucial for the appliance of the natural fibers, especially the strongest bundle fibers like sisal, banana, and abaca (Liu, Takagi, and Yang Citation2013). Researchers often improve the mechanical properties of natural fibers by changing the microstructure by treatments. In this context, to boost the performance of unidirectional composites, technical knowledge of the physical, thermal, chemical, and mechanical properties of natural fibers is important (Binoj et al. Citation2016).
Remarkably, the author reported that the best mechanical performance of reinforcement may be achieved by continuous fibers aligned within the direction of the applied load (Vasquez and Joy L Diaz Citation2017, Citation2010). Dessie et al. discovered that by arranging unidirectionally reinforced PP with enset bundle fiber treated with NaOH and Silane, the mechanical performance was improved (Dessie et al. Citation2021, Citation2021).
The present study aims to characterize these long enset bundle fibers and to utilize them economically by sustainable and cost-effective methods, and then the statistical analysis of the enset bundle fiber has an important economical role to propose the application. Furthermore, most of the previous studies of advanced statistical analysis were concentrated on the effect of fiber treatments on tensile strength(Amroune et al. Citation2015, Citation2021; Maache et al. Citation2017). However, there is a limited report related to the effect of fiber localization in the leaf sheath of the Enset fiber plant and gauge length on the original structure of long Enset bundle fibers and turn on their mechanical and physical properties. So, the tests were performed in different gage lengths in order to assess the effect of the length on the mechanical properties (Fidelis et al. Citation2013). The goal of the study is to improve the prediction of physical and mechanical characteristics by better understanding the stochastic nature of Enset fibers, with an emphasis on the effect of fiber portion along the leaf sheath on mechanical properties(Virk Citation2010).
For this purpose, the physical and the mechanical properties of enset fiber will be evaluated piecewise to obtain the desired properties by selecting fibers from different positions of the plant. To achieve this objective, the enset fibers were manually separated and arranged along the length from 4 different locations, and the physical and mechanical properties were measured (bottom, middle, top) with a selected three-gauge length (GL). Finally, the distribution of variation is investigated by one-way ANOVA and Weibull statistical analysis(Amroune et al. Citation2015; Citation2021; Dessie et al. Citation2022; Maache et al. Citation2017) was also used to quantify the variability of fiber strength.
Material and methods
Materials
The Enset fiber came from the Oromia Region, Wolkitie at about 1200 mm in length, and also the fibers were extracted from the leaf sheath of enset fiber plant by a mechanical process called decortication. Decorticated fibers were dried under the sunlight. An acceptable drying is important since the standard of the fiber depends on the reduced moisture content (Sinha, Bhattacharya, and Kumar Narang Citation2020). Figure Extraction and sample preparation of Enset bundle fibers from enset plant Pseudostem.
Sample preparation
Enset fiber extraction process has been done by manually/Indian model extraction machines After the fiber was dried and cut into four positions with 300 mm length in sequence from the bottom (fragment position 300 mm) to the top fragment position in the leaf sheath of enset fiber plant 1200 mm as presented in . During the whole sample preparation process, enset fiber was maintained and kept straight. Enset fibers were manually separated and aligned the bundle fiber, and leveling at four sections along the length from bottom to top namely :0–300 mm (EV-I),300–600 mm (EV-II),600–900 mm (EV-III), and 900–1200 mm (EV-IV). The characterization and evaluation of enset bundle fiber has been made by various gauge length at each position(section) along the length of leaf sheath (Pseudostem). Subsequently, the bundle fiber characterization and evaluation of physical and mechanical properties has been made.
