ABSTRACT
Cellulose fibers come from fast-growing renewable crops which characteristically are sustainable making them a desirable option for textile structures. Commercial medical Cannabis cultivation is a fast-growing market. Marijuana stems, which are often discarded or burnt, due to the absence of tetrahydrocannabinol (THC) provide an opportunity for the manufacturing of fibers. In this manuscript, the physical, chemical, morphological, and mechanical properties of Cannabis sativa L. stems, coming from medicinal marijuana grown crops, were analyzed to assess their viability for textile use. The content of hemicellulose, pectin, lignin, and cellulose in the fibers was assessed, and we found that the mechanical properties of these fibers are comparable to those of similar natural fibers such as coconut, hemp, sisal, and jute. The FTIR spectra showed all characteristic peaks present in most cellulosic substrates. We demonstrated that cellulosic fibers can be obtained via water-retting of marijuana plants hence opening an avenue for the utilization of agricultural waste in a myriad of potential applications such as water filtration, packaging, and rheological modifiers.Abbreviation: Terms: THC (tetrahydrocannabinol); CBD (cannabidiol); PVC (polyvinyl chlorides); SEM (scanning electron microscopy); FTIR (Fourier transform infrared).
摘要
纤维素纤维来自快速生长的可再生作物,其特点是可持续性,使其成为纺织结构的理想选择. 商业药用大麻种植是一个快速增长的市场。由于缺少四氢大麻酚(THC),大麻茎通常被丢弃或燃烧,这为纤维的制造提供了机会. 在这篇手稿中,分析了大麻茎的物理、化学、形态和机械特性,以评估其在纺织方面的可行性. 评估了纤维中半纤维素、果胶、木质素和纤维素的含量,我们发现这些纤维的机械性能与类似的天然纤维(如椰子、大麻、剑麻和黄麻)的机械性能相当. FTIR光谱显示了大多数纤维素基质中存在的所有特征峰. 我们证明,纤维素纤维可以通过大麻植物的水沤制获得,从而为农业废弃物在水过滤、包装和流变改性剂等多种潜在应用中的利用开辟了一条道路.
Introduction
Natural fibers are commonly classified as cellulose and protein based. Cellulose fibers come from fast-growing renewable crops which are sustainable making them a desirable option for fashion textiles (Gullingsrud Citation2017). Fibers such as hemp, jute, flax, bamboo, and kenaf are biodegradable, low cost, efficient at carbon dioxide sequestration, have low density, and are tough (Senwitz et al. Citation2016) allowing easier recyclability and textile longevity. Natural fibers have a low carbon footprint compared to that of synthetic-based textiles which derive from petrochemical sources (Mohammad Citation2016). An increasing understanding of the environmental importance of natural fiber is being driven by major fashion brands as well as consumers’ awareness and desirability, accelerating a shift to forest friendly fibers (Furness Citation2022).Cannabis sativa is a genus of an herbaceous plant originally from eastern Asia (Small Citation2017) and it has spread worldwide. Cannabis plants have been used for cloth creation dating back 9000 to 100,000 BC (Ingrouille and Eddie Citation2006). This plant species contains molecular components known as cannabinoids, which consist mainly of tetrahydrocannabinol (THC) which influences human psyche, and cannabidiol (CBD), which reduces psychoactive impact The Cannabis plant commonly known as “hemp” or “industrial hemp” has a lower content of THC but contains higher CBD, so it is used as a bast fiber in the textile industry due to its antimicrobial, durable, and breathable properties. The plant and seeds known as “marijuana” are grown for medicinal and recreational purposes due to its psychoactive intoxicant properties (Small Citation2017). For this study, we are focusing on marijuana stems which are often discarded or burnt, due to the absence of THC. While it is common to cutdown the plants after harvesting, making crops more efficient, approximately 90% of the plant is discarded or wasted after each season. The stems are not commonly used in fiber extraction because of their shorter length of 1–2 m (Small Citation2017) when compared to hemp which can extend from 3.5 to 4 m(Jonaitienė, Jankauskienė, and Stuogė Citation2016).Agricultural waste produced account for millions of tones on a yearly basis, and produce such as corn and sorghum stalks, rice husks, banana, and pineapple leaves and soybean straw has been used as a source of cellulose fibers (Mohammad Citation2016). Therefore, marijuana stems, a by product from discarded stalks used by the marijuana medical industry, offer an opportunity for textile fiber manufacturing. Using discarded agricultural materials creates an opportunity to reduce the use of synthetic (Suryanto et al. Citation2014). In order to qualify the viability of these discarded Cannabis sativa L. stems for textile performance, their physical, chemical, morphological, and mechanical properties were analyzed, and their content of hemicellulose, pectin, lignin, and cellulose were determined.
