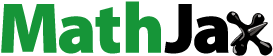
ABSTRACT
The current study investigated the use of polyester synthetic (PES) fiber and basalt (BS) and bagasse (BG) natural fibers on the strength and mechanical behavior of sandy soil in pavement construction projects. A series of California bearing ratio (CBR) and indirect tensile strength (ITS) tests were performed on fiber contents (FC) of 0.5,1 and 2% and fiber lengths (FL) of 2.5, 5, and 7.5 mm. Findings show that the maximum California bearing ratio (by increasing 3.3 times) and indirect tensile strength (by increasing 2.98 times) are obtained in the length of 7.5 mm and the content of 2% for polyester and basalt fibers, respectively, which is also evident in the scanning electron microscope (SEM) test results. Also, based on the analysis of variance (ANOVA) and according to various factors including P-value (<0.05), it was determined that the contrast between the values is statistically significant. Due to California bearing ratio – indirect tensile strength and California bearing ratio – resilient modulus experimental relationships, the relationship between resilient modulus-indirect tensile strength was provided with very good correlation coefficients. Furthermore, sensitivity analysis revealed that California bearing ratio and indirect tensile strength values are noticeably influenced by fiber content variations.
摘要
本研究调查了聚酯合成(PES)纤维、玄武岩(BS)和甘蔗渣(BG)天然纤维在路面建设项目中对沙土强度和力学性能的影响. 对0.5、1%和2%的纤维含量(FC)和2.5、5和7.5 mm的纤维长度(FL)进行了一系列加州承载比(CBR)和间接拉伸强度(ITS)测试. 结果表明,聚酯纤维和玄武岩纤维的最大加州承载比(增加3.3倍)和间接拉伸强度(增加2.98倍)分别在7.5 mm的长度和2%的含量下获得,这在扫描电子显微镜(SEM)测试结果中也很明显. 此外,基于方差分析(ANOVA),并根据包括P值(<0.05)在内的各种因素,确定值之间的对比具有统计学意义. 由于加州承载比-间接抗拉强度和加州承载比–弹性模量的实验关系,弹性模量-间接拉伸强度之间的关系具有非常好的相关系数. 此外,敏感性分析表明,加州承载比和间接抗拉强度值明显受纤维含量变化的影响.
Introduction
Nowadays, the increase in population and urbanization has increased construction. However, the construction of practical projects such as pavements on problematic soils is a great challenge for geotechnical engineers, which increases the thickness and continuous deformation of the pavement and disrupts the provision of traffic services (Sudhakaran, Sharma, and Kolathayar Citation2018; Tran, Satomi, and Takahashi Citation2018).
Therefore, in case of pavements built on cohesive less soils, it is very important to improve the bearing capacity of the soil (Hima Bindu and Mallikarjuna Citation2022). It is necessary to pay attention to soil type, project type, and the available budget when choosing soil improvement methods (Gautam et al. Citation2018). Recently, the use of various waste materials for soil improvement has become a common practice around the world. Fiber-reinforced soil is one of these methods that has multiple applications in retaining structures, road pavements, and slopes that increase the bearing capacity (Sujatha et al. Citation2020; Zhou et al. Citation2020; Sujatha et al. Citation2020; Wang et al. Citation2017; Zare et al. Citation2020).
BG fiber is a waste product that is extracted from sugarcane stalk and usually burned, and its use is important to prevent global warming alternately, BS natural fiber, which is extracted from BS volcanic rocks and has a very high resistance to corrosion and heat. BS fibers are cheaper than carbon and glass fibers and can be a good substitute for these fibers (Ralegaonkar et al. Citation2018; Sarasini, Tirillò, and Seghini Citation2018; Xiao et al. Citation2019; Wang et al. Citation2020; Li et al. Citation2020). PES fibers can be used as a synthetic fiber with unique properties in soil improvement (Nezhad, Tabarsa, and Latifi Citation2021; Xu et al. Citation2021).
Therefore, numerous experiments have been conducted to find the effect of the presence of fibers in the soil. Results showed that natural fibers of waste wheat straw could be used as reinforcements within the subgrade of rural roads (Meena et al. Citation2021). Basalt fibers have improved the CBR value at FC of 0.4% and FL 50 mm, due to the three-dimensional bond created between the soil and the fibers (Ayothiraman, Sahu, and Bhuyan Citation2021; Ramesh, Rao, and Kumar Citation2019; Shukla, Mittal, and Kumar Citation2021). The presence of BG fiber has also increased the tensile strength of the soils (Devadiga, Bhat, and Mahesha Citation2020). The positive effects of BS fibers were investigated and increased in improving and increasing soil tensile strength (Abbaspour et al. Citation2020; Liu et al. Citation2020: Yao et al. Citation2016). The addition of PES fibers increased the CBR from 3.2% to 6.7% (Gupta, Sood, and Gupta Citation2021; Mishra and Gupta Citation2018). The improvement of soil properties due to the presence of jute and coir fibers was investigated using extensive laboratory and field research as well as case studies, and as a result, it was found that cracking and other major damages that occur in the pavement have been reduced (Kumar, Kandasami, and Singh Citation2022). In general, two parameters of the CBR and (resilient modulus) are significant in estimating the subgrade soil strength and pavement design. Studies have shown relationships between these two parameters (El-Ashwah et al. Citation2020), But studies show that there is no relationship between
and ITS. It should be noted that, Reinforcement of pavement layers with fibers and also finding the relationship between tensile strength and resilient modulus (
) (as the main factors in the design of road layers) is essential for the optimal design of road pavements, is one of the main objectives of this article. In this study, a series of CBR and ITS tests were carried out to discover the impact of natural fibers including BS, BG, and PES fiber at different contents and lengths (the FCs = 0.5, 1, and 2% and the FLs = 2.5, 5, and 7.5 mm). Analysis of variance, multiple regression analysis, and sensitivity analysis were used to make the test results significant, to find the relationship between CBR and ITS and also to find the effect of variables (FC, FL) on ITS and CBR, respectively.
