ABSTRACT
In this study, copper nanoparticles were synthesized from Trapa natans L. shells and loaded within polyamide-12 for use as an adsorbent. The synthesized nanocomposite was used for the first time to remove toxic methyl orange and brilliant green dye molecules from synthetic aqueous solutions. In order to characterize the plant-based nanocomposite, its functional groups, surface texture, elemental composition, structure, surface area, and crystallinity were determined. Adsorption efficiency was found to be affected by factors like pH (2–10), time (5–240 min.), and temperature (288–308 K). It was at pH 6 that the adsorbent reached the point of zero charge. During the adsorption process, electrostatic attraction played an influential role. Adsorption isotherms and kinetics data were well-fitted by Langmuir isotherms and pseudo-second-order models, respectively. A maximum adsorption capacity of 166.60 mg/gm was calculated for 93.90% methyl orange removal and 55.24 mg/gm for 97.30% brilliant green removal. The adsorption process was endothermic, spontaneous, and physical. The rapid desorption of dye molecules confirms the potential, and practicality of the nanocomposite as a promising adsorbent for decolorizing colored wastewater on an industrial scale.
摘要
在本研究中,从Trapa natans L.壳中合成了铜纳米颗粒,并将其负载在聚酰胺-12中用作吸附剂. 合成的纳米复合材料首次用于去除合成水溶液中有毒的甲基橙和亮绿色染料分子. 为了表征植物基纳米复合材料,测定了其官能团、表面结构、元素组成、结构、表面积和结晶度. 发现吸附效率受pH(2-10)、时间(5-240分钟)和温度(288-308K)等因素的影响. 吸附剂在pH值为6时达到零电荷点. 在吸附过程中,静电吸引起了重要作用. 吸附等温线和动力学数据分别用Langmuir等温线和拟二阶模型很好地拟合. 对于93.90%的甲基橙去除率和97.30%的亮绿色去除率,计算出的最大吸附量分别为166.60 mg/gm和55.24 mg/gm. 吸附过程是吸热的、自发的和物理的. 染料分子的快速解吸证实了纳米复合材料作为一种有前途的吸附剂在工业规模上脱色有色废水的潜力和实用性.
Introduction
There is a continuous increase in dye pollution of water. As reported by the world health organization and the environmental pollution agency, their toxic effects on the environment are concerning. Since dye toxicity is expensive and time-consuming, most industries don’t pay attention to it. Methyl orange (C14H14N3NaO3S) is a vibrant orange-yellow azo dye used primarily as a pH indicator to titrate inorganic acids and alkalis. As a result of its industrial applications, methyl orange (MO) is released into the water environment through effluent stream contributing to the depletion of the water environment. A study has indicated that about 20% of azo dyes disposed of by textile factories are discharged directly into the environment as a waste product (Robinson et al. Citation2001). Brilliant green (C27H34N2O4S) dye is a cationic, triphenylmethane-based organic dye with no odor. BG is illegally used not only in aquaculture to treat fish diseases but also in pulp and paper production to color paper towels used in hospitals, factories, and other locations to dry hands after washing (Oplatowska et al. Citation2011). In humans, its fatal dose is 50–500 mg/kg, which makes it a highly toxic dye (Pohanish Citation2012). In order to treat dye-containing wastewater, many physical-chemical techniques have been implemented such as photodegradation (Siong et al. Citation2019), photo-Fenton (Muhammad et al. Citation2020), photocatalysis (Ikram et al. Citation2021), etc. In addition to their advantages, these technologies have some drawbacks as well. Among them, adsorption has long been the most popular and effective method for removing aqueous pollutants (Uddin Citation2017; Uddin and Rahaman Citation2017) and treating dyes containing wastewater for many years (Husein et al. Citation2021; Uddin et al. Citation2021). For this technique, an adsorbent material that is porous and efficient is needed. Many water treatment processes already use biomass (Rao, Ikram, and Kashif Uddin Citation2015; Khatoon, Uddin, and Rao Citation2018). Scientists who work on the protection of the environment and water treatment are interested in the search for new adsorbents. In order to reduce the toxic effects of water, nanotechnology and green chemistry can be combined with safe biological materials, such as plants, to fabricate nontoxic nanoparticles (Jadoun et al. Citation2021). A green synthesis process uses plant extracts that are cheap, widely available, and safe for humans. The biosynthesis of nanoparticles (NPs) by using a green approach has gained considerable attention due to the fact that plants and their wastes are readily available, are a cheaper alternative, show high removal rates, and can be reused (Giri et al. Citation2020). The synthesis of NPs is therefore based on natural resources, such as plant extracts, fungi, and bacteria. In nanomaterials, carbon is sourced primarily from plant extracts (Saddik et al. Citation2020). In recent reviews conducted by Qamar and Ahmad (Qamar and Nazeer Ahmad Citation2021) and Yang et al (Yang et al. Citation2022). concluded that two dominant approaches are used to synthesize nanoparticles (the bottom-up approach and top-down approach). ZnFe2O4 nanoparticles synthesized by using plants and their properties have also been recently reviewed for their use as catalysts, biomedical materials, and biomedical agents, among others (Nguyen et al. Citation2023). The preparation methods also have some limitations, such as top-down method requires wide size distribution and varies particle size, whereas bottom-up method requires chemical purification and cannot be produced at large scales. Among metal oxide nanoparticles, copper oxide nanoparticles have gained considerable attention for their many characteristics and applications. A unique antimicrobial activity of copper nanoparticles have been demonstrated (Thandapani et al. Citation2023). According to a recent review, there have been many attempts to synthesize copper oxide nanoparticles using different plant extracts (Chakraborty et al. Citation2022). The biosynthesis of Cu and Cu2O has been done by several plants (Al-Hakkani et al. Citation2021). Studies have demonstrated that metallic nanoparticles can effectively target cancer cells and manage them (Saddik et al. Citation2021, Citation2022).
