ABSTRACT
In order to prepare fiber-type nanohybrid composites for electrochemical and antimicrobial applications, polypyrrole was combined with carboxymethylcellulose and multiwalled carbon nanotubes (PPy/CMC/MWCNTS). These composite materials were synthesized using ultrasonication and in situ polymerization. By using analytical methods, the structure and morphology of the synthesized nanohybrid composite material were confirmed. Slurry of fiber-type PPY/CMC/MWCNT composites synthesized in ethanol was deposited as a thin-uniform layer with conductive nafion (5% ethanolic solution of nafion) binder. Based on electrochemical measurements in a phosphate buffer medium, Sb3+ ions have linear responses between 0.1 nM and 0.01 mM, which is known as linear dynamic range (LDR). A sensor’s sensitivity (22.4304 µAµM−1cm−2) is calculated using the LDR slope by considering the GCE surface area (0.0316 cm2). A signal-to-noise ratio of 3 is used to estimate the lower limit of detection which is equal to 96.82 ± 4.84 pM. Moreover, the antibacterial activity of the obtained polypyrrole/carboxymethylcellulose/MWCNT composite has also been evaluated “against Gram-positive bacteria B. subtilis along with S. aureus, as well as Gram-negative bacteria, P. aeruginosa along with Escherichia coli by utilizing” autoclave of agar media. In electrochemical analysis, the proposed Sb3+ cationic sensor exhibits appreciable reproducibility, response time, stability, and outstanding outcome in analysis of real samples. In the field of metal ion sensor, this reliable method might be prospective in the recent future.
摘要
为了制备用于电化学和抗菌应用的纤维型纳米杂化复合材料, 将聚吡咯与羧甲基纤维素和多壁碳纳米管(PPy/CMC/MWCNTS)相结合. 这些复合材料是通过超声处理和原位聚合合成的. 通过分析方法, 证实了合成的纳米杂化复合材料的结构和形态. 将在乙醇中合成的纤维型PPY/CMC/MWCNT复合材料浆料与导电nafion(5%的nafion乙醇溶液)粘合剂沉积为均匀的薄层. 根据在磷酸盐缓冲介质中的电化学测量,Sb3+离子具有0.1 nM和0.01 mM之间的线性响应, 这被称为线性动态范围(LDR). 通过考虑GCE表面积(0.0316 cm2), 使用LDR斜率计算传感器的灵敏度(22.4304 µAµM-1 cm-2). 使用3的信噪比来估计检测的下限,该下限相等96.82 ± 4.84 pM. 此外,还评估了所获得的聚吡咯/羧甲基纤维素/MWCNT复合物的抗菌活性,“通过使用”琼脂培养基的高压釜“对抗革兰氏阳性菌枯草芽孢杆菌和金黄色葡萄球菌, 以及革兰氏阴性菌、铜绿假单胞菌和大肠杆菌.” 在电化学分析中,所提出的Sb3+阳离子传感器在实际样品的分析中表现出可观的再现性、响应时间、稳定性和出色的结果. 在金属离子传感器领域,这种可靠的方法在最近的将来可能是有前景的.
Introduction
Our daily lives involve a wide variety of nano composites, depending on our needs, which are also found in nature due to their polymer content. Biopolymers such as cellulose are prevalent on earth and present in animals and plants. Many different resources have been used to extract cellulose, including pineapples, corn cobs, soy hulls, hemp fibers, rice husks, etc. (Costa et al. Citation2013; Dai, Fan, and Collins Citation2013; Johar, Ahmad, and Dufresne Citation2012; Silverio et al. Citation2013). It is the most abundant renewable organic raw material in the world and a biodegradable complex polysaccharide. Natural linear homopolysaccharides (polysaccharides) like cellulose are made up of D-glucopyranose rings linked by β-(1–4)-glycosidic chains (Nishino Citation2004). Cellulose has some limitations in its application due to its strong hydrogen bonding, including its difficulty in solubilizing. It has been proposed to overcome the problem of non-derivatizing solvents for cellulose, which do not interact chemically with the hydroxyl groups (Pinkert et al. Citation2009; Vitz et al. Citation2009). There have been several suggestions for overcoming this problem (Gousse et al. Citation2002, Citation2004; Grunert and Winter Citation2002; Ifuku et al. Citation2007; Yuan et al. Citation2006). Several methods have been proposed for chemically modifying cellulosic fillers, including using acetic anhydride, alkenyl succinic anhydrides, chlorosilanes, or hexamethyldisilazane. It was the first-time cellulose whisker-based composites were produced (Favier, Chanzy, and Cavaille Citation1995). In the following years, many more studies were carried out using polylactide (Espino-Perez et al. Citation2013), polymethylmethacrylate (Dong et al. Citation2012; Fahma et al. Citation2013; Thakur, Singh, and Misra Citation2011) and polyvinyl acetate (Garcia de Rodriguez, Thielemans, and Dufresne Citation2006).
