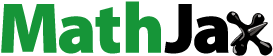
ABSTRACT
Commercial hair perming uses strong reducing agents and harms hair fiber’s quality even human health. In this study, tyrosine is adopted as a cross-linking agent between thiols and/or amines as the shape-changing of hair involves the breakage of disulfide bonds and the rearrangement of new bonds between keratin molecules. To investigate the mechanism of keratin cross-linking, bovine serum albumin (BSA) is used as a model protein. Disulfide bonds in BSA are successfully reduced by L-cysteine to provide free thiols. Four new cross-linked peptides are formed inter- or intra-BSA monomers, indicating that tyrosine can be adopted as a cross-linking agent not only between amines but also between thiols. Moreover, curling of Asian hair is conducted using tyrosine as a perming agent by the laccase-assisted reaction. The optimized operational conditions are hair with L-cysteine pre-treatment (50.0 mM) followed by laccase-assisted grafting with 3.0 mM tyrosine. The reshaped hair performed a better perming performance than commercial perming product before washing, although a lower perming efficiency after washing. The curling process could be accomplished without strength loss of hair fibers and with a blow-drier easily. Hence, this new methodology may lead to the development of a gentle and user-friendly approach in the hair care industry.
摘要
商业烫发中使用的强力还原剂会损害头发纤维的质量甚至人体健康,因此,该研究将酪氨酸用作巯基和/或氨基之间的交联剂,通过二硫键的断裂和角蛋白分子间新交联键的重排改变头发的形状. 为了研究角蛋白交联的机理,将牛血清白蛋白(BSA)用作模型蛋白. BSA中的二硫键成功地被L-半胱氨酸还原为巯基,后续的酪氨酸接枝使BSA单体之间或内部形成了四种新的交联肽段,表明酪氨酸不仅可以用作氨基之间的交联剂,也可以促使巯基之间的交联. 此外,以酪氨酸为烫发剂对亚洲人发进行卷曲改性. 最佳的实验条件为头发先经过50.0 mM的L-半胱氨酸预处理,然后使用漆酶催化3.0 mM的酪氨酸进行接枝. 改性后头发的卷曲效果优于传统商业烫发,尽管洗涤后头发的卷曲程度略有下降,但在卷曲改性过程中头发的强力几乎没有损失,并且该工艺可以用吹风机轻松完成. 因此,这种温和友好的卷发方法将会促进美发行业的发展.
Introduction
The hair cosmetic industry provides us with many products to match our requirements in daily life, mainly including hair dyeing, straightening, perming, and repairing (Cruz et al. Citation2016; Ito et al. Citation2017; Madnani and Khan Citation2013). Hair curling is one of the most popular choices for Asian people with straight hair. The one-time styling is usually conducted using a BaByliss at a high temperature (180°C–200°C), and most of the curls can be washed off with shampoo or even water. The traditional commercial perming process can hold the hair in a particular style for long wear, which consists of two main steps: reduction based on alkaline agents and neutralization with hydrogen peroxide (Joo et al. Citation2016). In this procedure, disulfide bonds in the hair are broken and rearranged to form a new shape of the hair. However, the chemical methods result in hair structure damage, tensile strength decline, and luster loss (Cruz et al. Citation2016; Fernandes et al. Citation2012; Lee et al. Citation2014; Miranda-vilela, Botelho, and Muehlmann Citation2014) and are harmful to human health and the environment (Galli et al. Citation2015).
The biology of hair care has drawn attention in recent years, such as dyeing (Tinoco et al. Citation2019), mechanical and thermal properties enhancement (Fernandes and Cavaco-Paulo Citation2012; Malinauskyte et al. Citation2021; Tinoco et al. Citation2019, Citation2021), and shape modeling (Basit et al. Citation2018; Cruz et al. Citation2017; Maneli, Smith, and Khumalo Citation2014; Martins, Silva, and Cavaco-Paulo Citation2019; Tinoco et al. Citation2021; Wang et al. Citation2019). Cysteine-containing peptides have the potential to cross-link with hair keratins and improve hair properties. Cruz et al. (Citation2017) selected eight cysteine-containing peptides to straighten African curly hair efficiently with Young’s modulus increasing. Martins, Silva, and Cavaco-Paulo (Citation2019) used α-chymotrypsin to catalyze KCL (Lysine-Cysteine-Leucine) ethyl ester and KSL (Lysine-Serine-Leucine) ethyl ester in situ coating on Asian hair. The KCL (containing cysteine residues) treated hair would hold the perming efficiency for around 60% after washing through the re-conformation of disulfide bonds between peptides and hair. Proteins were also adopted as new hair styling agents according to the desire. Chemically damaged curly hair was straightened and strength remediated via the Keratin K31 treatment (Basit et al. Citation2018). BSA and keratin showed the ability to promote and maintain hair curls, leading to an excellent perming efficiency close to that was measured for a commercial perming product (Tinoco et al. Citation2021).