It is relatively easy to mix copper oxide nanoparticles with polymers, and their chemical and physical properties are relatively stable (Allaker Citation2012). Developing polymer nanocomposite by incorporating organic or inorganic nanoparticles into polymeric matrices is a rapidly emerging research field (Wani et al. Citation2022). Adding nanoparticles to polymers allows modification of physical properties and implementation of new features (Hanemann and Vinga Szabó Citation2010). The use of polymer nanocomposites in many applications has been widely accepted (Alarifi, Prasad, and Kashif Uddin Citation2020). Polyamide-12 (PA-12) is a semi-crystalline thermoplastic polymer and is considered an efficacious material due to its ideal properties and multiple applications (Aldahash et al. Citation2022). A major characteristic of PA-12 is its inhomogeneous particle size, high specific surface area, high lamellar crystal size, and low crystallinity (Simha et al. Citation2021). Polyamide has attracted scientists to the development of new materials with an intriguing perspective and a large potential in many industrial fields, including wastewater treatment (Basaleh, Al-Malack, and Saleh Citation2019; Saleh, Tuzen, and Sarı Citation2018). In a recent critical review, polyamide-based desalination membranes were highlighted as being capable of separating toxic organic compounds from water (Shin et al. Citation2022). Currently, PA-12 is a relatively new material in the field of environmental science. A PA-12/cement nanocomposite has been synthesized and used to remove four different dye molecules in our latest article (Aldahash et al. Citation2022). Biosorbents, which are derived from plants, are among the most widely used low-cost materials for removing pollutants from water (Rao, Rehman, and Kashifuddin Citation2012; Uddin and Nasar Citation2020). The water chestnut (Trapa natans L.) is an aquatic plant that is also known as the horn chestnut, the Jesuit nut, and the water caltrop (Borojevic Citation2009). Various species of Trapa natans L. are grown in confined areas of water in the countries of China, India, and Thailand for human consumption (Borojevic Citation2009). Table S1 shows the composition of Trapa natans L. shells (Sverguzova et al. Citation2020). Recently, Qaiyum et al. have used alkali-modified shells of Trapa natans L. to remove malachite green from water (Qaiyum et al. Citation2022). Trapa natans L. peels treated with citric acid were used to remove brilliant green dye using a biosorption procedure (Hussain, Hussain et al. Citation2021). In Khan et al.‘s study, it was used to remove rhodamine B dye from an aqueous solution through biosorption (Khan, Nazir, and Khan Citation2013). Trapa natans L. husk activated carbon was tested for its ability to remove norfloxacin drug from aqueous solutions at a low cost (Xie et al. Citation2011). This study aims to produce eco-friendly copper nanoparticles by green synthesis from Trapa natans L. shells, and encapsulate them within PA-12 material for use as a novel adsorbent. A feature of this method is that nanoparticles can be synthesized without much equipment and at a low cost from plant extracts. The use of plant extracts for nanoparticle synthesis has advantages over other biological synthesis methods. The prepared material is tested for the removal of methyl orange and brilliant green dye molecules from aqueous solutions for industrial wastewater treatment.
Materials and methods
Description of methods and methods are available as supplementary material.
Results and discussion
Characterization
Fourier Transform Infrared (FTIR)
The FTIR spectrums of PA-12/CuONPs, MO and BG adsorbed PA-12/CuONPs are depicted in . As observed in PA-12/CuONPs, the broad peak at 3411 cm−1 was allocated to – OH stretching vibration. The peaks at 2,922.65 and 2852.12 cm−1 indicate asymmetric and symmetric stretching vibration of C – H (Rao and Kashifuddin Citation2014). The peaks at 2374.31 and 2343.86 cm−1 represent the adsorbed atmospheric carbon dioxide gas molecule. The peak at 1712.75 cm−1 is due to the stretching vibration of carbonyl (C=O) and may be the result of carboxylic acids or their esters (Feng Ting et al. Citation2007). Observation of the peak at 1639 cm−1 indicates stretching vibration of the Cu – O bond of CuONPs (Raul et al. Citation2014). Trapa natans L. exhibits characteristic lignin components that exhibit nonsymmetric stretching vibrations of C=O and COO near 1500 cm−1 (Ceylan and Kilic Pekgozulu Citation2019). Narrow peaks at 1561, 1553.01 and 1461.58 cm−1 were ascribed to amine (amide II band) stretching vibrations of N – H and C – N, respectively (Yin, Zhu, and Deng Citation2016). The peaks at 1434.55, 1418.27 and 1383.73 cm−1 are caused by the interaction of carboxylic acid functional groups with the O−H bonds. The peaks at 1221 and 1118.34 cm−1 are attributed to the stretching of the C – O bond due to the amorphous/crystalline structure of Cu2+ (Uddin, Ali Khan Rao, and Chandra Mouli Citation2018). The peak at 873 cm−1 can be correlated to stretching of and Cu-O-H while the various peaks appeared in the range from 779 to 425 cm−1 indicating the formation of CuO-NPs (Bialy et al. Citation2020). A small shift, weakening, and movement of the peak intensities indicated the interface between dye molecules and PA-12/CuONPs. As a result of MO adsorption, new absorption bands of wavenumbers ~ 3744, 2339, 2064, 1630, 1419, 1365 and 1270 cm−1 appeared, while after BG adsorption, new absorption bands of wavenumbers ~ 3778, 3402, 2064, 1465, 1547, 1187, 1088 and 600 cm−1 appeared. Figure S1 shows individual FTIR spectra of Trapa natans L., PA-12, CuONPs, MO, and BG. Table S2 specify the vibrational bands that appeared after the adsorption in each case.
