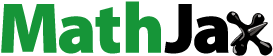
ABSTRACT
The construction industry is among the largest energy-consumer and waste-generating sectors, and it needs sustainable methods and materials to decrease its carbon footprint. One possible solution is to use secondary raw materials and crops as a basis for thermal insulation materials or passive buffers of moisture. This article presents the results of testing raw hemp and flax shives obtained from Polish crops for use in the construction industry. There are only a few works devoted to the parameters of the raw material itself, and no comparative studies are available on the shives themselves. Therefore, it is of utmost importance to compare the properties of shives obtained from these two fibrous plants, to understand their usability and possibilities. Our research focuses on measuring pore size distribution, hygroscopic sorption moisture, capillary rise, and contact angle. It was shown that both types of shives are characterized by similar porosity and hygroscopic sorption characteristics, but different pore size distribution and ability to absorb water through capillary action. Microbiological tests showed variations in the presence of fungi on the shives surfaces. The article also presents an example of the use of the tested materials as an internal wall covering and compares it to gypsum plaster.
摘要
建筑业是最大的能源消耗和废物产生部门之一,它需要可持续的方法和材料来减少碳足迹. 一种可能的解决方案是使用次要原材料和作物作为隔热材料或被动缓冲水分的基础. 本文介绍了从波兰作物中获得的用于建筑业的生大麻和亚麻皮的测试结果. 只有少数作品专门研究原材料本身的参数,而没有关于香菇本身的比较研究. 因此,比较从这两种纤维植物中获得的韭菜的特性,以了解它们的可用性和可能性是至关重要的. 我们的研究重点是测量孔径分布、吸湿吸湿性、毛细上升和接触角. 研究表明,这两种类型的水芹具有相似的孔隙率和吸湿性,但孔径分布和通过毛细管作用吸水的能力不同. 微生物测试揭示了香菇表面真菌数量的变化. 文章还介绍了一个使用测试材料作为内墙覆盖物的例子,并将其与石膏灰泥进行了比较.
Introduction
General information
The construction industry is the largest energy-consumer and waste-generating sector, the operation of buildings accounts for 30% of the global final energy consumption (info on: https://www.iea.org/energy-system/buildings), and construction and demolition are responsible for 37.5% of total waste generated in the EU (info on: https://ec.europa.eu/eurostat/statistics-explained/index.php?title= Waste_statistics#Total_waste_generation). Thermal insulation of buildings can significantly reduce the operational energy use and CO2 emission of buildings. However, currently, the most common thermal insulations are made of plastic or composites of inorganic fibers and organic binder matrix (info on: https://www.grandviewresearch.com/industry-analysis/building-thermal-insulation-market), which are made mostly from artificial or non-recycled materials. The available environmentally friendly thermal insulations are expensive or uncompetitive due to their low or unknown material characteristics. The need for decreasing energy consumption while also decreasing CO2 emission and waste generation is pressing the construction industry, which must increase the use of sustainable methods and materials (Serrano et al. Citation2017). Reducing the generated construction waste, recycling, and using more natural materials to create a circular economy is of paramount importance (Csanády and Nagy Citation2023). There is a huge potential for natural fibrous thermal insulations to emerge, if they can meet the performance with artificial and conventional materials (Asdrubali, D’Alessandro, and Schiavoni Citation2015); therefore, the solution for the construction industry’s above-mentioned problems could be offered by material development. Using more natural materials as thermal insulation materials in the construction industry may lead to decarbonization, reduced energy consumption, and waste generation (Csanády and Nagy Citation2023). There are certain crops, which are cultivated, among others, to obtain fiber for various applications, which are presumably suitable for thermal insulation purposes.
Characterization of shives
Shives are pieces of wood obtained from the stems of fibrous plants such as flax and hemp in the process of their mechanical processing (decortication). The mentioned fiber plants are already available in large quantities since they are used for their fibers in different industries, respectively. The woody part of the stem (after separating the fibers) is often agricultural waste. The wooden part of the hemp stalk constitutes approximately 70% of the total volume of the stalk (Marrot et al. Citation2022). Hemp shives are used as bedding for animals due to their high hygroscopicity and ability to absorb odors. These properties allow to maintain hygiene when breeding animals. Flax and hemp shives were also used in the production of wood-based particleboards (Essid et al. Citation2021; Zvirgzds et al. Citation2022), e.g. as components of furniture.
In recent years, hemp shives, less often flax shives, have been used as an aggregate in a lime-based composite used as an insulating filling for wooden frame constructions. Rahim et al. performed comparative tests on the moisture properties of hemp-lime and flax-lime composites (Rahim et al. Citation2015). The authors showed that due to the similar microstructure of flax and hemp shives, the hygroscopic sorption curves had a similar form, but the moisture content in composite based on flax shives was higher. The work (Benmahiddine et al. Citation2020) showed that high relative air humidity, the maximum content of absorbed moisture and the ability to store moisture increase with the amount of flax shives in the composite, while their fraction has no effect. Brzyski et al. (Brzyski, Gleń, et al. Citation2021) proved that shives tend to arrange their fibers perpendicular to the direction of compaction. The hemp-lime composite exhibits anisotropic properties both in terms of thermal conductivity and capillary rise.
Hygrothermal properties of shives
The shives are characterized by good thermal insulation parameters. In the work (Brachelet et al. Citation2020), the suitability of loose flax shives was examined as thermal insulation of a wooden ceiling, and in the work (Brzyski, Kosiński, et al. Citation2021) as thermal insulation of the attic. Flax shives have a thermal conductivity of 0.047–0.064 W/(m∙K) in the density range of 126–159 kg/m3 (Brachelet et al. Citation2020; Brzyski, Kosiński, et al. Citation2021), while hemp shives have 0.049–0.058 W/(m∙K) (Kosiński et al. Citation2022; Magniont Citation2010; Véronique.CEREZO Citation2005) in the density range of 108–135 kg/m3. In the work (Kosiński et al. Citation2022) a simulation of a frame wall filled with hemp shives in a loose-fill state was carried out. The value of thermal conductivity depends on the degree of compaction, but in the works cited above it was not a strict linear relationship. Low thermal conductivity results from the porosity of the shives themselves, around 78% (Kosiński et al. Citation2022), as well as the air spaces contained between the shives. The porosity of a stack of flax shives is, for example, 92.9% at a density of 90 kg/m3, while that of hemp shives is 90.1% at a density of 125 kg/m3 (Rahim et al. Citation2015). Benmahiddine et al. (Benmahiddine et al. Citation2020) showed that the structure of flax shives is shaped with capillary pores running along the growth of the plant, and these capillaries are connected by small cavities. This structure of flax shives dampens heat flow and increases the ability to absorb and transport water and water vapor. In the above mentioned work, pores with a diameter of approximately 17–33 μm were identified. Collet et al. (Collet et al. Citation2013), in turn, showed that the structure of hemp shives consists of a network of closed pores with a diameter of 5 to 50 µm.
Another thermal property that characterizes the shives is specific heat. Due to the presence of cellulose and hemicellulose, this material achieves values of this parameter at a level higher than traditional thermal insulation materials (Yousefi and Tariku Citation2021). The work (Qi et al. Citation2020) showed that the specific heat of the example cellulose at room temperature is from about 1100–1300 J/(kg∙K), in turn, in (Yousefi and Tariku Citation2021) this parameter was 1900 J/(kg∙K). Hemp shives contain approximately 36–45% cellulose and 31–37% hemicellulose (Balčiunas et al. Citation2013; Schwarzova, Stevulova, and Melichar Citation2017), while flax shives contain approximately 30–50% cellulose and 20–25% hemicellulose (Buranov and Mazza Citation2010). Novakova (Nováková Citation2018) mentioned that the specific heat of hemp shives is 1300 J/(kg∙K), while Pundience et al. (Pundiene et al. Citation2022) compared two species of hemp shives differing in specific heat 1240 and 1270 J/(kg∙K). The specific heat of flax shives is 1200–1600 J/(kg∙K) in the density range of 126–154 kg/m3 (Brachelet et al. Citation2020).
Due to their high porosity, flax and hemp shives are characterized by high water absorption. According to the literature (Dubois et al. Citation2018), 30 s after immersion, the water absorption of flax shives was 130%. In other studies, water absorption was 195% after 24 h of soaking in water (Brzyski, Kosiński, et al. Citation2021). Absorption increases dynamically in the first seconds after immersion in water. In turn, the water absorption of hemp shives, according to selected studies, 10 min after immersion in water was 250% (Arnaud and Gourlay Citation2012). Delhomme et al. (Delhomme et al. Citation2020) showed that hemp shives soaked in water for 1 min showed a water absorption of approximately 160–190%, and after 48 h, the water absorption was 350–400%, depending on the type of shives.
