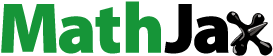
ABSTRACT
The brush-type adsorbent is an innovative material that provides significant advantages to adsorption processes and contains effective and diversifiable materials such as nanomaterials. The adsorbent can be separated from the environment by a single movement and it brings practicality, convenience, and low-cost to batch and column systems. The brush fibers were successfully coated with the adsorptive materials obtained from Fe(II)/Fe(III), Ag(I), Cu(II), Zn(II), and Ni(II) solutions. The particle size of magnetite nanoparticles formed on brush fibers is about 60 nm. SEM-EDX analysis showed that uncoated brush fibers mainly contained carbon, oxygen, and sulfur. Also, magnetite nanoparticles are spherical and contain iron and oxygen. The adsorbents have high removal efficiencies (80–94%) for arsenic, cadmium, lead, molybdenum, selenium, and thallium. The pseudo first-order, the pseudo second-order, Elovich, and the intraparticle diffusion kinetic models were fitted to the experimental data. The pseudo-second-order mechanism was predominant (R2 = 0.99–1.00) for all the elements, and the adsorptions are chemical in nature. The adsorbent can be produced for various purposes, in different contents and sizes. The use of this brush-type adsorbent for water and air pollutants will contribute to both the environment and the economy by facilitating and reducing the adsorption stages.
摘 要
刷式吸附剂是一种创新材料,为吸附过程提供了显著的优势,并包含有效和多样化的材料,如纳米材料. 吸附剂可以通过一次移动与环境分离,它为间歇式和柱式系统带来了实用性、方便性和低成本. 用从Fe(II)/Fe(III)、Ag(I)、Cu(II)、Zn(II)和Ni(II)溶液获得的吸附材料成功地涂覆刷状纤维. 在刷状纤维上形成的磁铁矿纳米颗粒的粒径约为60 nm。SEM-EDX分析表明,未涂覆的刷状纤维主要含有碳、氧和硫. 此外,磁铁矿纳米颗粒是球形的,含有铁和氧. 该吸附剂对砷、镉、铅、钼、硒和铊具有较高的去除效率(80–94%). 将伪一阶、伪二阶、Elovich和粒子内扩散动力学模型与实验数据拟合. 对于所有元素,伪二阶机制占主导地位(R2 = 0.99-1.00),并且吸附本质上是化学性质的. 吸附剂可以以不同的含量和尺寸生产用于各种目的. 对水和空气污染物使用这种刷式吸附剂将促进和减少吸附阶段,从而有助于环境和经济.
Introduction
The adsorption method is one step ahead of other treatment methods in terms of convenience, cost-effectiveness, and regeneration (Ali Citation2012; Dąbrowski Citation2001; Nitayaphat and Jintakosol Citation2020; Wang and Guo Citation2020). It is used for remediation and preconcentration, i.e., for analysis (Bryjak et al. Citation2016; Marczenko and Balcerzak Citation2000; Zolotov and Kuz’Min Citation1990). The adsorbent may be an adsorptive material that has been specifically prepared for adsorbing a substance, or it is prepared by attaching this adsorptive material to support. Many natural and chemically obtained materials are used as adsorbents. The use of natural fibers is increasing rapidly due to their abundance, low cost, and superiority to polymeric-based materials. Different fiber materials are used for the adsorption of heavy metals. Jute fibers were used for the adsorption of mercury and lead from aqueous solutions (Hassan and Zohdy Citation2018). Paramasivam et al., prepared banana pseudostem fiber and used for the removal of lead (Paramasivam et al. Citation2020). Human hair as a biogenic fiber was also used for multiple heavy metals from aqueous solutions (Zhang, Carrillo-Navarrete, and Palet-Ballús Citation2022). All three studies were fitted well to the pseudo-second kinetic model.