Characterization of Physical and mechanical properties
Enset bundle fiber was characterized by gauges lengths (GL) namely GL 50 mm, GL100 mm- and GL 250 mm for enset fiber portions along the length of 1200 mm at four positions EV-I, EV-II, EV-III, and EV-IV. The length of bundle fiber prepared was the GL plus 15% of the GL for extra length for the jaw fixation. 20 samples were prepared for each GL and each sample was sectioned into equal portions as per the standard. The mass distribution of each portion 4 × 20×3 = 240 samples were measured on an electronic balance with an accuracy of 0.0001 g. The linear mass density of fiber, which is the measure of the fineness of the fibers, is estimated as per the ASTM D 1577–07 standard. The linear density was calculated considering the length of each enset fiber portion 300 mm and the mass. The diameter was measured with a micrometer with an accuracy of 0.001 mm (Dessie et al. Citation2022). For each fiber portion, 20 fibers were measured. Fibers were carefully manually separated from the bundles. Fiber ends were glued onto a paper frame according to the preparation procedure described in ASTM D 3822–07 standard. The fiber cross-sectional area has been calculated by considering the parameter of fiber mass, fiber length, and fiber density (Brahim and Ben Cheikh Citation2007). The tensile strength is determined by universal Tensolab type tensile testing machines (Mesdan Lab) equipped with 20N load cell and its test speed was 100 mm/min for GL 50 and 100, for Gl 250 the test speed was 250 mm/min with 20 N load cel. Each sample of fiber has been loaded with each test length namely GL 50,100, and 250 mm, and the force to break, tenacity, and strain rate have been accessed from the machines. 20 samples were tested for each portion of fibers as per the selected GL and the average value was reported along with the variability of the data. When fixing the specimens onto the tester, special care was taken to prevent fibers misalignment. The distance between the grips was fixed according to requirement, and the upper end of the fiber was clamped first and the lower end of the fiber.
Statistical analysis
The effect of GL and fibers portion on physical and mechanical properties of enset bundle fibers component over the length of the enset fibers were examined using an ANOVA F-test analysis of variance (Valášek et al. Citation2017). Initially, the null hypothesis was assumed to be H0, which states that there is no statistically significant difference between the measured data (p > .05), and hypothesis H1 states that the hypothesis H0 is rejected, i.e., that there is a statistically significant difference between the measured data (p < .05). A one-way ANOVA was used to determine the relevance of fibers portions along the length on physical qualities, as well as the effect of GL and fibers portion on mechanical properties. For evaluation of mechanical properties of enset bundle fiber, two- parameters Weibull method has been applied(Amroune et al. Citation2021; Maache et al. Citation2017; TRUJILLO DE LOS RIOS et al. Citation2012 Zafeiropoulos and Baillie Citation2007; Zewdie Citation2012).
Result and discussion
Effect of fiber portions on physical properties
The distribution of physical parameters of the mass, diameter, cross-sectional area, and linear density along the segment of the fiber has been studied with GL 50,100 and 250 mm. In this regard, the fiber portions along the length of enset fiber concerning selected GL vs fibers portion of enset bundles fiber distribution are provided in . The investigation indicated that the mass, diameter, linear density, and the cross-sectional area distribution at EV-I, EV-II, EV-III, and EV-IV portions were decreased from the bottom to top for all three different GL namely GL50 mm, GL100 mm, and GL250 mm. Despite the value disparity, the inquiry of each gauge length discloses that the minimum value was documented from the top, and the maximum from the bottom, and a comparable examination was revealed for each three different GL. The difference in distribution might be due to the variation of chemical composition existing between the bundle fibers, growth conditions, and decoration process (Lefeuvre, Bourmaud, and Baley Citation2015). Largely, this suggests the dependence of the cross-section and the diameter of the location of the enset fiber placed within the plant sheath. Enset fiber, the cellulosic fibers obtained from the pseudostem with relatively good mechanical properties. The composition of enset pseudostem was obtained by elemental analysis, as determined by (Teli and Terega Citation2017). They are generally lignocellulosic, consisting of helically-wound cellulose microfibrils in an amorphous matrix of lignin and hemicellulose. The cellulose content serves as a deciding factor for mechanical properties along with microfibril angle. High cellulose content and low microfibril angle impart desirable mechanical properties for leaf fibers. The tenacity of the fibers significantly improved for the fibers with lower linear density (Mukhopadhyay, Fangueiro, and Shivankar Citation2009).
Table 1. The effect of fiber portions on physical property.