Brunauer-Emmett-Teller (BET) analysis
As shown in the , the N2 adsorption-desorption isotherm of PA-12/CuONPs belongs to type III according to IUPAC having H3 type hysteresis loop, indicating a typical mesoporous structure in the adsorbent. The H3 loop is attributed to wedge-shaped (tapering a thin edge at one end) pores formed by the loose collection of flaky particles composed of nonrigid aggregates of plate-like particles (Longfei et al. Citation2020). As type III indicates narrower mesopores, wider micropores, and a wider pore size distribution, which promotes sharp adsorption capacity (Mukhtar et al. Citation2020). Using BET, 34.309 m2/gm of surface area is calculated. As a result of the BJH method adsorption, the surface area is 26.3936 m2/gm, the pore volume is 0.299852 cc/gm, and the pore radius is 1.6919 nm, whereas as a result of the BJH method desorption, the surface area is 39.3279 m2/gm, the pore volume is 0.308586 cc/gm, and the pore radius is 8.63211 nm. As PA-12/CuONPs have pore widths not exceeding about 2 nm, they can be characterized as microporous solids. A recent study by Liu et al. showed that polymeric adsorbents with smaller pores could adsorb phenol and anionic surfactants more effectively (Liu et al. Citation2021).
High Resolution Transmission Electron Microscopy (HR-TEM)
HR-TEM analyses revealed a branched, stable structure comprised of segments interconnected by crosslinks and forming network aggregates. HR-TEM images at different magnifications () show nanoparticles with small, homogeneous and uniform particles, round surfaces, chains, and network structures. Also, PA-12 is encrusted with copper nanoparticles that have a darker color. Based on Image J software, a 23.21 nm average size was calculated.
Scanning Electron Microscopy (SEM) and energy dispersive X-ray (EDS) analysis
As shown in the SEM images (), the PA-12/CuONPs nanocomposite exhibits a rice-grain-shaped morphology with a spontaneous, narrow particle size distribution. There are agglomerated semi-spherical nano-sized particles, which are relatively homogenous. In the presence of MO and BG, the surface of PA/12-CuNPs underwent considerable changes (). MO and BG ions surrounded and accumulated on the surface after adsorption. As shown by the bright patches on the dye-loaded adsorbent’s surface, this facilitates the binding of dye molecules to PA-12/CuONPs. shows the elemental content and their abundances of PA-12/CuONPs, before and after adsorption by elemental mapping and EDS, respectively. According to the analysis, PA-12/CuONPs contains 80.71% copper, 7.82% carbon, 6.47% oxygen, 2.81% sulfur, and 0.75% sodium. In the element analysis of dye adsorbed PA-12/CuONPs, the rise in the C element indicates successful adsorption of MO and BG onto PA-12/CuONPs. In addition, the change in their respective weight confirms dye adsorption, which is also evident in the elemental mapping of PA-12/CuONPs where the detected elements are distributed on the surface.
Figure 4. SEM images of PA-12/CuONPs (a-b), MO adsorbed PA-12/CuONPs (c), BG adsorbed PA-12/CuONPs (d).
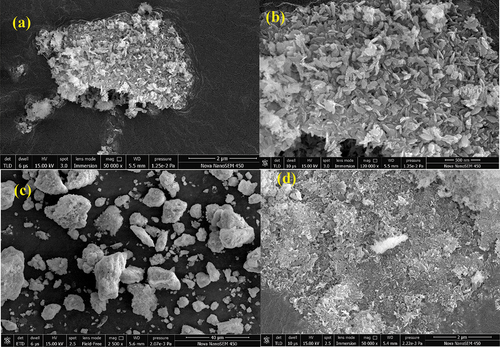
X-Ray diffraction analysis (XRD)
According to the XRD pattern for PA-12/CuONPs, there are intense diffraction peaks () indicating that PA-12 is loaded due to the occurrence of intense diffraction bands at 2θ values and crystal planes at ~ 35.55° and ~ 38.74° in accordance with the JCPDS (01-089-5895). The XRD also revealed a monoclinic crystal system of the adsorbent when the polyamide was intercalated. On the other hand, CuO peaks have been observed at ~ 32.43°, ~35.46°, ~38.59°, ~48.53°, ~57.97°, ~61.42° and ~ 66.02° (). Data from the JCPDS (01-089-5898) suggested the volume of the cell to be 81.16 pm. In PA-12, there are two distinct peaks at the 2θ values of ~ 20.9° and ~ 22.0°Corresponding to the unstable and intermediate phases of α and γ, respectively (Sommereyns et al. Citation2021) (). With the Miller indices, XRD peaks are identified (). In addition, the crystallite sizes of CuONPs before and after synthesis were calculated using the Scherrer equation and found to be 53.9696 nm and 13.32696 nm, respectively.
Thermo Gravimetric Analysis (TGA)
The TGA thermogram () of PA-12/CuONPs shows that weight percentage decreases with temperature, indicating that mass continuously changes due to thermal treatment. TGA curve showed that water was removed from steps 1–2 resulting in a 20% weight loss. Prior to melting temperature for step-1, no weight loss was observed, indicating that it is anhydrous. Starting at 420°C in steps-2–3, the melting peak is followed by a degradation peak indicating significant weight loss. Approximately 98% of it is degraded up to the end of a reaction. When the temperature is high, volatiles are lost, causing weight loss.
UV-Visible (UV-Vis) spectroscopy
Fig. S2 shows UV-vis of CuONPs and PA-12/CuONPs. In this study, copper sulfate solute (CuSO4.5 H2O) was reduced into copper oxide nanoparticles using UV-VIS spectroscopy (Perkin Elmer, USA). Upon synthesis of copper oxide nanoparticles and subsequent addition of Polyamide 12, the color of the solution gradually changed and was observed through Surface Plasmon Resonance (Fig. S2). A 3 ml sample of copper oxide nanoparticles and PA-12/CuONPs were injected into the cuvette and absorbance was measured between 400 and 600 nm, following the method of Sarwar et al (Sarwar et al. Citation2021). It was observed that the maximum absorption of copper nanoparticles before addition of PA-12 occurred at 500 nm, indicating the change in color from blue to green, whereas after addition of PA-12 it occurred at 590 nm, forming a dark brown paste.