In this study, various metal solutions {such as Fe(II)/Fe(III), Ag(I), Cu(II), Zn(II), and Ni(II)} were used to successfully obtain brush-type adsorbents. It was observed that nanomaterials could be effectively formed on brushes made of animal (horse and pig) fibers. It has been determined that the materials obtained in the first experiments can remain stable for 5 years without deterioration. It is possible to form an adsorptive material on brush fibers, which will be selected based on the treatment process and species to be adsorbed. This study presents the preparation of a novel adsorbent, which provides a new perspective on adsorption and opens up new applications, surface imaging investigations, and results for the adsorption of several toxic elements. The experiments were conducted using a brush-type adsorbent obtained by forming magnetite (from Fe(II)/Fe(III) solutions), which is one of the most frequently used adsorptive materials in the literature, on the brush fibers.
Experimental
Materials
Three different brushes were used for the examination. The first () was a test-tube cleaning brush (1.5 cm diameter) with braided fibers (9.5 cm long, horsehair fiber), the second () was a cleaning brush (2.5 cm diameter) with braided fibers (10.0 cm long, horsehair fiber), and the third () was a watercolor paintbrush made of pig hair fibers.
Figure 1. Images of the brushes and adsorption system a) the test-tube cleaning brush (1.5 × 9.5 cm) before coating, b) the test-tube cleaning brush (2.5 × 10.0 cm) before coating, c) the watercolor paintbrush before coating, d) the brush-A after coating with magnetite, e) the brush-B after coating with magnetite, f) the brush-C after coating with magnetite, g) the glass column and adsorbent separately, and h) the fixed bed adsorption column.
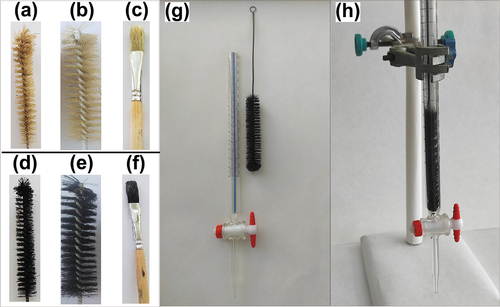
Procedure for the preparation of the adsorbent
An economical laboratory cleaning brush was used as the material to which magnetite, an adsorptive material, was attached. Fe(II) (0.1 M, prepared from FeCl2.4 H2O) and Fe(III) (0.2 M, prepared from FeCl3.6 H2O) solutions were added on the brush placed in a glass beaker large enough to contain all the brush fibers. Twenty-four hours later, the solution was decanted, and the nanoparticles were formed by adding NaBH4 solution (4% w/v) to the brush. After an hour, the brush was removed from the beaker, washed with distilled water and acetone, and dried at room temperature.
Characterization
Thermo Scientific Apreo S model scanning electron microscopy (SEM) and energy-dispersive X-ray analysis (EDX) were used to obtain high-resolution images and determine the elemental composition of the adsorbent (shown in ).
Adsorption studies
An element solution (at a concentration of 1000 µg/L) was prepared by diluting the ICP multi-element QC Standard solution (QCS-27, containing As, Se, Mo, Cd, Tl, and Pb at 100 mg/L). Before diluting with ultrapure water, the pH of the solution was adjusted to 4.0 with HCl or NaOH solutions. The brush-type adsorbent was inserted into a column (with a diameter of 1.0 cm and a height of 30 cm), and the element solution was passed through the column at a constant flow rate (1.0 mL/min). Quadrupole ICP-MS measurements were performed using the Agilent 7900 model ICP-MS instrument. Element concentrations before and after passing through the column were determined. Removal efficiencies are calculated according to EquationEquation 1(1)
(1) .
where,
Ci is the initial element concentration, µg/L,
Ce is the element concentration after adsorption, µg/L.