Materials and methods
Material
The sandy soil used is obtained from the Galugah city, located in the north of Iran, which is known as poorly graded sand soil (SP) according to the unified soil classification system (USCS). and show the grain size distribution curve and the geotechnical properties of sandy soils. The fibers used and their engineering properties, shown in and , respectively.
Figure 2. Used fibers: (a) Polyester (was obtained from the Sorin Polymer Khazar company in Kordkuy city, Golestan Province); (b) Bagasse (was obtained from the Haft Tappeh company in Khuzestan, Iran); (c) Basalt (was obtained from the Rioniz Vira Pars company located in Tehran, Iran).
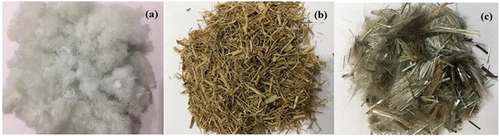
Table 1. Geotechnical properties of the used soil in the current study.
Table 2. Engineering properties of used fibers.
Specimen preparation
In this research, the soil was completely oven-dried at a temperature of 105℃ for 24 h. BS and BG natural fibers and the PES fiber are cut in 2.5, 5, and 7.5 mm of length. The fibers are mixed with sandy soil in proportions of 0.5, 1, and 2% of the dry weight of the soil, and the CBR and ITS values of the unreinforced and reinforced samples are obtained based on the corresponding experiments.
California bearing ratio and indirect tensile strength tests
The CBR tester consists of a grid plate, a tripod, and a hydraulic jack that exerts the same force of 44.5 kN by a loading rate of 1.27 mm/min to the soil sample. According to the ASTM D4254–16 standard, sandy soil samples in relative density of 50%, in the cylindrical mold with an inner diameter = 152.4 mm, height = 177.8 mm, using a 24.4 N hammer with a fall height = 305 mm, compacted in three layers with 56 blows (ASTM D1883 Citation2016). The compacted soil specimen in the mold was tested. The load-penetration curve was plotted by applying a load. Finally, the CBR was obtained by dividing the load obtained based on the load-penetration curve for 2.5 and 5 mm penetrations to the standard load (Ghasemi Nezhad, Tabarsa, and Latifi Citation2021).
The ITS experiment is a common method for calculating the tensile strength and has unique features, such as low cost, repeatability, and simplicity. Sandy soil samples were obtained at optimum humidity and a relative density of 50% and were pounded in three equal layers in a particular mold for this experiment with a height of 100 mm and a diameter of 50 mm. and show the ITS test and the experimental program (ASTM D4254–16). The ITS of sandy soil samples is obtained from the following equation (ASTM D 3967 Citation2016 ASTM C496/C496M Citation2017):
Figure 3. Indirect tensile strength test: (a) unconfined compressive strength test machine (b) unreinforced soil specimen failure (c) fiber-reinforced soil specimen failure.
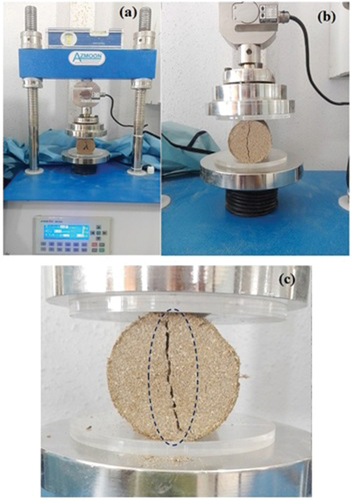
Table 3. Experimental program.
where = ITS; P = the maximum force applied to the soil specimen, d and l are the diameter and length of the cylindrical samples, respectively.