Susceptibility to biological corrosion of shives
Shives are susceptible to biological corrosion, which is the result of the impact of microorganisms on their structure. The main factors contributing to the development of biological corrosion of organic materials are humidity and ambient temperature (Johansson, Balksten, and Brigitte Strandberg-de Bruijn Citation2022; Silveira, Martins Pinto, and Simon Westphal Citation2019). Increased relative air humidity and water vapor condensation inside the wall or on its surface create a favorable environment for the development of microorganisms that may lead to the biodegradation of wall components (You et al. Citation2017). The high water absorption of shives and the ability to store moisture may favor the development of microorganisms in unfavorable environmental conditions, e.g. limited drying capacity. Some research has already been carried out on the problem of biological corrosion of shive-based wall material, i.e. hemp-lime or hemp-clay composite (Arizzi et al. Citation2018; Johansson, Balksten, and Brigitte Strandberg-de Bruijn Citation2022; Marceau et al. Citation2017; Walker, Pavia, and Mitchell Citation2014). Johansson et al. (Citation2022) showed that the critical moisture level for a hemp-lime composite was between 75% and 85% RH, while at 75% RH, no microbial growth occurred in the material. It was found that lime had a protective effect on shives as a mold inhibitor at variable temperatures and humidity. Marceau et al. (Marceau et al. Citation2017), in turn, they found that mold growth on hemp-lime composite samples occurred at high relative humidity levels, around 98%. The tests showed that hemp composite based on lime binder is resistant to biodegradation in variable thermal and humidity conditions prevailing on construction sites (in Europe), i.e. alternating dry and humid periods (Walker, Pavia, and Mitchell Citation2014). The authors also emphasized that shives do not provide the appropriate amount of nutrients for the development of microorganisms.
Purpose and scope of the paper
This article presents research aimed at characterizing flax and hemp shives in terms of selected moisture properties and microstructure. The susceptibility to biological corrosion of these materials was also described. Based on some of the tested parameters, the operation of an external wall finished with a layer of shives from the inside was simulated in variable ambient relative humidity conditions (in the Delphin software). This work is an extension of previous own works on selected properties of hemp (Kosiński et al. Citation2022) and flax shives (Brzyski, Kosiński, et al. Citation2021). Additional tests were performed, and the two materials were compared. There are many comparative studies on, for example, composites based on flax and hemp shives, but there are no comparative studies on the shives themselves, which can be used not only in composites. Our scope is to offer a direct comparison using a consistent methodology to unfold the benefits of hemp and flax shives for sustainable building practices using a consistent methodology. Besides the experimental testing, this article aims to present the application possibilities and construction-relevant conditions of the tested shives by hygrothermal simulations.
Materials and methods
Materials used in investigation
Hemp shives of the Białobrzeskie variety of the Polish producer Podlaskie Konopie (Białystok, Poland) were used for the experiment. They were obtained from crops intended for construction purposes. These were high-quality shives used mainly as a filler in a hemp-lime composite. They were characterized by a low dust and fiber content. During the mechanical processing of hemp stalks (performed by the manufacturer), hemp shives were separated from fibers and dusty fractions, which in excess negatively affect the parameters of the hemp-lime composite or other building materials. Their bulk density was 101.2 kg/m3, while the moisture content as delivered from the manufacturer was 9%. The flax shives used in the research were produced from the Polish variety Modran by the Institute of Natural Fibers and Medicinal Plants in Poznań. They were obtained from crops intended for the textile industry and constitute waste. However, they were of satisfactory purity in terms of fiber and dust content. The bulk density of flax shives was 115.2 kg/m3, while the moisture content in the air-dry state was about 8.8%. Both hemp and flax shives were obtained from straw subjected to retting in the field. The shives used in the research are shown in the photographs ().
The granulation of shives in terms of the share of individual lengths is presented in .
Table 1. Percentage of individual lengths of flax and hemp shives in the mass of the sample.
Methods
Hygroscopic sorption
The research of hygroscopic sorption of the tested samples was carried out following EN ISO 12,571:2021. The samples were randomly selected from the materials supplied to the laboratory. In the next stage, the samples were dried at 105°C to obtain a constant, moisture-free mass. The fragmented samples were placed on the pre-weighed aluminum laboratory molds. The molds were perforated to allow free moisture flow around the tested materials. After cooling the samples, the moisture-free materials were placed in desiccators over the saturated salt solution and sealed. Magnetic stirrers were used to maintain constant relative humidity over the salt solution. The authors of this paper prepared two specimens of hemp shives and two of flax shives. The mass of the dried specimens was 3.0 and 4.3 g in the case of flax shives, and 3.8 and 4.7 g in the case of hemp shives.
Different salt solutions were put in desiccators to maintain specific relative humidity conditions inside. The properties of the used salt solutions are given in . These solutions were used in the determination of hygroscopic sorption isotherms also in previous works (Kosiński et al. Citation2023; Kosiński, Brzyski, and Duliasz Citation2020; O’Brien Citation1948; Strandberg-de Bruijn and Johansson Citation2014; Tamborski Citation2009).
Table 2. Salt solutions and relative humidity (according to ISO 12,571:2021).
The authors of this paper prepared two specimens of hemp shives and two of flax shives to test hygroscopic sorption. In total, 23 mass measurements of specimens over five solutions maintaining different humidity levels were made. The relative humidity over salt solutions is presented in . Mass measurements were taken once a day at a constant interval of 24 h.
The control of the changing mass of the tested insulation materials took place cyclically, the readings were made once a day until the hygroscopic sorption equilibrium was obtained.
Sorption moisture is determined by the dry mass of the sample and is given by the formula (1):
Where: is the sorption moisture in relation to the dry mass [%];
is the sample mass in a dry state [g];
is the mass of the sample in a sorption equilibrium [g].
Capillary rise
The study of hemp shives (HS) capillary uptake was carried out on three specimens of average mass of 60 g, placed in a PVC tube with a diameter of 75 mm and a height of 120 mm. The bottom of the mold was covered with a fine mesh in the form of a viscose dressing bandage with a mesh size of approximately 2 mm × 2 mm. The prepared tube with shives was immersed in water to a depth of 10 mm. This procedure was also used in other own research on flax shives (Brzyski, Kosiński, et al. Citation2021). The shives were lightly compacted with a wooden element with a diameter of 30 mm in the direction perpendicular to the bottom of the tube, to eliminate empty spaces resulting from the random arrangement of the shives, filling the tube. It is a similar way, for example, in wall formwork, when shives are used as insulation in a mixture with lime (Brzyski, Gleń, et al. Citation2021). The way the shives were arranged after compacting ensured shape stability and prevented them from spilling out through the mesh. The walls of the tubes are protected by water absorption by the side surface of the thickened sample and also prevent water from evaporating from the sample, similar to a bituminous coating in the case of testing solid building materials. Mass gains of the specimens were read over 15 min to 48 h with varying frequency. This test time was determined based on the observation of the stabilization of the mass of the water pulled up by the material. This period was also adopted at work (Brzyski, Kosiński, et al. Citation2021). Further extension of the test time could result in water evaporating through the upper, unprotected surface, as well as biological corrosion. shows the test stand and shows the position of the shives relative to the water table. During compaction, the shives tend to arrange their fibers perpendicularly to the direction of compaction, which is why the majority of the shives are arranged with fibers parallel to the water table. The HS capillary rise results were compared with the flax shives (FS) capillary rise results from other own work (Brzyski, Kosiński, et al. Citation2021).
Contact angle
Detailed contact angle measurement methods are available in the literature (Bigelow, Pickett, and Zisman Citation1946; Taggart Arthur, Taylor, and Ince Citation1930; Wilhelmy Citation1863). The measurement of the contact angle informs about the degree of wetting of the material affected by the drop. Small contact angles (<90°) correspond to high wettability, while large contact angles (>90°) correspond to the low wettability of the material (Wójcik et al. Citation2017; Yuan and Lee Citation2013). What is important, is that there are materials whose contact angles are almost equal to 90°.
The authors of this study used the non-isothermal goniometer OCA 20 (the sitting drop method) for the described experiment. The goniometer consists of a: movable adjustable positioning table, a video camera, a syringe with liquid, and a light source. During the measurement, the drop shape was registered by the camera, then the image was processed by the software. The authors selected 10 specimens of each material. The selection was done randomly from a container with shives. Drops of demineralized water at room temperature were dropped perpendicularly to the surface of the shives.
Studies on the contact angle of fibrous natural-based materials mostly revealed their hydrophilic nature (Barnat-Hunek, Smarzewski, and Brzyski Citation2017; Fuentes et al. Citation2014; Schellbach, Monteiro, and Drelich Citation2016). On the other hand, a bit of processed material, like insulation made of waste cellulose fibers demonstrated a weekly hydrophilic, weekly hydrophobic nature, contact angle between 71° and 103° (Kosiński, Brzyski, and Duliasz Citation2020).