In CMC, some carboxymethyl groups are attached to hydroxyl groups in cellulose. Sodium carboxymethyl groups give cellulose its soluble and chemically reactive properties (Jackson et al. Citation2011; Tongdesoontorn et al. Citation2011). The properties of CMC include chemical resistance, membrane formation, and binding. A variety of technological applications can be derived from its interesting properties. Due to its hydroxyl groups and bonded water molecules, CMC is soluble in water and environmentally friendly. A carbon nanotube has a much finer scale than a common fiber. It’s made up of fullerene hemispheres that are rolled around graphene sheets (Foldyna, Foldyna, and Zeleňák Citation2016). CNTs show a lot of interesting properties due to sp2 hybridization of carbon – carbon bonds. Recently, dispersing carbon nanomaterials in nanocellulosic matrixes has enabled the development of functional hybrid composite (Bacakova et al. Citation2020; Chen et al. Citation2018; Du et al. Citation2017; Durairaj et al. Citation2021; Koga et al. Citation2013; Nguyen et al. Citation2019; Wang et al. Citation2020; Yu et al. Citation2020). Researcher have found that MWCNTs that contain a high concentration of negatively charged sulfate functional groups exhibit different behavior in the composite electrode when attached to negatively charged sulfate molecules (Durairaj et al. Citation2019). Over the past few years, nanocellulose/nanocarbon composites have become increasingly popular for electrochemical applications (Anirudhan et al. Citation2018; Khan Citation2020; Ortolani et al. Citation2019; Shalauddin et al. Citation2019; Zhao et al. Citation2020).Various anthropogenic sources contain antimony (Sb) as a trace element in the environment. In the mining, processing, and casting industries including pewter and tin, this element is extremely abundant. The element is also used in solder, lead storage batteries, bearings, sheet metal, castings, and pewter alloys. As well as being an excellent fire retardant, antimony can be found in the formulation of textiles, plastics, paints, paper, and rubber. It is antimony trisulfide that produces explosives, antimony salts, pigments, and ruby glass (Nam, Yang, and An Citation2009; Zanetti et al. Citation2002). The semiconductor industry uses antimony to produce optoelectrical devices and integrated circuits (Bustamante et al. Citation1997) in the form of gallium antimonide (GaSb) and indium antimonide (InSb). It has been found that antimony cation (Sb3+) can be used to treat AIDs, HPA-23 and tropical diseases (billarziosis, leishmaniasis, trypanosomiasis, and schistosomiasis) (Lonard and Gerber Citation1996; Rozenbaum et al. Citation1985). Environmental contamination is a high risk due to antimony’s excessive industrial use. When humans are exposed to antimony they may suffer damage to their organs, specifically their hearts, livers, kidneys, and lungs. Antimony may cause DNA damage or cancer through long-term occupational exposure (Kanematsu, Hara, and Kada Citation1980; Kuroda et al. Citation1991). Long term occupational exposure through drinking water or inhalation has been reported as causing lung tumors (Nikula et al. Citation1995). Trivalent antimony has been linked to cardiovascular disease, hemolytic anemia, and acute renal failure (Su et al. Citation2014; Vinhal et al. Citation2016). To ensure a safe and healthy workplace, antimony monitoring should also be implemented in the drinking water supply. The detection of Sb3+ cations has been carried out using conventional analytical methods, including capillary electrophoresis (Casiot et al. Citation1998), UV-Vis spectrophotometer (El-Sharjawy and Amin Citation2016), inductively coupled plasma optical emission spectrometry (Hansen and Pergantis Citation2006), laser-induced fluorescence (Enger et al. Citation1995), spectrofluorimetric (Yu et al. Citation2009) and HPLC-HGAFS (high-performance liquid chromatography coupled with hydride generation and atomic fluorescence spectrometry (De Gregori et al. Citation2007). The electrochemical (I-V) method is becoming more and more popular because it has several disadvantages compared to these conventional methods such as being expensive, heavy, complicated, time-consuming, and difficult to import. There have been numerous research studies to detect numerous heavy metal ions using an electrochemical approach (Hussein et al. Citation2018, Citation2019; Khan et al. Citation2016, Citation2017, Citation2018, Citation2019; Rahman et al. Citation2018a, Citation2018b). and these studies are based on organometallic composites. Thus, the purpose of this study is to develop an electrochemical sensor based on biodegradable composites for the detection of heavy and environmentally unsafe toxic metal ions. The combination of cellulose and carbon nanoparticles possesses several advantages over materials containing cellulose nanoparticles or carbon nanoparticles alone. In addition to increasing the mechanical strength of nanocellulose materials, adding carbon nanoparticles can further enhance their properties (Liu et al. Citation2017; Zhu, Liu, and Mathew Citation2017). Adding these compounds to diamond nanoparticles, graphene, carbon nanotubes, or graphene-containing nanocellulose can improve their electrical properties (Abol-Fotouh et al. Citation2019; Buzid et al. Citation2019; Siljander et al. Citation2018). A variety of industrial and technological applications can be made with nanocellulose/nanocarbon composites, such as the purification of water and the separation of atoms and molecules (Alizadehgiashi et al. Citation2018; Liang et al. Citation2019; Vetrivel et al. Citation2018). A polypyrrole/polysaccharide composite system can be considered an interesting area of research because of its low cost, stable redox properties, and interesting nanomorphologies. A conjugated polymer that is most prominent is polypyrrole, which is a promising conducting polymer in electronic, biological, and medical applications because it can be easily polymerized, stable in the environment, and highly conductible by altering doping levels (Ferenets and Harlin Citation2007). A nanohybrid composite of polypyrrole, carboxymethylcellulose, and multiwalled carbon nanotubes was developed for antimicrobial and electrochemical applications. A composite was confirmed using structural, morphological, and electrochemical sensor applications developed by GCE. An electrochemical sensor for detecting Sb3+ cation has been developed by electrochemically coating biodegradable composites of PPY/CMC/MWCNTS with GCE. Five analytical characteristics are calculated for the selective detection of antimony with fabricated sensor probe such as sensitivity, LDR, DL, reproducibility, and response time. A Sb3+ ion sensor was evaluated by analyzing real samples, which are collected from different locations of environmental sources. Consequently, it is the easiest and most facile method of constructing a metal ionic sensor probe based on PPY/CMC/MWCNTS/GCE, which could also demonstrate to be a novel approach for advancing metal ion sensors in a broad scale in the fields of environmental and health.
Experimental section
Materials and methods
The cellulose used was carboxymethyl cellulose (Fluka) that was not purified further. MWCNT was received from Applied Science Innovations, India. Nitric acid, and ethanol. The study required the use of chemicals of analytical grade, including HgCl2, Y(NO3)3, Ba(NO3)2, MgCl2, AsCl3, SnCl2, SbCl3, FeCl3, CrCl3 and AgNO3 were parched from Sigma-Aldrich company (USA) and without further refinement used directly. Nafion (5% suspension in ethanol) was used in this study in combination with monosodium and disodium phosphate buffers. PPy/CMC/MWCNT composite morphology has been obtained by using a JEOL JSM-7610 FPLUS model USIF, AMU Aligarh at various amplifications. The nutrient agar plate was prepared, by the pour plate technique after the autoclave sterilizer machine (JSR Autoclave, JSAC-80 Korea). The Keithley electrometer which is fabricated in the USA was applied to electrochemical (I-V) analysis.
Synthesized of PPY/CMC/MWCNT composite
During this study, pyrrole was polymerized in situ in the presence of a dispersion of CMC/MWCNT in water. PPY/CMC/MWCNT composites were prepared using the same CMC/MWCNTs in our previous studies (Khan Citation2020). The CMC/MWCNT composite was first dispersed in de-ionized water for 50 ml using a sonicator. Pyrrole was then added to the CMC/MWCNT composite under sonication for 1 hour to load the pyrrole into the CMC/MWCNT composite. The reaction solution was mixed with 30 mL of FeCl3 solution in DDW to polymerize pyrrole in situ within CMC/MWCNT composite. As a result of filtration, washes with ethanol and distilled water, and transfer to an alumina crucible, the precipitate was formed into a hybrid composite. After this, the PPY/CMC/MWCNT was placed in an oven overnight to dry. PPY/CMC/MWCNT had been sufficiently characterized and was ready for use.