Effect of Enset Fibers portion and gauge length on tensile strength
The tensile strength of Enset bundle fiber is the ability of the fiber of the material to withstand the tensile force applied to its longitudinal axis. This is one of the most important properties in the scheme of fibers and reinforced composites. In unidirectional specimens, the influence of gauge length and fiber sections along the bundle fiber section on the tensile mechanical properties is important. For this purpose, the distribution of mechanical properties of force to break, tenacity, elongation at break, and tensile modulus at every four portions along the length has been evaluated at GL50 mm, GL100 mm, and GL250 mm as presented in . The braking force (cN) distribution along the length of the enset fiber portions has been investigated that the breaking force increased by 23.61%,28.86%, and 21.21% at around EV-II locations and slightly decreased around EV-III portions by 14.47%, 13.52%, and 12.83% at the test GL 50 mm,100 mm and 250 mm respectively as shown in . Moreover, the decrease continues a sharp decrease at GL 50 mm and Gl 250 mm, and a slight decrease at GL100 mm by 31.78%,21.11% and 16.37%, at around EV-IV locations respectively. In all cases the maximum value has been found at Fiber bundle portion EV-II.
Concerning the tenacity/tensile strength of the bundle, fiber is determined in similar manner at each GL along with in each fiber portions of 1200 mm, and the result indicated that EV-I, EV-II, EV-III, and EV-IV as shown in . The investigation indicates that the tenacity of the Enset bundle fiber was notified at around above the height of 25% (300-600 mm) a sharp increase in tensile strength to a maximum by 23.59%,28,9%, and 21.09% at around EV-II locations and slightly decreased around EV-III portions by 14.42%,13.53% and 12.63% for GL50, GL100 and GL250 mm respectively as demonstrated in . Furthermore, at around EV-IV sites, the dropping continues with a strong fall at GL50 mm, slight decrease at GL50 mm and a strong decline at GL 250 mm by 31.84, 15.19, and 24.28%, respectively. Similar investigation has been found by (Charlet et al. Citation2007).
Table 2. Mechanical properties along fiber portion at each GL.
Two important facts can be generated from these investigations. First, the comparison of the four portions along the length has been evaluated at three different GL, and the investigations showed that the highest tenacity was achieved between EV-II and EV-III, and the minimum strength was recorded at the top portions of EV-IV. Second, the tenacity diminishes as the test GL increases, i.e., 50, 100, and 250 mm, therefore the longer the GL, the more likely the weak point will be involved. The longer the stressed distance of the Enset bundle fiber, the more inhomogeneities will be in the stressed fiber segment, weakening the structure (Bledzki and Gassan Citation1999). Similar behavior was observed by (Sia et al. Citation2014). Despite the differences, all GL at about the medium height shows an increase in tenacity; nevertheless, the cause of variation may be explained by three primary factors: test parameters/conditions, growing condition plant fiber characteristics, and area measurements. This argument was supported (Amroune et al. Citation2021; de Andrade Silva, Chawla, and de Toled Filho Citation2008). The number of defects in the cross-sectional area of the fiber increases with an increasing diameter which results in a major decrease in tensile strength. In the case of plant fibers, the strength of depends more on the defects rather than on the structural arrangements such as cellulose content and micro-fibril angle. Another fascinating comparison was made by calculating stress in MPa by considering the force and the cross-sectional area. As seen in , the bundle fiber strength tensile stress force (MPa) distribution along the length of the enset fiber portions has been investigated that the tensile force increased by 23.61%,28.86%, and 17.5% at around EV-II locations and slightly decreased around EV-III portions by 14.47%, 13.52%, and 12.83% at the test GL 50 mm,100 mm and 250 mm respectively. Moreover, the decrease continues a sharp decrease at GL 50 mm and Gl 250 mm, and a slight decrease at GL100 mm by 31.78%, 24.88% and 16.3% at around EV-IV locations respectively. In all cases, similar to the tenacity analysis, the maximum value has been found at Fiber bundle portion EV-II. Charlet et al observed similar behavior (Charlet et al. Citation2007).