Results and discussion
California bearing ratio value
Based on the obtained results, the CBR value for the used soil in this study is equal to 3.84%. The length of 7.5 mm for PES fibers gains the highest CBR value, and the value of CBR has increased 3.3 times, which indicates the efficient performance of these fibers in increasing the strength of sandy soils. Due to its unique basic physical and mechanical properties, PES fibers are particularly resistant to mechanical effects and exposure to heat and moisture. As a result, it increases flexibility and reduces the deformation of the material under mechanical stresses. This is probably due to the reinforcing activity of these fibers (PES), and the increase in friction between the fibers and soil particles. This result is usually comparable to what has been suggested by other researchers. Adding rubber particles to the soil by 5% increases the CBR in subsequent years (Yadav and Tiwari Citation2017). The inclusion of fibers in soil samples increases the interface of fibers and soils and ultimately changes the soil and fiber structure into a cohesive matrix with high resistance and ductility. Researchers found that the expansion of synthetic fibers extended vital factors such as CBR, ITS, and UCS (Rabab’ah et al. Citation2021; Syed, GuhaRay, and Goel Citation2022). Also, the highest CBR value for BG fibers was gotten within the FC of 2%. The results are in line with the previous studies. Reinforced sand with natural fibers was evaluated. The test results showed that sand mixed with 2% of fibers has a higher bearing capacity compared to other sand compounds mixed with other percentages (Jairaj and Prathap Kumar Citation2021). Among the two natural fibers of BS and BG and PES fiber, the highest CBR value is obtained by adding PES fibers at a length of 7.5 mm and an FC of 2%. At this FC and length, the CBR of the PES fiber is higher than that of BS fibers, and the CBR of basalt fibers is higher than BG fibers. The highest CBR value was obtained for BS, BG, and PES fibers at the FL of 7.5 mm and the FC of 2%, equal to 10.13, 8.94, and 12.64, respectively. Studies have been conducted to evaluate the presence of synthetic and natural fibers in the soil, and the results of these studies show that the impact of synthetic fibers on CBR is more remarkable than natural fibers (1.5 to 3 times more) (Rabab’ah et al. 2020; Ramkrishnan et al. Citation2018).
Indirect tensile strength value
The fibers used in the present study have a rough surface and can quickly increase friction with soil particles. Therefore, the tensile strength of the fibers is increased and they can withstand more tensile strength, which is the same as the results of previous research (Consoli, Festugato, and Heineck Citation2009). Applying a load causes tensile cracks to the soil sample. After failure, the crack opening increases, but the fibers alone withstand tensile stresses. They are gradually mobilized and bridged along the cracking surfaces to prevent the cracks from spreading (Ghadakpour, Choobbasti, and Kutanaei ; Lawer, Ampadu, and Owusu-Nimo Citation2021); then, based on the results, for the three fibers used in this study, it can be stated that by expanding the length of the fibers, the tensile strength value increases. appears the stress–strain curve due to the expansion of fibers to sandy soil specimens. The peak value of the curve of stress–strain for the reinforced samples is the same as the value of ITS. By adding the BG fiber to the sandy soil specimens, the best tensile strength will be linked with the FC of 2% and the length of 7.5 mm. Through expanding the BG FC, the value of tensile strength increment (Syed, GuhaRay, and Goel Citation2022). The unreinforced soil specimens have somewhat of a brittle behavior whose flexibility increases through the addition of fibers. According to , the tensile strength of a sample of unreinforced soil is 6.24 kPa. By adding fibers at the FC of 2% and the FL of 7.5 mm, the ITS increases almost 2.5 times by 14 kPa. According to the results, the best tensile performance among the three studied fibers is related to the BS fibers, which have almost tripled the tensile strength of the soil sample in the maximum length and FC. Studies show that as the fiber content increments, the tensile strength of soil increases (Nuruzzaman et al. Citation2020). In general, the expansion of fibers increments the value of peak stress, and as a result, the soil sample bears more load, and the soil strength increases (Anggraini et al. Citation2015). One of the main reasons for increasing the tensile strength of sandy soil due to the addition of fibers is that the fibers and soil particles form an interconnected matrix, which this integrity is improved by increasing the content of fibers. The improvement in tensile strength of the samples is also evident in the test results. As expected, the tensile stress increases with increasing strain. Tensile stress is then reduced (). Previous studies indicate that the most essential components controlling the adsorption of reinforcements are bond strength and friction between fibers and soil particles. Unreinforced specimens immediately after failure are not bearing capacity and are brittle. However, residual tensile stress is caused by the presence of fibers in the sandy soil samples. In other words, the presence of fibers reduces post-peak failure and enhances the tensile ductility of soil samples.
Previous studies have also shown that fiber engagement can reduce soil tensile fragility as well as improve soil compaction and shear ductility behavior (Choobbasti, Kutanaei, and Ghadakpour Citation2019). Chebbi, Guiras, and Jamei (Citation2020) investigated the effect of a wide range of natural and synthetic fibers on the tensile strength of soil and concluded that natural fibers prevent the expansion of tensile cracks due to tensile stresses compared to synthetic fibers. Therefore, soils reinforced with natural fibers have higher tensile strength than soils reinforced with synthetic fibers.