Adsorption studies
Effect of pH and point of zero charge
The pH plays a significant role in the adsorption study because it affects the surface binding sites of the adsorbent due to its effect on the ionizing power of dye molecules in the aqueous mixture. shows the effects of different pH values (2–10) on the adsorption of MO and BG on PA-12/CuONPs. For both dyes, the trend was the same and at pH 6, MO and BG adsorb best, but after that there is a gradual decline in qe values. In previous studies, pH 6 was also identified as the optimum pH for MO (Baig, Kashif Uddin, and Gondal Citation2020) and BG (Aldahash et al. Citation2022) adsorption. To understand how pH affects sorption, the point of zero surface (pHpzc) is an important parameter. pHpzc refers to the point where (pHi–pHf) becomes zero. Above pHpzc the adsorbent has a negatively charged surface, while below this point, the adsorbent has a positively charged surface (Uddin and Nasar Citation2020). A pH6 PZC value was obtained for PA-12/CuONPs (), thus, the surface of PA-12/CuONPs is positively and negatively charged below and above this pH, respectively. When pH is low, MO molecules generate a monovalent ion on nitrogen, which causes electrostatic repulsion with PA-12/CuONPs active sites (at < pH 6), thus decreasing MO adsorption. BG is a cationic dye and dissociate H+ ion which causes electrostatic repulsion at PA-12/CuONPs active sites (at < pH 6). At pH > 6, OH¯ ions associated with the PA-12/CuONPs surface caused precipitation and competition between MO and BG with negative adsorption sites, which hindered and decreased MO and BG adsorption at PA-12/CuONPs surfaces. It can therefore be assumed that MO and BG were adsorbing on PA-12/CuONPs through electrostatic interaction mechanism.
Adsorption isotherm
By using the isotherm equations, the experimental data of MO and BG adsorption onto PA-12/CuONPs were fitted into non-linear plots based on Langmuir, Freundlich, Temkin, and Dubinin-Radushkevich (D-R) isotherm models (). Langmuir and Freundlich models both perform equally well in describing the adsorption process of MO and BG onto PA-12/CuONPs based on their generated correlation coefficients (R2) (). These results indicate that both the dye molecules are adsorbed initially as monolayers, and later as multilayers onto PA-12/CuONPs surfaces. RL is less than 1 indicating a favorable adsorption process. A value of n of 1.04 is obtained for MO, and a value of 1.397 is acquired for BG. Based on the outcome that b, KF, RL and n are low and positive values, it is clear that dye molecules interact favorably with PA-12/CuONPs with high affinity. In comparison, the PA-12/CuONPs obtained maximum adsorption capacity (qm) values are higher than previously reported qm values for various MO and BG adsorbents. It was observed that MO exhibited an excellent qm of 166.60 mg/gm, which was greater than CO3O4 nanoparticles (46.08 mg/gm) (Uddin and Baig Citation2019), and ZnO supported red mud (102.64 mg/gm) (Qiao et al. Citation2022). A reasonable qm of 55.24 mg/gm was obtained for BG, which is superior to Peganum harmala-L-seeds (35.97 mg/gm) (Agarwal et al. Citation2017), and modified areca nut husk (18.21 mg/gm) (Sukla Baidya and Kumar Citation2021). This result demonstrates the feasibility and practicality of using PA-12/CuONPs to remove MO and BG from industrial wastewater. In order to confirm the nature of the adsorption process, D – R isotherm parameters were calculated. Adsorption is considered physical if adsorption energy (E) is less than 8 kJ/mol, and chemical if it is equal to or greater than 8 kJ/mol. For both MO and BG, the calculated E values were less than 8 kJ/mol, which supports the physical adsorption of MO and BG on PA-12/CuONPs via ion exchange. In addition, the Temkin isotherm model also BG adsorption with a reasonable R2. When the heat of sorption (B) value is less than 20–40 kJ/mol, physical adsorption occurs, and when it is 80–240 kJ/mol, chemical adsorption occurs. Adsorption involves both physical and chemical adsorption if the B value is between 20–40 kJ/mol (Uddin and Baig Citation2019). In terms of B, the value comes out to be 0.277 kJ/mol for MO and 0.296 kJ/mol for BG, again suggesting a physical adsorption process, also supported by the D-R isotherm model.
Table 1. Adsorption isotherm parameters of dyes’ adsorption onto PA-12/CuONPs.
Adsorption thermodynamics
Using vant’s Hoff plots, the thermodynamic parameters for MO and BG adsorption onto PA-12/CuONPs were determined at various temperatures and shown in and . According to the results, the values of –ΔG values increased with temperature, which indicates that MO and BG were adsorbed more with increasing temperature due to the endothermic and spontaneous nature of the process. The adsorption process of MO and BG was further attributed to physical adsorption since –ΔG values were below 20 kJ/mol (Ahmadi, Hazrati Niari, and Kakavandi Citation2017). For both the dyes, the positive values of ΔH presented the same indication of endothermic and spontaneous processes while the positive values of ΔS indicate increased disorder at the solid/solution boundary during the favorable adsorption of MO and BG onto PA-12/CuONPs.
Table 2. Thermodynamic parameters of dyes’ adsorption onto PA-12/CuONPs.
Adsorption kinetic
As a result of a higher R2 and closer qe (calculated) to qe (experimental) (), pseudo – second-order (PSO) was found to be superior to pseudo – first-order (PFO) in fitting the MO and BG adsorption data (). In general, PSO works well for the adsorption data since its equation does not take the effect of experimental errors into account (Plazinski, Rudzinski, and Plazinska Citation2009). As the values of PFO and PSO rate constants are low, K1 and K2, respectively, equilibrium was achieved at higher concentrations. Elovich’s model parameters of MO and BG adsorption as obtained by linear plots () show reasonable R2 values and higher values of A (adsorption constant) than B (desorption constant) (), confirming that adsorption is more feasible than desorption. The negative B values indicate endothermic processes, which confirms the thermodynamic results. To assess molecular diffusion, intraparticle diffusion model (IPD) plots were used (). It is evident from that MO adsorption is influenced by particle diffusion, as the initial phase of surface adsorption occurs quickly, followed by a gradual internal adsorption of MO onto PA-12/CuONPs, until the diffusion is constant. In terms of BG adsorption, IPD plot has a plateau profile that does not pass through the origin and thus not controlled by film diffusion.