A decrease in tenacity at the top is probably showing into account the defects caused during the decortication and subsequent process (Liu, Takagi, and Yang Citation2013). The case may be as the fiber diameter decrease the microfiber angle and the cellulose content increase (Liu, Takagi, and Yang Citation2013). Regarding the elongation at breakage, it reduced from bottom portions to top portion of the fiber portion respect to each GL50, GL100, and GL250 mm as presented in and . De Andrade Silva et al investigated the same idea (de Andrade Silva, Chawla, and de Toled Filho Citation2008; Fidelis et al. Citation2013). This tendency is explained and connected to the average size and distribution of faults in the fiber volume. It can be observed that the elongation percentage at breakage difference decreased with the increasing fiber location along the fiber proposing the much larger elongation at a percentage of the fiber at a lower location compared to the top location of the fiber, which is resulting from the wider variability of fiber in tensile strength. This fascinating research will need to be performed with a greater number of samples to get reliable average values of fiber mechanical properties.
As a remark, this investigation was observed a decrease in the breaking strain with an increase in the test length as shown in . This behavior of Enset fiber is similar to what is observed in the case of coir (Kulkarni et al. Citation1983).
Specifically, when the average tensile strength of the fiber is constant, if the distribution in fiber tensile strengths is larger, the remaining fibers have a higher ability to withstand the additional stress caused by the fracture of the previous fibers generating a larger elongation percentage at the break. On the other hand, for higher locations, the whole fiber is quickly ruptured when some weaker fibers start to rupture. Therefore, the decreasing tensile strength of enset fiber with increasing locations is mainly caused by the different stochastic nature of fiber fracture varied with the fiber location along with the fiber. This will have a direct relation to the decrease in elongation at breakage along the length. The ultimate strain of enset bundle fiber for different GL is plotted in . The reduction of elongation percentage at breakage of the enset bundles fiber can be explicated by the circumstance that the catastrophe of fiber may transfer the load to adjacent fibers, which avoids a catastrophic failure of the entire bundle.
The bundle fibers, with more steady cross-sections, are less sensitive to stress concentration and thus can reach higher catastrophe stresses for longer GL(Baley et al. Citation2020). (Fidelis et al. Citation2013), it can be seen that as the internal area of the lumens decrease and the secondary cell-wall thickness increases the fiber strength and young’s modulus increase. The fiber breaking force, toughness, and fracture strain all decreased as the gauge length increased (Virk Citation2010)., This behavior has been reported by (Charlet et al. Citation2007). A significant correlation between the fiber strength/fracture strain and the fiber gauge length was found. It was discovered that when gauge length rises, fiber strength/fracture strain declines. Regarding the tensile modulus, as the gauge length increases, the tensile modulus increase (Virk Citation2010). However, there is debate about the fact that recorded values of the modulus of elasticity increased with gauge length (Mizera et al. Citation2016).
Generally, the failure strain is only slightly influenced by the fiber cross-section, according to data from tensile tests, hence the coefficient of variation (CoV) for failure strain is consistently lower than the CoV for fracture stress (strength). In order to increase reliability in the design of these materials, it is advised to employ failure strain as the primary design criterion for NFCs because it is the most reliable failure criteria. (Virk Citation2010) offered an argument in favor of it.
The significant difference in enset bundle fiber strength along the length
The mechanical properties of Enset fiber portion along the length have been characterized at four locations: EV-I, EV-II, EV-III, and EV-IV with GL50 mm, 100, and 250 mm, and the interaction of their variance was evaluated with one-way ANOVA as shown in .
Table 3. Overall Anova analysis.