Tyrosine, as a kind of phenolic compound, would be catalyzed by laccase to form quinone radical, which can react with thiols via Michael addition. Herein, the core of Asian hair curling is the cross-linking between thiols using tyrosine as a moiety. The main components of human hair are keratin proteins, which molecular weight ranges from 40 to 70 kDa (Moll, Divo, and Langbein Citation2008). And human hair keratins are mainly in α-helix conformation (Cruz et al. Citation2013; Deb-Choudhury, Plowman, and Harland Citation2016). Similarly, BSA is an α-helix globular protein with around 66 kDa (Majorek et al. Citation2012), so it can be used as a model to analyze the mechanism of hair reshaping. In this work, L-cysteine is chosen as a disulfide bond reducing agent to replace strong alkaline solutions for declining damage to hair and human health. The ability of tyrosine to curl the hair is evaluated by the free thiol content, FTIR, and perming efficiency (PE) of grafted Asian hair. Laccase-assisted tyrosine grafting is proposed as an alternative approach to the commercial methods used for hair perming.
Material and methods
Materials
Laccase (EC 1.10.3.2) from Myceliophthora thermophila was supplied by Novozymes, Denmark (3000 U). Bovine serum albumin Fraction V (BSA) was provided by Sigma-Aldrich and used without further purification. Virgin Asian hair was purchased from the hair factory without further treatment.
Preparation of BSA samples
BSA samples were prepared following the procedures depicted in . The experimental conditions were (i) pre-treatment with L-cysteine (10.0 mM, pH = 8.0) at 40°C for 30 min; (ii) thiols alkylating with iodoacetamide (IAM, 20 mM) at 37°C for 30 min in the dark; and (iii) grafting with tyrosine (2.2 mM, pH = 5.0) via laccase-assisted (18 U/mL) at 40°C for 6 h with a speed of 500 rpm. Non-reacted IAM and tyrosine were removed by ultrafiltration (30 kDa), and the BSA samples were kept at 4°C for further measurement.
Laccase-assisted tyrosine grafting onto Asian hair
The hair samples (280 mg) were rolled on a glass rod, covered with L-cysteine in a solution of 20% ethanol, 15% propylene glycol, and 0.5% benzyl alcohol (pH = 10.0) for 30 min at 40°C (Li et al. Citation2021), washed, and dried. Tyrosine solution containing 18 U/mL of laccase was added to the surface of L-cysteine pre-treated hair (rolled on a glass), followed by the blow-drying at a temperature from 25°C to 55°C for the grafting development; afterward, hair samples were dried at higher temperature (55°C−85°C). All hair samples were then removed from the glass rods and washed with deionized water.
Free thiols and disulfide bond determination
The content of free thiols was measured using the Ellman method (Hansen et al. Citation2007). Briefly, 100 μL of 4 mg/mL Ellman’s reagent was added into 5 mL of BSA samples (1 mg/mL) or 5 mL of phosphate buffer (pH = 8.0) with 10 mg of hair, respectively. The absorbance was detected at 412 nm, and the amount of free thiol groups was calculated from the calibration curve measured using L-cysteine. The number of disulfide bonds in BSA samples was calculated by the differences in free thiol contents present before and after sodium borohydride reduction (Fernandes and Cavaco-Paulo Citation2012).
Molecular weight of BSA samples
The molecular weight distribution of BSA samples was measured by SDS-PAGE using 12.5% separating Tris-HCl gels on mini-protein tetra electrophoresis (Bio-Rad Laboratories, Inc., Hercules, USA). The BSA samples (0.1 mg/ml) were mixed with 4× Laemmli sample buffer (containing 10% of β-mercaptoethanol) at 3:1 (v: v) and then heated at 100°C for 5 min. Afterward, 10 μL of samples and markers (10–250 kDa) was loaded onto the wells and operated at 120 v. After electrophoresis, the gel was stained with Coomassie Bright Blue R250 for 1 h and decolorized with water overnight.
The Bruker UltrafleXtreme MALDI-TOF Mass Spectrometer (Bruker AXS Co., Germany) was adopted to measure the molecular mass of BSA samples. The sample/matrix (sinapic acid) mixtures (volume ratio of 1:1) were deposited on a steel target and followed by air-drying at room temperature. Then, the dried samples were conducted in the linear and positive-ion mode.