Scanning electron microscope (SEM) analysis
Scanning electron microscope (SEM) experiments have been used to supplement the experimental results and better interpret the observed sandy soil behaviors. As demonstrated in ) (unreinforced sandy soil), sandy soil often consists of rounded particles; they slide when a load is imposed on them. Moreover, voids in unreinforced sandy soil resulted in decreased soil strength, but fibers in soil increased the friction and formed an integrated system. The SEM analysis was also performed on fiber-reinforced specimens (FL = 7.5 mm and FC = 2%). ) expresses BG fiber and the soil matrix. As it is apparent, the surface of BG fibers contains a roughness that increments the friction between the soil and the fiber and the strength of the specimen, which is reliable with ITS and CBR tests. ) shows the BS fibers added to the soil. The contact of fibers with fibers and soil particles leads to increased flexibility, compressibility, and shrinkage of the soil and fiber mixture (Shenal Jayawardane et al. Citation2020). As shown in ), the interaction between fiber and soil is obvious, the fibers are well distributed throughout the soil, and a successful bonding between soil particles and fibers has been observed, resulting in the strength of the sandy soil sample reflected in test results. In addition, it should be noted that the roughness of the fiber surface and the effective contact surface with soil particles also affect the interactions of fibers and soil (Cui et al. Citation2018). Owing to the high resistance of reinforced soil to compaction, fiber-reinforced sand can show a higher void ratio (less porosity) than unreinforced soil (Choo et al. Citation2017). Another significant result is that by reducing the void ratio of soil samples, the ITS and CBR increase. This is similar to the results of the following studies: Trabelsi, Romero, and Jamei (Citation2018) found that they are inversely related to the void ratio and ITS. As it is known, the values of the porosity ratio to the dry unit weight of soil samples according to soil mechanical relationships are inversely related to each other. Gansonré et al. (Citation2021) found that as dry unit weight increased, the CBR increased and the void ratio decreased. Studies have shown that reinforced specimens withstand more tensile stress and a series of residual stresses appear after failure in the specimen, which is due to the presence of fibers as a bridge that withstands the tensile loads (Liu et al. Citation2020).
Statistical analysis
In this section, using analysis of variance (ANOVA), it is checked that the discrepancy between the values (CBR and FL, ITS and FL, CBR and FC, ITS and FC), in terms of is it statistically significant or not? If the contrast between the values is significant, there is a relationship between the two parameters, and by increasing one parameter, the other parameter increases. The significance of the difference between the values is based on three basic parameters, which are t-distribution, Fisher distribution, and P-value. To perform ANOVA, we first need to test the hypotheses using an appropriate regression model (EquationEquation 2(2)
(2) ). A suitable model with regression analysis at 95% confidence surface has been performed to anticipate the subordinate parameters. If the P-value is under 0.05, the disagreement between the values is considerable (Raavi and Tripura Citation2020; Stumpf et al. Citation2018). All investigations were performed by utilizing R statistical software version 3.3.2. Based on the following regression model, analysis of variance was performed:
According to EquationEquation 2(2)
(2) and the desired parameters, EquationEquations (3
(3)
(3) -Equation6
(6)
(6) ) are obtained, which are shown in , that Y is CBR or ITS, X is (
) and (
),
is the regression coefficients, e is the error. The ANOVA can be seen in .
Table 4. Equations obtained based on EquationEquation 2(2)
(2) .
Table 5. The results of ANOVA.
In the following, the parameters mentioned in the ANOVA table are introduced (MSR = mean of squares regression; DF = degree of freedom; MS = mean of squares; SS = sum of squares SSE = sum of squares error; SSR = sum of squares regression; SSR = sum of squares regression; MSE = mean of squares error; F = Fisher distribution). The SSR and SSE values for each of the variables are considered according to the results obtained from the statistical software. It should be noted that (,
and F=
). For the contrast between the values to be noteworthy, the value of F from must be more noteworthy than the value of F obtained from the statistical tables (). The value of F
, according to , is obtained based on three factors (
) which is
equal to 4.23, which is less than the values obtained from (results of statistical software R), so it is significant. In of values
to
(according to the results of software R), the opposite is zero and also the (P-value) values for all parameters ((L and CBR), (W and CBR), (L and ITS), (W and ITS)) are less than 0.05. It is important to note that, for the contrast between the values to be significant, the t-value must be larger than the values in the statistical tables (). The value of
, according to , is obtained based on two factors
which is
equal to 2.056, which is less than the values obtained from (results of statistical software R), so it is significant. pr(>|t|) is the probability value of the coefficients, which shows that the values obtained are very appropriate. Similar studies have been performed to find significance between parameters through ANOVA. Raavi and Tripura (Citation2020) obtained the relationship between tensile and compressive strength parameters (coir length, coir percentage, cement content, and density) using regression analysis. The results showed that the p-value for tensile strength and all variables is less than 0.05 (Raavi and Tripura Citation2020).
Table 6. Fisher distribution (Montgomery et al. Citation2021).
Table 7. T-distribution (Montgomery et al. Citation2021).
Correlation between resilient modulus and indirect tensile strength
Creating a relationship between Mr and ITS parameters is effective in the optimal design of roads. There are several methods for designing pavement layers, such as CBR and AASHTO methods, which are very common today. The Mr is one of the main parameters in the design of AASHTO. Some regulations recommend that the be used as a fundamental property in the analysis and the structural design of pavement layers (Mogili et al. Citation2020). In this study, a relationship between sandy soil ITS and CBR is presented. Studies show that there is an empirical relationship between the Mr and the CBR factor. According to AASHTO method, with the expansion of Mr, the structural number of pavement (SN), as well as the thickness of pavement layers, will be reduced and design (based on AASHTO method) will be engineering and economically viable. It is known that CBR can play a helpful role in determining the strength of pavement layers. Various studies have shown that there are relationships between ITS and the unconfined compressive strength (UCS), as well as the UCS and CBR. Therefore, it can provide a relationship between ITS and CBR. According to the results of the CBR and ITS tests, a relationship can be presented between the two parameters, as shown in . As it is evident, ITS and CBR are correlated with a good correlation coefficient (R = 0.8037).