In this relation, the assumption was the Null Hypothesis: means of all levels (fiber portions) are equal and an alternative hypothesis; the mean of one or portion means is a significant difference. The comparison of tensile strength of enset bundle fiber with overall ANOVA at the 0.05 level is not significantly different at the given of the three GL. However, the interactions between the portions EV-III with EV-I, and EV-III with EV-II are not a significant difference, and the other interactions are significant differences, and the details are presented in . From the statistical analysis in specific the fiber portion EV-IV (above the length of 900 mm) is significantly different compared to the other section. Concerning Weibull distribution of tensile strength of enset fiber was investigated as presented in for GL 50,100, and 250 mm respectively. The maximum value of Weibull modulus and tensile strength was found for all three GL around location EV-II, whereas low Weibull modulus and tensile strength were investigated in fiber portion EV-IV and sequentially EV-I as presented in . The higher the Weibull modulus value, the more homogeneous the material, and it indicates that the stress to cause failure at a given probability value will be higher. In terms of the effect of fiber location along the fiber length here performed, the Weibull modulus can thus be used indirect parameter to verify if the locations of the fiber influence the physical and mechanical properties. This maximum value indicated that the homogeneous distribution of the tensile strength along the length indicates that the minimum coefficient variation was found at location EV-II as well as maximum strength. Regarding the prediction of the strength characteristics, the maximum value was recorded also in location EV-II. Similarly, the analysis of Weibull modulus distribution at GL 100 mm indicates a similar analysis. In general, the Weibull modulus variation indicates that the localization of the fiber affects the tensile strength along the length of enset fiber. The analysis of the tensile strength along the portion of the length is not able to distinguish the effects caused by fragments/piecewise/while the Weibull modulus can be used to advantage as an indicative parameter of the effects caused by fiber portion along the length on the tensile strength of enset fibers, more accurately describing the effects of the fiber locations upon the fiber integrity.
Table 4. Interaction of fiber portions of means Comparisons by Tukey test.
Figure 7. Weibull distribution of tensile strength along with the location of the fiber at GL 50 mm.
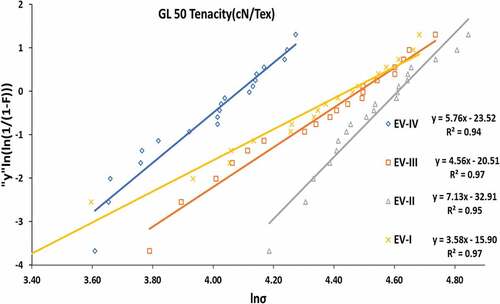
Figure 8. Weibull distribution of tensile strength along with the location of the fiber at GL 100 mm.
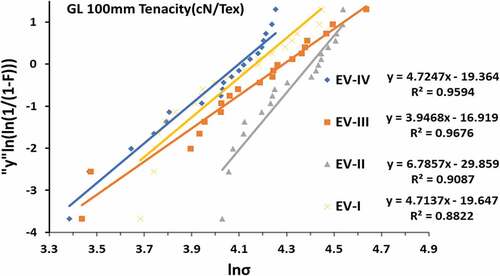
Figure 9. Weibull distribution of tensile strength along with the location of the fiber at GL 250 mm.
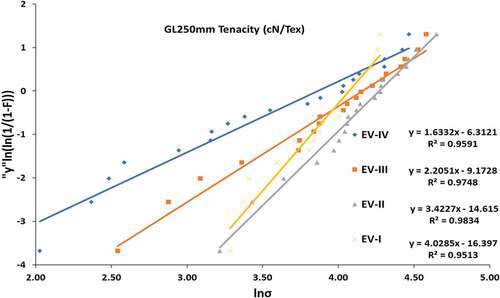
Table 5. Weibull distribution analyzing localizing at three different GL.
As a result, The Weibull modulus, m, is used to calculate the scatter in fiber strengths. For example, two fibers may have the same average tenacity, but they may have quite different scatter in their strengths, which could be crucial in some situations determining the likelihood of a structure failing. Increasing gage length resulted in a decrease in the Weibull modulus (de Andrade Silva, Chawla, and de Toled Filho Citation2008). Enset fibers exhibited variability in tensile strength which is quite characteristic of plant fibers. This variability can be explained by the distribution of defects within the fiber or on the fiber surface. The mean defect size for all materials controls the mean strength, but the number of defects controls the Weibull modulus. Thus, while the fibers with a lower gage length have a smaller number of defects, the average defect size is the same as that of the other gage lengths.