LC–MS analysis of BSA samples
Untreated BSA, Thiols-alkylated BSA, and Tyrosine-grafted BSA were hydrolyzed by trypsin (1000 U/mL) at 37°C for 24 h followed by adding 100 μl of β-mercaptoethanol and heated in a water bath at 100°C for 10 min. Hydrolyzed samples were centrifugated at 8000 rpm for 20 min, and the obtained supernatants (containing peptides) were clarified through a 0.22 μm Whatman microfilter. All samples were confirmed by LC–MS analysis, and the collected data were analyzed using the software MassLynx V4.1. The predicted molecular masses of trypsin hydrolyzed peptides of pure BSA were provided by ExPASy resources (ExPASy Citation2005).
Molecular dynamics sImulation
Molecular Dynamics (MD) simulations were carried out for the BSA wild type (PDB ID: 3V03, chain A) (Majorek et al. Citation2012) and its designed analog presented three reduced disulfide bonds, as pointed out by experimental results. The first system was named as BSAwt, and the second as BSA-3CysSH. The modeling details of BSA systems were shown in the Supporting information. Images generated for visual inspection and data analysis were prepared using PyMOL Version 2.0.
Characterization of grafted hair
The loops and length of tyrosine-grafted hair were measured before and after washing. The perming efficiency (PE) is calculated using EquationEquation (1)(1)
(1) .
FT-IR spectra of hair samples were detected using a Thermo Nicolet iS10 spectrometer (Thermo Fisher Scientific Co., Waltham, MA, USA). Data were collected from 4000 to 600 cm−1, with a resolution of 8 cm−1 and 32 scans.
Mechanical properties of hair were evaluated by Young’s modulus and breaking strength. For each sample set, 20 single hairs were mounted in the tensile jig with a fixed gauge length of 25 cm and a constant rate of 250 mm/min until breakage on an Instron 5565A tensile equipment. All the samples were kept under the same temperature (±25 ºC) and humidity (65%) before testing.
Results and discussion
Molecular weight and reactive residues of BSA
As shown in , BSA and BSA(cys) present bands at around 66 KDa, revealing that cleavage of disulfide bonds would not influence the molecular weight of BSA. When tyrosine and laccase are mixed with BSA(cys), the proteinic band at 66 KDa is wider and darker, indicating that tyrosine may graft on BSA(cys). However, this result does not convince the success of tyrosine-grafted BSA formation, so the MALDI-TOF analysis is conducted for quantification. The laccase shows a light color band at about 80 kDa, while the laccase band is not detected in the sample Tyrosine-grafted BSA due to a low amount of laccase after dilution.
Figure 2. SDS-PAGE of marker and BSA samples: (i) laccase (18 U/mL), (ii) BSA, (iii) BSA(cys), (iv) thiols-alkylated, and(v) tyrosine-grafted BSA.
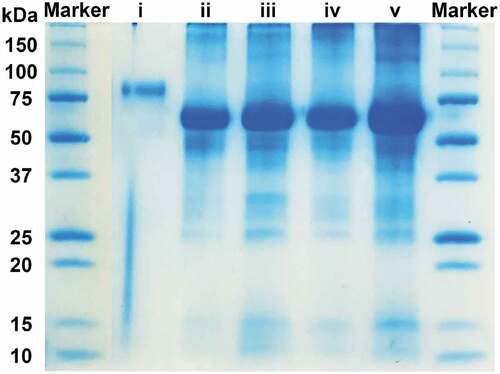
BSA monomer presents a peak at 66,516 m/z () and contains 2.40 μM/mg of free thiols and 133.23 μM/mg of disulfide bonds (), corresponding to one free thiol and 17 disulfide bonds (PDBsum Citation2012), respectively. A significant enhancement of free thiols is found in L-cysteine pre-treated BSA (32.97 μM/mg), resulting from the breakage of disulfide bonds. After IAM alkylating, free thiols are hardly detected, revealing that all free thiols in BSA(cys) are linked with -CH2CONH2 groups (Mw = 58) in IAM via C-S bonds. The molecular weight of sample thiols-alkylated BSA increases by 424 m/z, indicating that BSA(cys) contains seven free thiols caused by reduction of three disulfide bonds in BSA. Therefore, the band of sample thiols-alkylated BSA does not show significant migration to a higher molecular mass (). As shown in , about nine tyrosines are successfully grafted onto BSA(cys) via laccase-assisted with mass increasing by 1546 m/z. In the tyrosine grafting process, 13.12 μM/mg of free thiols (), corresponding to three thiol groups in BSA(cys), react with tyrosine. Meanwhile, the remaining four thiol groups re-form disulfide bonds, leading to the recovery of the disulfide bond content (122.51 μM/mg). Besides, tyrosine can also be linked to amines in BSA(cys).
Table 1. Free thiol and disulfide bond content of BSA, BSA(cys), Thiols-alkylated BSA, and Tyrosine-grafted BSA.