Since the pavement is affected by the continuous passage of vehicles, evaluating the soil strength should be conducted through tests in which the soil specimens are affected by loading and unloading. To determine the parameter, a device similar to the triaxial compression test can be used. However, since the
test is complex, costly, and time-consuming, the
dependence on the CBR and the proposed standard relationships can be used.
for the substrate soil with respect to the CBR value is obtained using the following experimental equations (Peter, Jayasree, and Balan Citation2020):
Using EquationEquation 4(4)
(4) and EquationEquation 5
(5)
(5) , as well as the results of the CBR test of sandy soil samples, the value of
was obtained, as shown in .
Table 8. The obtained Mr values for unreinforced and reinforced specimens.
Due to the major role of fibers in expanding the soil tensile strength in the pavement layer design and based on previous studies about lack of relationship between and ITS along with the experimental results, it is vital to determine the relationship between the
and ITS for practical projects. Based on , the equation with a correlation coefficient (R) of 0.8405 was obtained. the results of all fibers were considered in this figure. In , by dividing the results into the two types of natural (BG and BS) and (PES) fibers, an increase in the correlation coefficient was observed. Consequently, the relationship between
and ITS was presented separately for all the three fibers of BS, BG, and PES, which showed a significant increase in the correlation coefficient, as indicated in . The correlation coefficient (R) values for BG, PES, and BS fibers were 0.9562, 0.9763, and 0.9722, respectively. As a result, the fiber type is an influential factor in establishing the relationship between ITS and
.
Figure 8. Relationship between resilient modulus and indirect tensile strength: (a) Synthetic fibers (b) Natural fibers.
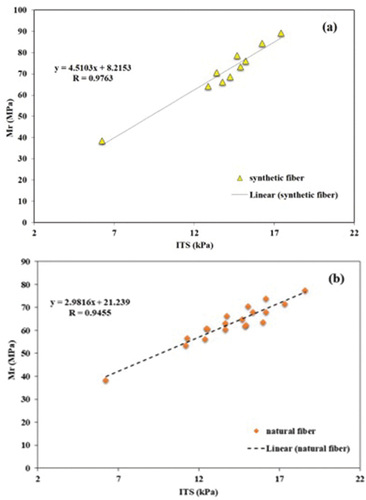
EquationEquations (6(6)
(6) -Equation8
(8)
(8) ) are the relationships between the
and ITS for BG, PES, and BS fibers, respectively.
Multiple regression and sensitivity analysis
This section aims to find a relationship between CBR and ITS with variables of fiber length and fiber content, using an analysis of multiple regression. Multiple regression analysis is used to obtain a good relationship between factors (fiber length and fiber content) with ITS and CBR values. Furthermore, based on the sensitivity analysis, it is examined how the output values of the model are affected by changes in the input amounts. The model of multiple regression is supposed as Eqs. (9) and (10) that is plain soil CBR value,
is plain soil ITS value and
-
is multiple regression coefficients (Sadrossadat, Heidaripanah, and Osouli Citation2016; Tran, Satomi, and Takahashi Citation2018).
Multiple regression variables are specified in Eqs. (11) and (12). The correlation coefficients (R) for these two equations held the values of 0.83 and 0.97, respectively.
Finally, in this research, sensitivity analysis was performed using equations (13) and (14) and considering equations (11) and (12) that ) is maximum outputs,
is minimum outputs, (n) is The number of input variables, (
is The domain of input variables,
is The effective degree of the input variables (Gandomi, Yun, and Alavi Citation2013).
According to Eqs. EquationEquation 14(14)
(14) and EquationEquation 14
(14)
(14) , when considering the mean values of the other factors,
and
are the most and least outputs for the required variable, respectively (Gandomi, Yun, and Alavi Citation2013). The effect of fiber length and fiber content parameters on CBR and ITS, based on the concepts of sensitivity analysis, was investigated. According to the study, fiber content (FC) and fiber length (FL) had effective degrees of 71.65% and 28.35% on CBR values, and 73.38% and 26.62% on ITS values, respectively. The results of the sensitivity analysis are shown in . The fiber content had previously shown to be more influential on the tensile strength rather than the FL.
Table 9. The results of the sensitivity analysis.
Conclusions
In this study, the effects of adding three materials (natural fibers of basalt, bagasse, and polyester synthetic fibers) on the sandy soils mechanical and geotechnical behavior under California bearing ratio and indirect tensile strength tests have been investigated. The results of the above tests are as follows:
The California bearing ratio test was performed on a series of unreinforced and reinforced specimens. It was found that the inclusion of fibers in the soil has a significant effect on the amount of California bearing ratio. Samples reinforced with polyester synthetic fibers have the highest amount of California bearing ratio. In addition, this result is achieved in the fiber length = 7.5 mm and fiber content = 2%.
Tensile strength is one of the main factors influencing the design of pavement layers. Specimens reinforced with basalt fibers showed acceptable tensile strength compared to other fibers. Basalt fibers have increased the tensile strength by about 3 times.
Analysis of variance (ANOVA) was performed using R statistical software. According to ANOVA results, the contrast between the values of fiber length and the California bearing ratio, fiber content and the California bearing ratio, fiber length and indirect tensile strength, fiber content, and indirect tensile strength is significant.
Using multiple regression analysis, a relationship is obtained between the variables (fiber length and fiber content) and each of the values of California bearing ratio and indirect tensile strength with correlation coefficients of 0.83 and 0.97, respectively.