The graph inclination shows the Weibull modulus. Generally, when the Weibull modulus is large, the variation in strength is small, and the reliability of the material is excellent (Nakamura, Hojo, and Sato Citation2010). Overall, the two-parameter Weibull distribution parameters for tensile strength revealed that as the test GL rose, the Weibull modulus grew, however, the characteristics of strength and experimental average tenacity declined. On lnln(1/1-F) vs lnσ, the linear relationship between the Weibull modulus, average tensile strength, and gauge length was established (). The Weibull modulus distribution result, on the other hand, was contradicted by (Virk Citation2010).
Conclusion
In this paper, Enset fibers were piecewise into sequence 4 locations. The physical parameters such as diameter, linear density, mass, and cross-sectional area were characterized. The tensile properties of fiber were tested. With increasing fiber localization from bottom to top, the average mass, cross-sectional area, diameter, and linear density of enset fiber decreased 20–30% for GL 50,100, and 250 mm. Due to the different physical properties among locations, the tensile strength of Enset fiber increased greatly to a maximum at EV-II locations, and then slightly decreased with the fiber portion toward the top. The decrease in breaking load and tenacity with increasing test length may be due to an increase in the probability of faults and weak links as the length of fibers rises. Overall, the one-way ANOVA variance suggests that fiber placement throughout the fiber part from the bottom to the top has a substantial effect on physical and mechanical qualities, which has been supported by Weibull distribution. The fiber portions and the effect of gauge length have a significant effect on mechanical and physical properties. The statistical data showed that there is a large standard deviation that the physical and mechanical properties have been observed, at the fiber portions around 300–600 mm(EV-II) and 600–900 mm (EV-III) have the best mechanical properties, maybe the biochemistry of cell wall may play a primary role. Overall, the investigation indicates that the geometrical dimensions of enset fibers, especially the fiber length depend mainly on fiber location within the plant sheath.
Highlights
Enset bundle fiber’s mechanical and physical characteristics are influenced by the gauge length and the placement of the enset leaf sheath.
The distribution of physical properties decreased along the fiber length, from bottom to top.
The distribution of tensile strength increased at a height of around at middle and dropped at top portions.
Variation of distribution has been confirmed by the Weibull distribution.
Acknowledgments
I would like to acknowledge the financial support of the German Development Bank, KfW for the collaborative research between the Donghua University, China, and the Ethiopian Institute of Textile and Fashion Technology, Bahir Dar University, Bahir Dar, Ethiopia.
Disclosure statement
No potential conflict of interest was reported by the author(s).
Additional information
Funding
References
- Amroune, S., A. Bezazi, A. Belaadi, C. Zhu, F. Scarpa, S. Rahatekar, and A. Imad. 2015. Tensile mechanical properties and surface chemical sensitivity of technical fibres from date palm fruit branches (Phoenix dactylifera L.). Composites Part A, Applied Science and Manufacturing 71:95–15. doi:10.1016/j.compositesa.2014.12.011.
- Amroune, S., A. Bezazi, A. Dufresne, F. Scarpa, and A. Imad. 2021. Investigation of the date palm fiber for green composites reinforcement: Thermo-physical and mechanical properties of the fiber. Journal of Natural Fibers 18 (5):717–34. doi:10.1080/15440478.2019.1645791.
- Ayele, A., and O. Sahu. 2014. Extension of Enset plant product for rural development in Ethiopia. Journal of Agricultural Economics, Extension and Rural Development 2 (3):031–040.
- Baley, C., M. Gomina, J. Breard, A. Bourmaud, and P. Davies. 2020. Variability of mechanical properties of flax fibres for composite reinforcement. A review. Industrial Crops and Products 145:111984. doi:10.1016/j.indcrop.2019.111984.
- Binoj, J. S., R. Edwin Raj, V. S. Sreenivasan, and G. Rexin Thusnavis. 2016. Morphological, physical, mechanical, chemical and thermal characterization of sustainable Indian Areca fruit husk fibers (Areca Catechu L.) as potential alternate for hazardous synthetic fibers. Journal of Bionic Engineering 13 (1):156–65. doi:10.1016/s1672-6529(14)60170-0.