Kinetic studies
In order to test the experimental data, kinetic models were used for the investigation of the sorption mechanism and the rate controlling steps. An elaborate kinetic study was performed for this purpose. Four different kinetic models for six different elements were applied to the experimental data. The adsorption kinetics of arsenic, selenium, cadmium, molybdenum, thallium, and lead were examined using the Lagergren pseudo first-order (Ho and McKay Citation1998), the pseudo second-order (Ho and McKay Citation1999), the Elovich (Ho and McKay Citation1998) and the Weber Morris intraparticle diffusion (Weber and Morris Citation1963) models. For this purpose, 40 mL of 1000 µg/L element solution at pH 4.0 was added onto the brush-type adsorbent and shaken for 15; 30; 75; 210; 300; 360; 1290 and 1410 min at 25°C to elucidate the adsorption kinetics. The solutions were analyzed by ICP-MS. The experimental data were fitted to the pseudo first-order (EquationEquation 2(2)
(2) ), the pseudo second-order (EquationEquation 3
(3)
(3) ), the Elovich (EquationEquation 4
(4)
(4) ), and the intraparticle diffusion (EquationEquation 5
(5)
(5) ) models. The equations are;
where,
qe and qt (µg/g) are the amount of adsorbed elements on the adsorbent at equilibrium and time t, respectively.
t, time (min),
k1 (1/min) is the rate constant of the pseudo-first-order adsorption,
k2 (g/µg.min) is the rate constant of the pseudo-second-order adsorption,
α is the initial adsorption rate (mg/g.min) to the extent of the surface coverage and β (mg/g.min) is the desorption constant related to the activation energy for chemisorptions.
kint (µg/g.min0.5) is the rate constant of the intraparticle diffusion.
Results and discussion
The brush-type adsorbent
The brushes to be coated with magnetite were prepared as described earlier. All the three varieties viz.; the test tube cleaning brush, the cleaning brush, and the watercolor paintbrush were effectively coated with nanoparticles ( ,(d–f)). It can be seen from the figure that the color of the uncoated brush bristles, which are beige () in their natural state, is black () as expected after magnetite coating. Experiments were continued using the brush shown in due to its shape being consistent with adsorption studies.
With the prepared adsorbent, a single movement will be sufficient to remove the adsorbent from the media, making the process easy. The prepared adsorbent will simplify the adsorption studies and allow cost reduction. Because fiber species’ processing method and size can vary, the brush-type adsorbent can be produced in various shapes and sizes for different applications. Its ability to modify the adsorptive material coated on the fibers provides endless application possibilities. For example, chemical substances at microsizes, nanoparticles, and molecularly imprinted polymers can be coated on the fibers based on the purpose of application. In case wastewater is discharged into the environment after industrial activities, it is necessary to give the water to the environment in accordance with allowable limits for trade effluent discharge to watercourse. The brush-type adsorbent is ideal for adsorbing pollutants by placing them inside pipes in discharge units. The adsorbent will be suitable for use not only in the liquid phase but also in the gas phase (e.g., adsorption of air pollutants). Since it is suitable for coating various materials on brush fibers, it will be more effective, efficient, and long-lasting than current filters. The brush-type adsorbent can be used not only for chemical contaminants but also for living organisms. If nanosilver is coated on the brush fibers, it can be used to disinfect against viruses, bacteria, and fungi. Besides applying the currently established techniques for adsorbent preparation, our method may also be applied to newly discovered techniques in the future.
Characterization
show the SEM images of the brush fibers before coating at 10,000× and 100,000× magnifications, respectively. shows the layers (cuticles) on the surface. , which is a closer shot, shows the smoothness of the fiber surface. In comparison to the uncoated fibers on the surface (), the nanoparticles formed on the fibers after coating can be observed in . also shows aggregates and individual nanoparticles. A closer shot (at a magnification of 100,000×) reveals that the nanoparticles on the surface are singular and spherical (). In addition, this figure shows that the average size of the nanoparticles is about 60 nm.
Figure 2. SEM images of the fibers a) the uncoated fiber at 10,000× magnification, b) the uncoated fiber at 100,000× magnification, c) the magnetite-coated fiber at 10,000× magnification, and d) the magnetite-coated fiber at 100,000× magnification.