Porosity
Tests using mercury intrusion porosimetry (MIP) were based on the assumption defined by Washburn (Washburn Citation1921), in which the pressure is inversely proportional to the radius of the cylindrical capillary. As a consequence, it is possible to determine the porosity distribution and the total porosity. The following values were adopted for the measurements: the surface tension angle of mercury was θ = 140°, while the surface tension γ = 485 mJ/m2. Based on previous porosity studies (Jang, Won Kang, and Sik Jang Citation2018; Jiang et al. Citation2017, Citation2018; Plötze and Niemz Citation2011; Shen et al. Citation2021), it was established that mercury porosimetry can be successfully used for comparative studies content and distribution of pores in hemp and flax shives. Porosimeter AutoPore IV 9500 was used for the measurements. The study was carried out to determine the content of pores in the range of 200 to 0.006 µm. A penetrometer with a vessel capacity of 3 cm3 and capillaries with an intrusion volume of up to 1.12 cm3 was used for the tests. The mass of the samples oscillated between 0.19 ± 0.034 g. All samples were dried to a constant mass at a temperature of 70.0°C.
Five randomly woody samples of flax shives (FS) were selected for testing. The FS porosity results, after calculating average values, were compared with the porosity results of hemp fibers previously tested and presented in the authors’ work (Kosiński et al. Citation2022). Accordingly, the mean values from the hemp fiber measurements were called the HS mean and the hemp fiber measurements were called the FS mean. Based on the MIP results, porosity and the percentage of pores with a specific diameter were calculated.
Scanning electron microscopy
The pore structure of flax and hemp shives was investigated using a scanning electron microscope (Phenom XL Desktop SEM, Phenom-World B.V., Eindhoven, The Netherlands). Its energy-dispersive X-ray spectroscopy (EDS) function was also used to study the atomic concentration and the elemental composition of the examined shives. Three specimens were prepared for both cross-section and fiber direction samples. At first, all samples were sputter-coated with gold using an automatic sputter coater (AGB7341, Agar Scientific Ltd., Essex, UK). Then, in the SEM, a 5 kV accelerating voltage was applied for the pore structure examination, firstly using the backscattered electron detector (BSD) of the instrument, which detects elastically scattered electrons. These are higher in energy from atoms below the sample surface. The experiment was started with BSD since it allows for lower vacuum levels (60 Pa) and has lower sample preparation requirements and minimizing beam damage. The SEM images were obtained at 120×, 1000×, and 2000× magnification for both cross-section and fiber direction specimens. Then the experiment moved to the secondary electron detector (SED), which is used both for pore structure using 5 kV accelerating voltage, with similar magnifications as the previous experiment using BSD. The SED gathered the topographical information from low energy inelastically scattered electrons close to the sample surface but requires a high vacuum (1 Pa) setup. The images were acquired using high resolution and a 3-s exposure time. Then EDS analysis was performed using 15 kV accelerating voltage, 1 Pa vacuum setup, and BSD using 269 μm field of view (FOV) on three randomly selected regions of the same specimens to identify the elements of the flax and hemp shives and obtain the atomic and weight concentration of the identified elements. The scanning electron microscope’s software automatically identifies all the specimen elements, but the operator can narrow or expand the range of identified elements. Worthy of note, the instrument cannot identify hydrogen, so it was omitted in the investigation. During the element identification, due to the sputter coating, gold (Au), mercury (Hg), niobium (Nb), platinum (Pt), Thallium (TI), and zirconium (Zr) were excluded from the analysis. In the case of the tested samples, the identified elements were reduced to oxygen (O) carbon (C), and other elements, since these elements are undoubtedly present in the highest mass percentages in lignocelluloses. Even with this simplification, the identified elements could be classified into the C or O category with 99% fitting.
Microbiological test
Microbiological tests were performed on samples of hemp and flax shives, which were visibly covered with a green coating after keeping them in 100% RH conditions for 120 days. To identify the microbiological quality of flax and hemp samples, imprints were taken directly from the places with a visible green coating and slides were stained with aniline blue in lactophenol using the Gerlach method (Gerlach Citation1972). This is a histological staining method used for the microscopic examination and identification of fungi. Lactophenol Aniline Blue is a stain that contains three main components: phenol which precipitates cytoplasmic proteins and inactivates enzyme systems within fungal cells; lactic acid which preserves fungal structures, and aniline blue which stains the fungal structures making them more distinct. Then, microscopic observations were carried out using the OLYMPUS B×51 microscope at ×100 magnification with a Charge Coupled Device (CCD) camera and a digital image archiving system, and photographic documentation was made.
The identification of the observed filamentous fungi was based on the structure of the sporangia (microscopic evaluation) and the morphological appearance of the colonies (macroscopic evaluation). The systematic affiliation of mold fungi was determined based on the “Atlas of clinical fungi” (de Hoog et al. Citation2019) and the taxonomic study of Borowska (Citation1986) and Fassatiova (Citation1983).
The morphological appearance of the colonies and the quantitative composition of the tested microorganisms in the analyzed material were assessed based on the microbial colonies grown on culture media. For this purpose, 1 g of the tested material was suspended in 9 ml of physiological fluid. The sample was homogenized on a magnetic stirrer to make it uniform. A series of dilutions were made from the obtained suspension, then 1 ml of the test material was sown on the RBC medium (Rose-Bengal Chloramphenicol Agar by MERCK) for the identification of filamentous fungi and incubated at 28°C for 5–8 days. The morphology of the colonies was determined by: the appearance and color of the mycelium, changes in color during sporulation, the color of the mycelium from the bottom, shape, and size of the colony, structure of the surface and edges of the colony, changes in the color of the substrate. The total number of bacteria on the TSA medium (Tryptic Soy Agar by MERCK) at 22°C/72 h was also determined analogously. The obtained results were given as cfu (colony forming units) in 1 g of the tested flax or hemp sample.
Results and discussion
Hygroscopic sorption
shows the average values of the measured hygroscopic sorption moisture of hemp shives. The research revealed that the hemp shives placed over saturated solutions of salts at constant air relative humidity (RH) reached the sorption equilibrium as follows: at RH 32% it reached 5.4% moisture content (mc) on the 10th day, at RH 54% - mc 9% on the 16th day, at RH 75% - mc 12.9% on the 14th day, at RH 95% - mc 23.2% on the 15th day, and at RH 100% - mc 22.8% on the 18th day.
shows the average values of the measured hygroscopic sorption moisture of flax shives. The research revealed that the flax shives placed over saturated solutions of salts at constant air relative humidity (RH) reached the sorption equilibrium as follows: at RH 32% it reached 5.3% moisture content (mc) on the 5th day, at RH 54% - mc 9.6% on the 19th day, at RH 75% - mc 13.1% on the 15th day, at RH 93% - mc 22.7% on the 17th day, and at RH 100% - mc 23.8% on the 12th day.
For both materials, it is visible that in RH 93% and 100% conditions, the moisture content increased during the whole investigation period. At the same time, in conditions of lower relative humidity, moisture content increase was slower. Finally, significant differences in moisture content were between samples stored over RH 32%, 54%, and 75%, while for those kept in RH 95% and 100% were very small.
The differences between the absorbability of hemp and flax shives are small. Hemp shives show greater stability and constant growth, while in flax material, in the growth for RH 93 and 100%, some peaks were observed. The maximum obtained sorption capacity of both shives at RH 32, 54 and 93% is comparable to the hemp fibers described in the work (Kosiński, Brzyski, and Duliasz Citation2020). As a rule, the achieved water absorption is lower than for cellulose fibers and much higher than for mineral wool. This indicates the potential of hemp and linen shives to accumulate moisture.
Capillary rise
The results of capillary rise measurements are presented in . Hemp shives showed a greater ability to absorb water through capillary rise compared to flax shives by approximately 56% (after 48 h of testing). HS are characterized by a lower bulk density than FS by approximately 12–20%. A lower bulk density may indicate a larger volume of free spaces between the shives, which may have been partially filled with water. Additionally, hemp shives are more porous than flax shives, as shown in the MIP study ( and ). As shown in the SEM images (), the walls of the longitudinal pores of hemp shives are perforated, which allows water to be transported also across the edge of the fibers. Perforation to such an extent was not noticed in the images made using a scanning electron microscope in the case of flax shives (). This is an important feature of hemp shives, which could have resulted in a greater ability to raise water by capillary action. The anisotropic character of hemp shives, based on the example of the capillary action of water through a lime-hemp composite, is presented in (Brzyski, Gleń, et al. Citation2021). The height of the rising water column, as shown in , is comparable for both types of shives, despite the difference in the length of the test period.
Figure 4. Capillary rise of three specimens of hemp shives (a), and flax shives (b) samples over time.
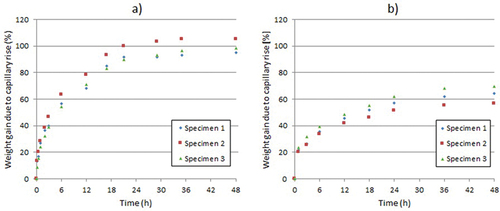
Figure 5. Range of the water raised up in flax shives (a) sample after 48 h of testing, and hemp shives (b) sample after 70 h of testing.