LC–MS of the peptides obtained by trypsin hydrolysis can provide the specific reactive cysteine residues in BSA. For sample thiols-alkylated BSA (), six peptides perform different masses, corresponding to the breakage of three disulfide bonds by L-cystine, which is consistent with the results of MALDI-TOF analysis. Observing the predicted molecular mass and peptide sequence of pure BSA (ExPASy Citation2005), six peptides are attributed to peptide sequence ETYGDMADCCEK (T1, 1364), NECFLSHK (T2, 977), YNGVFQECCQAEDK (T3, 1633), GACLLPK (T4, 701), DVCK (T5, 464), and EYEATLEECCAK (T6, 1388), respectively. According to the structure of BSA (PDBsum Citation2012), the three broken disulfide bonds are assigned with Cys-90 and Cys-101 in disulfide bond 3, Cys-167 and Cys-176 in disulfide bond 5, Cys-315 and Cys-360 in disulfide bond 10.
Table 2. Peptide mass (Mw) and sequence obtained by trypsin hydrolysis of BSA and Thiols-alkylated BSA.
Based on previous results (Li, Su, and Cavaco-Paulo Citation2021), tyrosine was grafted onto BSA by laccase-assisted via two paths. First, tyrosine was oxidized to monomer radical by laccase and then reacted with BSA to form BSA–tyrosine conjugate via Michael addition or Schiff base. Secondly, tyrosine was oxidized to dimers (mainly dityrosine) and dimer radicals, which may promote the cross-linking of BSA between amines.
Therefore, we assume that the formation of BSA(cys)–tyrosine conjugate is assembled by tyrosine monomer and BSA(cys). As listed in , for sample Tyrosine-grafted BSA, mass changes are detected in nine peptides (T2, T4, T5, T7, T8, T9, T10, T11, and T12). The Schiff base raises a molecular weight by 163 (without extra ions) of peptides T7, T8, and T9. Whereas tyrosines link to peptides T2, T4, T5, T10, T11, and T12 via Maillard addition, corresponding to molecular weight differences of 179 (without extra ions). The disulfide bond content in tyrosine-grafted BSA decreases by 13.12 μM/mg (assigned with three thiols), so we can confirm that tyrosines are grafted onto cysteine residues in peptides T2, T4, and T5 via C-S bond.
Table 3. Peptide mass (Mw) and sequence obtained by trypsin hydrolysis of BSA and Tyrosine-grafted BSA.
As only dimer tyrosine was formed by laccase-catalyzed in the absence or presence of BSA (Li, Su, and Cavaco-Paulo Citation2021), we may exclude the influence of other tyrosine oligomers on the BSA grafting system. In total, the LC–MS analysis provides four cross-linked peptides in sample Tyrosine-grafted BSA (). Dityrosine reacts as a “bridge” between peptides (T2 and T5, T4 and T8, T9 and T10, and T11 and T12), leading to a molecular weight increase of 358 m/z (without extra ions). The reactive residues are Cys-101 in T2, Cys-315 in T5, Cys-176 in T4, Lys-396 in T8, Lys-20 in T9, Lys-261 in T10, Lys-535 or Lys-537 in T11, and Lys-362 in T12. Hence, dityrosine can be adopted as a cross-linking agent not only between amines but also between thiols.
Table 4. Peak time at chromatogram spectra, peptide mass (Mw) and sequence of cross-linked peptides from Tyrosine-grafted BSA.
MD simulations were used to evaluate the overall stability of BSAwt and BSA-3CysSH monomers. Both Root Mean Square Deviation (RMSD) and the Dictionary of Secondary Structure of Proteins (DSSP) analysis () indicate that the two systems are very stable, maintaining a similar content of secondary structure (SS) to the one found in the X-ray structure (3V03) (). When the three disulfide bonds are reduced, a discretely smaller number of residues in helical conformation are found. Regarding the backbone and Cys RMSD values, an average value of around 0.4–0.55 nm is computed, and it seems that BSAwt deviates more from the experimental structure than its BSA-3CysSH analog. However, looking at , panels A-C, it is easy to conclude that RMSD values are due to the displacement of the loops and helices, but any unfolding is observed. Importantly, a great alignment is noticed when both modeled BSAs are superimposed (C). Panel D highlights the disulfide bond 5, linked and not linked, revealing that little perturbation is caused by the S-S reduction.
Figure 4. Representative snapshots of BSAwt and BSA-3Cyssh superimposed to the 3V03 ×-ray structure (a-b). Alignment of modeled BSAwt and BSA-3Cyssh (c) and zoom-in of Cys 176 linked (d left) and Cys 176 not linked (d right). In panel e, average RMSD (nm), using backbone fitting, and average no. of residues in a Secondary Structure (SS) in comparison to the experimental BSA. The color scheme presents gray for X-ray conformation, green for BSAwt and cyan for BSA-3Cyssh.