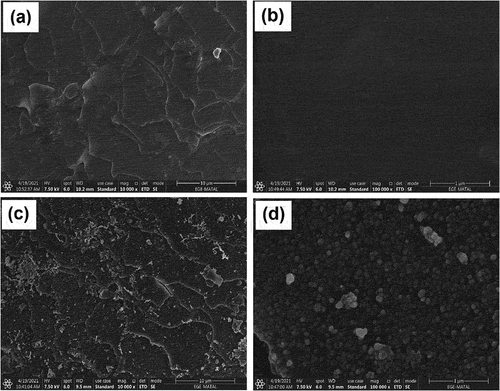
SEM-EDX analysis results () showed that the uncoated fibers are mainly (99.3% by atomic) made up of carbon (C), oxygen (O), and sulfur (S). Carbon is considered the dominant element in mammalian hair, and the hair matrix does not differ among mammals (Emara et al. Citation2013). EDX analysis also confirmed the presence of iron on the adsorbent ().
Adsorption studies
As shown in , the adsorption analysis yielded promising results. In addition to being advantageous in terms of usage and cost, it was determined to successfully remove toxic elements from water.
Table 1. The removal efficiencies (%) of the elements using the brush-type adsorbent (initial element concentrations were 1000 µg/L, n = 3).
In the proposed method, since the fibers on which the adsorptive material will be coated is attached to a single main body, a single movement will be sufficient to remove it from the environment. Therefore, no adsorbent loss is expected as the adsorptive material is attached to the fibers.
There is no need to use glass wool in column studies with this new type of adsorbent because the brush-type adsorbent cannot clog the column outlet. Similarly, it is impossible to disrupt the column filling arrangement. At this stage, superiority in terms of material and effort is achieved. In addition, the ease with which the adsorbent can be placed in the column provides a significant benefit over particle-type adsorbents. While it takes 10 s for the brush-type adsorbent to be inserted into the column, it takes at least 10 min to prepare a column of the same size. Similarly, when the process is complete, the brush-type adsorbent is removed from the column much quicker than other adsorbents. Like the column method, advantages are obtained in the batch adsorption method. As an alternative to the agitation method used to increase contact during the adsorption process, the ability of the brush to be rotated from a single center can also be used. Again, after the adsorption, the brush-type adsorbent can be removed from the environment in a single action without the need for any additional processes such as filtration, decantation, separation with a magnet, and centrifugation. Similarly, during the regeneration processes, the aforementioned ease of use, minimal processing, and low cost are all crucial considerations.
Kinetic studies
The pseudo-first-order adsorption, the pseudo-second-order adsorption, the Elovich, and the intraparticle diffusion model plots of the elements were drawn using the adsorption kinetics data and are shown in , respectively. The constants k1, k2, α, β, and kint were calculated from the slopes and the intercepts of the plots and are shown in .
Figure 4. Kinetic model for the adsorption of arsenic, selenium, molybdenum, cadmium, thallium and lead a) the pseudo first-order kinetic model, b) the pseudo second-order kinetic model, c) the Elovich model, d) Weber Morris Intraparticle diffusion model.
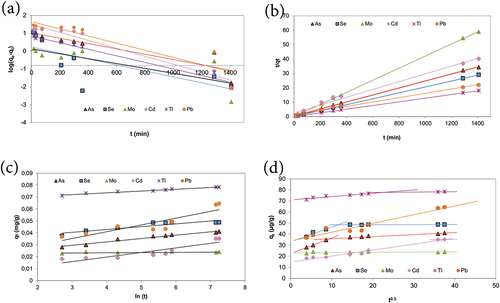
Table 2. Kinetic model equations, constants, and R2 values for the adsorption (40 mL of 1000 µg/L element solution at pH 4.0) using brush-type adsorbent.