Figure 13. Adsorption Kinetics: Pseudo-first-order (a), Pseudo-second-order (b), Elovich (c), Intraparticle diffusion (d).
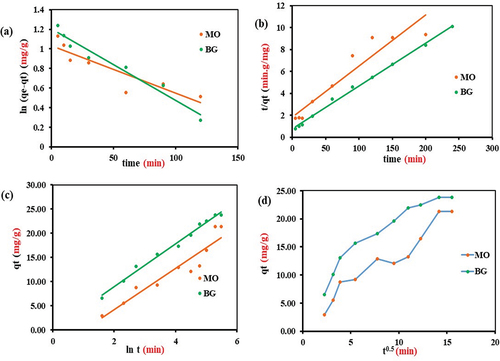
Table 3. Kinetic parameters of dyes’ adsorption onto PA-12/CuONPs.
Desorption
In , the percentage desorption efficiency of MO and BG adsorbed PA-12/CuONPs nanocomposite after five regeneration cycles is shown. According to this result, there is a constant decline in the percentage removal of MO and BG, which is suitable for the recycling of PA-12/CuONPs as well as its practicality and durability. Furthermore, it indicates that MO and BG were readily desorbed from PA-12/CuONPs, indicating a physical adsorption process. After five adsorption-desorption cycles, PA-12/CuONPs has stability of 52.57 and 48.63% for BG and MO, respectively.
Conclusion
This study presents a simple and convenient method for synthesizing copper oxide nanoparticles with polyamide using Trapa natans L. shells. In SEM images, the nanocomposites appear rice-grain-shaped with narrow, spontaneous particle sizes. The BET analysis of the adsorbent revealed an H3 type hysteresis loop, indicating a typical mesoporous structure. For MO and BG adsorption, pH 6 was found to be optimal. As a result of its excellent adsorption capacity toward MO and BG, the material is both feasible and practical. A maximum removal of 93.9% was achieved for MO as well as 97.3% for BG when using the adsorbent. Adsorption mechanisms involved electrostatic attraction and physical adsorption. The most fitted adsorption isotherm models for MO and BG are Langmuir and Temkin, respectively. According to the thermodynamic process, both dye molecules adsorb endothermically and spontaneously. Despite five adsorption-desorption cycles, the adsorbent showed good stability, which made it useful for industrial use. In addition to use of polyamide in buildings, polyamide nanocomposites can also be used to make products that treat water in houses.
Highlights
Copper nanoparticles were synthesized using Trapa natans L. shells.
In order to impregnate the nanoparticles, PA-12 was used.
The rapid desorption of dye molecules confirms the adsorptive potential of the synthesized nanocomposite.
As a result of its excellent adsorption capacity toward MO and BG, the material is both feasible and practical.
PA-12 can also be used to make nanocomposites that can treat water in houses.
The synthesized nanocomposite has stability of 52.57 and 48.63% for BG and MO.
Supplemental Material
Download Zip (530.9 KB)Acknowledgment
The authors would like to thank the Deanship of Scientific Research at Majmaah University for supporting this work under Project No. R-2023-474.
Disclosure statement
No potential conflict of interest was reported by the author(s).
Supplementary material
Supplemental data for this article can be accessed online at https://doi.org/10.1080/15440478.2023.2224976
Additional information
Funding
References
- Agarwal, S., V. Kumar Gupta, M. Ghasemi, and J. Azimi-Amin. 2017. Peganum Harmala -L Seeds adsorbent for the rapid removal of noxious brilliant green dyes from aqueous phase. Journal of Molecular Liquids 231 (April):296–17. doi:10.1016/j.molliq.2017.01.097.
- Ahmadi, M., M. Hazrati Niari, and B. Kakavandi. 2017. Development of maghemite nanoparticles supported on cross-linked chitosan (γ-Fe2O3@CS) as a Recoverable mesoporous magnetic composite for effective heavy metals removal. Journal of Molecular Liquids 248 (December):184–96. doi:10.1016/j.molliq.2017.10.014.
- Alarifi, I., B. Prasad, and M. Kashif Uddin. 2020. Conducting polymer membranes and their applications. 147–76. doi:10.1007/978-3-030-29522-6_5.
- Aldahash, S. A., P. Higgins, S. Siddiqui, and M. Kashif Uddin. 2022. Fabrication of polyamide ‑ 12/Cement nanocomposite and its testing for different dyes removal from aqueous solution: Characterization, adsorption, and regeneration studies. Scientific Reports 12 (1):1–19. no. 0123456789 Nature Publishing Group UK. doi:10.1038/s41598-022-16977-8.
- Al-Hakkani, M. F., S. H. A. Hassan, M. S. Saddik, M. A. El-Mokhtar, and S. A. Al-Shelkamy. 2021. Bioengineering, characterization, and biological activities of C@CuO@Cu nanocomposite based-mediated the vicia faba seeds aqueous extract. Journal of Materials Research and Technology 14 (September):1998–2016. doi:10.1016/j.jmrt.2021.07.076.
- Allaker, R. P. 2012. The use of antimicrobial nanoparticles to control oral infections . In Nano-antimicrobials, 395–425. Berlin, Germany: Springer Berlin Heidelberg. 10.1007/978-3-642-24428-5_14.