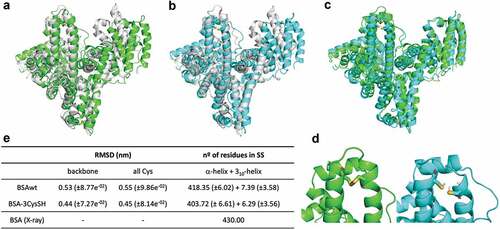
Hair shape changes
Hair fibers are composed of a hierarchical structure, and their amorphous region consists of sulfur-rich cysteine residues (Wagner and Joekes Citation2005). Disulfide bonds would form between two different cysteine residues in a single chain or between different peptide chains, which are responsible for protecting the stability of hair fibers (Liu et al. Citation2021). To change the shape of the hair, disulfide bonds are disrupted using L-cysteine, and tyrosine is used as the cross-linking agent between thiols.
The perming efficiency (PE) of tyrosine-grafted hair is compared with PE of commercial products (chemical treatment), control, and tyrosine-absorbed samples (). All tyrosine-grafted hair presents higher PE values than that measured for a commercial perming product before washing. However, only the sample which is pre-treated by 50.0 mM of L-cysteine holds the new shape after washing with PE of 55%–65% for all of the tested tyrosine concentrations. The best performance of hair curly formation and shape maintenance is accomplished by 50.0 mM of L-cysteine pre-treatment and 3.0 mM of tyrosine grafting via laccase-assisted.
Figure 5. Perming efficiency (PE) of tyrosine-grafted hair without and with L-cysteine pre-treatment compared to chemical treated and control samples.
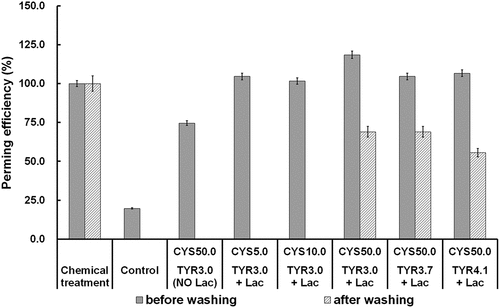
The commercial perming product keeps an excellent perming performance with its efficiency of almost 100% throughout washing procedures (Martins, Silva, and Cavaco-Paulo Citation2019). Observing , among the biological hair curling measurements, tyrosine grafting performs the second PE of 65% only to BSA coating (92%, Tinoco et al. Citation2021). In addition, tyrosine grafting can be conducted with a blow-drier at a relatively lower temperature (<85°C) and less time-consuming (about 45 min). The capacity of tyrosine to change and maintain hair curls can avoid using harsh chemicals, which negatively affect hair fiber properties, consumers’ health, and the environment. Therefore, adopting tyrosine as a hair curling agent is maneuverable and user-friendly in the hair industry.
Table 5. Biological methods used in hair curling and the hair’s perming efficiency throughout washing procedures.
FTIR spectra of raw, control, and tyrosine-grafted hair samples are depicted in . The typical bands of amide I (1630 cm−1), amide II (1520 cm−1), and amide III (1230 cm−1) appear in all hair samples. After grafting (line 5, 6), the phenolic hydroxyl groups in tyrosine are present at 3201 cm−1, and the stretching of C-O (1330 cm−1) in tyrosine and the typical tyrosine residue band (1042 cm−1) are increased (Navarro et al. Citation2018). Also, peaks at 1586 cm−1, 840 cm−1, and 795 cm−1 are caused by the changing of the aromatic structures in tyrosine (Shu et al. Citation2020). An additional peak at 649 cm−1 is attributed to C-S stretching (Tinoco et al. Citation2021), revealing the tyrosine links to thiols in hair via laccase-assisted grafting, which results in a decline of the free thiol content to about 0.5 μM/mg (). All results prove that tyrosine is successfully grafted onto curly hair.
Figure 6. FTIR spectra of hair samples: 1) untreated, Raw; 2) 50 mM L-cysteine pre-treated, CYS50.0; 3) treated with buffer, Control (before washing); 4) treated with buffer, Control (after washing); 5) L-cysteine pre-treatment (50 mM) followed by laccase-assisted tyrosine grafting (3.0 mM), CYS50.0-TYR3.0 + Lac (before washing), 6) L-cysteine pre-treatment (50 mM) followed by laccase-assisted tyrosine grafting (3.0 mM), CYS50.0-TYR3.0 + Lac (after washing).