Materials and methods
Material
The research material used in this study consists of a hybrid between Cannabis sativa and Cannabis indica, which we will now refer to Cannabis sativa L., stalks as shown in . Samples were obtained from agricultural waste corresponding to 4.5-month-old plants, grown for medicinal purposes, with an average height of 1.2 meters found in the Santa Elena district, in Medellín, Colombia. Stalks were harvested after panicles were cut off for THC extraction.
Fiber extraction
Fiber extraction of the Cannabis sativa L. was done through a water-retting process (). The length of each dry stalk was measured, ranging between one and two meters from the base. Stalks were divided into five bundles each weighing on average 70 grams (measured on a KERN EW Precision Balance scale). The five bundles were submerged into five polyvinyl chloride (PVC) tubes (1500 mm in length and 150 mm wide). Approximately 13 to 14 liters of tap water with a pH of 5, alkalinity of 110, chloride of 0.2, and hardness of 0 were obtained from the textile department facilities to fill each PVC tube. The water temperature in the PVC tubes ranged between 17 °C and 17.5 °C. The tubes were sealed with a PVC cap and a 100 mm opening was left for oxygenation. Stalks were soaked for 14 days inside the textile laboratory facilities. After 14 days of incubation, stalks were removed from the tubes by manually separating the fiber (approximately for 1 hour). The peeled wet fiber was easily detached from the stalk from top to bottom to avoid fiber fractures.
Figure 2. Fiber extraction process. Cannabis sativa L. stalk fiber (A and B) peeling; (C) washing; (D) separation for the drying process.
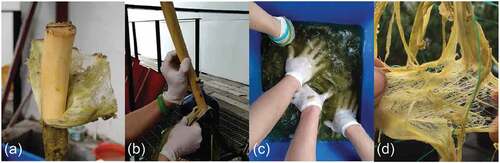
The peeled fibers were washed and cleaned using a solution prepared by adding 80 grams of sodium bicarbonate into 8 liters of water inside a polystyrene container and then scrubbed by hand for 30 minutes. The fibers were separated by bundles (from one to five) and dried for two days on a clothesline using the natural air current. Once dry, the fibers were stored in plastic bags and weighed per group on a KERN EW Precision Balance scale. Bundle #1 weighted 12.4 g, bundle #2 weighted 8.2 g, bundle #3 weighted 38.5 g, bundle #4 weighted 41.8 g and bundle #5 weighted 48.2 g.
The spinning process was done by artisans in Ramiriquí, Boyacá (143.5 kilometers from Bogotá), in Colombia (). The raw dry fiber was brushed and carded by hand into finer fibers using wooden brushes and hair combs for approximately 30 minutes separating and discarding imperfections and low-quality fibers. The finer raw fiber bundles were twisted to obtain a yarn sample. The yarn’s tip was attached to a wooden spindle machine and then twisted manually until a skein of yarn was formed.
Figure 3. Carding and Spinning process: (a) Carding; (b) Combing fibers; (c and d) Spinning; (e) Fiber length; (f) Weaved textile sample; (g) Weaved close-up sample; and (h) Cannabis sativa L. spun fiber sample.
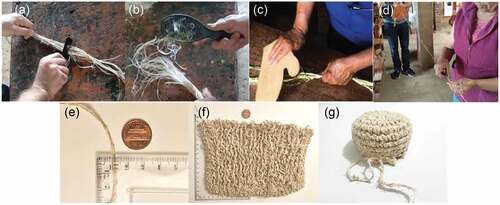
Analysis of structural components in natural fiber samples
Cellulose and lignin percentages were determined according to the Kürschner and Höffer method and the Klason method according to TAPPI 222. Hemicellulose was determined as the difference between holocellulose (Jayme-Wise method) and cellulose. Moisture content in the fibers was determined according to ASTM D2495–07.