- Baig, U., M. Kashif Uddin, and M. A. Gondal. 2020. Removal of hazardous azo dye from water using synthetic nano adsorbent: facile synthesis, characterization, adsorption, regeneration and design of experiments. Colloids and Surfaces A: Physicochemical and Engineering Aspects 584 (January):124031. Elsevier B.V. doi:10.1016/j.colsurfa.2019.124031.
- Basaleh, A. A., M. H. Al-Malack, and T. A. Saleh. 2019. Methylene blue removal using polyamide-vermiculite nanocomposites: kinetics, equilibrium and thermodynamic study. Journal of Environmental Chemical Engineering 7 (3):103107. doi:10.1016/j.jece.2019.103107.
- Bialy, E., E. Badr, R. A. Hamouda, M. A. A. Eldaim, S. S. El Ballal, H. S. Heikal, H. K. Khalifa, and W. N. Hozzein. 2020. Comparative toxicological effects of biologically and chemically synthesized copper oxide nanoparticles on mice. International Journal of Nanomedicine 15:3827–42. doi:10.2147/IJN.S241922.
- Borojevic, K. 2009. Cultural history of water chestnut (Trapa Natans L.) from Prehistory to nowadays = Kulturna Istorija Vodenog Oraha (Trapa Natans L.) Od Preistorije Do Danas. Rad Muzeja Vojvodine 5 (91):159–73.
- Ceylan, E., and A. Kilic Pekgozulu. 2019. Utilization of Trapa Natans. Journal of Anatolian Environmental and Animal Sciences 4 (4):688–94. doi:10.35229/jaes.645341.
- Chakraborty, N., J. Banerjee, P. Chakraborty, A. Banerjee, S. Chanda, K. Ray, K. Acharya, and J. Sarkar. 2022. Green synthesis of copper/copper oxide nanoparticles and their applications: A review. Green Chemistry Letters and Reviews 15 (1):187–215. doi:10.1080/17518253.2022.2025916.
- Feng Ting, L., H. Yang, Y. Zhao, and X. Ran. 2007. Novel modified pectin for heavy metal adsorption. Chinese Chemical Letters 18 (3):325–28. doi:10.1016/j.cclet.2007.01.034.
- Giri, B. S., S. Gun, S. Pandey, A. Trivedi, R. Thapar Kapoor, R. Prasad Singh, O. M. Abdeldayem, E. R. Rene, S. Yadav, P. Chaturvedi, et al. 2020. Reusability of brilliant green dye contaminated wastewater using corncob biochar and brevibacillus parabrevis: hybrid treatment and kinetic studies. Bioengineered 11 (1):743–58. doi:10.1080/21655979.2020.1788353.
- Hanemann, T., and D. Vinga Szabó. 2010. Polymer-nanoparticle composites: from synthesis to modern applications. Materials 3 (6):3468–517. doi:10.3390/ma3063468.
- Husein, D. Z., M. Kashif Uddin, M. Omaish Ansari, and S. S. Ahmed. 2021. Green synthesis, characterization, application and functionality of nitrogen-doped MgO/Graphene nanocomposite. Environmental Science and Pollution Research 28 (22):28014–23. doi:10.1007/s11356-021-12628-z.
- Hussain, M. S., R. Rehman, M. Imran, and A. Figoli. 2021. Isothermal and kinetic investigation of exploring the potential of citric acid-treated trapa natans and citrullus lanatus peels for biosorptive removal of brilliant green dye from water. Edited by Alberto Figoli. Journal of Chemistry 2021 (November):1–23. doi:10.1155/2021/6051116.
- Ikram, M., M. Rashid, A. Haider, S. Naz, J. Haider, A. Raza, M. T. Ansar, M. Kashif Uddin, N. M. Ali, S. S. Ahmed, et al. 2021. A review of photocatalytic characterization, and environmental cleaning, of metal oxide nanostructured materials. Sustainable Materials and Technologies 30 (August):e00343. Elsevier B.V. doi:10.1016/j.susmat.2021.e00343.
- Jadoun, S., R. Arif, N. Kumari Jangid, and R. Kumar Meena. 2021. Green synthesis of nanoparticles using plant extracts: A review. Environmental Chemistry Letters 19 (1):355–74. doi:10.1007/s10311-020-01074-x.
- Khan, T. A., M. Nazir, and E. A. Khan. 2013. Adsorptive removal of rhodamine B from textile wastewater using water chestnut (Trapa Natans L.) Peel: Adsorption dynamics and kinetic studies. Toxicological & Environmental Chemistry 95 (6):919–31. doi:10.1080/02772248.2013.840369.
- Khatoon, A., M. Kashif Uddin, and R. Ali Khan Rao. 2018. Adsorptive remediation of Pb(II) from aqueous media using schleichera oleosa bark. Environmental Technology & Innovation 11 (August):1–14. doi:10.1016/j.eti.2018.04.004.
- Liu, W., Y. Zhang, S. Wang, L. Bai, Y. Deng, and J. Tao. 2021. Effect of pore size distribution and amination on adsorption capacities of polymeric adsorbents. Molecules 26 (17):5267. doi:10.3390/molecules26175267.
- Longfei, X., J. Zhang, J. Ding, T. Liu, G. Shi, L. Xingqi, W. Dang, Y. Cheng, and R. Guo. 2020. Pore structure and fractal characteristics of different Shale Lithofacies in the dalong formation in the western area of the lower yangtze platform. Minerals 10 (1):72. doi:10.3390/min10010072.
- Muhammad, T., M. Muhammad, J. Khan, A. Raziq, M. Kashif Uddin, A. Niaz, S. S. Ahmed, and A. Rahim. 2020. Removal of Rhodamine B Dye from aqueous solutions using photo-fenton processes and novel Ni-Cu@MWCNTs photocatalyst. Journal of Molecular Liquids 312 (August):113399. Elsevier B.V. doi:10.1016/j.molliq.2020.113399.