The effect of fiber length and fiber content parameters on California bearing ratio and indirect tensile strength, based on the concepts of sensitivity analysis, was investigated. According to the study, fiber content (FC) and fiber length (FL) had effective degrees of 71.65% and 28.35% on CBR values, and 73.38% and 26.62% on ITS values, respectively.
According to the results of tests on sandy soil samples, a significant relationship between indirect tensile strength – California bearing ratio and indirect tensile strength-resilient modulus parameters with a suitable correlation coefficient was obtained.
The relationship between resilient modulus and indirect tensile strength was presented separately for all the three fibers of Basalt, Bagasse, and Polyester. The correlation coefficient (R) values for Bagasse, Polyester, and Basalt fibers were 0.9562, 0.9763, and 0.9722, respectively. As a result, the fiber type is an influential factor in establishing the relationship between indirect tensile strength and resilient modulus.
Acknowledgements
The authors would like to extend their gratitude to authorities of the soil mechanics laboratory of Golestan University.
Disclosure statement
No potential conflict of interest was reported by the authors.
References
- Abbaspour, M., S. Sheikhi Narani, E. Aflaki, and F. Moghadas Nejad. 2020. Behavior of a subgrade soil reinforced by waste tire textile fibers under static and cyclic loading. Journal of Materials in Civil Engineering 32 (8):04020208. doi:10.1061/(ASCE)MT.1943-5533.0003279.
- Anggraini, V., A. Asadi, B. B. K. Huat, and H. Nahazanan. 2015. Effects of coir fibers on tensile and compressive strength of lime treated soft soil. Measurement 59:372–18. doi:10.1016/j.measurement.2014.09.059.
- ASTM C496/C496M, Standard test method for splitting tensile strength of cylindrical concrete specimens, ASTM International, West Conshohocken, PA, 2017, www.astm.org
- ASTM D1883, Standard test method for California bearing ratio (CBR) of laboratory-compacted soils, ASTM International, West Conshohocken, PA, 2016, www.astm.org.
- ASTM D3967, Standard test method for splitting tensile strength of intact rock core specimens, ASTM International, West Conshohocken, PA, 2016, www.astm.org.
- Ayothiraman, R., R. Sahu, and P. Bhuyan. 2021. Strength and deformation behavior of fine-grained soils reinforced with hair fibers and its application in pavement design. Journal of Natural Fibers 19:1–18.. doi:10.1080/15440478.2021.1954129.
- Chebbi, M., H. Guiras, and M. Jamei. 2020. Tensile behaviour analysis of compacted clayey soil reinforced with natural and synthetic fibers: Effect of initial compaction conditions. European Journal of Environmental and Civil Engineering 24 (3):354–80.. doi:10.1080/19648189.2017.1384762.
- Choobbasti, A. J., S. S. Kutanaei, and M. Ghadakpour. 2019. Shear behavior of fiber-reinforced sand composite. Arabian Journal of Geosciences 12 (5):1–6.. doi:10.1007/s12517-019-4326-z.
- Choo, H., B. Yoon, W. Lee, and C. Lee. 2017. Evaluation of compressibility and small strain stiffness characteristics of sand reinforced with discrete synthetic fibers. Geotextiles and Geomembranes 45 (4):331–8.. doi:10.1016/j.geotexmem.2017.04.005.
- Consoli, N. C., L. Festugato, and K. S. Heineck. 2009. Strain-hardening behavior of fibre- reinforced sand in view of filament geometry. Geosynthetics International 16 (2):109–15. doi:10.1680/gein.2009.16.2.109.
- Cui, H., Z. Jin, X. Bao, W. Tang, and B. Dong. 2018. Effect of carbon fiber and nanosilica on shear properties of silty soil and the mechanisms. Construction and Building Materials 189:286–95.. doi:10.1016/j.conbuildmat.2018.08.181.
- Devadiga, D. G., K. S. Bhat, and G. T. Mahesha. 2020. Sugarcane bagasse fiber reinforced composites: Recent advances and applications. Cogent Engineering 7 (1):1823159.. doi:10.1080/23311916.2020.1823159.
- El-Ashwah, A. S., E. Mousa, S. M. El-Badawy, and M. A. Abo-Hashema. 2020. Advanced characterization of unbound granular materials for pavement structural design in Egypt. International Journal of Pavement Engineering 23 (2):476–88.. doi:10.1080/10298436.2020.1754416.
- Gandomi, A. H., G. J. Yun, and A. H. Alavi. 2013. An evolutionary approach for modeling of shear strength of RC deep beams. Materials and Structures 46 (12):2109–19. doi:10.1617/s11527-013-0039-z.
- Gansonré, Y., P. Breul, C. Bacconnet, M. Benz, and R. Gourvès. 2021. Estimation of lateritic soils elastic modulus from CBR index for pavement engineering in Burkina Faso. International Journal of Pavement Research and Technology 14 (3):348–56.. doi:10.1007/s42947-020-0118-9.
- Gautam, P. K., P. Kalla, A. S. Jethoo, R. Agrawal, and H. Singh. 2018. Sustainable use of waste in flexible pavement: A review. Construction and Building Materials 180:239–53.. doi:10.1016/j.conbuildmat.2018.04.067.