Assessment of antibacterial activity of PPY/CMC/MWCNT composite
Zone inhibition assay
The synthetic materials were tested for their antibacterial properties against Gram-positive bacteria S. aeruginosa and B. subtilis, and Gram-negative bacteria P. aeruginosa and E. coli. These bacterial cultures were grown in Luria Bertani broth, and a loopful culture was inoculated into the 100 ml liquid broth and brooded in a rotatory incubator for optimum growth acquisition. A fresh culture of each strain was obtained by the re-culturing method in the same media and optimum condition. The nutrient agar plate was prepared, by the pour plate technique after the autoclave of media at the ideal temperature of 121°C and pressure at 15 psi for 20 min in a sterilizer machine (JSR Autoclave, JSAC-80 Korea). After the media pouring into the Petri plates wait 10 min, the media becomes solidified. From the fresh culture tubes, each bacterial strain was spread on the media plates and held up for 10 min to retain the way of life by the plate’s media. Further, 6 mm wells were set up in each plate for an explicit sum of 25, 50, and 100 µg suspensions of the newly synthesized compound were added into the wells. The compound from the media wells becomes diffused into the surrounding media and uncovers the antimicrobial activity around the wells after the optimum brooding overnight at 37°C. Standard antibiotic amoxicillin was utilized as a control.
PPY/CMC/MWCNT composite MIC and MBC determination
The newly synthesized MWCNT/cellulose composite effect on bacterial growth in broth was assayed and determined the minimum inhibitory concentration and minimum bactericidal concentration. Newly synthesized MWCNT/cellulose composite was suspended in the mili Q water, and vertex was further utilized as an antibacterial agent against selected test microorganisms. To determine whether bacteria can survive or not against a test agent or composite material, the broth dilution method is commonly used. The synthesized composite material was supplemented at a varying concentration from 0 to 100 µg/ml. First, 100 ml broth media was set up into twenty 500 ml flasks and autoclaved concurring measures techniques in the sterilizer. Further, the four sets taught by test strains and 25 to 100 µg/ml amount of compound were supplemented in the medium and incubated at a rotatory incubator at 37°C for overnight. The bacterial culture was standardized according to the McFarland 0.5 protocol for testing the antimicrobial susceptibility of compounds. The serial dilutions of the composite materials were done with a broth medium and then 5 × 106 CFU containing bacterial culture was inoculated for a 16 h incubation period. After the incubation period, bacterial culture turbidity and plate count were measured.
Fabrication of working electrode by PPY/CMC/MWCNTS composite
This study focuses on developing a working electrode for the proposed sensor. A thick fiber-type slurry of synthesized PPY/CMC/MWCNTS NCs was suspended in ethanol, formed into a thin uniform layer, and deposited on GCE (surface area 0.0316 cm2). Once the modified GCE had dried with PPY/CMC/MWCNTS, Nafion was added as a conductor coating binder between composite and electrode. An hour was needed for the product to completely dry in the oven. Keithley electrometers were used to assemble the electrochemical sensor, with the modified GCE and Pt wires connected in series. In order to implement the desired analyte, a sequence of solutions ranging in concentration from 0.1 mM to 0.1 nM were prepared from the stock (0.1 mM) solution of Sb3+ ion. Sb3+ ion concentration vs. current was explored in a plot known as calibration. GCE’s surface area and the slope of the calibration curve were used to calculate the sensitivity. A signal-to-noise ratio of 3 was used to estimate the sensor’s detection limit. I-V analysis was carried out using 10.0 mL of buffer solution in the detection beaker throughout the investigation. Keithley electrometer-based sensors rely on two electrodes for their operation.
Results and discussion
In , the steps for the preparation of PPY/CMC/MWCNTS are shown. PPY is produced by the oxidative chemical polymerization of ferric chloride in an aqueous solution that contains CMC/MWCNTS. In the process of separating the solution from the reaction product, ethanol and distilled water were used to wash the product. This was done to yield a dark dispersion that could then be precipitated. After drying and washing in ethanol, it was examined by SEM. As-synthesized materials can be identified by analyzing their shape, size, growth, and morphology through SEM. SEM graphs of PPY/CMC/MWCNTS composites () show that the particles are mostly spherical, like PPY that settles on CMC/MWCNTS fibers in irregular clusters. PPY molecules conformation and rearrangement are greatly influenced by the extent of hydrogen bonding between and within a composite material. A PPY chain forms a pi–pi stack in sp2 carbon of MWCNTs when it is attached to carboxylic and hydroxyl groups, but it forms inter/intra hydrogen bonds between its hydrogen atoms in CMC molecules (Janaki et al. Citation2013). In order to understand material properties, XRD techniques are widely used. The results of XRD analysis of the composite PPY/CMC/MWCNTS are shown in . The sharp peaks at 2θ = 25.8° indicate parallel and perpendicular lattice periodicity in the composite and might indicate a certain degree of crystallinity. In accordance with previous studies (Zavadskii Citation2004), PPy/CMC/MWCNT composite exhibits broad and weak peaks due to its PPY shell layers. Higher peaks at 2θ = 42–44◦ may be attributed to MWCNTs, indicating successful synthesis of PPY/CMC/MWCNTS composite (El-Shishtawy et al. Citation2018; Khan et al. Citation2019).
Figure 1. Schematic representation of electrochemical detection by PPY/CMC/MWCNTS Cs/binder/GCE, as well as the antibacterial activity by PPY/CMC/MWCNTS composite.
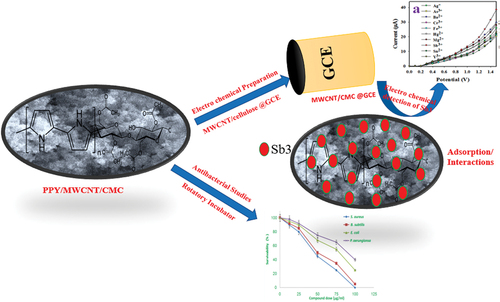
Electrochemical detection of Sb3+ with PPY/CMC/MWCNTS NCs/binder/GCE
PPY/CMC/MWCNTS NCs were successfully synthesized and used to develop an electrochemical sensor selective to Sb3+ in phosphate buffer with pH 7.0. NCs in ethanol were deposited as thin films onto the flat top of the GCE (0.0316 cm2) with a conducting nafion (5% suspension in ethanol) binder to improve its stability. Nafion, known as a conductive copolymer, has been applied as a binding agent. This has improved the binding strength between NCs and GCE, as well as increased the electron transfer rate of working sensors. Previous reports (Alam et al. Citation2019; Rahman, Alam, and Asiri Citation2019) have reported similar observations relating to the detection of various metal ions and chemicals using the I-V method. PPY/CMC/MWCNTS NCs/binder/GCE thin films are electrochemically analyzed at the applied potential by measuring the resulted current and holding time in the electrometer at 1.0 s. This study aims to detect selectivity first. An electrochemical sensor based on PPY/CMC/MWCNTS NCs/binder/GCE was recently assembled to analyze a number of heavy metal ions of 0.1 M concentration, including Hg2+, Y3+, Ba2+, Mg2+, As3+, Sn2+, Sb3+, Fe3+, Cr3+, and Ag+ in phosphate buffer medium of pH 7.0 as illustrated in . As shown in , considering that the antimony (Sb3+) ion exhibits a very high I-V response, sensor assembly toxic metal ions may be defined as selective toxic metal ions. The I-V analysis of the Sb3+ ion was carried out with various concentrations ranging from 0.1 nM to 0.1 mM, and the results are shown in . shows that the measurements were performed in a potential range of 0 ~ +1.5 V in a phosphate buffer of pH 7.0. There was a sequence of I-V responses from lower to higher concentrations of Sb3+ ions. As a result, it has been shown that the I-V responses during the analysis of Sb3+ are directly related to its concentration, and an electrochemical approach has been used to detect a wide range of toxic chemicals in previous articles of ours (Karim et al. Citation2019; Rahman et al. Citation2018).