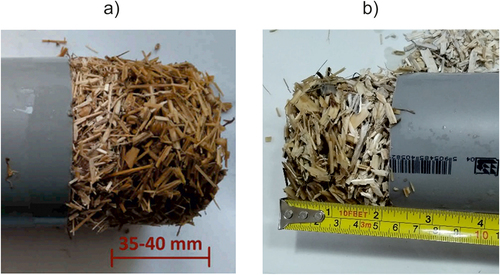
Table 3. Material properties determined during the MIP analysis.
Contact angle
presents the values of contact angles measured for 10 different specimens of each of the tested shives. The shives for testing and their surfaces were intentionally selected randomly so that the test could reflect the real conditions of selecting the fibers for utility purposes. The results are presented from the largest to the lowest values. In the selected group of shives, the contact angle values for the tested flax shives are larger than for hemp shives. The average value of the measured angle for flax shives is 104.7° (standard deviation 20.4°) and for hemp shives 88.7° (standard deviation 16.9°). It was noticed that the contact angle strongly depends on the tested material specimen, its structure, and size. Seven out of ten tested flax shives and three out of ten hemp shives show an angle above 90°.
Any time the inner surface of the flax specimen (closer to the core of the plant) was tested, the measured contact angle was smaller than 90°. In the case of hemp shives, such results were measured even on some of the tested outer surfaces of specimens. Finally, hydrophilic properties (contact angle < 90°) were observed in the case of flax specimens 1–7 and hemp specimens 1–3, while hydrophobic properties (contact angle > 90°) in the case of flax specimens 8–10 and hemp specimens 4–10.
Both tested materials are of natural origin, and, as a rule, they cannot have uniform features like synthetic insulation, e.g. mineral wool. However, there are significant differences in the results obtained between flax and hemp. One of the reasons is the structure of plants, which influences cell connections, hardness, and porosity. The size of the samples is another reason for the differences in contact angle testing. Flax has thinner stems than hemp. Thus, flax shives are naturally smaller than hemp shives. Both materials were tested with the same water drop sizes. It was observed that water drops stayed longer on the flax shives’ surfaces than on the hemp shives. This may be caused by the different surface roughnesses or the ratio between the drop size and the shive width. This feature requires further research.
The tested shives showed a contact angle between 67.6 and 130.9. Thus, some of the tested sample surfaces had hydrophilic properties, and some had hydrophobic properties.
When shives are used as a building material, there is no chance to arrange direction of them just to expose to possible moisture source with their hydrophobic surface. The size of shives is small enough that their application makes directed installation impossible. However, it should be noted that flax shives have a larger wetting angle, and therefore the risk of getting wet is lower than that of hemp shives.
Porosity
Mercury intrusion porosimetry was performed on five FS specimens. Average values were derived from the received data and are presented in . Parameters such as total intrusion volume, total pore area, median pore diameter, average pore diameter, apparent density, and total porosity were determined. To compare the microstructure of flax shives with hemp shives HS, the results were compared with the values obtained in the publication (Kosiński et al. Citation2022).
Total intrusion volume, total pore area, median and average pore diameter, skeletal density, and porosity were determined. The biggest difference was noticed in the properties of the average pore diameter. The measured value of the average pore diameter of hemp shives is 0.691 ± 0.144 µm and in flax shives, the diameter is almost two times smaller and is equal to 0.391 ± 0.067 µm. The other parameters are similar, respectively: total intrusion volume for hemp shives is 3.21 ± 0.30 mL/g whereas for flax shives 2.73 ± 0.21 mL/g. Apparent (skeletal) density for both types of fibers amounted to approx 1.16–1.12 g/ml. The porosity of hemp shives is 78.70 ± 1.64%, while that of flax shives is 77.51 ± 0.79%.
The distribution of average porosities (obtained from 5 material specimens) of hemp and flax shives is presented in . For hemp fibers, there are two main peaks, the first in the pore diameter range from 50.0 to 9.0 µm and the second from 2.0 to 0.9 µm, while for flax fibers the first peak occurs, similarly to hemp fibers, in the range from 50.0 to 9.0 µm, while the second dominant range occurs in the range from 0.6 to 0.006 µm. In the diameter range from 90.0 to 50.0 µm; 9.0–3.0 µm and above 0.08 µm the share of pores is small. In the case of hemp shives, pores up to 0.9 microns in diameter prevail and their share is 66.21%, while concerning flax shives pores with a diameter below 0.9 microns (63.60%) have a larger share. The possibility of sample crushing due to excessive pressure may have occurred at the highest pressure level, however, as indicated by publications on other natural fibrous materials (Plötze and Niemz Citation2011) the possibility of compression is less than 5% of the cumulative pore volume.
The total porosities of flax and hemp shives are very similar. However, the characteristics of the pore size distributions in the tested materials are different. According to (Jan and Wyrwał Citation2010) in porous materials with a dominant content of pores with a diameter greater than 2 microns, molecular diffusion is mainly present, therefore it can be concluded that this type of mass transport will dominate in materials with a significant content of hemp fibers. The distribution, size, and even shape of the pores affect the sorption characteristics of the materials.
While only woody parts of plant stems were selected for porosimetry tests, it should be remembered that in the volume of all fibers, there are also soft parts of the plant. Therefore, porosimetry tests should be extended with additional measurements, enabling the determination of porosity in the entire structure of the shives tested.
SEM and EDS observations
presents the chemical composition of the tested shives measuring three specimens each by EDS using the desktop SEM apparatus. Two of the used SEM images are shown in and the analyzed area, covering the whole picture, is indicated with white dashed line marking. The main components of the shives are composed of hydrogen, carbon, and oxygen, such as cellulose (C6H10O5), lignin (C9H10O2, C10H12O3, C11H14O4), hemicellulose (C18H32O16, C24H42O21), and pectin (C6H10O7). Other elements identified (for example due to sample contamination) were mainly nitrogen (N), sodium (Na), Calcium (Ca), and Silicon (Si).
Table 4. Chemical composition of the shives.
An observation of the FS and HS shives () at 120× magnification shows that the outer surfaces of both materials are porous. The pores visible on the HS surface are slightly larger (from several to 80 μm) than on the FS surface (from several to 50 μm). In the case of HS, the share of pores exceeding 50 μm is greater. Observation under 1,000 magnification of cross-sections reveals the complex structure of materials. In both cases, the structure located away from the large pores is ordered, and the walls separating the internal pores are parallel to each other in one of the directions. The walls separating the pores are approximately 1 μm thick regardless of the material. The pores are primarily oblong, forming an ellipse. In the case of FS, the dimensions of pores are usually approx. 16 × 20 μm, 10 × 30 μm, and occasionally larger pores with dimensions of up to 50 × 60 μm are visible. While in the case of HS, pores usually have dimensions of 20 × 25 μm, and 19 × 35 μm, occasionally larger pores with dimensions up to 40 × 95 μm are visible. Importantly, air cells limited by HS walls have a less regular shape and their edges bordering them are more jagged than those of FS and elongated along one of the directions. Near large pores, the structure of HS and FS is disturbed and less regular.
Figure 8. Cross-section through flax (a, c) and hemp (b, d) shives, magnification 120× (a, b), 1000× (c, d).
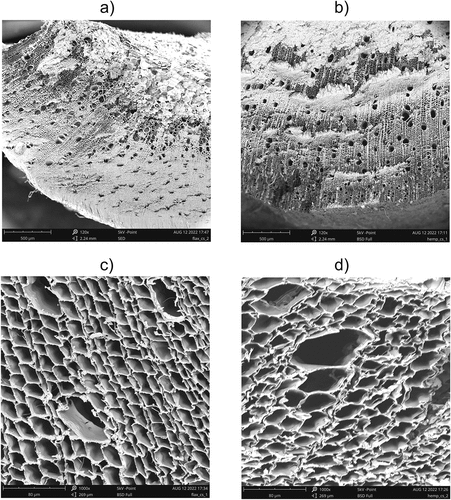
An observation in the direction perpendicular to the fibers () shows the pore diameters in the range of 12–25 μm in the case of FS, and the range of 17–28 μm in the case of HS. In the case of both materials, the capillaries adhere to each other and face in one direction.
SEM observations of both materials indicate that in HS the pores are slightly larger when compared to FS and the walls limiting them are more jagged.