The first step for cellulose and lignin analysis involves extractive removal from the fiber sample. Extractives (non-structural components such as fats, waxes, proteins, gums, terpenes, resins, glycosides, saponins, fatty acids, pectins, sugars, starches, phenolic compounds, and flavonoids) isolation was performed by Soxhlet extraction using an eluotropic series of benzene and ethanol. The extractive-free sample was used to determine lignin and cellulose (see below).
Cellulose was determined by the Kürschner and Höffer method. The extractive-free fiber sample was dispersed in a nitric acid-ethanol mixture (1:4 v/v) and heated for one hour. The insoluble solid was isolated by filtration and subjected twice to the same treatment (dispersion on a nitric acid-ethanol mixture and heated for 1 hour). The solid residue was washed with distilled water until obtaining a neutral pH. Then it was dried and weighed to determine the cellulose content.
Lignin was determined by the Klason method according to TAPPI 222. The sample measured free from extractives was hydrolyzed with concentrated sulfuric (reflux, 30 min), and then diluted to continue the reaction for five hours. The insoluble substrate corresponded to lignin.
Holocellulose was determined by the Jayme-Wise method. The sample of extractive-free fibers was heated (70°C) in an aqueous mixture of acetic acid and sodium chlorite. A fresh solution of acetic acid/sodium chloride was added to the reaction mixture at one-hour intervals four times. The fiber samples were left in the acidified chlorite solution for 12 h at 70°C. The solid residue was washed with distilled water until obtaining a neutral pH and then dried and weighed to determine holocellulose (a mixture of cellulose and hemicellulose).
The fiber extraction method used for the study was replicated two additional times using the same materials and conditions with similar success rates ().
Physical and mechanical properties of natural fibers
Physical properties
Moisture determination test in plastics by weight loss. Moisture content was evaluated by weight loss according to the American Society for Testing and Materials (ASTM) D 6980–17 standard test method using a Precisa XM 120-HR analytical balance. The sample was dried in an oven at 105°C until a constant weight was obtained. The amount of moisture was calculated considering the difference in the net weight of the oven-dried sample compared to the net weight of the initial sample. A total of five readings per sample were obtained.
Density test on plastics by immersion. The density of the sample was evaluated according to the ASTM D 792–20 standard test method for density and specific gravity of plastics by displacement. The test method used was the one described by the “B-for testing solid plastics in liquids other than water” section of the standard. Ethanol was used as the immersion liquid. The test equipment used was a Sartorius Extend 220 g analytical balance, with a resolution of 0.0001 g. A total of five readings per sample were obtained.
Mechanical properties
Tensile properties of yarns by the single-strand method. Tensile properties were evaluated according to the ASTM D2256/2256 M standard test method. An INSTRON universal machine equipped with a 0.5 kN load cell was used. The standard conditions in the test room were 20.1°C and relative humidity of 57.7%.
Specimens with a nominal width of 12.41 mm and a nominal thickness of 10.56 mm were used. The test was carried out with an effective length of 250 mm and a test speed of 300 mm min−1, with the aim of maintaining a constant breaking time. To obtain average test results, the test was conducted on 10 samples. Results obtained present high dispersion due to the shape of the fiber since its braiding varies along its length.
Fourier transform infrared (FTIR) analysis
Two samples of the Cannabis sativa L. fiber, coming from medicinal marijuana grown crops, were placed on a FTIR- ATR (Attenuated Total Reflectance) cell from PICO instruments which was connected to a ThermoNicolet FTIR microscope. The samples were scanned 64 times in the IR spectra and the average of the signals is presented in the spectra of .
Results and discussion
Cannabis sativa L. fibers morphology
Fibers obtained varied from 10 to 40 mm long, with a linear weight varying between 12 to 20 denier, and a content of fiber impurities of around 30%. The morphology of the Cannabis sativa L. stems was analyzed using scanning electron microscopy (SEM) as shown in . The morphological characteristics of the Cannabis specimens show a fibrous and stringy surface.
Chemical properties analysis
Physical and Mechanical Properties of Cannabis sativa L. Fibers
Physical properties. shows the results for moisture content and density of the sample. The average value of moisture content was 8.14% and the average density at 23 ºC was 1.34 g cm−3. These results are comparable to those reported for hemp, coir, sisal, jute, cotton, and abaca where moisture content ranges from 6.5 to 15% and density values range from 1.15 g cm−3 and 1.60 g cm−3 ().
Table 1. Cannabis sativa L. physical properties.