Figure 4. Analysing the electrochemical behavior of PPY/CMC/MWCNTS Cs in the presence of binders/GCEs. (a) Using 0.1 µM concentration of analytes at potential 0 ~ +1.5 V, (b) the electrochemical response (I-V) to Sb3+, (c) the calibration of the Sb3+ ion sensor, (d) current vs. log[Sb3+] ion.
![Figure 4. Analysing the electrochemical behavior of PPY/CMC/MWCNTS Cs in the presence of binders/GCEs. (a) Using 0.1 µM concentration of analytes at potential 0 ~ +1.5 V, (b) the electrochemical response (I-V) to Sb3+, (c) the calibration of the Sb3+ ion sensor, (d) current vs. log[Sb3+] ion.](/cms/asset/41ec72ab-8f8a-48e2-8caf-8d6565cee28d/wjnf_a_2214383_f0004_oc.jpg)
Based on the linear relationship of current vs. concentration of Sb3+ ions shown in , a calibration plot was constructed to determine the calibration of the Sb3+ cationic sensor. To explore the current vs. concentration of Sb3+ ions, the currents at potential +1.5 V from the experiment shown in , are collected. clearly displays linear dynamic range (LDR) defined as data scattered between 0.1 nM and 0.01 mM on a line, and the equation of this line can be expressed by the equation y = 0.7088×+35.842. Based on the calibration curve slope (0.7088 µAµM−1) and the area of the GCE (0.0316 cm2), the calculated sensitivity is 22.4304 AM−1cm−2, which is an impressive value. In an experiment with a signal-to-noise ratio of 3, 96.82 ± 4.84 pM was calculated as the detection limit and a satisfactory lower limit of detection was determined.
Based on PPY/CMC/MWCNTS NCs/binders/GCE, the proposed Sb3+ ion sensor must prove to be reliable. As demonstrated in , various experiments were conducted to compare the I-V responses of coated and bare GCEs, response time, reproducibility, and long-term performance under identical conditions. An electrochemical study with 0.01 M Sb3+ ion solution at 0.1 V potential in phosphate buffer was performed with coated and bare GCEs in . Obviously, the GCE coated with PPY/CMC/MWCNTS NCs shows the highest intensity. It is very relevant to measure the response time of an electrochemical sensor in order to evaluate its efficiency. It is a measure of the time required for a full I-V response to develop at steady state. Therefore, the response time of Sb3+ ion sensor was performed with 0.1 µM Sb3+ ion solution in phosphate buffer medium as illustrated in . As illustrated in , the projected Sb3+ ion sensor has a very short response time to analyze Sb3+ ions.
Figure 5. Based on PPY/CMC/MWCNTS Cs/binders/GCE, tests are performed to establish the reliability of the Sb3+ ion sensor. Among them are: (a) an I-V comparison between coated and bare GCE for 0.1 M Sb3+ ion in phosphate buffer medium, (b) an evaluation of the proposed Sb3+ ion sensor with 0.1 M Sb3+ ion, (c) a reproducibility test, and (d) a long-term performance evaluation.
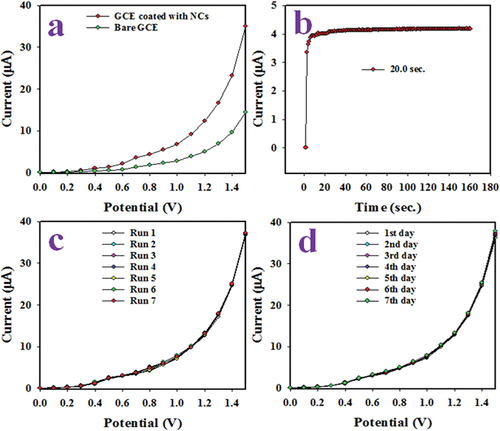
Response of electrochemical sensors must be reproducible, which is defined as the ability to produce similar I-V responses under identical conditions. In the phosphate buffer medium, pH 7.0, 0.1 µM Sb3+ ions were used as a sensor calibration sample for a reproducibility analysis in . Seven consecutive runs produced no distinctive or dispersed results and the coated GCE returned the same results after each run, even after being washed. As a result of this test, it has been confirmed that all real samples can be used to measure the selective Sb3+ ions. In terms of percentage of relative standard deviation (%RSD), the precision of the I-V responses of the reproducibility test has been measured within 0 to +1.5 V potential and is found as 0.56%. The performance of real-time applications must be high for a long period of time, while maintaining the same level of precision. A reproducibility test was performed on the proposed sensor under identical conditions for around 7 days. The observation from shows the same result as in the reproducibility performances. Therefore, the Sb3+ ion can be assumed to be stable for a long period of time in the phosphate buffer phase. The scientific acceptability of this study is demonstrated and presented in , which represents the analytical performance of the Sb3+ ion sensor, including its sensitivity, LDR, and DL. shows that the Sb3+ ionic sensor based on PPY/CMC/MWCNTS NCs/binder/GCE provides appreciable outstanding performance.
Table 1. Sensors analytical performance toward Sb3+ cation compared with other composite materials.
Investigation of real samples by fabricated sensor assembly
Finally, using the recovery method, it was examined a number of real samples such as tap-water, well-water, sea-water, and mineral-water for the detection of Sb3+ with fabricated PPY/CMC/MWCNTS NCs/binders/GCE as a sensor probe by electrochemical method. The results are presented in which seem quite satisfactory.
Table 2. Validation of environmental samples analyses using PPY/CMC/MWCNTS BCs, binders, and GCE sensors.
PPY/CMC/MWCNTS composite for antibacterial performance
An antimicrobial test was performed on the newly synthesized composite PPY/CMC/MWCNTS against gram-negative and gram-positive strains of bacteria. This composite material showed incredible antimicrobial activities against both grams of positive and negative bacterial strains. Gram-positive bacterial strain was more significantly affected than Gram-negative bacterial strain in both assays. The bacterial strain of S. aureus and B. subtilis growth was significantly influenced and developed a bacterial growth hindrance zone around the well, where the compound diffused into the surrounding media. Dissemination of antimicrobial mixes on media controls the growth and development of bacterial growth layers on media plates. The most significant effect in the form of zone inhibition range 22 ± 0.50 and 19 ± 0.25 mm appeared against S. aureus and B. subtilis separately and 18 ± 0.25 and 17 ± 0.5 mm zone inhibition was recorded against E. coli and P. aeruginosa at 100 µg/ml concentration (). These outcomes were more critically contrasted with the standard antibiotic. Further, antimicrobial action was checked in a broth and analyzed MIC and MBC. The inhibitory concentration of up to 30 ± 5 and 100 ± 10 µg/ml against Gram-positive and Gram-negative strains respectively was observed. All test microorganisms’ development was effectively influenced when increasing dose concentration in liquid media connected to the media plate and observing the bacterial survivability against drugs (). Overall, the newly synthesized composite material has an antimicrobial activity that makes it rust-free and applicable in the medical device coating.