Microbiological analyzes
Based on the microbiological analysis of flax and hemp samples, the presence of filamentous fungi was found, the number of which was 4.41 × 108 CFU/1 g and 2.1 × 107 CFU/1 g, respectively. In the tested flax samples, 5.0 × 104 CFU/1 g of the total number of bacteria (determined on Tryptic Soy Agar (TSA) at 22°C/72 h) were also found, while these microorganisms were not found in the hemp samples. Similar results were obtained by Buick and Magee (Buick and Magee Citation1999) in samples of flax from a textile plant, where they determined the average counts of bacteria and fungi at the level of 2.2 × 109 CFU/g. However, Kymalainen et al. (Kymäläinen et al. Citation2005) and Nykter et al. (Nykter et al. Citation2008) identified bacteria and fungi on hemp fibers in the number of 4.5 × 104–1.7 × 106 CFU/g and on linseed fibers from 3.4 × 102 to 3.7 × 104 CFU/g, respectively. The range of the number of bacteria and filamentous fungi in materials of plant origin varies and depends on the environmental conditions to which these structures are exposed. Increased humidity above 60% and temperature above 25°C favor the proliferation of microorganisms, especially mold fungi. The qualitative composition of identified mold fungi infecting plant fibers depends on their type or season of harvesting these materials. From hemp, flax, cotton, and linseed fibers, mainly fungi of the following genera were isolated: Aspergillus (including A. flavus and A. niger), Alternaria, Mucor, Penicillium, Chaetomium, Cladosporium, Trichoderma, Trichothecium, Rhizopus, Stachybotrys (Buick and Magee Citation1999; Górny et al. Citation2015; Kymäläinen et al. Citation2005; Nykter et al. Citation2008; Pekhtasheva et al. Citation2012).
Based on the own research and microscopic analysis of preparations, the presence of mainly Aspergillus species was found in the tested samples of flax and hemp. Based on the morphological features of the colonies and the structure of the sporangia, these fungi were classified as Aspergillus flavus (). These molds dominated mainly in flax samples. In the preparations prepared from hemp samples, mainly fungi belonging to Engyodontium () and Acremonium () were found, and less frequently Aspergillus hyphae were determined.
Figure 10. Colonies of filamentous fungi grown on Rose-Bengal Chloramphenicol Agar (RBC) medium from flax shives (a) and hemp shives (b).
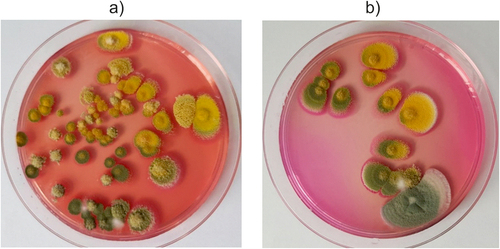
Figure 11. Microscopic views (x100) of Aspergillus flavus conidiophores isolated from flax shives samples.
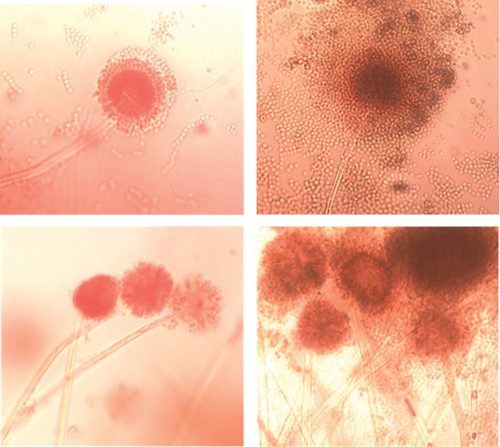
Aspergillus flavus is a cosmopolitan fungus, usually found as a saprophyte in soil and decaying organic matter. It can contaminate agricultural products, causing them to spoil. Some strains produce aflatoxins and can cause mycotoxicosis in humans and animals. Although the presence of Aspergillus spp. does not necessarily indicate the absence of toxins, early identification of these microorganisms is important for the safety of the materials or food products (Kosegarten et al. Citation2017; Mandeel Citation2005; Perrone et al. Citation2007).
Possible application of hemp and flax shives in construction industry
Flax and hemp shives’ thermal conductivities are small enough to use these materials as thermal insulations. Examples of using FS and HS as loose fill insulations in walls or ceilings () are presented in more detail by (Brzyski, Kosiński, et al. Citation2021). FS of the Modran variety and HS of the Białobrzeskie variety reach bulk densities in the range of 125–162 kg/m3 and 108–126 kg/m3, respectively. In both materials thermal conductivity increases with increasing density, in the case of HS from 0.049 to 0.052 W/(m∙K) and in the case of FS from 0.047 to 0.053 W/(m∙K).
The authors calculated thermal transmittances for external walls and frame ceilings filled with loose-fill FS or HS. The calculations were made according to ISO 6946. The following layer system was assumed for the calculations: OSB board 0.025 m, loose-fill insulation made of shives, OSB board 0.025 m. Finishing layers and water vapor retarders were not taken into account due to their negligible thermal resistance. Surface resistances were assumed for the calculations as for the vertical direction of heat flow upwards, which means that the calculation results will also meet the requirements for walls. presents the minimum thickness of the insulation layer in external ceilings to achieve heat transfer coefficients of 0.1–0.3 W/m2K. Calculations were made for heterogeneous walls and ceilings, assuming the axial spacing of wooden structural elements with a width of 0.06 m every 0.76 m, and the thermal conductivity of the shives 0.051 W/(mK). To reduce the impact of thermal bridges, you can use I-section columns with a web made of e.g. fiberboard about 1 cm thick. For wall thicknesses above 0.30 m, additional stiffeners in the form of horizontal battens should be designed.
Table 5. Minimal thickness of loose-fill insulation made of HS or FS to achieve required U-value of building element (according to ISO 6946).
In addition to being used as bulk insulation, shives can be used to produce hempcrete and flexcrete insulation mixtures. These are composites based on shives and a binder, mainly in the form of lime with additives, but also clay. Due to the presence of porous shives, they are characterized by low values of thermal conductivity coefficient of 0.074–0.138 W/(m·K) (Benfratello et al. Citation2013; Stevulova et al. Citation2013; Walker and Pavía Citation2014) and low density in the range of 300–600 kg/m3 (Benfratello et al. Citation2013; Łapka et al. Citation2023; Rahim et al. Citation2015). Composites are used as a monolithic filling of a wooden frame or as an insulating wall material in the form of blocks. In addition to the ability to provide thermal insulation, they are also characterized by a high thermal capacity of the order of 1000–1600 J/(kg∙K) (Barclay, Holcroft, and Shea Citation2014; Maalouf et al. Citation2014; Tran Le et al. Citation2010), thanks to which they can store heat and improve the thermal stability of rooms.
The authors of the study want to draw attention to another possible way of using shives in construction. Due to their high ability to absorb moisture, shives could serve as an internal moisture buffer. A layer of a few mm thick could be applied to the adhesive substrate. This would be an alternative to commonly used gypsum plasters, which do not have high buffering capacity or release moisture to the indoor environment.
Hygrothermal simulation
The heat and moisture transfer simulations were performed to present the hemp or flax shives properties as internal finishing (a layer of shives as a wallpaper glued to a full ceramic brick wall). The finishing layer of shives was compared with the commonly used gypsum plaster. From the outside, the wall was insulated with mineral wool glued to the wall, covered with a cement-lime plaster. The diagram of the wall is shown in . The simulations were performed using the Control Volume Method in the Delphin 6.1 software.
Figure 16. Climate conditions and model for simulation: (a) relative humidity and (b) temperature for Olsztyn, based on Typical Meteorological Year (c) internal temperature and relative humidity, (d) cross section of the wall: 1) cement-lime plaster, external (10 mm); 2) mineral wool (150 mm); 3) glue mortar (5 mm); 4) ceramic brick wall (250 mm); 5) glue mortar (5 mm); 6) gypsum plaster/shives layer, internal (3 mm).
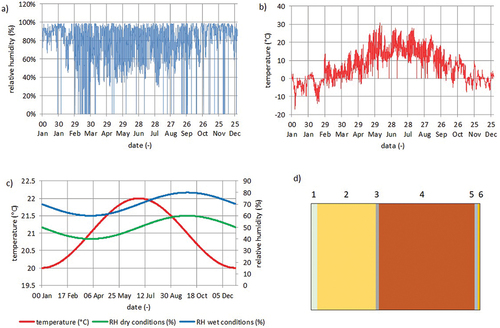
The external boundary conditions were adopted based on the Typical Meteorological Year in Olsztyn, Poland (info on: https://www.gov.pl/web/archiwum-inwestycje-rozwoj/dane-do-obliczen-energetycznych-budynkow). Internal conditions were set as sinusoidal temperature and relative humidity. Temperature in the range of 20–22°C, with a peak at the turn of June/July, while relative humidity in two variants: dry with a minimum of 40% at the turn of February/March, with a peak of 60% in September and humid with a minimum 60% at the turn of February/March, with a peak of 80% in September. The distribution of temperature and humidity is shown in the graph ().
The purpose of the simulation was to compare the accumulation of moisture in the finishing inner layer with a thickness of 3 mm, made of gypsum plaster or shives.
presents the material data used for the simulation purposes. HS and FS properties were investigated by the authors, the specific heat of HS is based on (Nováková Citation2018), while the specific heat of FS is based on (Brachelet et al. Citation2020). Properties of other materials are implemented in the Delphin software.