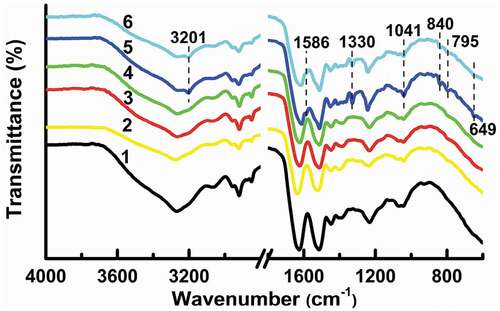
Table 6. Free thiol content, Young’s modulus, and breaking strength of raw hair, CYS50.0 pre-treated hair, control hair, and CYS50.0-TYR3.0 + Lac grafted hair.
The mechanism of hair curling is described in . The breakage of disulfide bonds and the rearrangement of new bonds between keratin molecules are the core technologies in hair modeling. L-cysteine pre-treatment plays a role in the disruption of disulfide bonds, and tyrosine acts as a cross-linking agent between thiols of cysteine residues in hair keratin. For the BSA grafting system, dityrosine is proved to be linked to two different thiol groups directly by LC–MS analysis. Tri-, tetra-, and oligo-tyrosine, even poly-tyrosine, may perform the same reaction (Li et al. Citation2022).
Figure 7. Mechanism of hair curling via L-cysteine pre-treatment followed by laccase-assisted tyrosine grafting.
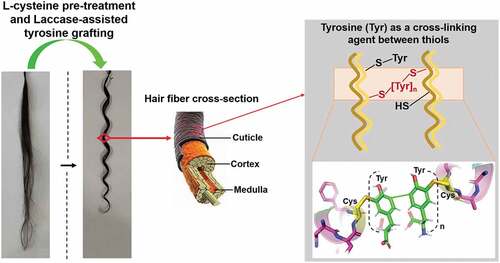
Disulfide cross-linking impacts hair’s mechanical properties, especially Young’s modulus (Robbins Citation2012). Increases of Young’s modulus and breaking strength are found in tyrosine-grafted hair (), due to the formation of new cross-linkages (S-dityrosine-S, S-dityrosine-NH, and NH-dityrosine-NH) between hair keratins. Tyrosine-grafted hair behaves with higher stiffness, having similar results with peptide coating methods used in hair reshaping (Malinauskyte et al. Citation2021; Tinoco et al. Citation2019, Citation2021). All hair samples show a decline in breaking strength after washing. Whereas the loss of breaking strength of tyrosine-grafted hair is not so pronounced compared to raw hair, which is consistent with previous results (Li et al. Citation2021). The establishment of new bonds plays a role in maintaining not only a new shape but also the structural integrity of the hair.
Conclusions
The curling formation of Asian hair is conducted using tyrosine as a cross-linking agent between thiols in L-cysteine pre-treated hair for the first time. The best operational conditions for the Asian hair modeling are conducted with L-cysteine pre-treatment (50.0 mM) and then grafting with tyrosine (3.0 mM), using laccase (18 U/mL) as a catalyst assisted with a blow-drier. The reshaped hair shows higher perming efficiency than commercial products before washing, while a lower curly performance after the washing procedure. The tyrosine grafting method has several advantages since no hash chemical agents are used, requiring a lower temperature and less time. Furthermore, the mechanical properties of grafted hair are performed without compromising since the new cross-linked bonds are established between tyrosine and hair. Moreover, the cross-linking of L-cysteine pre-treated hair is evidenced by using BSA as a protein model. Tyrosine can be used as a cross-linking agent between thiols and/or amines in BSA or hair.
Therefore, this new hair perming method promoted, L-cysteine pre-treatment followed by laccase-assisted tyrosine grafting, would be applied as an alternative approach to commercial hair perming. Tyrosine demonstrates a high potential to reshape keratin fibers and can be used as a “green” hair perming agent. The developed route herein presented may open a mild and maneuverable way to change the shape of keratin fibers.
Highlights
Disulfide bonds in BSA or hair are reduced by L-cysteine efficiently.
Tyrosine can be performed as a cross-linking agent between thiols and/or amines.
Curling of the Asian hair is accomplished by laccase-assisted tyrosine grafting.
This method offers a mild and maneuverable way to change the shape of keratin fibers.
Supplemental Material
Download MS Word (49.2 KB)Acknowledgements
This study was supported by the National Natural Science Foundation of China (Grant No. 52003108), China Postdoctoral Science Foundation funded project (2022M721359) and the Fundamental Research Funds for the Central Universities (JUSRP121027). This study was also supported by the Portuguese Foundation for Science and Technology (FCT) under the scope of the strategic funding of UIDB/04469/2020 unit.
Disclosure statement
No potential conflict of interest was reported by the authors.
Supplemental data
Supplemental data for this article can be accessed online at https://doi.org/10.1080/15440478.2023.2187506.
Additional information
Funding
References
- Basit, A., M. W. Sadaf, S. Akhtar, and M. W. Akhtar. 2018. Health improvement of human hair and their reshaping using recombinant keratin K31. Biotechnology Reports 20:e00288. doi:10.1016/j.btre.2018.e00288.