The linear fit indicates that the adsorption follows the pseudo-second order model best (R2 > 0.990 for all the elements studied). Also, the theoretical qe values for the adsorption of As (41.2 µg/g), Se (48.8 µg/g), Mo (23.9 µg/g), Cd (37.0 µg/g), Tl (78.7 µg/g), Pb (66.7 µg/g) are very close to the experimental values As (41.1 µg/g), Se (48.7 µg/g), Mo (24.0 µg/g), Cd (35.1 µg/g), Tl (78.3 µg/g), Pb (64.4 µg/g) with the pseudo-second order model. For all the elements studied, the correlation coefficients (R2) for the pseudo-second-order model were higher as compared to all the elements. It was concluded that the pseudo-second-order adsorption mechanism was predominant and the overall rate of the adsorption process of As, Se, Mo, Cd, Tl, and Pb appeared to be controlled by chemical processes. Febrianto et al. reported that, compared to the pseudo-first-order model, the pseudo-second-order model is more suitable for representing kinetic data in heavy metal biosorption systems. This indicates that the rate limiting steps are chemisorptions (such as valence forces through sharing or exchange of electrons between sorbent and sorbate, complexation, coordination, and/or chelation) in biosorption (Febrianto et al. Citation2009).
Since the pseudo-first-order adsorption and the pseudo-second-order adsorption models do not identify the diffusion mechanism, kinetic results were also analyzed using the intraparticle diffusion model. According to the intraparticle diffusion model, qt - t0.5 pilot should give a straight line with a slope of kint and an intercept of C. If the linear fit of the data points is linear and passes through the origin, then intraparticle diffusion is the sole rate-limiting step. As shown in , the plots for the elements are not linear implying that intraparticle diffusion is not the only rate-limiting step for arsenic, selenium, cadmium, and thallium. It is seen that the adsorption process tends to be followed by two phases. Two phases in the intraparticle diffusion plots suggest that the adsorption process proceeds by surface adsorption and intraparticle diffusion. The intraparticle diffusion rate constants (kint1 and kint2) for each phase are shown in . Also, the linear fit of the data points is linear for molybdenum and lead but again does not pass through the origin, and intraparticle diffusion is not the sole rate-limiting step.
These preliminary experiments were conducted to prove the effectiveness of the brush-type adsorbent. For each element, elaborate studies can be conducted to determine the working and removal conditions. Moreover, it is also possible to work with numerous adsorptive materials other than magnetite.
Conclusions
In this study, an innovative method that will contribute a new perspective to the literature is presented. The brush fibers were successfully coated with nano-sized (60 nm) magnetite. The adsorption studies showed that, the tested elements were adsorbed with high removal efficiencies. The removal efficiencies (%) were 91.0 ± 0.4, 83.4 ± 0.3, 85.9 ± 0.4, 79.7 ± 1.0, 93.7 ± 0.2, 91.9 ± 0.3 for arsenic, cadmium, molybdenum, lead, selenium, and thallium, respectively. According to the R2 values (>0.99 for all the elements studied) and the experimental qe values (close to the theoretical qe values) the pseudo-second-order model best fitted to the experimental data. This explains that the adsorption is controlled by chemical processes. The brush-type adsorbent to be prepared for purpose will provide a different perspective to existing adsorption studies and pave the way for future research.
I confirm that this manuscript has not been published elsewhere and is not under consideration by another journal.
Highlights
A novel adsorbent, brush-type adsorbent, was synthesized.
Magnetite, the adsorptive material, successfully formed on the bristles.
The particle size was determined to be about 60 nm.
The adsorbent is suitable for all adsorption methods and toxic substances.
It is a new easy-to-use design for economic, efficient, and high-performance adsorbents.
Acknowledgments
This study was deemed worthy of support for patent application and the application costs were covered by Ege University, Turkey. Ege University applied to the Turkish Patent and Trademark (filed on October 13, 2017) and the International Patent System–WIPO (filed on 5 October 2018) for the “brush-type adsorbent” described in this study, the inventor of which is Tülin Deniz Çiftçi (application numbers are 2017/15670 and PCT/TR2018/050557 for TURKPATENT and WIPO, respectively).
Patent application correspondence was made by Ege University, Science and Technology Centre, Turkey.