- Ghadakpour, M., A. J. Choobbasti, and S. S. Kutanaei. 2020. Investigation of Kenaf fiber hybrid length on the properties of the cement-treated sandy soil. Transportation Geotechnics 22:100301. doi:10.1016/j.trgeo.2019.100301.
- Gupta, G., H. Sood, and P. K. Gupta. 2021. Performance review of polyester fibre reinforced soil for sustainable construction. Sustainable Development Through Engineering Innovations 113:223–34.
- Hima Bindu, G., and V. Mallikarjuna. 2022. Improving the geotechnical properties of silty sands by using GGBS and coir fibre. In Recent Advances in Civil Engineering, eds. L. Nandagiri, M. C. Narasimhan, and M. Shriram, 277–88. Singapore: Springer.
- Jairaj, C., and M. T. Prathap Kumar. 2021. Strength behavior of sand reinforced with treated sisal fibers. Ground Improvement Techniques 118: 23–32.
- Kumar, N., R. K. Kandasami, and S. Singh. 2022. Effective utilization of natural fibres (coir and jute) for sustainable low-volume rural road construction–a critical review. Construction and Building Materials 347:128606.. doi:10.1016/j.conbuildmat.2022.128606.
- Lawer, A. K., S. I. K. Ampadu, and F. Owusu-Nimo. 2021. The effect of randomly distributed natural fibers on some geotechnical characteristics of a lateritic soil. SN Applied Sciences 3 (6):1–12.. doi:10.1007/s42452-021-04634-0.
- Liu, J., Y. Bai, Z. Song, D. P. Kanungo, Y. Wang, F. Bu, Z. Chen, and X. Shi. 2020. Stabilization of sand using different types of short fibers and organic polymer. Construction and Building Materials 253:119164.. doi:10.1016/j.conbuildmat.2020.119164.
- Li, Z., X. Zhang, C. Fa, Y. Zhang, J. Xiong, and H. Chen. 2020. Investigation on characteristics and properties of bagasse fibers: Performances of asphalt mixtures with bagasse fibers. Construction and Building Materials 248:118648. doi:10.1016/j.conbuildmat.2020.118648.
- Meena, S. K., R. Sahu, and R. Ayothiraman. 2021. Utilization of waste wheat straw fibers for improving the strength characteristics of clay. Journal of Natural Fibers 18 (10):1404–18. doi:10.1080/15440478.2019.1691116.
- Mishra, B., and M. K. Gupta. 2018. Use of randomly oriented polyethylene terephthalate (PET) fiber in combination with fly ash in subgrade of flexible pavement. Construction and Building Materials 190:95–107.. doi:10.1016/j.conbuildmat.2018.09.074.
- Mogili, S., H. Mudavath, K. K. Gonavaram, and Y. Paluri. 2020. Strength and resilient behavior of lime modified pond ash as pavement layer. MaterialsToday: Proceedings United Kingdom, 32: 567–73.
- Montgomery, D. C., E. A. Peck, and G. G. Vining. 2021. Introduction to linear regression analysis. USA: John Wiley & Sons.
- Nezhad, M. G., A. R. Tabarsa, and N. Latifi. 2021. Effect of natural and synthetic fibers reinforcement on California bearing ratio and tensile strength of clay. Journal of Rock Mechanics and Geotechnical Engineering 13 (3):626–42. doi:10.1016/j.jrmge.2021.01.004.
- Nuruzzaman, D. M., N. M. Kusaseh, N. M. Ismail, A. K. M. A. Iqbal, M. M. Rahman, A. Azhari, Z. Hamedon, and C. S. Yi. 2020. Influence of glass fiber content on tensile properties of polyamide-polypropylene based polymer blend composites. MaterialsToday: Proceedings United Kingdom, 29: 133–37.
- Peter, L., P. K. Jayasree, and K. Balan. 2020. Optimization, quantification of strength and design benefits of flexible pavements resting on soft soil subgrades treated with coir waste and lime. Journal of Natural Fibers 19:1–14. doi:10.1080/15440478.2020.1788490.
- Raavi, S. S. D., and D. D. Tripura. 2020. Predicting and evaluating the engineering properties of unstabilized and cement stabilized fibre reinforced rammed earth blocks. Construction and Building Materials 262:120845.. doi:10.1016/j.conbuildmat.2020.120845.
- Rabab’ah, S., O. Al Hattamleh, H. Aldeeky, and B. A. Alfoul. 2021. Effect of glass fiber on the properties of expansive soil and its utilization as subgrade reinforcement in pavement applications. Case Studies in Construction Materials 14:e00485. doi:10.1016/j.cscm.2020.e00485.
- Ralegaonkar, R., H. Gavali, P. Aswath, and S. Abolmaali. 2018. Application of chopped basalt fibers in reinforced mortar: A review. Construction and Building Materials 164:589–602.. doi:10.1016/j.conbuildmat.2017.12.245.
- Ramesh, A., C. N. Rao, and M. Kumar. 2019. Experimental study on geocell and of fibre reinforced soil sub-grade under static and repetitive load. Geotechnics for Transportation Infrastructure 2:139–49.
- Ramkrishnan, R., M. R. Sruthy, A. Sharma, and V. Karthik. 2018. Effect of random inclusion of sisal fibres on strength behavior and slope stability of fine grained soils. Materials Today: Proceedings United Kingdom, 5(11): 25313–22.