- Cruz, C. F., C. Costa, A. C. Gomes, T. Matamá, and A. Cavaco-Paulo. 2016. Human hair and the impact of cosmetic procedures: A review on cleansing and shape-modulating cosmetics. Cosmetics 3:26. doi:10.3390/cosmetics3030026.
- Cruz, C. F., M. M. Fernandes, A. C. Gomes, L. Coderch, M. Martí, S. Méndez, L. Gales, N. G. Azoia, U. Shimanovich, A. Cavaco-Paulo. 2013. Keratins and lipids in ethnic hair. International Journal of Cosmetic Science 35 (3):244–12. doi:10.1111/ics.12035.
- Cruz, C. F., M. Martins, J. Egipto, H. Osório, A. Ribeiro, and A. Cavaco-Paulo. 2017. Changing the shape of hair with keratin peptides. RSC Advances 7:51581–92. doi:10.1039/C7RA10461H.
- Deb-Choudhury, S., J. E. Plowman, and D. P. Harland. 2016. Isolation and analysis of keratins and keratin-associated proteins from hair and wool. Methods in Enzymology 568:279–301. doi:10.1016/bs.mie.2015.07.018.
- ExPASy. 2005. SIB bIoinformatics resource portal, PeptideMass. Retrieved from https://web.expasy.org/cgi-bin/peptide_mass/peptide-mass.pl
- Fernandes, M., and A. Cavaco-Paulo. 2012. Protein disulphide isomerase-mediated grafting of cysteine-containing peptides onto over-bleached hair. Biocatalysis and Biotransformation 30:10–19. doi:10.3109/10242422.2012.644436.
- Fernandes, M., C. F. Lima, A. Loureiro, A. C. Gomes, and A. Cavaco-paulo. 2012. Keratin-based peptide: Biological evaluation and strengthening properties on relaxed hair. International Journal of Cosmetic Science 34:338–46. doi:10.1111/j.1468-2494.2012.00727.x.
- Galli, C. L., F. Bettin, P. Metra, P. Fidente, E. De Dominicis, and M. Marinovich. 2015. Novel analytical method to measure formaldehyde release from heated hair straightening cosmetic products: Impact on risk assessment. Regulatory Toxicology and Pharmacology 72:562–68. doi:10.1016/j.yrtph.2015.05.010.
- Hansen, R. E., H. Østergaard, P. Nørgaard, and J. R. Winther. 2007. Quantification of protein thiols and dithiols in the picomolar range using sodium borohydride and 4, 4′-dithiodipyridine. Analytical Biochemistry 363:77–82. doi:10.1016/j.ab.2007.01.002.
- Ito, A., K. Nishioka, H. Kanto, A. Yagami, S. Yamada, M. Sugiura, C. Yasunaga, K. Yoshii, H. Kobayashi, A. Adachi, et al. 2017. A multi-institutional joint study of contact dermatitis related to hair colouring and perming agents in Japan. Contact Dermatitis. 77(1):42–48. doi:10.1111/cod.12783.
- Joo, K. M., A. R. Kim, S. N. Kim, B. M. Kim, H. K. Lee, S. J. Bae, J. -H. Lee, and K. -M. Lim. 2016. Metabolomic analysis of amino acids and lipids in human hair altered by dyeing, perming and bleaching. Experimental Dermatology 25 (9):729–31. doi:10.1111/exd.13025.
- Lee, Y., Y. D. Kim, L. Q. Pi, S. Y. Lee, H. Hong, and W. S. Lee. 2014. Comparison of hair shaft damage after chemical treatment in Asian, White European, and African hair. International Journal of Dermatology 53:1103–10. doi:10.1111/ijd.12247.
- Li, Y., J. Noro, J. Li, C. Silva, J. Su, A. Cavaco-Paulo, Q. Gao, C. Wei, and S. Miao. 2022. Grafting of poly(tyrosine) by laccase improves the tensile strength and anti-shrinkage of wool. Journal of Natural Fibers 19 (15):1–13. doi:10.1080/15440478.2021.2002785.
- Li, Y., J. Noro, M. Martins, S. Jing, C. Silva, and A. Cavaco-Paulo. 2021. Changing the shape of wool yarns via laccase-mediated grafting of tyrosine. Journal of Biotechnology 339:73–80. doi:10.1016/j.jbiotec.2021.08.001.
- Li, Y., J. Su, and A. Cavaco-Paulo. 2021. Laccase-catalyzed cross-linking of BSA mediated by tyrosine. International Journal of Biological Macromolecules 166:798–805. doi:10.1016/j.ijbiomac.2020.10.237.