Table 6. Material properties.
In the first step, a simulation was performed under dry internal conditions (RH range from 40% to 60%). The distribution of accumulated moisture is shown in . The blue color represents gypsum plaster, the green color – hemp shives, while the red color represents the flax shives. The gypsum layer accumulated 17.98–20.04 kg/m3 of moisture, hemp shives 24.05–35.79 kg/m3 of moisture, and the flax shives 23.69–35.25 kg/m3 of moisture. All the analyzed materials demonstrated the ability to absorb moisture during the period of the highest internal humidity in the room. During dry periods, all release moisture into the environment. It is visible that shives absorb more moisture than gypsum plaster. Moisture accumulation in HS is around 1.5% higher than in FS. During dry periods, HS and FS release around 49% of accumulated moisture to the environment, while gypsum plaster only 11%. During the period of the highest humidity, HS and FS absorb 79%, and 76%, respectively, more moisture than gypsum.
Figure 17. Moisture content profiles under dry conditions in the internal finish layer made of gypsum plaster or hemp shives or flax shives.
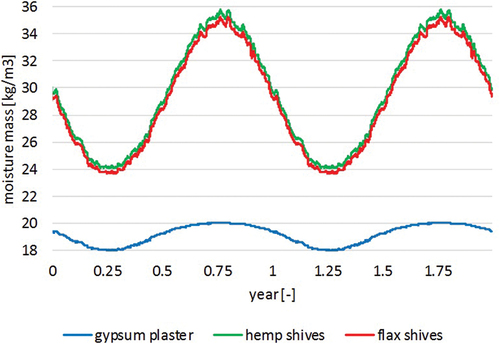
The gypsum layer accumulated 20.04–20.36 kg/m3 of moisture, HS 35.77–56.85 kg/m3 of moisture, and FS 35.23–55.98% of moisture. Moisture accumulation in HS is around 1.5% higher than in FS. All the materials demonstrated the ability to absorb moisture during the period of highest internal humidity in the room. Similarly, in dry conditions (), the materials can absorb moisture in the period of the highest internal relative humidity. In the dry period, they release moisture into the environment, but for gypsum, the annual amplitude does not exceed 2%. HS and FS always accumulate more moisture than gypsum. In the period with lower RH, it is 78% and 76% respectively, and in the highest RH period, it is 179% and 175%, respectively. A comparison of the materials indicates a greater ability of HS and FS to release moisture into the environment with decreasing RH in the room – an annual amplitude of 59%.
The simulation results indicate the possibility of using shives as the inner layer of walls. This can contribute to passively maintaining a high-quality indoor climate. The ambient humidity must not exceed 93%, because, as research has shown, mold growth may begin.
Conclusions
This article presents an experiment-based direct comparison using a consistent methodology to unfold the differences in the properties of flax and hemp shives in the loose form based on the selected moisture, microstructure and microbiological analysis. This paper extends previous research by not only characterizing and comparing the shives but also by simulating their application as internal wall finishing materials. It compares these natural materials against traditional gypsum plaster in terms of moisture buffering capacity, showcasing the potential of flax and hemp shives to improve indoor climate control. A thorough analysis of the obtained results enabled to formulate the following conclusions:
Hemp shives have a lower ability to absorb water through capillary action. After 48 h of testing, they absorbed 56% less water than flax shives. This may be related to the lower bulk density and the higher average pore diameter of hemp shives.
The sorption curves of both hemp and flax shives are similar. The increase in moisture content over the salt solutions with the highest humidity, i.e., 93% and 100%, was visible throughout the research period, while in the case of lower humidity, the stabilization was achieved faster.
Microbiological tests showed that Aspergillus flavus was the dominant mold in flax shive samples. Acremonium and Engyodontium fungi predominated in hemp shive samples. The total number of filamentous fungi in flax shives was 4.41 × 108 CFU/1 g, while in hemp shives it was 2.1 × 107 CFU/1 g. However, the degree of infection is influenced by many factors, e.g. humidity, temperature, and harvest season.
It is recommended to subject both shives, before use, to fumigation or other methods that prevent or limit the multiplication of microorganisms to eliminate their biocorrosion and negative impact on human health.
The microstructure of hemp shives is dominated by pores with an average diameter almost twice as large as the average pore diameter in flax shives. The total porosity of flax and hemp shives is very similar within the range of 77.51–78.70.
The median pore diameter (area) in HS is almost twice as large as in FS and is 0.691 ± 0.144 µm. Large pore diameters in the range of up to 0.6 µm are mainly responsible for capillary transport. Diameters range from approx. 0.6 µm and less for sorption transport. It can be concluded that capillary transport will dominate in hemp fibers. FS median pore diameter (area) was 0.391 ± 0.067 µm. It should also be concluded that materials containing flax fibers will have a higher percentage of sorption transport in total liquid transport than hemp fibers.
Porosity tests confirmed the results from capillary action and sorption tests for both materials in terms of sorption characteristics.
The hygrothermal simulation proved the possibility of using shive wallpapers as a passive way to maintain humidity comfort indoors. During the period of the highest internal humidity, shives absorb moisture, and during drought, they release it to the surroundings. Compared to gypsum plaster, this is a more favorable situation.
The authors see great potential in the application of flax and hemp shives in constructions as insulating and moisture-buffering materials. Perhaps, this is one way to reduce the carbon footprint in the construction sector. However, this requires continued research and identification of further hygrothermal properties regarding heat and mass transport.
Highlights
The first publication that presents comparative studies on the flax and hemp shives themselves.
Both materials are of great potential in the application in constructions as insulating and moisture-buffering materials.
Hemp shives have a lower ability (almost 56%) to absorb water through capillary action than flax shives.
The microstructure of hemp shives is dominated by pores with an average diameter almost twice as large as the average pore diameter in flax shives.
Storing shives too long in a high humidity surrounding may prove to microbiological degradation. Thus, it is recommended to subject shives to fumigation before use.
Disclosure statement
No potential conflict of interest was reported by the author(s).
References
- Arizzi, A., M. Brümmer, I. Martín-Sánchez, E. Molina, and G. Cultrone. 2018. “Optimization of Lime and Clay-Based Hemp-Concrete Wall Formulations for a Successful Lime Rendering.” Construction and Building Materials 184:76–24. https://doi.org/10.1016/j.conbuildmat.2018.06.225.
- Arnaud, L., and E. Gourlay. 2012. “Experimental Study of Parameters Influencing Mechanical Properties of Hemp Concretes.” Construction and Building Materials 28 (1): 50–56. https://doi.org/10.1016/j.conbuildmat.2011.07.052.
- Asdrubali, F., F. D’Alessandro, and S. Schiavoni. 2015. “A Review of Unconventional Sustainable Building Insulation Materials.” Sustainable Materials and Technologies 4:1–17. https://doi.org/10.1016/j.susmat.2015.05.002.
- Balčiunas, G., S. Vejelis, S. Vaitkus, and A. Kairyte. 2013. “Physical Properties and Structure of Composite Made by Using Hemp Hurds and Different Binding Materials.” Procedia Engineering 57:159–166. https://doi.org/10.1016/j.proeng.2013.04.023.
- Barclay, M., N. Holcroft, and A. D. Shea. 2014. “Methods to Determine Whole Building Hygrothermal Performance of Hemp–Lime Buildings.” Building & Environment 80:204–212. https://doi.org/10.1016/j.buildenv.2014.06.003.
- Barnat-Hunek, D., P. Smarzewski, and P. Brzyski. 2017. “Properties of Hemp–Flax Composites for Use in the Building Industry.” Journal of Natural Fibers 14 (3): 410–425. https://doi.org/10.1080/15440478.2016.1212764.
- Benfratello, S., C. Capitano, G. Peri, G. Rizzo, G. Scaccianoce, and G. Sorrentino. 2013. “Thermal and Structural Properties of a Hemp–Lime Biocomposite.” Construction and Building Materials 48:745–754. https://doi.org/10.1016/j.conbuildmat.2013.07.096.
- Benmahiddine, F., R. Cherif, F. Bennai, R. Belarbi, A. Tahakourt, and K. Abahri. 2020. “Effect of Flax Shives Content and Size on the Hygrothermal and Mechanical Properties of Flax Concrete.” Construction and Building Materials 262:120077. https://doi.org/10.1016/j.conbuildmat.2020.120077.
- Bigelow, W. C., D. L. Pickett, and W. A. Zisman. 1946. “Oleophobic Monolayers. I. Films Adsorbed from Solution in Non-Polar Liquids.” Journal of Colloid Science 1 (6). https://doi.org/10.1016/0095-8522(46)90059-1
- Borowska, A. 1986. “Grzyby (Mycota). Tom XVI.” In Grzyby niedoskonałe (Deuteromycetes)/Strzępczakowe (Hyphomycetales)/Ciemnobarwniakowe fialidowe (Dematiaceae Phialoconidiae). Polska Akademia Nauk, Instytut Botaniki, Warszawa – Kraków.