Figure 6. Antimicrobial effect of PPY/CMC/MWCNTS composite against both grams of positive and negative bacterial strains. Image showing excellent dose-response, increasing concentration significantly retard the bacterial.
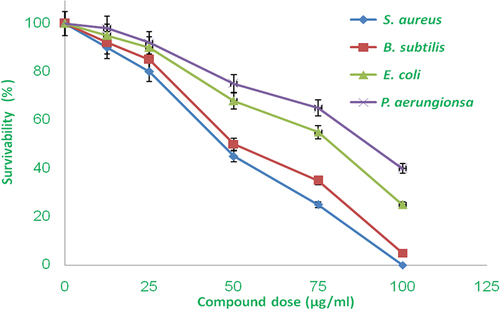
Table 3. Zone inhibition Table of PPY/CMC/MWCNTS BCs against pathogens.
Conclusion
The present study attempted to assess the efficiency of PPY/CMC/MWCNTS composite for the Sb3+ cationic sensor and their potential application for antimicrobial activities. GCE coated with PPY/CMC/MWCNTS composite fabricated the Sb3+ cationic sensor and nafion binder and the detailed investigation in reliable I-V approach was elaborately investigated. The assembled sensor showed appreciable results such as sensitivity, long-term stability, LDR, DL, response time, and reproducibility. Besides this, the sensor probe exhibited very good performance in the investigation of real sample for validation. Simultaneously, the antibacterial property of PPY/CMC/MWCNTS composite is estimated against four pathogenic bacteria, such as B. subtilis along with S. aureus of (G +ve) and P. aeruginosa along with E. coli for (G -ve) by using autoclave of agar media. The proposed technique is ideal for quality management laboratories along with scientific studies wherein time and economic systems are important.
Highlights
A fiber-type nanohybrid PPY/CMC/MWCNTS composite was prepared for electrochemical and antimicrobial applications.
To investigate the Sb3+ cationic sensor using the I-V method on a glassy carbon electrode coated with PPY/CMC/MWCNTS composite.
Antibacterial study results showed that PPY/CMC/MWCNTS composite was most effective against Bacillus bacteria, as well as Staphylococcus aureus, respectively.
A lower limit of detection is equal to 96.82 ± 4.84 pM at the signal-to-noise ratio at an SNR of 3 (signal-to-noise ratio).
Acknowledgements
This research work was funded by the Institutional Fund Project under grant no. (IFPHI-279-130-2020). Therefore, authors gratefully acknowledge technical and financial support from the Ministry of Education and King Abdulaziz University, DSR, Jeddah, Saudi Arabia.
Disclosure statement
No potential conflict of interest was reported by the authors.
Additional information
Funding
References
- Abol-Fotouh, D., B. Dorling, O. Zapata-Arteaga, X. R. Martinez, A. Gomez, J. S. Reparaz, A. Laromaine, A. Roig, and M. Campoy-Quiles. 2019. Farming thermoelectric paper. Energy & Environmental Science 12 (2):716–15. doi:10.1039/C8EE03112F.
- Alam, M. M., A. M. Asiri, M. T. Uddin, Inamuddin, M. A. Islam, M. R. Awual, M. M. Rahman, and I. Inamuddin. 2019. One-step wet-chemical synthesis of ternary ZnO/CuO/Co3O4 nanoparticles for sensitive and selective melamine sensor development. New Journal of Chemistry 43 (12):4849–58. doi:10.1039/C8NJ06361C.
- Alizadehgiashi, M., N. Khuu, A. Khabibullin, A. Henry, M. Tebbe, T. Suzuki, and E. Kumacheva. 2018. Nanocolloidal hydrofiber for heavy metal scavenging. ACS Nano 12 (8):8160–68. doi:10.1021/acsnano.8b03202.
- Anirudhan, T. S., J. R. Deepa and Binussreejayan B. 2018. Electrochemical sensing of cholesterol by molecularly imprinted polymer of silylated graphene oxide and chemically modified nanocellulose polymer. Materials Science & Engineering C 92:942–56. doi:10.1016/j.msec.2018.07.041.
- Bacakova, L., J. Pajorova, M. Tomkova, R. Matejka, A. Broz, J. Stepanovska, S. Prazak, A. Skogberg, S. Siljander, and P. Kallio. 2020. Applications of nanocellulose/nanocarbon composites: Focus on biotechnology and medicine. Nanomaterials 10 (2):1–32. doi:10.3390/nano10020196.
- Bustamante, J., D. Lennart, V. Marie, F. Bruce, and O. Sten. 1997. The semiconductor elements arsenic and indium induce apoptosis in rat thymocytes. Toxicology 118 (2–3):129–36. doi:10.1016/S0300-483X(96)03607-4.
- Buzid, A., P. E. Hayes, J. D. Glennon, and J. H. T. Luong. 2019. Captavidin as a regenerable biorecognition element on boron-doped diamond for biotin sensing. Analytica chimica acta 1059:42–48. doi:10.1016/j.aca.2019.01.058.
- Casiot, C., M. C. B. Alonso, O. F. X. Donard, M. Potin-Gautier, J. Boisson, and J. Boisson. 1998. Simultaneous speciation of arsenic, selenium, antimony and tellurium species in waters and soil extracts by capillary electrophoresis and UV detection. The Analyst 123 (12):2887–93. doi:10.1039/a805954c.
- Chen, W., H. Yu, S. Y. Lee, T. Wei, J. Li, and Z. Fan. 2018. Nanocellulose: A promising nanomaterial for advanced electrochemical energy storage. Chemical Society Reviews 47 (8): 2837−28. doi:10.1039/C7CS00790F.
- Costa, L. M., G. M. Olyveira, B. M. Cherian, A. L. Leao, S. F. Souza, and M. Ferreira. 2013. Bio-nanocomposites from electrospun PVA/pineapple nanofibers/Stryphnodendron adstringens bark extract for medical applications. Industrial Crops & Products 41:198. doi:10.1016/j.indcrop.2012.04.025.
- Dai, D., M. Fan, and P. Collins. 2013. Fabrication of nanocelluloses from hemp fibers and their application for the reinforcement of hemp fibers. Industrial Crops & Products 44:192. doi:10.1016/j.indcrop.2012.11.010.