- Mukhtar, A., N. Mellon, S. Saqib, S.-P. Lee, and M. Azmi Bustam. 2020. Extension of BET theory to CO2 adsorption isotherms for ultra-microporosity of covalent organic polymers. SN Applied Sciences 2 (7):1232. doi:10.1007/s42452-020-2968-9.
- Nguyen, N. T. T., T. T. T. Nguyen, D. T. C. Nguyen, and T. Van Tran. 2023. Green synthesis of znfe2o4 nanoparticles using plant extracts and their applications: A review. The Science of the Total Environment 872 (May):162212. doi:10.1016/j.scitotenv.2023.162212.
- Oplatowska, M., R. F. Donnelly, R. J. Majithiya, D. Glenn Kennedy, and C. T. Elliott. 2011. The potential for human exposure, direct and indirect, to the suspected carcinogenic triphenylmethane dye brilliant green from green paper towels. Food and Chemical Toxicology 49 (8):1870–76. doi:10.1016/j.fct.2011.05.005.
- Plazinski, W., W. Rudzinski, and A. Plazinska. 2009. Theoretical models of sorption kinetics including a surface reaction mechanism: A review. Advances in Colloid and Interface Science 152 (1–2):2–13. doi:10.1016/j.cis.2009.07.009.
- Pohanish, R. P. 2012. Sittig’s handbook of toxic and hazardous chemicals and carcinogens. Elsevier. doi:10.1016/B978-1-4377-7869-4.00017-5.
- Qaiyum, M. A., J. Mohanta, R. Kumari, P. Priyadarsini Samal, B. Dey, and S. Dey. 2022. Alkali treated water chestnut (Trapa Natans L.) shells as a promising phytosorbent for malachite green removal from water. International Journal of Phytoremediation 24 (8):822–30. doi:10.1080/15226514.2021.1977912.
- Qamar, S. U. R., and J. Nazeer Ahmad. 2021. Nanoparticles: Mechanism of biosynthesis using plant extracts, bacteria, fungi, and their applications. Journal of Molecular Liquids 334 (July):116040. doi:10.1016/j.molliq.2021.116040.
- Qiao, C.-L., X. Yan-Ming, Y. Yin, X. Yu-Xing, Y.-H. Xiao, and C.-Q. Liu. 2022. Adsorption of methyl orange on ZnO supported by seawater-modified Red Mud. Water Science and Technology 85 (7):2208–24. doi:10.2166/wst.2022.102.
- Rao, R. A. K., S. Ikram, and M. Kashif Uddin. 2015. Removal of Cr(VI) from aqueous solution on seeds of artimisia absinthium (novel plant material). Desalination & Water Treatment 54 (12):3358–71. Taylor and Francis Inc. doi:10.1080/19443994.2014.908147.
- Rao, R. A. K., and M. Kashifuddin. 2014. Kinetics and isotherm studies of Cd(II) adsorption from aqueous solution utilizing seeds of bottlebrush plant (Callistemon Chisholmii). Applied Water Science 4 (4):371–83. Springer Science and Business Media LLC. doi:10.1007/s13201-014-0153-2.
- Rao, R. A. K., F. Rehman, and M. Kashifuddin. 2012. Removal of Cr(VI) from electroplating wastewater using fruit peel of leechi (Litchi Chinensis). Desalination and Water Treatment 49 (1–3):136–46. doi:10.1080/19443994.2012.708211.
- Raul, P. K., S. Senapati, A. K. Sahoo, I. M. Umlong, R. R. Devi, A. Jyoti Thakur, and V. Veer. 2014. CuO nanorods: A potential and efficient adsorbent in water purification. RSC Advances 4 (76):40580–87. doi:10.1039/C4RA04619F.
- Robinson, T., G. McMullan, R. Marchant, and P. Nigam. 2001. Remediation of dyes in textile effluent: A critical review on current treatment technologies with a proposed alternative. Bioresource Technology 77 (3):247–55. doi:10.1016/S0960-8524(00)00080-8.
- Saddik, M. S., F. M. Alsharif, M. A. El-Mokhtar, M. F. Al-Hakkani, M. M. El-Mahdy, H. S. Farghaly, and H. A. Abou-Taleb. 2020. Biosynthesis, characterization, and wound-healing activity of phenytoin-loaded copper nanoparticles. AAPS PharmScitech 21 (5):175. doi:10.1208/s12249-020-01700-5.
- Saddik, M. S., M. M. A. Elsayed, M. S. A. Abdelkader, M. A. El-Mokhtar, J. A. Abdel-Aleem, A. M. Abu-Dief, M. F. Al-Hakkani, H. S. Farghaly, and H. A. Abou-Taleb. 2021. Novel green biosynthesis of 5-fluorouracil chromium nanoparticles using harpullia pendula extract for treatment of colorectal cancer. Pharmaceutics 13 (2):226. doi:10.3390/pharmaceutics13020226.
- Saddik, M. S., M. M. A. Elsayed, A. A. Abdel-Rheem, M. A. El-Mokhtar, E. S. Mosa, M. F. Al-Hakkani, S. A. Al-Shelkamy, A. Khames, M. A. Daha, and J. A. Abdel-Aleem. 2022. A novesadl C@Fe@Cu nanocomposite loaded with doxorubicin tailored for the treatment of hepatocellular carcinoma. Pharmaceutics 14 (9):1845. doi:10.3390/pharmaceutics14091845.
- Saleh, T. A., M. Tuzen, and A. Sarı. 2018. Polyamide magnetic palygorskite for the simultaneous removal of Hg(II) and methyl mercury; with factorial design analysis. Journal of Environmental Management 211 (April):323–33. doi:10.1016/j.jenvman.2018.01.050.
- Sarwar, N., U. Bin Humayoun, M. Kumar, S. Farrukh Alam Zaidi, J. Hyeon Yoo, N. Ali, D. In Jeong, J. Heon Lee, and D. Ho Yoon. 2021. Citric acid mediated green synthesis of copper nanoparticles using cinnamon bark extract and its multifaceted applications. Journal of Cleaner Production 292:125974. Elsevier Ltd. doi:10.1016/j.jclepro.2021.125974.