- Bledzki, A.K., and J. Gassan. 1999. Composites reinforced with cellulose based fibres. Progress in Polymer Science 24 (2):221–74. doi:10.1016/S0079-6700(98)00018-5.
- Bledzki, A.K., A.A. Mamun, and O. Faruk. 2007. Abaca fibre reinforced PP composites and comparison with jute and flax fibre PP composites. Express Polymer Letters 1 (11):755–62. doi:10.3144/expresspolymlett.2007.104.
- Blomme, G., Z. Yemataw, K. Tawle, V. Sinohin, L. Gueco, R. Kebede, and A. Lalusin. 2018. Assessing enset fibre yield and quality for a wide range of enset [Ensete ventricosum (Welw.) Cheesman] landraces in Ethiopia. Fruits 73 (6):328–41.
- Brahim, S. B., and R. Ben Cheikh. 2007. Influence of fibre orientation and volume fraction on the tensile properties of unidirectional Alfa-polyester composite. Composites Science and Technology 67 (1):140–47. doi:10.1016/j.compscitech.2005.10.006.
- Charlet, K., C. Baley, C. Morvan, J. P. Jernot, M. Gomina, and J. Bréard. 2007. Characteristics of Hermès flax fibres as a function of their location in the stem and properties of the derived unidirectional composites. Composites Part A, Applied Science and Manufacturing 38 (8):1912–21. doi:10.1016/j.compositesa.2007.03.006.
- de Andrade Silva, F., N. Chawla, R. de Toled Filho, and Technology. 2008. Tensile behavior of high performance natural (sisal) fibers. Composites Science and Technology 68 (15–16):3438–43. doi:10.1016/j.compscitech.2008.10.001.
- Dessie, E., L. Fanxizi, T. Tesfaye, R. Kipchirchir Gideon, A. Dagnaw Gudayua, and Y. Qiu. 2021. Effect of silane treatment on tensile strength, moisture absorption and thermal property of unidirectional woven mat enset fibers reinforced polypropylene composite. Composite Interfaces 1–21. doi:10.1080/09276440.2021.2015151.
- Dessie, E., T. Tesfaye, R. Kipchirchir Gideon, M. Getnet Tadesse, and Y. Qiu. 2022. The Influence of Location along the Pseudostem on Enset Fiber Physio-Mechanical Properties: Application of Weibull Distribution Statistics. Applied Sciences 12 (14):7323. doi:10.3390/app12147323.
- Dessie, E., Q. Yiping, T. Tesfaye, R. Kipchirchir Gideon, and L. Fanxizi. 2021. Effect of Surface Treatment on the Mechanical Performance of Unidirectional Enset Fiber Reinforced Polypropylene Composite. Journal of Natural Fibers 1–14. doi:10.1080/15440478.2021.1982441.
- Fidelis, M. E. A., T. Vitorino Castro Pereira, O. da Fonseca Martins Gomes, F. de Andrade Silva, and R. Dias Toledo Filho. 2013. The effect of fiber morphology on the tensile strength of natural fibers. Journal of Materials Research and Technology 2 (2):149–57. doi:10.1016/j.jmrt.2013.02.003.
- Godara, S.S. 2019. “Effect of chemical modification of fiber surface on natural fiber composites: A review.” Materials Today: Proceedings. Department of Mechanical Engineering, Rajasthan Technical University Kota, India. 18:3428–34. doi:10.1016/j.matpr.2019.07.270.
- Kulkarni, A.G., K.G. Satyanarayana, P.K. Rohatgi, and K. Vijayan. 1983. Mechanical properties of banana fibres (Musa sepientum). Journal of Materials Science 18 (8):2290–96. doi:10.1007/BF00541832.
- Lefeuvre, A., A. Bourmaud, and C. Baley. 2015. Optimization of the mechanical performance of UD flax/epoxy composites by selection of fibres along the stem. Composites Part A, Applied Science and Manufacturing 77:204–08. doi:10.1016/j.compositesa.2015.07.009.
- Liu, K., H. Takagi, and Z. Yang. 2013. Dependence of tensile properties of abaca fiber fragments and its unidirectional composites on the fragment height in the fiber stem. Composites Part A, Applied Science and Manufacturing 45:14–22. doi:10.1016/j.compositesa.2012.09.006.