- De Gregori, I., W. Quiroz, H. Pinochet, F. Pannier, and M. Potin-Gautier. 2007. Speciation analysis of antimony in marine biota by HPLC-(UV)-HG-AFS: Extraction procedures and stability of antimony species. Talanta 73 (3):458–65. doi:10.1016/j.talanta.2007.04.015.
- Dong, H., K. E. Strawhecker, J. F. Snyder, J. A. Orlicki, R. S. Reiner, and A. W. Rudie. 2012. Cellulose nanocrystals as a reinforcing material for electrospun poly(methyl methacrylate) fibers: Formation, properties and nanomechanical characterization. Carbohydrate Polymers 87 (4):2488–95. doi:10.1016/j.carbpol.2011.11.015.
- Durairaj, V., P. Li, Liljeström, T. N. Wester, J. Etula, I. Leppänen, Y. Ge, K. S. Kontturi, T. Tammelin, T. Laurila, et al. 2021. Functionalized nanocellulose/multiwalled carbon nanotube composites for electrochemical applications. Acs Applied Nano Materials 4 (6):5842–53. doi:10.1021/acsanm.1c00774.
- Durairaj, V., N. Wester, J. Etula, T. Laurila, J. Lehtonen, O. J. Rojas, N. Pahimanolis, and J. Koskinen. 2019. Multiwalled carbon nanotubes/nanofibrillar cellulose/nafion composite-modified tetrahedral amorphous carbon electrodes for selective dopamine detection. The Journal of Physical Chemistry C 123 (40):24826–36. doi:10.1021/acs.jpcc.9b05537.
- Du, X., Z. Zhang, W. Liu, and Y. Deng. 2017. Nanocellulose-based conductive materials and their emerging applications in energy devices - a review. Nano Energy 35:299–320. doi:10.1016/j.nanoen.2017.04.001.
- El-Sharjawy, A. A. M., and A. S. Amin. 2016. Use of cloud-point preconcentration for spectrophotometric determination of trace amounts of antimony in biological and environmental samples. Analytical Biochemistry 492:1–7. doi:10.1016/j.ab.2015.08.008.
- El-Shishtawy, R. M., H. A. Al-Ghamdi, M. M. Alam, Z. M. Al-Amshany, A. M. Asiri, and M. M. Rahman. 2018. Development of Cd2+sensor based on BZNA/Nafion/Glassy carbon electrode by electrochemical approach. Chemical Engineering Journal 352:225–31. doi:10.1016/j.cej.2018.07.034.
- Enger, J., A. Marunkov, N. Chekalin, and O. Axner. 1995. Direct detection of antimony in environmental and biological samples at trace concentrations by laser-induced fluorescence in graphite furnace with an intensified charge coupled device. Journal of Analytical Atomic Spectrometry 10 (8):539–49. doi:10.1039/ja9951000539.
- Espino-Perez, E., J. Bras, V. Ducruet, A. Guinault, A. Dufresne, and S. Domenek. 2013. Influence of chemical surface modification of cellulose nanowhiskers on thermal, mechanical, and barrier properties of poly(lactide) based bionanocomposites. European Polymer Journal 49 (10):144–3154. doi:10.1016/j.eurpolymj.2013.07.017.
- Fahma, F., N. Hori, T. Iwata, and A. Takemura. 2013. The morphology and properties of poly(methyl methacrylate)-cellulose nanocomposites prepared by immersion precipitation method. Journal of Applied Polymer Science 128:1563–68. doi:10.1002/app.38312.
- Favier, V., H. Chanzy, and J. Y. Cavaille. 1995. Polymer nanocomposites reinforced by cellulose whiskers. Macromolecules 28 (18):6365–67. doi:10.1021/ma00122a053.
- Ferenets, M., and A. Harlin. 2007. Chemical in situ polymerization of polypyrrole on poly(methyl methacrylate) substrate. Thin Solid Films 515 (13):5324–28. doi:10.1016/j.tsf.2007.01.008.
- Foldyna, J., V. Foldyna, and M. Zeleňák. 2016. Dispersion of carbon nanotubes for application in cement composites. Procedia Engineering 149:94–99. doi:10.1016/j.proeng.2016.06.643.
- Garcia de Rodriguez, N. L., W. Thielemans, and A. Dufresne. 2006. Sisal cellulose whiskers reinforced polyvinyl acetate nanocomposites. Cellulose 13 (3):261–70. doi:10.1007/s10570-005-9039-7.
- Gousse, C., H. Chanzy, E. Cerrada, and E. Fleury. 2004. Surface silylation of cellulose microfibrils: Preparation and rheological properties.Polymer. Polymer 45 (5):1569–75. doi:10.1016/j.polymer.2003.12.028.
- Gousse, C., H. Chanzy, G. Excoffier, L. Soubeyrand, and E. Fleury. 2002. Stable suspensions of partially silylated cellulose whiskers dispersed in organic solvents. Polymer 43 (9):2645. doi:10.1016/S0032-3861(02)00051-4.
- Grunert, M., and W. T. Winter. 2002. Nanocomposites of cellulose acetate butyrate reinforced with cellulose nanocrystals.J. Journal of Polymers and the Environment 10 (1/2):27–30. doi:10.1023/A:1021065905986.
- Hansen, H. R., and S. A. Pergantis. 2006. Detection of antimony species in citrus juices and drinking water stored in PET containers. Journal of Analytical Atomic Spectrometry 21 (8):731–33. doi:10.1039/B606367E.
- Hussein, M. A., M. M. Alam, N. A. Alenazi, K. A. Alamry, A. Asiri, and M. M. Mahman. 2018. Nanocomposite based functionalized Polyethersulfone and conjugatedternary ZnYCdO nanomaterials for the fabrication of selective Cd2+ sensor probe. Journal of Polymer Research 25 (12):262. doi:10.1007/s10965-018-1643-y.
- Hussein, M. A., M. M. Alam, A. M. Asiri, Z. M. Al-Amshany, K. S. Hajeeassa, and M. M. Rahman. 2019. Ultrasonic-assisted fabrication of polyvinyl chloride/mixed graphene-carbon nanotube nanocomposites as a selective Ag+ ionic sensor. Journal of Composite Materials 1 (16):14. doi:10.1177/0021998318825293.
- Ifuku, S., M. Nogi, K. Abe, K. Handa, F. Nakatsubo, and H. Yano. 2007. Surface modification of bacterial cellulose nanofibers for property enhancement of optically transparent composites: Dependence on acetyl-group DS. Biomacromolecules 8 (6):1973. doi:10.1021/bm070113b.
- Jackson, J. K., K. Letchford, B. Z. Wasserman, L. Ye, W. Y. Hamad, and H. M. Burt. 2011. The use of nanocrystalline cellulose for the binding and controlled release of drugs. International Journal of Nanomedicine 6:321. doi:10.2147/IJN.S16749.