- Brachelet, F., M. Asli, E. Antczak, F. Brue, D. Defer, and A. Lucas. 2020. “Hygrothermal Behaviour of Flax Shives Used as Insulation Material in an Attic of a Traditional House.” MATEC Web of Conferences 307:01017. https://doi.org/10.1051/matecconf/202030701017.
- Brzyski, P., P. Gleń, M. Gładecki, M. Rumińska, Z. Suchorab, and G. Łagód. 2021. “Influence of the Direction of Mixture Compaction on the Selected Properties of a Hemp-Lime Composite.” Materials 14 (16): 4629. https://doi.org/10.3390/ma14164629.
- Brzyski, P., P. Kosiński, A. Zgliczyńska, P. Iwanicki, and J. Poko. 2021. “Mass Transport and Thermal Conductivity Properties of Flax Shives for Use in Construction Industry.” Journal of Natural Fibers 18 (7): 995–1006. https://doi.org/10.1080/15440478.2019.1675216.
- Buick, J. B., and T. R. A. Magee. 1999. “Microbial Contamination of Flax Dust.” Resources, Conservation and Recycling 27 (1–2): 99–104. https://doi.org/10.1016/S0921-3449(98)00089-5.
- Buranov, A. U., and G. Mazza. 2010. “Extraction and Characterization of Hemicelluloses from Flax Shives by Different Methods.” Carbohydrate Polymers 79 (1): 17–25. https://doi.org/10.1016/j.carbpol.2009.06.014.
- Collet, F., J. Chamoin, S. Pretot, and C. Lanos. 2013. “Comparison of the Hygric Behaviour of Three Hemp Concretes.” Energy and Buildings 62:294–303. https://doi.org/10.1016/j.enbuild.2013.03.010.
- Csanády, D., and B. Nagy. 2023. “Development of a Fire-Resistant Straw- and Waste Glass-Based Thermal Insulation Board.” RILEM Bookseries 45:885–898. https://doi.org/10.1007/978-3-031-33465-8_68.
- de Hoog, G. S., J. Guarro, J. Gené, A. Figueras, S. Al-Hatmi, A. M. S., M. J. Figueras, and R. G. Vitale. 2019. Atlas of Clinical Fungi Online. 3rd ed. Reus, Utrecht. http://www.clinicalfungi.org/.
- Delhomme, F., A. Hajimohammadi, A. Almeida, C. Jiang, D. Moreau, Y. Gan, X. Wang, and A. Castel. 2020. “Physical Properties of Australian Hurd Used As Aggregate for Hemp Concrete.” Materials Today Communications 24:100986. https://doi.org/10.1016/j.mtcomm.2020.100986.
- Dubois, V., A. Leblanc, O. Carpentier, G. Alhaik, and E. Wirquin. 2018. “Performances of Flax Shive-Based Lightweight Composites with Rapid Hardening.” Construction and Building Materials 165:17–27. https://doi.org/10.1016/j.conbuildmat.2017.12.239.
- Essid, S., V. J. Hegde, A. Mahieu, L. Bizet, N. Leblanc, and A. Saouab. 2021. “Comparison of the Properties of Flax Shives Based Particleboards Prepared Using Binders of Bio-Based Lignin and Partially Bio-Based Epoxy Resin.” International Journal of Adhesion and Adhesives 109:102915. https://doi.org/10.1016/j.ijadhadh.2021.102915.
- Fassatiova, O. 1983. “Microscopic Fungi in Technical Microbiology.” Wydawnictwo Naukowo-Techniczne, Warszawa (In Polish).
- Fuentes, C. A., K. Beckers, H. Pfeiffer, L. Q. N. Tran, C. Dupont-Gillain, I. Verpoest, and A. W. Van Vuure. 2014. “Equilibrium Contact Angle Measurements of Natural Fibers by an Acoustic Vibration Technique.” Colloids and Surfaces A: Physicochemical and Engineering Aspects 455 (1): 164–173. https://doi.org/10.1016/j.colsurfa.2014.04.054.
- Gerlach, D. 1972. Basics of Botanical Microtechnique. Warszawa: PWRiL.
- Górny, R. L., A. Ławniczek-Wałczyk, A. Stobnicka, M. Gołofit-Szymczak, and M. Cyprowski. 2015. “Włokna Jako Nośniki Cząstek Mikrobiologicznych.” Medycyna Pracy 66 (4): 511–523. https://doi.org/10.13075/mp.5893.00231.
- Jan, K., and J. Wyrwał. 2010. “Podstawy fizyki materiałów budowlanych.” In Budownictwo ogólne t-2, edited by J. Kubik and J. Wyrwał, 10–52. Warszawa: Arkady. In Polish.
- Jang, E. S., C. Won Kang, and S. Sik Jang. 2018. “Comparison of the Mercury Intrusion Porosimerty, Capillary Flow Porometry and Gas Permeability of Eleven Species of Korean Wood.” Journal of the Korean Wood Science and Technology 46 (6): 681–691. https://doi.org/10.5658/WOOD.2018.46.6.681.
- Jiang, Y., M. P. Ansell, X. Jia, A. Hussain, and M. Lawrence. 2017. “Physical Characterisation of Hemp Shiv: Cell Wall Structure and Porosity.” 2nd International Conference on Bio-Based Building Materials, no. April, Clermont-Ferrand, France.
- Jiang, Y., M. Lawrence, M. P. Ansell, and A. Hussain. 2018. “Cell Wall Microstructure, Pore Size Distribution and Absolute Density of Hemp Shiv.” Royal Society Open Science 5 (4): 171945. https://doi.org/10.1098/rsos.171945.
- Johansson, S., K. Balksten, and P. Brigitte Strandberg-de Bruijn. 2022. “Risk for Mould Growth on Hemp-Lime at Different Relative Humidity.” Bio-Based Building Materials 1:588–594. https://doi.org/10.4028/www.scientific.net/cta.1.588.
- Kosegarten, C. E., N. Ramírez-Corona, E. Mani-López, E. Palou, and A. López-Malo. 2017. “Description of Aspergillus Flavus Growth Under the Influence of Different Factors (Water Activity, Incubation Temperature, Protein and Fat Concentration, PH, and Cinnamon Essential Oil Concentration) by Kinetic, Probability of Growth, and Time-To-Detection Models.” International Journal of Food Microbiology 240:115–123. https://doi.org/10.1016/j.ijfoodmicro.2016.04.024.
- Kosiński, P., P. Brzyski, and B. Duliasz. 2020. “Moisture and Wetting Properties of Thermal Insulation Materials Based on Hemp Fiber, Cellulose and Mineral Wool in a Loose State.” Journal of Natural Fibers 17 (2): 199–213. https://doi.org/10.1080/15440478.2018.1477086.
- Kosiński, P., P. Brzyski, M. Tunkiewicz, Z. Suchorab, D. Wiśniewski, and P. Palczyński. 2022. “Thermal Properties of Hemp Shives Used as Insulation Material in Construction Industry.” Energies 15 (7): 2461. https://doi.org/10.3390/en15072461.
- Kosiński, P., B. Kask, M. Franus, M. Piłat-Rożek, J. Szulżyk-Cieplak, and G. Łagód. 2023. “The Possibility of Using Sewage Sludge Pellets as Thermal Insulation.” Advances in Science and Technology Research Journal 17 (2): 161–172. https://doi.org/10.12913/22998624/159724.
- Kymäläinen, H. R., M. Nykter, M. Hautala, and A. Maija Sjöberg. 2005. “Hygienic Quality of Stem Fractions of Mechanically Processed Fibre Hemp and Linseed.” Agricultural and Food Science 14 (2): 143. https://doi.org/10.2137/145960605774825993.
- Łapka, P., P. Brzyski, K. Pietrak, Ł. Cieślikiewicz, and Z. Suchorab. 2023. “Hygro-Thermal Characterization of the Hemp Concrete Modified with the Gum Arabic Admixture.” Construction and Building Materials 368:130392. https://doi.org/10.1016/j.conbuildmat.2023.130392.
- Maalouf, C., A. D. T. Le, S. B. Umurigirwa, M. Lachi, and O. Douzane. 2014. “Study of Hygrothermal Behaviour of a Hemp Concrete Building Envelope Under Summer Conditions in France.” Energy and Buildings 77:48–57. https://doi.org/10.1016/j.enbuild.2014.03.040.
- Magniont, C. 2010. “Contribution à La Formulation et à La Caractérisation d’un Écomatériau de Construction à Base d’agroressources.” Laboratoire Navier - UMR 8205 PhD (572).