Table 2. Physical properties reported for other natural fibers.
Mechanical properties. shows the values obtained for breaking load, p rup [N], maximum resistance [MPa], elongation at maximum load, L max [%] of the analyzed samples, and their respective average. A maximum elongation value of 9.88% is reported. Results obtained when measuring mechanical properties showed a high dispersion because of the non-uniform shape of the fiber since the braiding was done manually. For this reason, maximum stress values were not calculated, given that they are highly dependent on the cross-sectional area.
Table 3. Mechanical properties of 10 fiber samples.
illustrates the behavior of the displacement (mm) versus tensile force at the various loads.
Figure 6. Tensile force versus displacement evaluated according to the ASTM D2256/2256 M standard test method. To obtain average test results, the test was conducted on 10 different samples of the Cannabis sativa L. fiber obtained from medicinal marijuana grown crops.
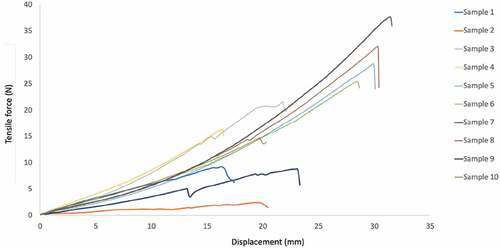
shows the average elongation percentage at a maximum load in comparison to values reported in the literature (Silva et al. Citation2000; Simbaña et al. Citation2020). The values we report are the average of 10 samples. The standard deviation of the measurements is also reported. Maximum tensile elongation values were relatively high compared to those of other fibers such as hemp (1.6%), jute (1.16–1.5%), and sisal (7–8%).
Table 4. Mechanical properties of the Cannabis sativa L. fiber were analyzed in comparison to other fibers found in the literature.
Our fibers present variations in elongation at maximum load when compared to other fibers (). For example, a significant increase in elongation at maximum load was observed for the Cannabis sativa L. fiber (9.88%) in comparison to hemp (1.6%).
Fourier transform infrared (FTIR) analysis
shows the absorbance FTIR spectra of the Cannabis sativa L. specimen. The spectra show peaks at 3400 indicating O-H stretching, 2900 which corresponds to C-H stretching, 1600 which indicates stretching vibrations of O-H bonds, and a large one at 1000 cm−1 corresponding to the C-O-C vibration of the pyranose ring. All these peaks are traditionally noted in most cellulosic substrates. The inset in shows an optical image of the area used by the FTIR microscope to obtain the spectra. It is important to note that the optical image also shows qualitative similarities with the SEM images in .
Conclusions
We report on the successful extraction of fibers from discarded Cannabis sativa L. stems coming from plants grown for medicinal purposes using a water-retting process. We demonstrated that the fibers could be easily processed via knitting and their mechanical properties are comparable to those of similar natural fibers such as coir, hemp, sisal, and jute. Considering we used an agricultural by-product as a raw material, the proposed methodology allows for maximum utilization of all resources in Cannabis crops. The reuse of agricultural waste promotes a circular economic model for the Cannabis industry.
The chemical composition of the surface of the two Cannabis specimens studied in this project was identical as evidenced by the FTIR analysis. The spectra showed all characteristic peaks of most cellulosic substrates. This paper shows that cellulosic fibers can also be obtained via water-retting of marijuana plants which opens an avenue for the utilization of agricultural waste in a myriad of potential applications such as water filtration, packaging, and rheological modifiers.
Highlights
Cellulose fibers come from fast-growing renewable crops
Cannabis sativa L. stems are discarded or burnt, due to the absence of THC
Cellulosic fibers can be obtained via water-retting from Cannabis sativa L. stems
The fibers can be processed via knitting
Mechanical properties are comparable to those of coir, hemp, sisal, and jute
Acknowledgements
We thank Annie Amaya for her valuable support during the analyses of the physical characterization of the fibers. We also thank Cannalivio for providing the Cannabis sativa L. stem samples.
Disclosure statement
No potential conflict of interest was reported by the author(s).
References
- Furness, V. 2022. “Retailers accelerate shift to forest friendly fibres at COP27.” Reuters, November 14, 2022, sec. COP27. https://www.reuters.com/business/cop/retailers-acclerate-shift-forest-friendly-fibres-cop27-2022-11-14/.
- Gullingsrud, A. 2017. Fashion fibers: Designing for sustainability. New York, NY, USA: Bloomsbury Publishing USA.