- Janaki, V., K. Vijayaraghavan, B. T. Oh, A. K. Ramasamy, and S. K. Kannan. 2013. Synthesis, characterization and application of cellulose/polyaniline nanocomposite for the treatment of simulated textile effluent. Cellulose 20 (3):1153–66. doi:10.1007/s10570-013-9910-x.
- Johar, N., I. A. Ahmad, and A. Dufresne. 2012. Extraction, preparation and characterization of cellulose fibres and nanocrystals from rice husk. Industrial Crops & Products 37 (1):93. doi:10.1016/j.indcrop.2011.12.016.
- Kanematsu, N., M. Hara, and T. Kada. 1980. Rec assay and mutagenicity studies on metal compounds. Mutation Research/Genetic Toxicology 77 (2):109–16. doi:10.1016/0165-1218(80)90127-5.
- Karim, M. R., M. M. Alam, M. O. Aijaz, A. M. Asiri, M. A. Dar, and M. M. Rahman. 2019. Fabrication of 1,4-dioxane sensor based on microwave assisted PAni-SiO2 nanocomposites. Talanta 193:64–6966. doi:10.1016/j.talanta.2018.09.100.
- Khan, A. A. P. 2020. Electrocatalytic behavior and determination of amitriptyline drug with MWCNT@Celllulose composite modified glassy carbon electrode. Materials 13 (7):1708. doi:10.3390/ma13071708.
- Khan, A. A. P., A. Khan, M. M. Alam, A. M. Asiri, J. Uddin, and M. M. Rahman. 2019. SDBS-functionalized MWCNT/poly(o-toluidine) nanowires modified glassy carbon electrode as a selective sensing platform for Ce3+ in real samples. Journal of Molecular Liquids 279:392–99. doi:10.1016/j.molliq.2019.01.159.
- Khan, A. A. P., A. Khan, M. M. Alam, M. Oves, A. M. Asiri, and M. M. Rahman, Inamuddin I. 2018. Chemical sensing platform for the Zn+2 ions based on poly (o-anisidine-co-methyl anthranilate) copolymer composites and their environmental remediation in real samples. Environmental Science & Pollution Research 25 (28):27899–911. doi:10.1007/s11356-018-2819-z.
- Khan, A. A. P., A. Khan, M. M. Rahman, and A. M. Asiri. 2016. Conventional surfactant-doped poly (o-anisidine)/GO nanocomposites for benzaldehyde chemical sensor development. Journal of Sol-Gel Science and Technology 77 (2):361–70. doi:10.1007/s10971-015-3862-6.
- Khan, A. A. P., A. Khan, M. M. Rahman, A. M. Asiri, and M. Oves. 2017. Sensor development of 1, 2 Dichlorobenzene based on polypyrrole/Cu-doped ZnO (PPY/CZO) nanocomposite embedded silver electrode and their antimicrobial studies. International Journal of Biological Macromolecules 98:256–67. doi:10.1016/j.ijbiomac.2017.02.005.
- Koga, H., T. Saito, T. Kitaoka, M. Nogi, K. Suganuma, and A. Isogai. 2013. Transparent, conductive, and printable composites consisting of TEMPO-Oxidized nanocellulose and carbon nanotube. Biomacromolecules 14 (4):1160–65. doi:10.1021/bm400075f.
- Kuroda, K., G. Endo, A. Okamato, Y. S. Yoo, and S. Horiguchi. 1991. Genotoxicity of beryllium, gallium and antimony in short-term assays. Mutation Research Letters 264 (4):163–70. doi:10.1016/0165-7992(91)90072-C.
- Liang, Y., J. Liu, L. Wang, Y. Wan, J. Shen, and Q. Bai. 2019. Metal affinity-carboxymethyl cellulose functionalized magnetic graphene composite for highly selective isolation of histidine-rich proteins. Talanta 195:381–89. doi:10.1016/j.talanta.2018.11.074.
- Liu, X., H. Shen, S. Song, W. Chen, and Z. Zhang. 2017. Accelerated biomineralization of graphene oxide – incorporated cellulose acetate nanofibrous scaffolds for mesenchymal stem cell osteogenesis. Colloids and Surfaces, B, Biointerfaces 159:251–58. doi:10.1016/j.colsurfb.2017.07.078.
- Lonard, A., and G. B. Gerber. 1996. Mutagenicity, carcinogenicity and teratogenicity of antimony compounds. Mutation Research/Reviews in Genetic Toxicology 366 (1):1–8. doi:10.1016/S0165-1110(96)90003-2.
- Nam, S. H., C. Y. Yang, and Y. J. An. 2009. Effects of antimony on aquatic organisms (larva and embryo of oryzias latipes, moina macrocopa, simocephalus mixtus, and pseudokirchneriella subcapitata). Chemosphere 75 (7):889–93. doi:10.1016/j.chemosphere.2009.01.048.
- Nguyen, H. K., J. Bae, J. Hur, S. J. Park, M. S. Park, and I. T. Kim. 2019. Tailoring of aqueous-based carbon nanotube–nanocellulose films as self-standing flexible anodes for lithium-ion storage. Nanomaterials 9 (4):655. doi:10.3390/nano9040655.
- Nikula, K. J., M. G. Snipes, E. B. Barr, W. C. Griffith, R. F. Henderson, and J. L. Mauderly. 1995. Comparative pulmonary toxicities and carcinogenicities of chronically inhaled diesel exhaust and carbon black in F344 rats. Fundamental and Applied Toxicology 25 (1):80–94. doi:10.1006/faat.1995.1042.
- Nishino, T. 2004. Natural fiber sources, green composites: Polymer composites and the environment. ed, Baillie, c. Cambridge England: Woodhead Publishing Ltd.
- Ortolani, T. S., T. S. Pereira, M. H. M. T. Assumpcą, F. C. Vicentini, G. Gabriel de Oliveira, and B. C. Janegitz. 2019. Electrochemical sensing of purines guanine and adenine using single walled carbon nanohorns and nanocellulose. Electrochimica acta 298:893–900. doi:10.1016/j.electacta.2018.12.114.
- Pinkert, A., K. N. Marsh, S. Pang, and M. P. Staiger. 2009. Ionic liquids and their interaction with cellulose. Chemical Reviews 109 (12):6712–28. doi:10.1021/cr9001947.
- Rahman, M. M., M. M. Alam, and A. M. Asiri. 2019. Development of an efficient phenolic sensor based on facile Ag2O/Sb2O3 nanoparticles for environmental safety. Nanoscale Advances 1 (2):696–705. doi:10.1039/C8NA00034D.
- Rahman, M. M., M. M. Alam, A. M. Asiri, and M. A. Islam. 2018. 3,4-Diaminotoluene sensor development based on hydrothermally prepared MnCoxOy nanoparticles. Talanta 176:17–25. doi:10.1016/j.talanta.2017.07.093.