- Mandeel, Q. A. 2005. “Fungal Contamination of Some Imported Spices.” Mycopathologia 159 (2): 291–298. https://doi.org/10.1007/s11046-004-5496-z.
- Marceau, S., P. Glé, M. Guéguen-Minerbe, E. Gourlay, S. Moscardelli, I. Nour, and S. Amziane. 2017. “Influence of Accelerated Aging on the Properties of Hemp Concretes.” Construction and Building Materials 139:524–530. https://doi.org/10.1016/j.conbuildmat.2016.11.129.
- Marrot, L., K. Candelier, J. Valette, C. Lanvin, B. Horvat, L. Legan, and D. B. DeVallance. 2022. “Valorization of Hemp Stalk Waste Through Thermochemical Conversion for Energy and Electrical Applications.” Waste and Biomass Valorization 13 (4): 2267–2285. https://doi.org/10.1007/s12649-021-01640-6.
- Nováková, P. 2018. “Use of Technical Hemp in the Construction Industry.” MATEC Web of Conferences 146:03011. https://doi.org/10.1051/matecconf/201814603011.
- Nykter, M., H. Riitta Kymäläinen, A. Belinda Thomsen, H. Lilholt, H. Koponen, A. Maija Sjöberg, and A. Thygesen. 2008. “Effects of Thermal and Enzymatic Treatments and Harvesting Time on the Microbial Quality and Chemical Composition of Fibre Hemp (Cannabis Sativa L.).” Biomass and Bioenergy 32 (5): 392–399. https://doi.org/10.1016/j.biombioe.2007.10.015.
- O’Brien, F. E. M. 1948. “The Control of Humidity by Saturated Salt Solutions.” Journal of Scientific Instruments 25 (3): 73–76. https://doi.org/10.1088/0950-7671/25/3/305.
- Pekhtasheva, E., A. Neverov, S. Kubica, and G. Zaikov. 2012. “Biodegradation and Biodeterioration of Some Natural Polymers.” Chemistry and Chemical Technology 6 (3): 263–280. https://doi.org/10.23939/chcht06.03.263.
- Perrone, G., A. Susca, G. Cozzi, K. Ehrlich, J. Varga, J. C. Frisvad, M. Meijer, P. Noonim, W. Mahakamchanakul, and R. A. Samson. 2007. “Biodiversity of Aspergillus Species in Some Important Agricultural Products.” Studies in Mycology 59:53–66. https://doi.org/10.3114/sim.2007.59.07.
- Plötze, M., and P. Niemz. 2011. “Bestimmung der Porosität und Porengrößenverteilung verschiedener Hölzer mittels Quecksilberdruckporosimetrie.” European Journal of Wood and Wood Products 69 (4): 649–657. https://doi.org/10.1007/s00107-010-0504-0.
- Pundiene, I., L. Vitola, J. Pranckeviciene, and D. Bajare. 2022. “Hemp Shive-Based Bio-Composites Bounded by Potato Starch Binder: The Roles of Aggregate Particle Size and Aspect Ratio.” Journal of Ecological Engineering 23 (2): 220–234. https://doi.org/10.12911/22998993/144637.
- Qi, C., S. Hou, J. Lu, W. Xue, and K. Sun. 2020. “Thermal Characteristics of Birch and Its Cellulose and Hemicelluloses Isolated by Alkaline Solution.” Holzforschung 74 (12): 1099–1112. https://doi.org/10.1515/hf-2019-0285.
- Rahim, M., O. Douzane, A. D. Tran Le, G. Promis, B. Laidoudi, A. Crigny, B. Dupre, and T. Langlet. 2015. “Characterization of Flax Lime and Hemp Lime Concretes: Hygric Properties and Moisture Buffer Capacity.” Energy & Buildings 88:91–99. https://doi.org/10.1016/j.enbuild.2014.11.043.
- Schellbach, S. L., S. N. Monteiro, and J. W. Drelich. 2016. “A Novel Method for Contact Angle Measurements on Natural Fibers.” Materials Letters 164:599–604. https://doi.org/10.1016/j.matlet.2015.11.039.
- Schwarzova, I., N. Stevulova, and T. Melichar. 2017. “Lightweight Composites Based on Technical Hemp Hurds in Construction Industry.” Chemical Engineering Transactions 57:1369–1374. https://doi.org/10.3303/CET1757229.
- Serrano, S., D. Ürge-Vorsatz, C. Barreneche, A. Palacios, and L. F. Cabeza. 2017. “Heating and Cooling Energy Trends and Drivers in Europe.” Energy 119:425–434. https://doi.org/10.1016/j.energy.2016.12.080.
- Shen, X., P. Jiang, D. Guo, G. Li, F. Chu, and S. Yang. 2021. “Effect of Furfurylation on Hierarchical Porous Structure of Poplar Wood.” Polymers 23 (1): 32. https://doi.org/10.3390/polym13010032.
- Silveira, V. D. C., M. Martins Pinto, and F. Simon Westphal. 2019. “Influence of Environmental Factors Favorable to the Development and Proliferation of Mold in Residential Buildings in Tropical Climates.” Building and Environment 166:106421. https://doi.org/10.1016/j.buildenv.2019.106421.
- Stevulova, N., L. Kidalova, J. Cigasova, J. Junak, A. Sicakova, and E. Terpakova. 2013. “Lightweight Composites Containing Hemp Hurds.” Procedia Engineering 65:69–74. https://doi.org/10.1016/j.proeng.2013.09.013.
- Strandberg-de Bruijn, P., and P. Johansson. 2014. “Moisture Transport Properties of Lime–Hemp Concrete Determined Over the Complete Moisture Range.” Biosystems Engineering 122:31–41. https://doi.org/10.1016/j.biosystemseng.2014.03.001.
- Taggart Arthur, F., T. C. Taylor, and C. R. Ince. 1930. “Experiments with Flotation Agents.” Transactions of the American Institute of Mining, Metallurgical, and Petroleum Engineers (87): 285–386.
- Tamborski, Z. 2009. “Wpływ temperatury na przebieg izoterm sorpcji wody przez cukier.” Żywność Nauka Technologia Jakość 5 (66): 72–82. In Polish.
- Tran Le, A. D., C. Maalouf, T. H. Mai, E. Wurtz, and F. Collet. 2010. “Transient Hygrothermal Behaviour of a Hemp Concrete Building Envelope.” Energy and Buildings 42 (10): 1797–1806. https://doi.org/10.1016/j.enbuild.2010.05.016.
- Véronique.CEREZO. 2005. “Propriétés Mécaniques, Thermiques et Acoustiques d’un Matériau à Base de Particules Végétales : Approche Expérimentale et Modélisation Théorique.” INSA, LYON. Thèse Doctorale, no. June 2005.
- Walker, R., and S. Pavía. 2014. “Moisture Transfer and Thermal Properties of Hemp–Lime Concretes.” Construction and Building Materials 64:270–276. https://doi.org/10.1016/j.conbuildmat.2014.04.081.
- Walker, R., S. Pavia, and R. Mitchell. 2014. “Mechanical Properties and Durability of Hemp-Lime Concretes.” Construction and Building Materials 61:340–348. https://doi.org/10.1016/j.conbuildmat.2014.02.065.
- Washburn, E. W. 1921. “The Dynamics of Capillary Flow.” Physical Review 17 (3): 273–283. https://doi.org/10.1103/PhysRev.17.273.
- Wilhelmy, L. 1863. “Ueber Die Abhängigkeit Der Capillaritäts-Constanten Des Alkohols von Substanz Und Gestalt Des Benetzten Festen Körpers.” Annalen der Physik 195 (6). https://doi.org/10.1002/andp.18631950602.
- Wójcik, R., A. Panuś, M. Tunkiewicz, and M. Hamdy. 2017. “Influence of Chemical Damp Proof Cream on the Capillary Action and Microstructure of Mortars.” Energy Procedia 132:670–675. https://doi.org/10.1016/j.egypro.2017.10.004.
- You, S., W. Li, T. Ye, F. Hu, and W. Zheng. 2017. “Study on Moisture Condensation on the Interior Surface of Buildings in High Humidity Climate.” Building & Environment 125:39–48. https://doi.org/10.1016/j.buildenv.2017.08.041.
- Yousefi, Y., and F. Tariku. 2021. “Thermal Conductivity and Specific Heat Capacity of Insulation Materials at Different Mean Temperatures.” Journal of Physics: Conference Series 2069 (1): 012090. https://doi.org/10.1088/1742-6596/2069/1/012090.
- Yuan, Y., and T. R. Lee. 2013. “Contact Angle and Wetting Properties.” In Surface Science Techniques, edited by G. Bracco and B. Holst, 3–34. Berlin: Springer Berlin Heidelberg.
- Zvirgzds, K., E. Kirilovs, S. Kukle, and U. Gross. 2022. “Production of Particleboard Using Various Particle Size Hemp Shives As Filler.” Materials 15 (3): 886. https://doi.org/10.3390/ma15030886.