- Ingrouille, M., and B. Eddie. 2006. Plants: Diversity and evolution. New York, NY, USA: Cambridge University Press.
- Jonaitienė, V., Z. Jankauskienė, and I. Stuogė. 2016. Hemp cultivation opportunities and perspectives in lithuania. In Natural fibres: Advances in science and technology towards industrial applications, ed. R. Fangueiro and S. Rana, 407–10. New York, NY, USA: Springer.
- Li, Y., and Y. O. Shen. 2015. The use of Sisal and Henequen fibres as reinforcements in composites. In Biofiber reinforcements in composite materials, ed. O. Faruk and M. Sain, 165–210. Sawston, Cambridge, United Kingdom: Elsevier.
- Madsen, B., P. Hoffmeyer, A. Belinda Thomsen, and H. Lilholt. 2007. Hemp yarn reinforced composites–i. yarn characteristics. Composites Part A, Applied Science and Manufacturing 38 (10):2194–203. doi:10.1016/j.compositesa.2007.06.001.
- Mohammad, F. 2016. Sustainable natural fibres from animals, plants and agroindustrial wastes—an overview. Sustainable Fibres for Fashion Industry 31–44.
- Oksman, K., L. Wallström, L. A. Berglund, and R. Dias Toledo Filho. 2002. Morphology and mechanical properties of unidirectional sisal–epoxy composites. Journal of Applied Polymer Science 84 (13):2358–65. doi:10.1002/app.10475.
- Ponce, D. S., and V. Guerrero. 2014. Propiedades Mecánicas de Compuestos Biodegradables Elaborados a Base de Ácido Poliláctico Reforzados Con Fibras de Abacá. Revista Politécnica 33 (2). https://revistapolitecnica.epn.edu.ec/ojs2/index.php/revista_politecnica2/article/view/56
- Rinawa, M., P. Chauhan, D. Suresh, S. Kumar, and R. Santhosh Kumar. 2021. A review on mechanical properties of natural fiber reinforced polymer (nfrp) composites. Materials Today: Proceedings. doi:10.1016/j.matpr.2021.07.275.
- Senwitz, C., A. Kempe, C. Neinhuis, J. Lau Mandombe, M. Futuro Branquima, and T. Lautenschläger. 2016. Almost forgotten resources–biomechanical properties of traditionally used bast fibers from Northern Angola. BioResources 11 (3):7595–607. doi:10.15376/biores.11.3.7595-7607.
- Shahzad, A. 2012. Hemp fiber and its composites–a review. Journal of Composite Materials 46 (8):973–86. doi:10.1177/0021998311413623.
- Silva, G. G., D. A. De Souza, J. C. Machado, and D. J. Hourston. 2000. Mechanical and thermal characterization of native Brazilian coir fiber. Journal of Applied Polymer Science 76 (7):1197–206. doi:10.1002/(SICI)1097-4628(20000516)76:7<1197:AID-APP23>3.0.CO;2-G.
- Simbaña, E. A., P. E. Ordóñez, Y. F. Ordóñez, V. H. Guerrero, M. C. Mera, and E. A. Carvajal. 2020. Abaca: Cultivation, obtaining fibre and potential uses. In Handbook of natural fibres, ed. R. M. Kozłowski and M. Mackiewicz-Talarczyk, 197–218. Sawston, Cambridge, United Kingdom: Elsevier.
- Small, E. 2017. Classification of Cannabis sativa l. in relation to agricultural, biotechnological, medical and recreational utilization. In Cannabis Sativa L.-Botany and Biotechnology, ed. S. Chandra, H. Lata, M. A. ElSohly, 1–62. New York, NY, USA: Springer.
- Suryanto, H., E. Marsyahyo, Y. Surya Irawan, and R. Soenoko. 2014. Morphology, structure, and mechanical properties of natural cellulose fiber from mendong grass (fimbristylis globulosa). Journal of Natural Fibers 11 (4):333–51. doi:10.1080/15440478.2013.879087.
- Yan, L., N. Chouw, L. Huang, and B. Kasal. 2016. Effect of alkali treatment on microstructure and mechanical properties of coir fibres, coir fibre reinforced-polymer composites and reinforced-cementitious composites. Construction and Building Materials 112:168–82. doi:10.1016/j.conbuildmat.2016.02.182.