- Rahman, M. M., N. A. Alenazi, M. A. Hussein, M. M. Alam, K. A. Alamry, and A. M. Asiri. 2018a. Hybride ZnCdCrO embedded aminated polyethersulfone nanocomposites for the development of Hg2+ ionic sensor. Materials Research Express 5 (6):065019. doi:10.1088/2053-1591/aac681.
- Rahman, M. M., N. A. Alenazi, M. A. Hussein, M. M. Alam, K. A. Alamry, and A. M. Asiri. 2018b. Nanocomposites-based nitrated polyethersulfone and doped ZnYNiO for selective As3+ sensor application. Advances in Polymer Technology 37 (8):1–12. doi:10.1002/adv.22153.
- Rahman, M. M., T. Sheikh, R. M. AEl-Shishtawy, M. N. Arshad, F. A. Al-Zahrani, and A. M. Asiri. 2018. Fabrication of Sb 3+++ sensor based on 1,1′-(-(naphthalene-2,3++-diylbis(azanylylidene))bis(methanylylidene))bis(naphthalen-2-ol)/nafion/glassy carbon electrode assembly by electrochemical approach. RSC Advances 8 (35):19754–64. doi:10.1039/C8RA01827H.
- Rozenbaum, W., D. Dormont, B. Spire, E. Vilmer, M. Gentilini, C. Gaiscelli, L. Montagnier, F. Barre-Sinoussi, and C. Cherman. 1985. Antimoniotungstate (HPA 23) treatment of three patients with AIDS and one with prodrome. The Lancet 1 (8426):450–51. doi:10.1016/S0140-6736(85)91162-6.
- Shalauddin, M., S. Akhter, W. J. Basirun, S. Bagheri, N. S. Anuar, and M. R. Johan. 2019. Hybrid nanocellulose/f-MWCNTs nanocomposite for the electrochemical sensing of diclofenac sodium in pharmaceutical drugs and biological fluids. Electrochimica acta 304:323–33. doi:10.1016/j.electacta.2019.03.003.
- Siljander, S., P. Keinanen, A. Raty, K. R. Ramakrishnan, S. Tuukkanen, V. Kunnari, A. Harlin, J. Vuorinen, and M. Kanerva. 2018. Effect of surfactant type and sonication energy on the electrical conductivity properties of nanocellulose-CNT nanocomposite films. International Journal of Molecular Sciences 19 (6):1819. doi:10.3390/ijms19061819.
- Silverio, H. A., W. P. Flauzino Neto, N. O. Dantas, and D. Pasquini. 2013. Extraction and characterization of cellulose nanocrystals from corncob for application as reinforcing agent in nanocomposites. Industrial Crops & Products 44:427. doi:10.1016/j.indcrop.2012.10.014.
- Su, J., S. Zhong, X. Li, and H. Zou. 2014. Determination of trace antimony by square-wave adsorptive cathodic stripping voltammetry at an ex situ prepared bismuth film electrode. Journal of the Electrochemical Society 161 (9):H512–16. doi:10.1149/2.0521409jes.
- Thakur, V. K., A. S. Singh, and B. N. Misra. 2011. Graft copolymerization of methyl methacrylate onto cellulosic biofibers. Journal of Applied Polymer Science 122 (1):532–44. doi:10.1002/app.34094.
- Tongdesoontorn, W., L. J. Mauer, S. Wongruong, P. Sriburi, and P. Rachtanapun. 2011. Effect of carboxymethyl cellulose concentration on physical properties of biodegradable cassava starch-based films. Chemistry Central Journal 5 (1):6. doi:10.1186/1752-153X-5-6.
- Vetrivel, S., M. S. A. Saraswathi, D. Rana, and A. Nagendran. 2018. Fabrication of cellulose acetate nanocomposite membranes using 2D layered nanomaterials for macromolecular separation. International Journal of Biological Macromolecules 107:1607–12. doi:10.1016/j.ijbiomac.2017.10.027.
- Vinhal, J. O., A. D. Gonçalves, G. F. B. Cruz, and R. J. Cassella. 2016. Speciation of inorganic antimony (III & V) employing polyurethane foam loaded with bromopyrogallol red.Talanta. Talanta 150:539–45. doi:10.1016/j.talanta.2015.12.080.
- Vitz, J., T. Erdmenger, C. Haensch, and U. S. Schubert. 2009. Extended dissolution studies of cellulose in imidazolium based ionic liquids. Green Chemistry 11 (3):417–24. doi:10.1039/b818061j.
- Wang, H., S. K. Biswas, S. Zhu, Y. Lu, Y. Yue, J. Han, X. Xu, Q. Wu, and H. Xiao. 2020. Self-healable electro-conductive hydrofibers based on core-shell structured nanocellulose/carbon nanotubes hybrids for use as flexible supercapacitors. Nanomaterials 10 (1):1–21. doi:10.3390/nano10010112.
- Yuan, H., Y. Nishiyama, M. Wada, and S. Kuga. 2006. Surface acylation of cellulose whiskers by drying aqueous emulsion. Biomacromolecules 7 (3):696. doi:10.1021/bm050828j.
- Yu, J., P. Dai, S. Ge, Y. Zhu, L. Zhang, and X. Cheng. 2009. Catalytic dynamic spectrofluorimetry determination of trace antimony using new type arsenoxylphenylazo rhodamine. Spectrochimica Acta, Part A, Molecular and Biomolecular Spectroscopy 72 (1):17–21. doi:10.1016/j.saa.2008.06.047.
- Yu, Z., C. Hu, A. B. Dichiara, W. Jiang, and J. Gu. 2020. Cellulose nanofibril/carbon nanomaterial hybrid aerofibers for adsorption removal of cationic and anionic organic dyes. Nanomaterials 10 (1):1–19. doi:10.3390/nano10010169.
- Zanetti, M., G. Camino, D. Canavese, A. B. Morgan, F. J. Lamelas, and C. A. Wilkie. 2002. Fire retardant halogen−Antimony−Clay synergism in polypropylene layered silicate nanocomposites. Chemistry of Materials 14 (1):189–93. doi:10.1021/cm011124t.
- Zavadskii, A. E. 2004. X-ray diffraction method of determining the degree of crystallinity of cellulose materials of different anisotropy. Fibre Chemistry 36 (6):425–30. doi:10.1007/s10692-005-0031-7.
- Zhao, D., Y. Zhu, W. Cheng, W. Chen, Y. Wu, and H. Yu. 2020. Cellulose-based flexible functional materials for emerging intelligent electronics. Advanced Materials 33 (28):2000619. doi:10.1002/adma.202000619.
- Zhu, C., P. Liu, and A. P. Mathew. 2017. Self-assembled TEMPO cellulose nanofibers: Graphene oxide-based biohybrids for water purification. ACS Applied Materials & Interfaces 9 (24):21048–58. doi:10.1021/acsami.7b06358.