- Liu, H., L. Zhao, G. Zhang, F. Pan, and W. Yu. 2021. Mechanics and hierarchical structure transformation mechanism of wool fibers. Textile Research Journal 91:496–507. doi:10.1177/0040517520944249.
- Madnani, N., and K. Khan. 2013. Hair cosmetics. Indian Journal of Dermatology, Venereology and Leprology 79:654–67. doi:10.4103/0378-6323.116734.
- Majorek, K. A., P. J. Porebski, A. Dayal, M. D. Zimmerman, K. Jablonska, A. J. Stewart, M. Chruszcz, and W. Minor. 2012. Structural and immunologic characterization of bovine, horse, and rabbit serum albumins. Molecular Immunology 52 (3–4):174–82. doi:10.1016/j.molimm.2012.05.011.
- Malinauskyte, E., R. Shrestha, P. Cornwell, S. Gourion-arsiquaud, and M. Hindley. 2021. Penetration of different molecular weight hydrolysed keratins into hair fibres and their effects on the physical properties of textured hair. International Journal of Cosmetic Science 43 (1):26–37. doi:10.1111/ics.12663.
- Maneli, M. H., P. Smith, and N. P. Khumalo. 2014. Elevated formaldehyde concentration in “Brazilian keratin type” hair-straightening products: A cross-sectional study. Journal of the American Academy of Dermatology 70 (2):276–80. doi:10.1016/j.jaad.2013.10.023.
- Martins, M., C. Silva, and A. Cavaco-Paulo. 2019. α-Chymotrypsin catalysed oligopeptide synthesis for hair modelling. Journal of Cleaner Production 237:117743. doi:10.1016/j.jclepro.2019.117743.
- Miranda-vilela, A., A. D. J. Botelho, and L. Muehlmann. 2014. An overview of chemical straightening of human hair: Technical aspects, potential risks to hair fibre and health and legal issues. International Journal of Cosmetic Science 36 (1):2–11. doi:10.1111/ics.12093.
- Moll, R., M. Divo, and L. Langbein. 2008. The human keratins: Biology and pathology. Histochemistry and Cell Biology 129:705–33. doi:10.1007/s00418-008-0435-6.
- Navarro, J., J. Swayambunathan, M. Santoro, and J. Fisher. 2018. Assessment of the effects of energy density in crosslinking of keratin-based photo-sensitive resin. Paper presented at the annual meeting for the 2018 IX International Seminar of Biomedical Engineering (SIB), Bogota, Colombia, May 16-18.
- PDBsum. 2012. Pdbsum entry 3V03. Retrieved from https://www.ebi.ac.uk/thornton-srv/databases/cgi-bin/pdbsum/GetPage.pl?pdbcode=3v03&template=main.html
- Robbins, C. R. 2012. Chemical and physical behavior of human hair. Berlin: Springer.
- Shu, T., Y. Sun, Y. Bai, X. Lin, Z. Zhou, L. Su, and X. Zhang. 2020. Rational design of “Three-in-One” ratiometric nanoprobes: Protein-caged dityrosine, CdS quantum dots, and gold nanoclusters. ACS Omega 5 (15):8943–51. doi:10.1021/acsomega.0c00711.
- Tinoco, A., E. Antunes, M. Martins, F. Gonçalves, A. C. Gomes, C. Silva, A. Cavaco-Paulo, and A. Ribeiro. 2019. Fusion proteins with chromogenic and keratin binding modules. Scientific Reports 9 (1):14044. doi:10.1038/s41598-019-50283-0.
- Tinoco, A., A. F. Costa, S. Luís, M. Martins, A. Cavaco-Paulo, and A. Ribeiro. 2021. Proteins as hair styling agents. Applied Sciences 11:4245. doi:10.3390/app11094245.
- Tinoco, A., F. Gonçalves, A. F. Costa, D. S. Freitas, A. Cavaco-Paulo, and A. Ribeiro. 2021. Keratin: Zein particles as vehicles for fragrance release on hair. Industrial Crops and Products 159:113067. doi:10.1016/j.indcrop.2020.113067.
- Tinoco, A., J. Gonçalves, C. Silva, A. Cavaco-Paulo, and A. Ribeiro. 2019. Crystallin fusion proteins improve the thermal properties of hair. Frontiers in Bioengineering and Biotechnology 7:298. doi:10.3389/fbioe.2019.00298.
- Wagner, R. D. C. C., and I. Joekes. 2005. Hair protein removal by sodium dodecyl sulfate. Colloids and Surfaces B-Biointerfaces 41 (1):7–14. doi:10.1016/j.colsurfb.2004.10.023.
- Wang, L., A. Cavaco-Paulo, B. Xu, and M. Martins. 2019. Polymeric hydrogel coating for modulating the shape of keratin fiber. Frontiers in Chemistry 7:749. doi:10.3389/fchem.2019.00749.