This study was carried out with the facilities of Ege University, Faculty of Science, Department of Chemistry, Turkey.
I am grateful to Ege University Planning and Monitoring Coordination of Organizational Development and Directorate of Library and Documentation for their support in editing and proofreading service of this study.
Disclosure statement
No potential conflict of interest was reported by the authors.
References
- Ali, I. 2012. New generation adsorbents for water treatment. Chemical Reviews 112 (10):5073–10. doi:10.1021/cr300133d.
- Bryjak, M., N. Kabay, B. L. Rivas, and J. Bundschuh. 2016. Innovative materials and methods for water treatment: Solutions for arsenic and chromium removal. 1st. London: CRC Press. doi:10.1201/b19577.
- Dąbrowski, A. 2001. Adsorption — from theory to practice. Advances in Colloid and Interface Science 93 (1–3):135–224. doi:10.1016/S0001-8686(00)00082-8.
- Emara, E. M., H. Imam, M. A. Hassan, and S. H. Elnaby. 2013. Biological application of laser induced breakdown spectroscopy technique for determination of trace elements in hair. Talanta 117:176–83. doi:10.1016/j.talanta.2013.08.043.
- Febrianto, J., A. N. Kosasih, J. Sunarso, Y. -H. Ju, N. Indraswati, and S. Ismadji. 2009. Equilibrium and kinetic studies in adsorption of heavy metals using biosorbent: a summary of recent studies. Journal of Hazardous Materials 162 (2–3):616–45. doi:10.1016/j.jhazmat.2008.06.042.
- Hassan, M. S., and M. H. Zohdy. 2018. Adsorption kinetics of toxic heavy metal ions from aqueous solutions onto grafted jute fibers with acrylic acid by gamma irradiation. Journal of Natural Fibers 15 (4):506–16. doi:10.1080/15440478.2017.1330721.
- Ho, Y. S., and G. McKay. 1998. A comparison of chemisorption kinetic models applied to pollutant removal on various sorbents. Process Safety and Environmental Protection 76 (4):332–40. doi:10.1205/095758298529696.
- Ho, Y. S., and G. McKay. 1999. Pseudo-second order model for sorption processes. Process Biochemistry 34 (5):451–65. doi:10.1016/S0032-9592(98)00112-5.
- Marczenko, Z., and M. Balcerzak. 2000. Separation, preconcentration and spectrophotometry in inorganic analysis. ed. E. Kloczko, 1st, Amsterdam: Elsevier Science.
- Nitayaphat, W., and T. Jintakosol. 2020. Adsorption of Ag (I) from aqueous solutions using regenerated silk fibroin adsorbent beads. Journal of Natural Fibers 19 (9):3365–77. doi:10.1080/15440478.2020.1848697.
- Paramasivam, S. K., D. R. Panneerselvam, D. Panneerselvam, K. N. Shiva, and U. Subbaraya. 2020. Influence of operating environments on adsorptive removal of lead (Pb (II)) using banana pseudostem fiber: Isotherms and kinetic study. Journal of Natural Fibers 19 (12):4485–95. doi:10.1080/15440478.2020.1863295.
- Wang, J., and X. Guo. 2020. Adsorption isotherm models: Classification, physical meaning, application and solving method. Chemosphere 258:127279. doi:10.1016/j.chemosphere.2020.127279.
- Weber, W. J., and J. C. Morris. 1963. Kinetics of adsorption on carbon from solutions. Journal of the Sanitary Engineering Division 89 (2):31–39. doi:10.1061/JSEDAI.0000430.
- Zhang, H., F. Carrillo-Navarrete, and C. Palet-Ballús. 2022. Human hair biogenic fiber as a biosorbent of multiple heavy metals from aqueous solutions. Journal of Natural Fibers 19 (6):2018–33. doi:10.1080/15440478.2020.1798841.
- Zolotov, Y. A., and N. M. Kuz’Min. 1990. Preconcentration of trace elements. Netherlands: Elsevier.