- Shin, M. G., W. Choi, S.-J. Park, S. Jeon, S. Hong, and J.-H. Lee. 2022. Critical review and comprehensive analysis of trace organic compound (TOrC) removal with polyamide RO/NF membranes: mechanisms and materials. Chemical Engineering Journal 427 (January):130957. doi:10.1016/j.cej.2021.130957.
- Simha, M., A. S. Gražyna, G. Kratošová, K. Čech Barabaszová, S. Študentová, J. Klusák, S. Brožová, T. Dokoupil, and S. Holešová. 2021. Polyamide 12 materials study of morpho-structural changes during laser sintering of 3D printing. Polymers 13 (5):810. doi:10.3390/polym13050810.
- Siong, V. L. E., K. Mun Lee, J. Ching Juan, C. Wei Lai, X. Hong Tai, and C. Seong Khe. 2019. Removal of methylene blue dye by solvothermally reduced graphene oxide: A metal-free adsorption and photodegradation method. RSC Advances 9 (64):37686–95. doi:10.1039/C9RA05793E.
- Sommereyns, A., T. Hupfeld, S. Gann, T. Wang, W. Cunchen, E. Zhuravlev, A. Lüddecke, S. Baumann, J. Rudloff, M. Lang, et al. 2021. Influence of sub-monolayer quantities of carbon nanoparticles on the melting and crystallization behavior of polyamide 12 powders for additive manufacturing. Materials & Design. 201(March):109487. doi:10.1016/j.matdes.2021.109487.
- Sukla Baidya, K., and U. Kumar. 2021. Adsorption of brilliant green dye from aqueous solution onto chemically modified areca nut husk. South African Journal of Chemical Engineering 35 (January):33–43. doi:10.1016/j.sajce.2020.11.001.
- Sverguzova, S. V., I. G. Shaikhiev, E. V. Fomina, and R. Z. Galimova. 2020. Use of chestnut sheel (Castánea) as adsorption material for removing pollutants from natural and sewage waters: A review. IOP Conference Series: Materials Science and Engineering 945 (1):012072. doi:10.1088/1757-899X/945/1/012072.
- Thandapani, G., K. Arthi, P. Pazhanisamy, J. J. John, C. Vinothini, V. Rekha, K. Santhanalakshmi, and V. Sekar. 2023. Green synthesis of copper oxide nanoparticles using spinacia oleracea leaf extract and evaluation of biological applications: Antioxidant, antibacterial, larvicidal and biosafety assay. Materials Today Communications 34 (March):105248. doi:10.1016/j.mtcomm.2022.105248.
- Uddin, M. K. 2017. A review on the adsorption of heavy metals by clay minerals, with special focus on the past decade. Chemical Engineering Journal 308 (January):438–62. doi:10.1016/j.cej.2016.09.029.
- Uddin, M. K., R. Ali Khan Rao, and K. V. V. Chandra Mouli. 2018. The artificial neural network and box-behnken design for Cu2+ removal by the pottery sludge from water samples: equilibrium, kinetic and thermodynamic studies. Journal of Molecular Liquids 266:617–27. doi:10.1016/j.molliq.2018.06.098.
- Uddin, M. K., and U. Baig. 2019. Synthesis of Co3O4 nanoparticles and their performance towards methyl orange dye removal: Characterisation, adsorption and response surface methodology. Journal of Cleaner Production 211 (February):1141–53. doi:10.1016/j.jclepro.2018.11.232.
- Uddin, M. K., F. Mashkoor, I. M. AlArifi, and A. Nasar. 2021. Simple one-step synthesis process of novel MoS2@bentonite magnetic nanocomposite for efficient adsorption of crystal violet from aqueous solution. Materials Research Bulletin 139 (July):111279. Elsevier Ltd. doi:10.1016/j.materresbull.2021.111279.
- Uddin, M. K., and A. Nasar. 2020. Walnut shell powder as a low-cost adsorbent for methylene blue dye: Isotherm, kinetics, thermodynamic, desorption and response surface methodology examinations. Scientific Reports 10 (1):7983. doi:10.1038/s41598-020-64745-3.
- Uddin, M. K., and P. F. Rahaman. 2017. A Study on the Potential Applications of Rice Husk Derivatives as Useful Adsorptive Material. In Inorganic Pollutants in Wastewater. Methods of Analysis, Removal and Treatment, ed. Inamuddin Mohammad, Ali, and Abdullah M. Asiri, 149–86. Materials Research Forum LLC. doi: 10.21741/9781945291357-4.
- Wani, A., M. S. Ajaz, S. Wazed Ali, S. Ziauddin Ahammad, and M. Kashif Uddin. 2022. Recent advances and future perspectives of polymer-based magnetic nanomaterials for detection and removal of radionuclides: A review. Journal of Molecular Liquids 365 (November):119976. doi:10.1016/j.molliq.2022.119976.
- Xie, H., W. Liu, J. Zhang, C. Zhang, and L. Ren. 2011. Sorption of Norfloxacin from Aqueous Solutions by Activated Carbon Developed from Trapa Natans Husk. Science China Chemistry 54 (5):835–43. doi:10.1007/s11426-010-4132-7.
- Yang, Y., G. I. N. Waterhouse, Y. Chen, D. Sun-Waterhouse, and L. Dapeng. 2022. Microbial-enabled green biosynthesis of nanomaterials: current status and future prospects. Biotechnology Advances 55 (March):107914. doi:10.1016/j.biotechadv.2022.107914.
- Yin, J., G. Zhu, and B. Deng. 2016. Graphene oxide (GO) enhanced polyamide (PA) thin-film nanocomposite (TFN) membrane for water purification. Desalination 379 (February):93–101. doi:10.1016/j.desal.2015.11.001.