- Maache, M., A. Bezazi, S. Amroune, F. Scarpa, and A. Dufresne. 2017. Characterization of a novel natural cellulosic fiber from Juncus effusus L. Carbohydrate Polymers 171:163–72. doi:10.1016/j.carbpol.2017.04.096.
- Mizera, Č., D. Herák, P. Hrabě, M. Müller, and A. Kabutey. 2016. Effect of length of false banana fibre (Ensete ventricosum) on mechanical behaviour under tensile loading. Scientia agriculturae bohemica 47 (2):90–96. doi:10.1515/sab-2016-0013.
- Mukhopadhyay, S., R. Fangueiro, and V. Shivankar. 2009. Variability of tensile properties of fibers from pseudostem of banana plant. Textile Research Journal 79 (5):387–93. doi:10.1177/0040517508090479.
- Nakamura, Y., S. Hojo, and H. Sato. 2010. The effect of surface roughness on the Weibull distribution of porcelain strength. Dental Materials Journal 29 (1):30–34. doi:10.4012/dmj.2009-059.
- Sia, C. V., Y. Nakai, D. Shiozawa, and H. Ohtani. 2014. Statistical analysis of the tensile strength of treated oil palm fiber by utilisation of Weibull distribution model. Open Journal of Composite Materials 2014:72–77. doi:10.4236/ojcm.2014.41008.
- Sinha, A. K., S. Bhattacharya, and H. Kumar Narang. 2020. Abaca fibre reinforced polymer composites: A review. Journal of Materials Science 56 (7):4569–87. doi:10.1007/s10853-020-05572-9.
- Teli, M. D., and J. M. Terega. 2017. Study on the chemical composition, physical properties and structural analysis of raw and alkali treated Sansevieria roxburghiana fibre. International Research Journal of Engineering and Technology 4 (12): 67–75.
- TRUJILLO DE LOS RIOS, E. E., M. Moesen, L. R. Osorio Serna, A. W. Van Vuure, J. Ivens, and I. Verpoest. 2012. “Weibull statistics of bamboo fibre bundles: Methodology for tensile testing of natural fibres.” 15th European Conference on Composite Materials, Padova: University of Padova.
- Valášek, P., M. Müller, V. Šleger, V. Kolář, M. Hromasová, R. D’Amato, and A. Ruggiero. 2021. Influence of Alkali Treatment on the Microstructure and Mechanical Properties of Coir and Abaca Fibers. Materials 14 (10):2636. doi:10.3390/ma14102636.
- Vasquez, J. Z., and L. Joy L Diaz. 2017. Unidirectional abaca fiber reinforced thermoplastic starch composite. Materials Science Forum 894:56–61. https://doi.org/10.4028/www.scientific.net/MSF.894.56.
- Virk, A. S. 2010. Numerical models for natural fibre composites with stochastic properties. A thesis submitted to the University of Plymouth in partial fulfilment for the degree of Doctor of Philosophy, University of Plymouth PEARL. http://hdl.handle.net/10026.1/517.
- Yemataw, Z., T. Kassahun, G. Murray, D. J. Studholme, A. Chala, and K. Tesfaye. 2018. Multivariate analysis of morphological variation in enset (Ensete ventricosum (Welw.) Cheesman) reveals regional and clinal variation in germplasm from south and south western Ethiopia. AJCS 6 (4):1135. doi:10.21475/ajcs.18.12.12.p1135.
- Zafeiropoulos, N. E., and C. A. Baillie. 2007. A study of the effect of surface treatments on the tensile strength of flax fibres: Part II. Application of Weibull statistics. Composites Part A, Applied Science and Manufacturing 38 (2):629–38. doi:10.1016/j.compositesa.2006.02.005
- Zewdie, A. 2012. Assessment of performance and adoption of improved enset processing technologiesDegree of master, A case study in Enemorena Ener district, Guragie zone, Southern Nations, Nationalities and Peoples Regional State, Ethiopia, University of Applied science Va Hal Larensten.