- Sadrossadat, E., A. Heidaripanah, and S. Osouli. 2016. Prediction of the resilient modulus of flexible pavement subgrade soils using adaptive neuro-fuzzy inference systems. Construction and Building Materials 123:235–47. doi:10.1016/j.conbuildmat.2016.07.008.
- Sarasini, F., J. Tirillò, and M. C. Seghini. 2018. Influence of thermal conditioning on tensile behaviour of single basalt fibres. Composites Part B: Engineering 132:77–86.. doi:10.1016/j.compositesb.2017.08.014.
- Shenal Jayawardane, V., V. Anggraini, E. Emmanuel, L. L. Yong, and M. Mirzababaei. 2020. Expansive and compressibility behavior of lime stabilized fiber- reinforced marine clay. Journal of Materials in Civil Engineering 32 (11):04020328. doi:10.1061/(ASCE)MT.1943-5533.0003430.
- Shukla, S., A. Mittal, and S. Kumar. 2021. Improvement in soil subgrade using natural fibre (kenaf and coir fibre). Proceedings of the Indian Geotechnical Conference India 2019, 211–18.
- Stumpf, L., O. D. Anjos Leal, E. A. Pauletto, L. F. S. Pinto, D. A. Reis, M. A. B. Pinto, and I. K. Tuchtenhagen. 2018. Tensile strength and organic matter fractions in aggregates of a grass-covered mined soil under early stage recovery. Soil and Tillage Research 176:69–76.. doi:10.1016/j.still.2017.11.006.
- Sudhakaran, S. P., A. K. Sharma, and S. Kolathayar. 2018. Soil stabilization using bottom ash and areca fiber: Experimental investigation and reliability analysis. Journal of Materials in Civil Engineering 30 (8):04018169. doi:10.1061/(ASCE)MT.1943-5533.0002326.
- Sujatha, E. R., P. Atchaya, S. Darshan, and S. Subhashini. 2020. Mechanical properties of glass fibre reinforced soil and its application as subgrade reinforcement. Road Materials and Pavement Design 22 (10):2384–95. doi:10.1080/14680629.2020.1746387.
- Syed, M., A. GuhaRay, and D. Goel. 2022. Strength characterisation of fiber reinforced expansive subgrade soil stabilized with alkali activated binder. Road Materials and Pavement Design 23(5): 1037–1060.
- Trabelsi, H., E. Romero, and M. Jamei. 2018. Tensile strength during drying of remoulded and compacted clay: The role of fabric and water retention. Applied Clay Science 162:57–68.. doi:10.1016/j.clay.2018.05.032.
- Tran, K. Q., T. Satomi, and H. Takahashi. 2018. Improvement of mechanical behavior of cemented soil reinforced with waste cornsilk fibers. Construction and Building Materials 178:204–10. doi:10.1016/j.conbuildmat.2018.05.104.
- Wang, Y. X., P. P. Guo, W. X. Ren, B. X. Yuan, H. P. Yuan, Y. L. Zhao, P. Cao, and P. Cao. 2017. Laboratory investigation on strength characteristics of expansive soil treated with jute fiber reinforcement. International Journal of Geomechanics 17 (11):04017101. doi:10.1061/(ASCE)GM.1943-5622.0000998.
- Wang, D., H. Wang, S. Larsson, M. Benzerzour, W. Maherzi, and M. Amar. 2020. Effect of basalt fiber inclusion on the mechanical properties and microstructure of cement-solidified kaolinite. Construction and Building Materials 241:118085.. doi:10.1016/j.conbuildmat.2020.118085.
- Xiao, Y., X. He, T. M. Evans, A. W. Stuedlein, and H. Liu. 2019. Unconfined compressive and splitting tensile strength of basalt fiber-reinforced biocemented sand. Journal of Geotechnical and Geoenvironmental Engineering 145 (9):04019048. doi:10.1061/(ASCE)GT.1943-5606.0002108.
- Xu, M., S. Song, L. Feng, J. Zhou, H. Li, and V. C. Li. 2021. Development of basalt fiber engineered cementitious composites and its mechanical properties. Construction and Building Materials 266:121173. doi:10.1016/j.conbuildmat.2020.121173.
- Yadav, J. S., and S. K. Tiwari. 2017. A study on the potential utilization of crumb rubber in cement treated soft clay. Journal of Building Engineerin 9:177–91. doi:10.1016/j.jobe.2017.01.001.
- Yao, Y., D. Zhu, H. Zhang, G. Li, and B. Mobasher. 2016. Tensile behaviors of basalt, carbon, glass, and aramid fabrics under various strain rates. Journal of Materials in Civil Engineering 28 (9):04016081.
- Zare, P., S. S. Narani, M. Abbaspour, A. Fahimifar, S. M. M. M. Hosseini, and P. Zare. 2020. Experimental investigation of non-stabilized and cement-stabilized rammed earth reinforcement by Waste Tire Textile Fibers (WTTFs). Construction and Building Materials 260:120432.. doi:10.1016/j.conbuildmat.2020.120432.
- Zhou, C., L. Cai, Z. Chen, and J. Li. 2020. Effect of kenaf fiber on mechanical properties of high-strength cement composites. Construction and Building Materials 263:121007.. doi:10.1016/j.conbuildmat.2020.121007.